Open Access
ARTICLE
Carbon Abatement Cost-Sharing Strategy for Electric Power Sector Based on Incentive and Subsidy Mechanisms
College of Economics and Management, Shanghai University of Electric Power, Shanghai, 200090, China
* Corresponding Author: Wen Wang. Email:
Energy Engineering 2024, 121(10), 2907-2935. https://doi.org/10.32604/ee.2024.052665
Received 10 April 2024; Accepted 12 July 2024; Issue published 11 September 2024
Abstract
The green and low carbon transition and development of the electricity industry is the most crucial task in realizing the “dual-carbon target”, and it is urgent to explore the incentive and subsidy mechanism to promote green electricity consumption and the cost-sharing strategy of carbon reduction, to alleviate the pressure of carbon abatement cost of each subject of the electricity supply chain. Against this background, this paper takes into account the low-carbon subsidies provided by the government and the incentive subsidies for users, and studies the optimal decision-making of each subject in the electricity supply chain, so that each of them can obtain the optimal profit and achieve carbon emission reduction at the same time. Firstly, taking into account the direct power purchase mode of large users and the electricity-selling companies emerging after the reform of the power sales side, we have established a cooperative mechanism for sharing the cost of carbon emission reduction in the electricity supply chain and clarified the relationship between the supply and demand of electricity among the main parties. Subsequently, considering government low-carbon subsidies and user incentive subsidies, the optimal decision-making model is established under two scenarios of decentralized and centralized cooperative games in the supply chain, respectively, with the objective of maximizing profits and carbon reduction rates. Solving for the optimal proportion of carbon abatement costs shared by each participant in the electricity supply chain in achieving game equilibrium. Finally, we analyze the role of the government’s low-carbon subsidies, users’ incentive subsidies, and other factors on the profit and carbon reduction effect of the electricity industry through the example analysis and further analyze the impact of carbon abatement cost-sharing measures to provide recommendations for the electricity industry to realize low-carbon abatement and make decisions.Keywords
Nomenclature
Carbon abatement rate of power producer (%) | |
Level of incentive subsidies for electricity selling company, i.e., the rate of increase in electricity sales after the incentive subsidy (%) | |
Impact coefficient of power users’ low-carbon preference on the demand (MW·h) | |
The ratio of large users who prefer the direct mode of electricity supply (%) | |
Initial market demand (MW·h) | |
The cost coefficient of the incentive subsidy (RMB) | |
Impact coefficient of incentive subsidy of regular users (MW·h) | |
Impact coefficient of the incentive subsidy of the large industrial and commercial users (MW·h) | |
Profit earned from selling units of electricity to regular users (RMB·(MW·h)−1) | |
Profit earned by generator selling units of electricity to electricity selling company (RMB·(MW·h)−1) | |
Profit earned by generator selling units of electricity to large industrial and commercial users (RMB·(MW·h)−1) | |
The market price of each unit of carbon emission (RMB·t−1 CO2) | |
Free carbon emission allowance granted by the government (t CO2) | |
Carbon abatement cost coefficient (RMB) | |
Initial carbon emissions per unit of electricity (t CO2·(MW·h)−1) | |
The ratio of government subsidies for carbon emission reduction inputs by power producer (%) | |
The ratio of incentive subsidy costs shared by generators (%) | |
The ratio of carbon abatement costs shared by electricity selling company (%) | |
Profit of electricity selling company (RMB) | |
Profit of power producer (RMB) | |
Total profit of power producer and electricity selling company (RMB) |
Since the industrial revolution, global warming and other environmental problems have become increasingly severe [1]. China has taken over the high-pollution and high-energy-consumption industries of many developed countries and, at the same time, faces the problems of ecological deterioration, declining competitiveness of high-carbon-emission products and energy security [2]. Against this background, China is reducing carbon emissions from the power sector, currently the most significant single carbon-emitting industry, through initiatives such as establishing a carbon trading market [3]. In July 2023, the Central Committee for Comprehensively Deepening Reforms considered and passed the Guidelines for Deepening Reform of the Electric Power System and Accelerating the Construction of a New Electric Power System, which puts clean and low carbon at the top of the list. Exploring the relevant incentive mechanisms in the power industry and implementing carbon abatement cost sharing among the subjects of the electricity supply chain, which are closely related to each other, is conducive to reducing the pressure on the cost of carbon reduction of each subject and realizing a low-carbon transition in the power sector [4].
1.1 Research on the Electricity Supply Chain Cost-Sharing
How to reasonably transfer and share costs among various subjects in the power supply chain has been a concern of scholars, and cost-sharing strategies have a wide range of applications in the electricity industry. Herbert et al. [5], in their study on the Impact of cost-sharing strategies on the economics of small wind power mini-grid projects in Kenya, found that how to allocate costs to different types of users affects their level of participation and whether they get the desired benefits. Khoddami et al. [6] investigated the critical role of a cost-sharing strategy in the efficiency and profitability of the biomass power supply chain. Uski et al. [7] proposed a cost-sharing strategy related to energy storage systems to balance the interests of various participants in a study of alternative microgrid investments. He et al. [8] proposed a cost-sharing methodology to solve the problem of heat and power supply. This method was developed during research aimed at enhancing the energy utilization efficiency of cogeneration. He et al. [9] also suggested apportioning the FM auxiliary service costs based on the “initiator pays” principle. This recommendation was made to study costs triggered by the joint operation of generating units and loads. Based on the theory of Carbon Emission Flow (CEF), Bian et al. [10] proposed a source-load carbon responsibility factor, which apportions the responsibility of carbon emission to both sources and loads, including new energy sources. Perrault et al. [11–13] discussed the role of Shapley’s value theory in cost-sharing among the subjects of the electricity supply chain from different perspectives, respectively. Lin et al. [14] constructed an ex-post cost-sharing mechanism to allocate each renewable energy producer’s share of responsibility in the total system cost according to the weight of the consequences of the imbalance in the power system caused by the producer. Brown et al. [15] implemented a cost-sharing strategy to facilitate cost control when conducting distributed energy projects.
In addition, more scholars study the cost-sharing strategy among the subjects in the electric power industry by constructing a game model. He et al. [16] constructed a distributed photovoltaic power cost-sharing game model to realize the optimal sharing of power generation costs among power producers and users. Xu et al. [17,18] proposed a cost-sharing model based on cooperative game theory to study the cost allocation among the government, users, and power enterprises. Chen et al. [19] established a cooperative game cost-sharing model and compared the bilateral carbon liability-sharing mechanism with the unilateral carbon liability burdening mechanism for generators or users. An et al. [20] solved the transaction problem among multiple players of regional integrated energy by establishing a cooperative game model. They used Shapley value for cost-sharing among various players. Existing literature primarily focuses on sharing carbon abatement costs between power producers and users. Still, it ignores the carbon abatement costs shared between power supply chain subjects such as power producers, electricity-selling companies, power users, etc. This paper examines the issue of cost-sharing of carbon reduction in the power sector from this perspective.
1.2 Research on the Supply Chain Cost-Sharing under Carbon Cap and Trade Policies
Under the carbon cap-and-trade policy, there are many studies on joint carbon emission reduction decisions among multiple actors in the supply chain. The electricity supply chain is a kind of supply chain, and the relevant research results have significant reference value for realizing a low-carbon decrease in the power supply chain. Wu et al. [21] found that upstream enterprises tend to set higher carbon decrease rates and need to bear higher carbon abatement costs by establishing four Stackelberg models that consider different free carbon quota allocation schemes and carbon abatement cost-sharing schemes. Xu et al. [22] found that both wholesale prices and carbon reduction cost-sharing strategies play a role in supply chain coordination with optimal production (optimal abatement), first decreasing (increasing) and then remaining constant as the carbon trading price increases. Wang et al. [23,24] modelled independent and joint emission reduction in a two-level supply chain. They found that the implementation of cost-sharing strategies can improve the level of carbon emission reduction and supply chain profits. Ma et al. [25] used a differential game model to analyze the role of carbon abatement strategies for each subject in the cold chain. They found that a two-way cost-sharing strategy is more effective than a one-way cost-sharing strategy. Users’ low-carbon preferences affect demand for commodities. Ghosh et al. [26,27] verified that carbon abatement cost-sharing could potentially improve economic and environmental benefits considering consumers’ low-carbon preference. Wu et al. [28] analyzed the impacts of consumers’ low-carbon preference and carbon abatement cost-sharing ratio on the profits of each subject in the supply chain. In addition to cost-sharing strategies, scholars have also studied benefit-sharing strategies. Yang et al. [29–31] analyzed the impacts of retailers’ profit-sharing and cost-sharing strategies on manufacturers’ implementation of carbon abatement measures. They showed that the two strategies are beneficial to the improvement of the overall efficiency of the supply chain and the decrease in manufacturers, and both the manufacturers and retailers prefer the incentive strategy with a higher level of cooperation. The study of Yi et al. [32] proved that government subsidies and carbon tax policies are conducive to promoting the synergy of supply chain actors for energy saving and emission reduction. In the electricity supply chain, Chen et al. [33], in studying the impacts of the benchmarking mechanism on each subject, found that the investment in carbon reduction technology increases with the increase of the unit carbon quota and the adoption of the benefit-sharing strategy and the cost-sharing strategy can promote the coordination of the electricity supply chain [34].
1.3 Research on the Electricity Supply Chain Incentives
When each subject in the power supply chain assumes responsibility for carbon emission reduction, multi-party cooperation among the subjects can further promote the power industry to realize the “dual carbon” target and profit growth. At the same time, it is essential to explore scientific and reasonable incentive mechanisms. Currently, the primary way to guide users in carrying out demand response (DR) is to adjust the price of electricity or take compensation means to guide users in actively making load transfer or load reduction behaviour. For example, Luo et al. [35] constructed a model of power sales company and power sales contract selection based on logit theory. They analyzed the Impact of each type of power sales contract on the power sales company. Muratori et al. [36] quantitatively assessed the Impact of time-of-use tariff strategies of electricity-selling companies on customer demand response behaviour. Lu et al. [37,38] proposed a dynamic pricing DR algorithm that considers both the profits and costs of power users and the selling companies in a tiered electricity market. Dey et al. [39] proposed incentive tariffs to increase the margins of electricity-selling companies by considering the different utility and demand elasticities of various types of users during peak and trough periods. Electricity-selling companies set dynamic subsidy prices based on the incentive-based demand response algorithm proposed by Jiang et al. [40] to reduce costs. The dynamic tariff incentive proposed by Bejan et al. [41] reduced consumer surplus by about half compared to the time-of-use (TOU) incentive. In contrast, the pricing scheme proposed by Dewangan et al. [42] took into account peak-to-average ratios for each interval of TOU pricing, avoiding possible load accumulation during low-priced hours and lowering customer costs. Li et al. [43] proposed a two-tier scheduling model for regional microgrids to balance interests between microgrids and consumers through a real-time pricing mechanism. Guo et al. [44] constructed an incentive-based demand response master-slave game model between electricity sellers and users. Chai et al. [45,46] considered the utility and demand elasticity of the users. They changed the users’ demand through incentive pricing to maximize the benefits for both the electricity-selling company and the user. Zhang et al. [47] proposed a framework for multiple vendors to jointly establish incentive-based demand response cooperation for the same distributed data centre cluster. Tsaousoglou et al. [48] proposed a personalized real-time pricing mechanism that considers user satisfaction, minimizes generation costs and fairness, and uses energy cost savings as an incentive subsidy for responsive users. Xu et al. [49] constructed a comprehensive demand response management mechanism, including incentive-based demand response management by the grid company to the electricity-selling company and price-based demand response management by the electricity-selling company to large industrial and commercial users. Dai et al. [50] concluded that government subsidies for electricity sellers and user incentives under the integrated demand response mechanism could promote renewable energy consumption and reduce carbon emissions. However, the current study only considers incentivizing user demand scheduling behaviour, ignoring the realization of the dual-carbon goal and the wide application of renewable energy, which is crucial in achieving the dual-carbon goal. Although China is actively promoting the development of green power, the proportion of green power transactions in the electricity market remains to be improved due to its high price, cyclicality and uncertainty. Power sales companies take positive incentives to encourage users to consume green power, which, on the one hand, is conducive to maximizing their profits; on the one hand, promoting the development and growth of green power is conducive to the realization of the energy green low-carbon transition, and then take the lead in the power industry to achieve the dual-carbon goals.
Power producers need to invest more in carbon reduction to cope with the government’s increasingly stringent carbon emission control policies. At the same time, electricity-selling companies must pay for incentives and subsidies when adopting attractive green power marketing strategies. Generators and sales companies share the same goal of promoting customers’ consumption of green power and thus achieving profit growth. Carbon emission reduction cost sharing is an essential way for power producers and electricity-selling companies to carry out in-depth cooperation, which is conducive to reducing the cost pressure of each subject to achieve common goals and has become an essential issue for developing the power industry.
Because of previous related studies, the paper explores the optimization of carbon abatement cost-sharing strategies for each participant in the electricity supply chain under the incentive subsidy mechanism based on two scenarios. Firstly, we consider the decentralized decision-making of carbon abatement cost-sharing for each subject in the electricity supply chain with or without government low-carbon subsidy and incentive subsidy cost-sharing. Then, the centralized decision-making of carbon abatement cost-sharing of each subject in the power supply chain is established under the government’s low-carbon subsidy and mutual sharing of carbon abatement cost between the power producer and power-selling company, and the optimal carbon abatement cost-sharing ratio between the two parties is derived. Through example analysis, we study the impacts of changes in parameters such as government low-carbon subsidy ratio, incentive subsidy cost coefficient and carbon abatement cost coefficient on profits and carbon abatement rate, and further analyze the impacts of carbon reduction cost-sharing ratio on the profits of the electricity industry.
2 Cooperative Gaming Strategies Considering Carbon Reduction Cost-Sharing
This paper considers four leading players in the electricity market: Power producers, electricity-selling companies, large industrial and commercial users, and regular users. Power producers are responsible for electricity production, and electricity-selling companies are responsible for selling electricity. Electricity can be sold directly to industrial and commercial power users by power producers through direct power purchase mode on the one hand and sold to industrial and commercial power users and regular users through electricity-selling companies on the other hand. Considering the Impact of government decisions on the electricity market, the government guides power producers to reduce CO2 emissions through carbon market trading, free carbon emission quotas and carbon abatement subsidies. To incentivize power users to consume green power, the electricity-selling company adopts price incentives for power users, thus incurring the cost of incentive subsidies. The cooperative mechanism for sharing the cost of carbon emission reduction in the electricity supply chain is shown in Fig. 1.
Figure 1: Cooperative mechanism for carbon abatement cost sharing in the electricity supply chain
The proposal of “No. 1439” promotes the entry of all industrial and commercial large users into the electricity market and abolishes the industrial and commercial catalogue tariffs, and the multiplication of users will bring more development space to the power sales company [51]. At the same time, to reduce costs, industrial and commercial users will further seek to purchase electricity directly from power producers. In January 2024, the government department issued the Notice on Strengthening the Interface between Green Power Certificates and Energy Saving and Carbon Reduction Policies and Greatly Promoting the Consumption of Non-Fossil Energy; the notice points out that we need to accelerate the establishment of high-energy-consuming enterprises mandatory consumption of renewable energy mechanism, the use of green certificates to eliminate the carbon footprint. The “dual carbon” target not only requires power producers to increase their carbon abatement rate and the share of green power in the electricity market but also requires users to increase their consumption of green power. The government has provided some free carbon emission quotas and carbon abatement subsidies to power producers to promote the increase of the carbon abatement rate by power producers. A cooperative game is formed between power producers and power selling companies, whereby power producers share the cost of incentive subsidies for power sales companies, reducing the cost pressure on power sales companies and increasing the level of incentive subsidies for power sales companies. Electricity sales companies share part of the carbon emission reduction costs of power generation companies to encourage power generation companies to increase their carbon reduction rates. An increase in the rate of carbon reduction by the power generator and the level of incentive subsidies offered by the electricity-selling company can improve environmental benefits on the one hand and enable incremental market benefits for the power generator and electricity-selling company on the other. The incremental market benefits received by the power producer and the electricity-selling company constitute the overall cooperative surplus, distributed as cost subsidies to each other.
In order to explore the impacts of incentive subsidy and carbon abatement cost-sharing strategy on the profit and carbon emission of the electric power industry, we constructed a decentralized cooperative game model and a centralized cooperative game model. By comparing the results under different assumptions, we gradually analyze the roles of incentive subsidies and carbon abatement cost-sharing strategies and finally obtain the optimal carbon abatement cost-sharing ratio.
3 Carbon Abatement Cost-Sharing Strategies for Decentralized Cooperative Gaming in Electricity Supply Chains
3.1 Emission Reduction Strategies without Low Carbon Subsidies and without Incentive Subsidy Cost-Sharing
When the government does not subsidize the power producer, the electricity-selling company independently bears the cost of the incentive subsidy. The power producer uses the carbon abatement rate (
The revenue function of the electricity-selling company is shown below:
where
The profit function for power producers is as follows:
where
Since
From Eq. (3), we have
According to the first-order optimality condition, the optimal solution for the carbon abatement rate of the power producer can be obtained as follows:
where
3.2 Emission Reduction Strategies with Low Carbon Subsidies and No Incentive Subsidy Cost-Sharing
In a scenario where the government provides low-carbon subsidies to power producers, the electricity-selling company independently bears the cost of the incentive subsidy. It is assumed that the government’s share of the carbon abatement costs of power producers is
The revenue function of the electricity-selling company is shown below:
The profit function for power producers is as follows:
The optimal solutions for the level of incentive subsidy for the selling company and the carbon abatement rate of the power producer are solved as follows:
3.3 Emission Reduction Strategies that Combine Low-Carbon Subsidies with Cost-Sharing of Incentive Subsidies
When government authorities provide low-carbon subsidies to the generator, the generator shares the cost of incentive subsidies paid by the electricity-selling company to customers. It is assumed that the proportion of the cost of the incentive subsidy that the power producer shares in the cost of the incentive subsidy to the selling company is
The revenue function of the electricity-selling company is shown below:
The profit function for power producers is as follows:
Since
At this point, the determinant of the power producer’s profit function concerning the Hessian matrix of
When
From Eqs. (14) and (15), the optimal solution for the carbon reduction rate of the power producer as follows:
The power producers’ share of the cost of the incentive subsidy to the selling company is:
The optimal solution for the level of incentive subsidy for the selling company is as follows:
4 Carbon Abatement Cost-Sharing Strategies for Centralized Cooperative Gaming in Electricity Supply Chains
4.1 Centralized Decision-Making That Integrates Low-Carbon and Incentive Subsidies
The electricity supply chain aims to maximize profits when government authorities provide low-carbon subsidies to generators and electricity-selling companies provide incentive subsidies to consumers.
The function of total profit in the electricity supply chain is:
Similar to the solution for
By comparing the results under the three scenarios of decentralized cooperative gaming emission reduction decision-making with the results obtained under centralized decision-making, it can be concluded that: (1)
4.2 Carbon Abatement Cost-Sharing Strategies for Cooperative Games Combining Low-Carbon Subsidies and Incentive Subsidies
When government authorities provide low-carbon subsidies to power producers and electricity-selling companies provide subsidy incentives to customers, power producers and electricity-sales companies share the cost of carbon reduction to maximize the total profit of the supply chain in a cooperative game. Assuming that the government subsidizes the carbon abatement costs of the power producer at a rate of
The revenue function of the electricity-selling company is shown below:
The profit function for power producers is as follows:
Taking the first-order partial derivative with respect to
Taking the first-order partial derivative with respect to
According to the first-order optimality conditions, the optimal solutions for the level of incentive subsidy of the electricity-selling company and the carbon abatement rate of the power producer are, respectively:
When the generator and the electricity-selling company achieve equilibrium in the game, the optimal solution can reach the level of the ideal solution for centralized decision-making, there are
The incentive subsidy cost-sharing ratio for generators is:
5.1 Example Hypothesis and Parameter Settings
This paper assumes that the potential demand for electricity (
Under the relevant parameter settings, the profit and carbon abatement rate of each subject of the power supply chain under five scenarios are solved, and the optimal solution results are shown in Table 1.
Table 1 shows that without incentive cost sharing, the government’s sharing of the cost of carbon reduction by power producers has a significant effect on the increase in the rate of carbon emission reductions by power producers, which shows that the government’s subsidy measures play a positive role in reducing carbon emissions. In the meantime, the profitability of all supply chain actors has increased. When the government subsidizes carbon reduction, adopting incentive cost-sharing measures significantly increases the optimal level of incentive subsidies, while the optimal carbon abatement rate and the profits of the electricity-selling company and the power producer increase. In the decentralized decision with government subsidies and incentive cost-sharing, the optimal carbon reduction rate, optimal incentive subsidy level, and overall profit are not as good as in the centralized decision. However, they are higher than in the other decentralized decisions. The main reason is that power sales companies and power generators do not aim to maximize the overall profit of the power supply chain when they make their own independent decisions, and the optimality of each subject does not achieve the overall optimality. With government low-carbon subsidies and incentive subsidy mechanisms in place and viewing the electricity-selling company and the power producer as an integral part of the electricity supply chain, the optimal carbon abatement rate, the optimal level of incentive subsidies, and the total profit of the electricity industry are all maximized by information exchanging and joint participation in decision-making between the parties. Power sales companies and power producers engage in cooperative gaming. In the case of maximizing the overall benefits of the supply chain, they can further obtain the proportion of carbon emission reduction costs that both parties share.
5.3.1 Impact of Each Parameter on Total Profit
It can be seen from (a) in Fig. 2 that the total profit of power producers and electricity-selling companies is inversely related to the market carbon trading price. The production operations of power producers mainly cause the cost of carbon emission expenditure. When the carbon emissions of power producers exceed the free carbon credits issued by the government, they must purchase additional carbon credits. The increase in carbon trading price in the market leads to a rise in carbon expenditure cost and a decrease in overall profit. Therefore, power producers should improve the carbon reduction rate and reduce carbon emissions by increasing investment in technology research, development, and other initiatives.
Figure 2: Impact of each parameter on total profit. (a) Effect of
As seen from (b) in Fig. 2, the total profit of power producers and electricity-selling companies are positively related to the low-carbon preference of users. With the establishment and development of the mandatory renewable energy consumption mechanism for high energy-consuming enterprises, power users are required to increase the proportion of green power consumption. At the same time, industrial and commercial users are actively improving their consumption of green electricity to create a green corporate brand image, enhance the export competitiveness and international competitiveness of their products, and eliminate their carbon footprint with green certificates. Therefore, the government should further improve the mandatory consumption mechanism of renewable energy and actively publicize the green, low-carbon concept to enhance users’ awareness of low-carbon production and life. The increase in profit is conducive to power producers increasing carbon abatement investment and realizing a virtuous cycle.
As seen from (c) and (d) in Fig. 2, when the electricity-selling company expands the demand of ordinary customers and large industrial and commercial customers through the adoption of incentive and subsidy measures, it significantly increases the total profit. Among them, incentives and subsidies to regular users of demand caused by profit growth than the Impact of large commercial and industrial users of demand caused by profit growth. The reason is that the time of production activities and electricity consumption of large commercial and industrial users is more fixed than that of regular users, and changes in production activity plans are more complicated. Regular users have substantial flexibility.
As seen from (e) in Fig. 2, the total profit is positively related to government subsidies to power producer’s research and development (R&D) investment in carbon abatement technologies, and the growth of total profit becomes more prominent with the increase of subsidies. The government subsidizes the carbon abatement initiatives of power producers, which, on the one hand, reduces the carbon abatement cost expenditures of power producers. On the other hand, the carbon abatement initiatives increase the supply of green power and promote users’ consumption of green energy. The total profit increases significantly under the combined effect of cost reduction and revenue increase.
As seen from (f) in Fig. 2, the total profit is inversely related to the carbon abatement cost coefficient. When
As seen from (g) in Fig. 2, the electricity-selling companies’ total profit and the cost coefficient of incentive subsidies become an inverse change relationship. Similar to the trend in the role of carbon abatement cost coefficients on total profits, the decline in total profit flattened from a significant decrease as the incentive subsidy cost coefficient increased. When the users’ green power consumption capacity tends to be saturated, the electricity-selling company needs to pay more to incentivize users to consume green power, making the total profit decline.
In summary, total profit is lowest without government low-carbon subsidies and without cost-sharing of incentive subsidies. Government low-carbon subsidies to power producers, power producers’ share of the cost of incentive subsidies to electricity-selling companies, and electricity-selling companies’ share of the cost of power producers’ R&D investment in carbon-emission-reducing technologies are all practical measures to increase total profits, with the first two having a more significant effect on total profits. The government can provide economic subsidies and other favourable policies to support the power sector in realizing a low-carbon transition, which will help the electric power industry to improve total profits. When the power producer shares the cost of incentive subsidies from the electricity-selling company, the level of incentive subsidies from the electricity-selling company increases significantly, which promotes the consumption of green electricity by users, thus enabling the power producer to realize profit growth. At the same time, power producers realize profit growth, increase carbon abatement investments, and increase the supply of green power, resulting in a virtuous cycle. The initiative of power sales companies to share the carbon emission reduction costs of power producers has not been particularly effective due to the low profitability of power sales companies.
In contrast, the carbon abatement costs of power producers are enormous, and the ability of power-selling companies to share them is limited. Analysis of the three ways to help the power industry increase its total profit shows that, with government subsidies and policy support, it should expand users’ demand for green power through incentives to subsidize users, low-carbon environmental protection publicity for users, and other initiatives. The profits should be invested in carbon reduction initiatives such as technology research and development, thus realizing a virtuous cycle of profit growth.
5.3.2 Impact of Each Parameter on Carbon Reduction Rates
It can be seen from (a) in Fig. 3 that the carbon abatement rate is positively correlated with the market carbon trading price in each case. That is, as the market carbon trading price rises, the carbon abatement rate of the power producer also rises. On the one hand, as the market price of carbon trading increases and the government provides limited free carbon emission credits, power producer needs to pay more for carbon emissions beyond the credits, and the fundamental way to reduce the cost of carbon emissions is to increase the rate of carbon reduction. On the other hand, with the relaunch of China’s certified emission reductions (CCERs) and the launch of the “Specialized Study on Expanding the Industry Coverage of the National Carbon Market”, carbon quota trading will become more flexible and efficient. Power producers will be able to increase their carbon abatement rates and sell excess carbon allowances for profit. The increase in the rate of change in the carbon abatement rate with the growth of the market price of carbon trading is more considerable and more effective after the government subsidizes power producers to provide carbon abatement technology inputs. The electricity-selling company shares the carbon abatement costs of the power producer, and the power producer shares the incentive subsidy costs of the electricity-selling company; both measures also contribute to further increasing the rate of change.
Figure 3: Impact of each parameter on carbon reduction rate. (a) Effect of
It can be seen from (b) in Fig. 3 that the carbon abatement rate is positively correlated with the coefficient of influence of users’ low-carbon preference on demand in all five cases. The larger the coefficient of influence of users’ low-carbon preference on demand, the higher the carbon abatement rate of power producers. The enhancement of low-carbon and environmental protection awareness of power users, the establishment of the mandatory consumption mechanism of renewable energy for high-energy-consuming enterprises, and the creation of a green corporate brand image by industrial and commercial users have all put forward higher requirements for the proportion of green power consumption. It will force power producers to increase the carbon reduction rate and reduce carbon emissions.
It can be seen from (c) and (d) in Fig. 3 that when the electricity-selling company does not incentivize subsidies to power users, the growth of the demand impact factor of the electricity-selling company’s incentive subsidies to power users does not change the power producer’s carbon reduction rate. When the electricity-selling company provides incentive subsidies to power users, the carbon abatement rate of the power producer increases as the demand impact coefficient of the electricity-selling company’s incentive subsidies on users grows. The electricity-selling company provides incentive subsidies to users, increasing their green electricity consumption. It increases the power producer’s profit, providing more funds for investment in carbon abatement initiatives and resulting in lower carbon emissions.
It can be seen from (e) in Fig. 3 that government subsidies positively affect increasing carbon reduction rates. When the proportion of government subsidies for low-carbon technology R&D investment of power producers rises, the carbon reduction rate also increases, and the magnitude of change is apparent. Carbon abatement initiatives have problems with significant investment, long cycles, and slow effects, leading to power producers’ low willingness to invest entirely on their own. The government’s increased subsidies for power producers’ carbon abatement input costs in the early stage can increase the motivation of power producers to reduce carbon emissions and increase the carbon abatement rate. Due to the low carbon preference of the users, the power producer to improve the carbon abatement rate can promote the consumption of green power, thereby increasing profit. Then, increase the carbon abatement input, forming a virtuous cycle.
It can be seen from (f) in Fig. 3 that when the electricity-selling company does not take incentive subsidies for users, the carbon abatement rate is independent of the incentive subsidy coefficient. When the electricity-selling company gives incentive subsidies to users, carbon abatement first rapidly declines with the increase of the incentive subsidy cost coefficient. Then, the decline rate tends to level off. The rise in the incentive subsidy cost coefficient leads to the increase in the cost of the power-selling company, the willingness of the electricity-selling company to take incentive subsidy measures decreases, the green power consumption of the user decreases, and the profit of the power producer decreases, which reduces the carbon abatement input. At this time, the power producer shares the cost of incentive subsidies of the electricity-selling company, which can reduce the cost pressure of the electricity-selling company and increase the green power consumption of users. The electricity-selling company needs to explore more efficient and lower-cost incentive subsidy methods to reduce the cost factor of incentive subsidies, to increase the profit of the electricity-selling company and power producer, and thus increase the rate of carbon reduction.
It can be seen from (g) in Fig. 3 that the carbon reduction rate and carbon reduction cost coefficient become inverse relationship. As the carbon abatement cost coefficient increases, the carbon abatement rate decreases, and the rate of change shows a trend of first fast and then slow. The increase in the carbon abatement cost coefficient will increase the abatement cost of power producers and reduce the willingness to invest in carbon reduction. The government’s policy guidance and cost subsidies and the sharing of carbon abatement costs by power companies are conducive to reducing the costs of power producers, which in turn maintains the continuous investment in carbon reduction and improves the carbon abatement rate.
To summarize, the inverse factor that causes the enormous change in carbon abatement rate is the coefficient of carbon abatement cost of power producers. The government subsidizes the carbon abatement cost of the power producers, the power producer shares the cost of incentive subsidies from the electricity-selling companies to power users, and the electricity-selling company shares the carbon abatement cost of the power producers are All practical measures to increase the carbon abatement rate. And the government’s subsidy to the carbon abatement cost of power producers has the most pronounced effect. Therefore, the most effective way to increase the carbon abatement rate is to improve the investment in innovation and effectively reduce the carbon reduction cost coefficient with the support of government subsidies.
5.3.3 Impact of Parameters on Cost-Sharing Ratios
It can be seen from (a) in Fig. 4 the proportion of carbon abatement cost shared by the electricity selling company to the power producer changes negatively with the carbon trading price. As the carbon trading price rises, the abatement cost of the power producer increases, and the proportion of costs shared by the electricity-selling company that the power producer can bear decreases significantly.
Figure 4: Impact of parameters on cost-sharing ratios (a) Effect of
It can be seen from (b) in Fig. 4 that the proportion of carbon abatement cost shared by electricity-selling companies with power producers is negatively related to the proportion of low-carbon subsidies provided by the government to power producers. The government’s increase in the proportion of low-carbon subsidies for power producers reduces the pressure of carbon emission reduction subsidies on power producers. Accordingly, the weighting of the power-selling company in sharing the pressure of emission reduction costs on power producers decreases.
It can be seen from (c) and (d) in Fig. 4 that when the demand impact factor of the incentive subsidy on the customer increases, the share of the carbon abatement cost of the electricity selling company to the electricity producer decreases. When the incentive subsidy to the customer increases the company’s cost, the company will reduce its share of the carbon abatement cost of the power producer in order to ensure its interests.
It can be seen from (e) in Fig. 4 the proportion of carbon abatement cost shared by the electricity selling company to the power producer changes positively with the carbon abatement cost coefficient. When the carbon abatement cost coefficient of the power producer increases, the carbon abatement cost of the power producer increases. The carbon reduction cost-sharing ratio first rises rapidly to reduce the pressure of carbon abatement costs on power producers. Then, it tends to flatten out because it gradually approaches the maximum value that the electricity-selling company can bear.
It can be seen from (f) in Fig. 4 that the proportion of carbon abatement costs shared by electricity-selling companies with power producers varies positively with the coefficient of influence of users’ low-carbon preference on demand. When users prefer to consume more green power, the electricity-selling company can make a profit from it and then hope that the power producer can increase the carbon reduction rate. The electricity-selling company’s incentive to share the power producer’s carbon reduction cost will increase.
It can be seen from (g) in Fig. 4 that the generator’s share of the cost of incentive subsidies to the electricity-selling company decreases when the coefficient of Impact of incentive subsidies on customer demand increases. When the incentive subsidy initiative has a significant effect, the electricity selling company increases the subsidy, which leads to an increase in the cost of the incentive subsidy, and the generator reduces its share of the cost of the incentive subsidy.
It can be seen from (h) in Fig. 4 that when the incentive subsidy cost factor increases, the generator’s share of the cost of the incentive subsidy to the selling company decreases, but to a lesser extent. Thus, the effect of incentive subsidies on customer demand plays a significant role in the decline in the incentive subsidy cost share.
5.3.4 Impact of Carbon Abatement Cost-Sharing Ratio on Profitability
As seen in Fig. 5, the profit of the electricity-selling company is negatively correlated with the proportion of the electricity-selling company’s share of the power producer’s abatement cost (
Figure 5: Impact of
As seen from Fig. 6, with the increase of the share of incentive costs from the power producer to the electricity-selling company and the share of carbon abatement costs from the electricity-selling company to the power producer, the profit of the power producer, in general, shows a tendency of increasing and then decreasing. The incremental profit of the power producer mainly comes from the increase in green power consumption brought about by the rise in the incentive level. When
Figure 6: Impact of
As seen in Fig. 7, the total profit has the same trend as the optimal profit of the power producer, showing a trend of increasing first and then decreasing because the power producer occupies a dominant position in the market and obtains the main profit. When the power producer shares 93.70% of the incentive subsidy cost of the electricity-selling company, and the electricity-selling company shares 1.20% of the carbon abatement cost of the power producer, the total profit reaches the maximum value of RMB 2,121,962.70. The total profit is RMB 2,062,565.80 when there is a government carbon abatement subsidy, but the power producer and electricity-selling company do not share their respective costs. The initiative of sharing carbon abatement costs brings an increase of about 2.90% to the total profit, which verifies the initiative’s effectiveness.
Figure 7: Impact of
This paper constructs a cooperation game model between power producers and electricity-selling companies, taking into account the government’s low carbon subsidies for power producers and incentive subsidies for power users by electricity-selling companies, and derives the optimal carbon abatement rate and optimal incentive subsidy level in different scenarios. It analyzes the Impact of factors such as market carbon trading price, carbon abatement cost coefficient, and users’ low-carbon preference on the power supply chain’s total profit and carbon abatement rate. The influence of relevant parameters on the cost-sharing ratio is explored, and the cost-sharing ratio of carbon emission reduction between the two parties is further clarified to put forward suggestions for carbon emission reduction cooperation among various subjects of the power supply chain.
The study results show that subsidies provided by government authorities to electricity providers and the sharing of carbon abatement costs between power producers and electricity-selling companies are effective measures to increase the carbon abatement rate, which can reduce carbon emissions and increase the total profit of the electricity supply chain. Therefore, on the one hand, the government can adjust the market carbon trading price and subsidy ratio to encourage power producers to increase carbon abatement investment and further improve the mandatory consumption mechanism of renewable energy and the green certificate to eliminate the carbon footprint mechanism. On the other hand, power producers and electricity-selling companies can increase the carbon abatement rate and profit by reasonably sharing the cost of carbon reduction. The government and the power industry should strengthen low-carbon and environmental protection publicity so that power users can understand and improve the consumption of green power.
When power generators and electricity sales companies cooperate under the carbon emission reduction cost-sharing cooperation mechanism, the government should supervise and coordinate. For example, many emerging electricity sales companies are private enterprises facing insufficient capital strength and credit; the government should investigate and manage them and adopt policies to support well-run electricity sales companies, such as credit endorsement and financial support, to ensure that the cost-sharing agreement is fair and mutually beneficial. In addition, the carbon emission reduction cost-sharing cooperation mechanism also faces related risks and challenges. The actual power generation and consumption of market players generally deviate from the contracted amount of power, and the medium- and long-term market cannot accurately and clearly define the carbon emission reduction responsibilities of market players in the context of the still imperfect power spot market mechanism. Due to livelihood policy constraints, such as supporting general industrial and commercial users and maintaining the primary stability of residential electricity prices, there is still room to explore the potential for the user side to assume responsibility for carbon emission reduction.
Currently, this paper has not further subdivided the power generators into conventional power generators and renewable energy generators in the modelling, has not studied the green certificate trading mechanism among the power supply chain, and has not considered the competition and cooperation between multiple power generators and power selling companies, nor has it considered the participation of cross-province and cross-region foreign power in the power market trading. Therefore, using the green certificate trading mechanism, our following research explores the involvement of multiple power generators, power sales companies, and external power suppliers in the electricity market. We will consider as many primitive factors as possible when constructing the model equations to be closer to reality, such as the increased cost of new energy installation, equipment upgrading and modification costs, etc. At the same time, the change in users’ demand is not well considered when constructing the model. The following research step will build a user demand function closer to reality to solve the problem. After considering many factors, we will further explore each subject’s carbon emission reduction cost-sharing strategy in the electricity supply chain.
Acknowledgement: The authors are grateful to Congcong Wang and Meiping Huang for their insightful discussions.
Funding Statement: This work was supported by the Project of Philosophy and Social Science Foundation of Shanghai, China (Grant No. 2020BGL011).
Author Contributions: The authors confirm contribution to the paper as follows: Study conception and design: Hui Wang, Wen Wang., Wenhui Zhao; data collection: Wen Wang; analysis and interpretation of results: Hui Wang, Wen Wang; draft manuscript preparation: Wen Wang. All authors reviewed the results and approved the final version of the manuscript.
Availability of Data and Materials: The authors confirm that the data supporting the findings of this study are available within the article.
Ethics Approval: Not applicable.
Conflicts of Interest: The authors declare that they have no conflicts of interest to report regarding the present study.
References
1. M. Hasanuzzaman, U. S. Zubir, N. I. Ilham, and H. S. Che, “Global electricity demand, generation, grid system, and renewable energy policies: A review,” Wires. Energy Environ., vol. 6, no. 3, pp. 639–647, May–Jun. 2017. doi: 10.1002/wene.222. [Google Scholar] [CrossRef]
2. S. H. Wang, Z. W. Yang, W. Zhang, and S. L. Zhang, “A review of carbon emission literature under the “dual carbon” background: Based on the visualization of scientific knowledge mapping and the combing of development context,” (in Chinese), Ecol. Econ., vol. 39, no. 3, pp. 222–229, 2023. [Google Scholar]
3. H. D. Jiang, Q. R. Yang, and K. Y. Dong, “Economic-energy-environmental impact of low-carbon transformation policies in China’s power industry,” (in Chinese), CPREE, vol. 32, no. 6, pp. 30–40, 2022. [Google Scholar]
4. J. L. Li, M. Ho, C. P. Xie, and N. Stern, “China’s flexibility challenge in achieving carbon neutrality by 2060,” Renew Sust. Energ. Rev., vol. 158, pp. 112, Apr. 2022. doi: 10.1016/j.rser.2022.112112. [Google Scholar] [CrossRef]
5. C. Herbert and E. Phimister, “Private sector-owned mini-grids and rural electrification: A case study of wind-power in Kenya’s tea industry,” Energy Policy, vol. 132, pp. 1288–1297, Sep. 2019. doi: 10.1016/j.enpol.2019.06.031. [Google Scholar] [CrossRef]
6. S. Khoddami, F. Mafakheri, and Y. Zeng, “A system dynamics approach to comparative analysis of biomass supply chain coordination strategies,” Energies, vol. 14, no. 10, pp. 2808, May 2021. doi: 10.3390/en14102808. [Google Scholar] [CrossRef]
7. S. Uski, K. Forssén, and J. Shemeikka, “Sensitivity assessment of microgrid investment options to guarantee reliability of power supply in rural networks as an alternative to underground cabling,” Energies, vol. 11, no. 10, pp. 2831, Oct. 2018. doi: 10.3390/en11102831. [Google Scholar] [CrossRef]
8. Y. X. He, T. Xia, B. Wang, W. Xiong, and J. H. Wang, “Novel cogeneration cost-sharing mechanism for energy savings and emission reduction in China,” Int. J. Global Warm, vol. 6, no. 1, pp. 58–78, Jan. 2014. doi: 10.1504/IJGW.2014.058755. [Google Scholar] [CrossRef]
9. Y. X. He, L. Zhou, Y. X. Pang, Z. Y. Fei, S. T. Li and X. Gao, “Design of frequency auxiliary service cost-sharing mechanism based on triggered responsibility under the new electricity reform,” (in Chinese), Autom. Electr. Power Syst., vol. 43, no. 18, pp. 88–94, Aug. 2019. [Google Scholar]
10. X. Y. Bian, S. Wu, J. Zhao, L. Xu, B. Zhou and S. F. Lin, “Multi-level flexibility resource planning for new power systems considering source-load carbon responsibility sharing,” (in Chinese), Autom. Electr. Power Syst., vol. 44, no. 2, pp. 155–164, Apr. 2024. [Google Scholar]
11. A. Perrault and C. Boutilier, “Approximately stable pricing for coordinated purchasing of electricity,” in Proc. IJCAI, Buenos Aires, Argentina, Jul. 2015, pp. 25–31. [Google Scholar]
12. Q. Zhou, D. H. Feng, C. B. Xu, C. Feng, T. Sun and T. Ding, “Comparative study of carbon emission responsibility direct sharing methods on the load side,” (in Chinese), Autom. Electr. Power Syst., vol. 39, no. 17, pp. 153–159, Sep. 2015. [Google Scholar]
13. J. Gu, M. H. You, and K. L. Wu, “Integrated energy service provider trading strategy considering carbon emission sharing,” (in Chinese), Electr. Power Autom. Equip., vol. 44, no. 5, pp. 11–18, Nov. 2024. [Google Scholar]
14. W. Lin and E. Bitar, “Forward electricity markets with uncertain supply: Cost sharing and efficiency loss,” in Proc. the IEEE 53rd CDC, Los Angeles, CA, USA, Dec. 2014, pp. 15–17. [Google Scholar]
15. D. Brown and D. Sappington, “Optimal procurement of distributed energy resources,” Energ. J., vol. 39, no. 5, pp. 131–155, Sep. 2018. doi: 10.5547/01956574.39.5.dbro. [Google Scholar] [CrossRef]
16. H. Y. He, H. Q. He, and J. Wang, “Distributed photovoltaic generation cost sharing considering carbon footprint and trading,” (in Chinese), Elect. Power Construct., vol. 41, no. 6, pp. 85–92, Jun. 2020. [Google Scholar]
17. Y. Xu, “Comprehensive benefit analysis and cost sharing study of regional electricity substitution,” (in ChineseM.S. thesis, Kunming Univ. of Sci. and Tech., Kunming, China, 2020. [Google Scholar]
18. X. Luo, “Cost sharing study on distributed photovoltaic systems for residential buildings under the constraints of different regional resources in China,” Ph.D. dissertation, Xi’an Univ. of Architecture and Technology, Xi’an, China, 2017. [Google Scholar]
19. L. X. Chen, T. Sun, Y. Zhou, C. Zhou, C. Fang and D. H. Feng, “Power system generation and load side joint carbon responsibility sharing method,” (in Chinese), Autom. Electr. Power Syst., vol. 42, no. 19, pp. 106–111, Aug. 2018. [Google Scholar]
20. E. An, Q. Sun, and D. Ma, “Research on integrated energy regional trading strategy based on cooperative game,” in Proc. the 31st Chinese Control and Decision Conf., Nanchang, China, Jun. 2019. [Google Scholar]
21. P. Wu, Y. Yin, S. Li, and Y. L. Huang, “Low-carbon supply chain management considering free emission allowance and abatement cost sharing,” Sustainability, vol. 10, no. 7, pp. 2110, Jul. 2018. doi: 10.3390/su10072110. [Google Scholar] [CrossRef]
22. X. Xu, P. He, H. Xu, and Q. P. Zhang, “Supply chain coordination with green technology under cap-and-trade regulation,” Int. J. Prod. Econ., vol. 183, no. 3, pp. 433–442, Jan. 2017. doi: 10.1016/j.ijpe.2016.08.029. [Google Scholar] [CrossRef]
23. Z. Wang, A. Brownlee, and Q. Wu, “Production and joint emission reduction decisions based on two-way cost-sharing contract under cap-and-trade regulation,” Comput. Ind. Eng., vol. 146, no. 3, pp. 106549, Aug. 2020. doi: 10.1016/j.cie.2020.106549. [Google Scholar] [CrossRef]
24. A. Taleizadeh, S. Niaki, and N. Alizadeh-Basban, “Cost-sharing contract in a closed-loop supply chain considering carbon abatement, quality improvement effort, and pricing strategy,” RAIRO-Oper. Res., vol. 55, pp. S2181–S2219, Mar. 2021. doi: 10.1051/ro/2020072. [Google Scholar] [CrossRef]
25. X. Ma, Y. Zhao, Q. Luo, and Q. G. Bai, “Preservation technology investment and carbon abatement strategies in a supplier-retailer cold chain based on a differential game,” Comput. Ind. Eng., vol. 172, pp. 108540, Oct. 2022. doi: 10.1016/j.cie. [Google Scholar] [CrossRef]
26. D. Ghosh and J. Shah, “Supply chain analysis under green sensitive consumer demand and cost sharing contract,” Int. J. Prod. Econ., vol. 164, no. 10, pp. 319–329, Jun. 2015. doi: 10.1016/j.ijpe.2014.11.005. [Google Scholar] [CrossRef]
27. J. Shi, W. Jiao, K. Jing, Q. Yang, and K. K. Lai, “Joint economic-environmental benefit optimization by carbon-abatement cost sharing in a capital-constrained green supply chain,” Processes, vol. 11, no. 1, pp. 226, Jan. 2023. doi: 10.3390/pr11010226. [Google Scholar] [CrossRef]
28. D. Wu and Y. Yang, “The low-carbon supply chain coordination problem with consumers’ low-carbon preference,” Sustainability, vol. 12, no. 9, pp. 3591, Apr. 2020. doi: 10.3390/su12093591. [Google Scholar] [CrossRef]
29. H. Yang and W. Chen, “Retailer-driven carbon emission abatement with consumer environmental awareness and carbon tax: Revenue-sharing versus cost-sharing,” Omega-Int. J. Manage. S., vol. 78, no. SI, pp. 179–191, Jul. 2018. doi: 10.1016/j.omega.2017.06.012. [Google Scholar] [CrossRef]
30. K. Xue, G. Sun, and T. Yao, “Incentive mechanisms for carbon emission abatement considering consumers’ low-carbon awareness under cap-and-trade regulation,” Int. J. Env. Res. Pub. He., vol. 19, no. 7, pp. 4104, Apr. 2022. doi: 10.3390/ijerph19074104. [Google Scholar] [PubMed] [CrossRef]
31. Q. Bai, J. Xu, and Y. Zhang, “Emission reduction decision and coordination of a make-to-order supply chain with two products under cap-and-trade regulation,” Comput. Ind. Eng., vol. 119, no. 1, pp. 131–145, May 2018. doi: 10.1016/j.cie.2018.03.032. [Google Scholar] [CrossRef]
32. Y. Yi and J. Li, “Cost-sharing contracts for energy saving and emissions reduction of a supply chain under the conditions of government subsidies and a carbon tax,” Sustainability, vol. 10, no. 3, pp. 895, Mar. 2018. doi: 10.3390/su10030895. [Google Scholar] [CrossRef]
33. W. Chen, Y. Ma, and W. Hu, “Electricity supply chain coordination with carbon abatement technology investment under the benchmarking mechanism,” J. Ind. Manag. Optim., vol. 18, no. 2, pp. 713–730, Mar. 2022. doi: 10.3934/jimo.2020175. [Google Scholar] [CrossRef]
34. W. Chen, H. Wang, and J. He, “Research on electricity supply chain strategy coordination considering peak-valley pricing policy and service quality investment,” RAIRO-Oper. Res., vol. 56, no. 2, pp. 583–599, Mar. 2022. doi: 10.1051/ro/2022020. [Google Scholar] [CrossRef]
35. Q. Luo and Y. Q. Song, “Marketing strategy considering interruptible load in the electricity market,” (in Chinese), Autom. Electr. Power Syst., vol. 39, no. 17, pp. 134–139, Sep. 2015. [Google Scholar]
36. M. Muratori and G. Rizzoni, “Residential demand response: Dynamic energy management and time-varying electricity pricing,” IEEE Trans. Power Syst., vol. 31, no. 2, pp. 1108–1117, Mar. 2016. doi: 10.1109/TPWRS.2015.2414880. [Google Scholar] [CrossRef]
37. R. Lu, S. Hong, and X. Zhang, “A dynamic pricing demand response algorithm for smart grid: Reinforcement learning approach,” Appl. Energy., vol. 220, no. 1, pp. 220–230, Jun. 2018. doi: 10.1016/j.apenergy.2018.03.072. [Google Scholar] [CrossRef]
38. R. Lu, S. Hong, and M. Yu, “Demand response for home energy management using reinforcement learning and artificial neural network,” IEEE Trans. Smart Grid, vol. 10, no. 6, pp. 6629–6639, Nov. 2019. doi: 10.1109/TSG.2019.2909266. [Google Scholar] [CrossRef]
39. B. Dey, S. Misra, and F. Marquez, “Microgrid system energy management with demand response program for clean and economical operation,” Appl. Energy., vol. 334, no. 8, pp. 120717, Mar. 2023. doi: 10.1016/j.apenergy.2023.120717. [Google Scholar] [CrossRef]
40. H. Jiang and Y. F. Wang, “Incentive demand response for residential users based on online learning,” (in Chinese), Adv. Technol. Electr. Eng. Energy, vol. 42, no. 6, pp. 23–33, Jun. 2023. [Google Scholar]
41. I. Bejan, C. L. Jensen, L. M. Andersen, and L. G. Hansen, “Inducing flexibility of household electricity demand: The overlooked costs of reacting to dynamic incentives,” Appl. Energy, vol. 284, no. 3, pp. 116283, Feb. 2021. doi: 10.1016/j.apenergy.2020.116283. [Google Scholar] [CrossRef]
42. C. Dewangan, S. Singh, S. Chakrabarti, and K. Singh, “Peak-to-average ratio incentive scheme to tackle the peak-rebound challenge in TOU pricing,” Electr Pow. Syst. Res., vol. 210, no. 4, pp. 108048, Sep. 2022. doi: 10.1016/j.epsr.2022.108048. [Google Scholar] [CrossRef]
43. Y. Li, K. Li, Z. Yang, Y. Yu, R. Xu and M. Yang, “Stochastic optimal scheduling of demand response-enabled microgrids with renewable generations: An analytical-heuristic approach,” J. Clean. Prod., vol. 330, pp. 129840, Nov. 2022. doi: 10.1016/j.jclepro.2021.129840. [Google Scholar] [CrossRef]
44. K. J. Guo, C. W. Gao, G. Y. Lin, S. X. Lu, and X. F. Xiao, “Optimal design of electricity plans for active demand response of power demand side,” (in Chinese), Autom. Electr. Power Syst., vol. 44, no. 15, pp. 28–35, May 2020. [Google Scholar]
45. Y. X. Chai, Y. Xiang, J. Y. Liu, C. H. Gu, W. T. Zhang and W. T. Xu, “Incentive-based demand response model for maximizing benefits of electricity retailers,” J. Mod. Power Syst. Clean Energy, vol. 7, no. 6, pp. 1644–1650, Nov. 2019. doi: 10.1007/s40565-019-0504-y. [Google Scholar] [CrossRef]
46. J. Hou, X. Liu, J. Yu, Z. Z. Lin, Y. Li and Z. Zhang, “Optimal design of electricity plans for active demand response of power demand side,” in Proc. the 2019 IEEE 3rd Conf. Energ. Internet and Energy Syst. Integrat., Changsha, China, Nov. 2019, pp. 8–10. [Google Scholar]
47. P. Zhang, P. Yang, and Z. Zhao, “A framework for several electricity retailers cooperatively implementing demand response to distributed data center,” IEEE Trans. Smart Grid, vol. 14, no. 1, pp. 277–289, Jan. 2023. doi: 10.1109/TSG.2022.3189835. [Google Scholar] [CrossRef]
48. G. Tsaousoglou, N. Efthymiopoulos, P. Makris, and E. Varvarigos, “Personalized real time pricing for efficient and fair demand response in energy cooperatives and highly competitive flexibility markets,” J. Mod. Power Syst. Cle., vol. 1, no. 1, pp. 151–162, Jan. 2019. doi: 10.1007/s40565-018-0426-0. [Google Scholar] [CrossRef]
49. B. Xu, X. M. Yue, Y. Guan, Z. Y. Hou, and Z. P. Fan, “Hybrid electricity demand response strategy for ‘peak-valley inversion’ of electricity load,” (in Chinese), Syst. Eng. Theory Pract., vol. 42, no. 8, pp. 2129–2138, Apr. 2022. [Google Scholar]
50. Y. M. Dai, Y. L. Gao, H. Yin, and X. Feng, “Comprehensive demand response strategy for electricity market considering retailer’s renewable energy subsidy,” (in Chinese), Chin J. Manag. Sci., vol. 24, no. 41, pp. 1–21, Mar. 2022. [Google Scholar]
51. H. Wang, C. Wang, and W. Zhao, “Decision on mixed trading between medium- and long-term markets and spot markets for electricity sales companies under new electricity reform policies,” Energies, vol. 15, no. 24, Dec. 2022. doi: 10.3390/en15249568. [Google Scholar] [CrossRef]
52. C. Che, H. X. Zheng, X. Geng, Y. Chen, and X. G. Zhang, “Research on carbon emission reduction investment decision of power energy supply chain-based on the analysis of carbon trading and carbon subsidy policies,” Energies, vol. 15, no. 17, pp. 6151, Jul. 2022. doi: 10.3390/en15176151. [Google Scholar] [CrossRef]
Cite This Article
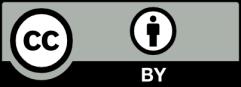
This work is licensed under a Creative Commons Attribution 4.0 International License , which permits unrestricted use, distribution, and reproduction in any medium, provided the original work is properly cited.