Open Access
REVIEW
A Comprehensive Review of Design and Technological Advancements across Various Types of Solar Dryers
School of Mechanical Engineering, VIT Bhopal University, Sehore, 466114, India
* Corresponding Authors: Ganesh There. Email: ; Rohit Sharma. Email:
Energy Engineering 2024, 121(10), 2851-2892. https://doi.org/10.32604/ee.2024.049506
Received 09 January 2024; Accepted 09 July 2024; Issue published 11 September 2024
Abstract
This analysis investigates the widespread use of solar drying methods and designs in developing countries, particularly for agricultural products like fruits, vegetables, and bee pollen. Traditional techniques like hot air oven drying and open sun drying have drawbacks, including nutrient loss and exposure to harmful particles. Solar and thermal drying are viewed as sustainable solutions because they rely on renewable resources. The article highlights the advantages of solar drying, including waste reduction, increased productivity, and improved pricing. It is also cost-effective and energy-efficient. The review study provides an overview of different solar drying systems and technologies used in poor nations, aiming to identify the most effective and efficient designs. The focus is on comparing current models of solar dryers for optimal performance. The review underscores the importance of solar drying as a long-term, eco-friendly approach to drying food in developing countries. This review aims to evaluate how using solar-powered drying techniques can enhance food preservation, minimize waste, and enhance the quality and marketability of agricultural goods. The paper will specifically focus on examining the efficacy of these methods for drying bee pollen and pinpointing where enhancements can be made in their advancement.Graphic Abstract
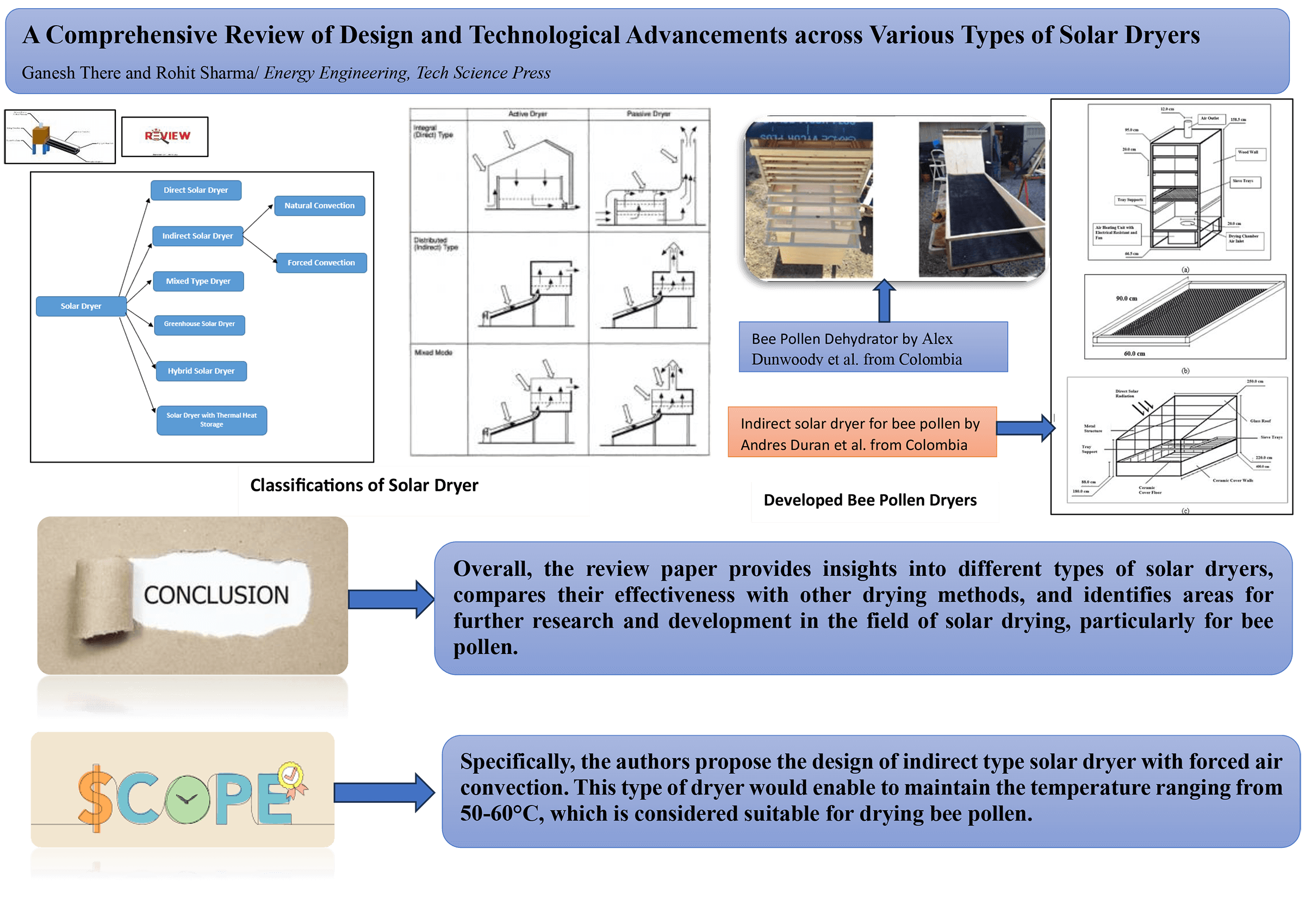
Keywords
The growing population poses a major challenge to preserving agricultural food and products to meet the increasing demand. While food management and drying are important strategies to address this issue, there are other alternatives available. The Food and Agricultural Organization (FAO) recognizes the significance of food management and drying in reducing food waste and losses [1]. Proper food management techniques involve handling, storing, processing and distributing food to prevent waste and spoilage. By drying food, it can be preserved for longer periods and hinder the growth of bacteria, reducing the likelihood of food decay [2]. Ensuring the maintenance of nutritional parameters in agricultural products throughout the post-harvest process is indeed crucial to minimize losses and preserve the quality of the food until it reaches the consumer. Various steps are followed after harvesting to achieve this goal, including collection, grading, sorting, bagging, storage, shipping, and conservation [3]. According to the latest available data on agricultural produce, it is estimated that 20% of food products are lost, and in some regions such as Asia and Africa the level of spoilage can be as high as 45%–50% [4]. These losses can occur due to various factors, including inadequate storage conditions, improper handling, and transportation issues. Post-harvest damages can occur due to mechanical and physical conditions and the growth of fungi and bacteria [5]. Moisture content plays a significant role in the spoilage of agricultural products. More moisture percentage can support the growth of bacteria and fungi, leading to spoilage. Therefore, it is important to store moisture-sensitive products in properly designed thermal storage facilities to control and maintain the appropriate moisture levels [6,7]. Solar drying is indeed an efficient and sustainable method of preserving food and maintaining the nutritional quality of agriculturally based products. By harnessing the power of the sun, solar dryers can reduce the moisture content of crops, which is a crucial step in preserving them [8]. In contrast to artificial dryers that rely on electricity or non-renewable fuels, solar dryers offer a more accessible and cost-effective solution, particularly in remote areas where grid-based electricity and other energy sources may be scarce or unaffordable for small farmers [9,10]. These dryers require an initial investment for installation but have lower running costs, making them more suitable for farmers in developing countries like India. Dried fruits, vegetables, and other agricultural products are essential sources of vitamins, nutrients, proteins, carbohydrates, and high-density lipids in human diets [11]. By using solar drying techniques, the nutritional parameters of these products can be better preserved compared to uncontrolled sun drying. When food is exposed to uncontrolled sun drying, it is susceptible to contamination by insects, dust particles, and unexpected rainfall, which can compromise its quality and safety. Solar drying offers a controlled environment that protects the agricultural products from external contaminants, ensuring better food safety and reducing losses due to spoilage. Additionally, solar drying can be combined with other methods such as blanching or pre-treatment to further enhance the preservation of nutritional parameters and extend the life span of the dried products. Overall, solar drying is an effective and sustainable technology that addresses the challenges faced by small farmers in remote areas with limited access to electricity and non-renewable energy sources. It enables them to preserve the nutritional quality of their agricultural products, reduce post-harvest losses, and contribute to food security and sustainability [12]. Solar drying is one of the old techniques of drying crops and food products that have been used for centuries. It involves utilizing the energy from the sun to remove moisture from the products, thereby preserving them for longer periods and improving their quality. This method is particularly relevant in India, where agriculture and Agri-based small industrial sectors contribute significantly to the country’s GDP [13]. The use of solar dryers can indirectly contribute to increasing India’s GDP by improving the quality of agricultural products. By drying the products in solar dryers, the need for traditional drying methods, such as open sun drying, can be reduced. This is important because open sun drying is highly dependent on weather conditions and can be inefficient and time-consuming. Solar drying, on the other hand, provides a more controlled environment for drying, resulting in faster and more efficient drying processes. One of the main drivers for the adoption of solar drying in India is the increasing cost of electricity [14]. As the price of electricity rises, there is a growing interest in utilizing sustainable energy sources for various applications, including drying of Agri-based food products. Solar energy being abundant in India due to its geographical location, presents a viable and cost-effective alternative for drying. Solar energy consists of both light and heat, which reach the surface of the earth. When agricultural products are exposed to solar radiation, they absorb some of this energy, generating heat. This heat is then utilized to remove moisture from the products, effectively dehydrating them. The remaining solar radiation that is not absorbed is reflected away [15]. By harnessing solar energy for drying agricultural products, India can reduce its dependence on conventional energy sources and promote sustainable practices. This can have positive economic and environmental impacts, contributing to the overall development of the country’s agricultural and Agri-based small industrial sectors [16].
Anwar et al. [17] investigated how the open sun drying technique dehydrates cauliflower, onion, and potato slices. They found that potatoes and cauliflower had higher moisture content and required more time to dry compared to other items. However, the study did not mention the exact duration needed for drying.
Doymaz et al. [18] explored the process of open sun drying for grapes with and without seeds. Their goal was to reduce the moisture in the grapes from 78% to 20% (on a wet basis). While they did not specify a particular drying duration, they did successfully achieve their desired moisture level.
Ismail et al. [19] conducted open sun drying experiments on cherry tomatoes. Found that natural cherry tomatoes took approximately 22–26 h to dry completely. Pre-treated (presumably pre-processed or pre-treated with a specific method) cherry tomatoes required less drying time compared to natural tomatoes.
Ismail et al. [20] concluded that the pre-treatment method applied to the beans and the ambient temperature during drying both have significant effects on the dehydration rate and time, respectively. These findings provide valuable insights for optimizing the open sun drying process of pre-treated beans, potentially enhancing the quality and efficiency of the drying process in bean-related industries.
The study conducted by Khadraoui et al. [21] investigated the drying process of pepper slices using two different methods: solar drying and solar greenhouse dryer. The researchers compared the drying time of pepper slices between the two approaches and found significant differences. Drying the pepper slices in the mixed-mode solar greenhouse dryer only took two days. This means that the controlled environment created by the greenhouse combined with the use of solar energy improved the drying efficiency and minimized the total drying time compared to open sun drying.
The experiment conducted by Pochont et al. [22] compared the drying of Indian red chili peppers using two methods: open sun drying and a greenhouse dryer. These results showed that the drying process in the greenhouse dryer was qualitatively more efficient than solar drying. The researchers also mentioned that a hybrid solar dryer could be a suitable alternative for drying Indian red chilies.
The article presents a thorough evaluation of solar drying technology currently available for use with a particular focus on drying bee pollen. It also explores the nutritional benefits of employing solar dryers for this purpose. A major discovery of the research is the absence of specialized solar dryers in India for drying bee pollen. This implies a prospect for further innovation and progress in this specific field within the country’s agricultural industry. By examining the current designs of solar dryers and their appropriateness for drying bee pollen, the article highlights the potential advantages of investing in specialized equipment tailored to the requirements of this business. The conclusion stresses the significance of filling this gap in the market to enhance the drying process for bee pollen in India, improving its overall quality and nutritional value. As a result, there is an opportunity for growth in India in terms of developing solar dryers for bee pollen.
Solar-operated dryers indeed represent an efficient means of utilizing solar radiation to facilitate the dehydration process for various products. By harnessing solar energy to generate heat, these dryers contribute to reducing the relative humidity and moisture content of perishable items, thereby extending their shelf life. This preservation capability is particularly valuable in agricultural contexts where maintaining the quality of harvested produce is critical [23]. Moreover, solar dryers offer several advantages beyond simple dehydration. They enable control over the dehydration rate, which is crucial for ensuring optimal drying without compromising product quality. Additionally, by operating in a controlled environment, they help prevent contamination from foreign particles, pollutants, and insects, thereby enhancing the safety and hygiene of the dried products. Overall, solar-operated dryers present a sustainable and environmentally friendly solution for food preservation and processing. Their ability to leverage renewable energy sources not only reduces operational costs but also contributes to mitigating the environmental impact associated with traditional drying methods. As such, they hold significant promise for improving food security, reducing post-harvest losses, and enhancing the overall efficiency of agricultural value chains.
Solar dryers can be classified according to their mode of operation and heat-based heating methods, including the temperature range in which they operate. Fig. 1 shows an illustrative classification of solar dryers [24]. Here are the main categories:
Figure 1: Classification of dryers. Reprinted from Sevada et al. [24]
Direct Dryers: These dryers directly expose the products to solar radiation, allowing them to absorb heat energy for dehydration.
Indirect Dryers: In indirect dryers, the drying products are not supposed to directly come in contact with solar radiation. Instead, the solar energy is absorbed by a heat transfer fluid (e.g., air or water), which then transfers the heat to the products for drying.
Mixed-Type Dryers: Mixed-type dryers combine elements of both direct and indirect dryers. They use a combination of direct exposure and heat transfer fluids to achieve the desired drying effect.
Greenhouse Dryers: These dryers incorporate the concept of a greenhouse to create a controlled environment for drying. Solar radiation enters the greenhouse, creating a warm, enclosed space for the products to dry.
Hybrid Solar Dryers: Hybrid solar dryers utilize multiple energy sources, such as solar energy and electricity or fossil fuels, to provide a more consistent and efficient drying process.
Dryers with Heat Energy Storage System: Solar dryers that use heat energy storage systems, like thermal or phase change materials, can save excess solar energy to maintain continuous drying processes even when there is no sunlight. With a range of options for operating procedures, heating methods, and temperature ranges, these dryers are versatile and effective for dehydrating products while preserving their quality [25].
Dryers that run on solar thermal energy are considered non-traditional since they rely on the sun’s renewable power. In contrast, fossil fuel-powered dryers are traditional as they depend on non-renewable resources. High-temperature output dryers are ideal for situations where quick drying is necessary. They offer speedy drying, which is beneficial in specific applications. Conversely, low-temperature dryers maintain constant air ventilation, enabling the product’s moisture content to reach equilibrium. These dryers are often used for products that require slower drying or when higher temperatures might damage the product’s quality. Solar dryers come in various designs, with the main goal of drying agricultural products to preserve their nutritional properties. When designing solar dryers, several criteria are considered:
Movement of air throughout the dryer: The airflow inside the dryer required to be properly designed to ensure efficient and uniform drying of the products.
Type of insulation: Insulation is crucial to maintain the required temperature inside the dryer. It helps minimize heat loss and improves the overall energy efficiency.
Flow of air in a specific direction: Controlling the direction of airflow within the dryer can optimize the process of drying and enhance the uniformity of the drying process across the product.
Setup of dryer and arrangement: The physical layout and arrangement of the solar dryer play a significant role in its performance. Factors such as the size, shape, and positioning of the drying chambers and the arrangement of the product trays need to be considered.
Solar radiation and assessment: The amount of solar radiation available in the specific location of the dryer is a critical factor. This information helps determine the sizing and capacity of the solar dryer to ensure optimal utilization of solar energy.
Products to be dried: Different products have varying moisture content and drying requirements. Solar dryers need to be designed considering the specific characteristics of the products being dried, such as their size, shape, and moisture content.
By considering these criteria, solar dryers can be tailored to meet the specific needs of farmers and provide an efficient and sustainable solution for drying agricultural products.
3 Literature Review on available Solar Operated Dryers
3.1 Solar Dryer in Direct Mode
A natural convection dryer also known as a solar dryer operates in the direct mode which uses solar radiation to dry products. The dryer functions based on the principle of natural convection, meaning that airflow is generated by natural buoyancy forces rather than mechanical devices like fans or blowers. Its drying process is solely dependent on solar radiation.
Illustrated in Fig. 2 is the direct mode solar drying method for dehydrating products. This process utilizes solar radiation to eliminate moisture content from the product. The initial phase involves the entry of solar radiation through the transparent glass on the dryer, permitting heated air to enter the insulating chamber. The glass reflects some solar radiation, while the rest infiltrates the thermal collector [26]. The insulating chamber is designed to trap heat and maintain a suitable temperature for drying. The absorbed solar radiation increases the temperature inside the insulating chamber, which in turn raises the temperature of the product to be dried. This temperature rise facilitates to extraction of moisture from the product. To ensure proper airflow and ventilation, air holes are provided inside the insulating chamber. These air holes allow the entry and exit of air, creating a flow that aids in the drying process. However, it is important to note that since solar radiation directly comes in contact with the material to be dried, the quality of the product can be negatively affected. This could be due to factors such as overheating, uneven drying, or potential damage caused by prolonged exposure to direct sunlight. It is essential to consider these aspects and employ appropriate measures to minimize any negative impact on product quality during the direct process of solar drying [27].
Figure 2: Direct solar dryer. Adapted from El-Mesery et al. [25] and Ogheneruona et al. [26]
The study conducted by Sodha et al. [28], a solar cabinet dryer was tested against traditional sun drying methods to evaluate its efficacy in drying fresh mangoes. The dryer depicted in Fig. 3, was able to reduce the moisture content of 1 cm thick mangoes from 95% to 13% within 24 h during the summer season. However, this process took 34 h in winter conditions. It’s important to note that these drying times are based on the specific conditions of the experiment and may vary depending on factors like solar radiation intensity and ambient temperature. Overall, the study indicates that the solar dryer performed better than open sun drying regarding preserving the quality of the dried product. The dryer allowed for faster moisture reduction and a more reliable and controlled drying environment compared to traditional methods. This shows that solar cabinet dryers have the potential to be a highly efficient and effective alternative for preserving agricultural products while maintaining their quality.
Figure 3: Solar cabinet dryer design. Reprinted from Sodha et al. [28]
In the case of Hallak et al. [29], designed a solar cabinet dryer that featured a metal staircase with a chimney attachment at the top of the insulating chamber as seen in Fig. 4. The staircase and chimney were designed to improve the drying efficiency and airflow by allowing hot air to flow more easily through the dryer. Gbaha et al. [30] have created a dryer for bananas and mangoes that is highly thermally efficient and designed with precision. Solar cabinet dryers are an excellent way to dry agricultural products, as they make use of renewable solar energy. This sustainable method reduces reliance on fossil fuels and can improve the preservation and value of crops.
Figure 4: Solar cabinet type dryer with staircase. Extracted from Hallak et al. [29]
The study conducted by Borah et al. [31] aimed at the development and application of a solar conduction dryer (SCD) for the drying of turmeric rhizomes. The SCD utilized all three modes of heat transfer such as conduction, convection, and radiation to facilitate the drying process. The experiment took place in Assam, an area characterized by high humidity and relatively low solar intensity. The solar conduction type dryer was developed by a team of innovators associated with Science for Society (S4S), an organization dedicated to promoting scientific advancements for the betterment of society. The dryer, also referred to as a food dehydrator dryer, aimed to provide an efficient and sustainable solution for drying turmeric rhizomes using solar energy. During the drying of turmeric, it is observed that SCD has achieved 39°C–51°C concerning an ambient temperature of 25°C–28°C. The physical model of solar conduction dryer is illustrated in Fig. 5.
Figure 5: Solar conduction dryer. Reprinted from Borah et al. [31]
The turmeric had an initial moisture content of 78.65% but after 12 h of continuous drying, the moisture percentage of both the solid and sliced samples was reduced to 6.36% and 5.50%, respectively. To achieve the same moisture content levels using open sun drying, it would take 25.5 h. The solar convection type dryer (SCD) used in the drying process has a calculated total surface area of 1.04 m2 and is made with semi-transparent polycarbonate sheet multiwall panels that allow solar radiation to pass through. The SCD consists of four drying trays coated with a food-grade black color, which helps absorb solar radiation to aid in the drying process. This information outlines the drying process for turmeric using a solar convection dryer, including the materials used and the reduction in moisture content.
Fig. 6 describe the drafting, i.e., front view, side view and top view of SCD. Air is entering from the front side of the tray and removes moisture from the rhizomes through the chimney. Table 1 describe the studies conducted on the development of direct solar dryers for fruits, vegetables and other similar food products.
Figure 6: Drawing layout of SCD (all dim. are in mm). Reprinted from Borah et al. [31]
A solar drying system called an indirect solar dryer is utilized for dehydrating a range of materials. This dryer is fabricated having two primary parts: a solar thermal collector and an insulated chamber, as depicted in Fig. 7. The solar thermal collector is covered with transparent glass allowing solar radiation to enter. Within the collector, there is a black surface that absorbs the solar thermal radiation and transforms it into heat energy. This heats the air inside the collector. The heated air then slowly moves into the insulated chamber. The collector and insulated chamber are both insulated using materials like glass wool, puffing and heatlon sheets to reduce heat loss, ensuring that heat is retained within the system, thus enhancing the quality of drying. To eliminate moisture from the drying materials, the heated air with moisture content is allowed to escape through a chimney located above the insulated chamber. This ventilation enables the removal of moisture from the drying chamber. Some indirect solar dryers may also include a blower for air circulation. An indirect dryer is a type of dryer that operates without making contact with the material being dried. It uses hot gas to transfer heat to the material, which is usually contained in a rotating drum or a series of stationary pipes. The hot gas comes into contact with a heat exchanger, which then heats the material indirectly. This type of dryer is commonly used in the food, chemical, and pharmaceutical industries. A dryer that doesn’t make contact with the material being dried is known as an indirect dryer. It works by using hot gas to transfer heat to the material, which is typically found within a rotating drum or stationary pipes. A heat exchanger comes into contact with the hot gas, and this indirect heat is used to dry the material. This type of dryer is often found in the chemical, food, and pharmaceutical fields [32]. The blower is vital for circulating air which accelerates and enhances the drying process. Indirect solar dryers are more efficient than direct ones because they offer more control over the drying environment. With better control, the drying performance is improved. Indirect solar dryers are an excellent alternative to direct solar dehydration methods while overcoming the limitations. Hence, they are preferred in specific situations [33].
Figure 7: Indirect solar dryer. Adapted from Lotfalian et al. [32]
Solar dryers come in three types: direct, indirect and mixed. These can be further classified as either passive or active, depending on the airflow. Fig. 8 illustrates the different designs of solar dryers. Many different solar dryers have been created over the years to meet the drying needs of various products. Designers must take into account factors such as quantity, characteristics, and materials to be dried when creating a dryer. The design of a dryer can vary greatly depending on the specific type of food product being dried [34].
Figure 8: Classification of solar dryers. Reprinted from Visavale et al. [34]
A research study conducted by Basumatary et al. [35] on a solar-based cabinet dryer, which was designed, constructed and calibrated to extract moisture from chili peppers over 7 h. In the afternoons, the dryer recorded a peak temperature of 77°C, while the average temperature ranged between 64°C and 66°C from 9:30 am to 3:30 pm from March to August. The experiment was conducted at the Central Institute of Technology in Kokrajhar, Assam, where the maximum average temperature ranges from 29°C during these months. The dryer successfully extracted 49% of moisture from the product during the 7 h. The dryer consists of two main components, a thermal collector, and an insulating chamber. The insulating chamber is built with wooden flanks and a G.I. sheet coating on the inside, while the thermal collector is made of G.I. metals and painted black to absorb solar radiation. Please refer to Fig. 9 for the design of the solar cabinet-type dryer developed by Basumatary et al. Constructed using bamboo nets, these devices allow for the efficient flow of warm air from the base of the container to its upper regions.
Figure 9: Design and dimension of (a) insulating chamber, (b) drying tray, and (c) thermal collector of solar cabinet dryer. Adapted from Basumatary et al. [35]
The experiment conducted by Singh et al. [36] in his research paper. It seems that they designed an indirect type solar dryer with a specific configuration and tested it on potato chips and chili samples. The solar dryer had an 800 mm × 1300 mm thermal collector size. It featured an absorbing plate made of 2 mm thick aluminium, which was covered with black paint to attract heat absorption. The dryer included an insulating chamber divided into four compartments by three drying trays. These trays were fabricated with a fine wire mesh to allow heated air to pass. J-type thermocouples were installed at the inlet, inside the insulating chamber, and at the outlet of the insulating chamber. These thermocouples were used to measure the temperature difference at different points during the drying process. Three samples, each weighing 500 g, were tested for drying. Tray 1, which was the first tray in contact with the heated air, showed a rapid decrease in mass compared to trays 2 and 3. Moisture loss and temperature readings were recorded at regular intervals of 2 h. The experiment was conducted for a total of 32 h. The results indicated a moisture loss of 53.07% in the chili samples.
Fig. 10 describe an actual view of the thermal collector and cabinet insulating chamber developed by Singh et al.
Figure 10: Collector and drying cabinet. Adapted from Singh et al. [36]
An experiment conducted by Ekechukwu et al. [37] investigate the development of natural-type indirect solar dryers for dehydrating tropical crops in Ireland. The study produced two types of indirect solar dryers: distributed natural circulation and integral natural circulation. The distributed solar dryer is illustrated in Fig. 11 and is commonly known as an indirect passive solar dryer, utilizing natural airflow. The crops are stored in an insulated chamber and heated by hot air flow, while the chimney located at the top removes moisture from the crops.
Figure 11: Distributed (indirect) type natural convection solar-based dryer. Reprinted from Ekechukwu et al. [37]
Sarsilmaz et al. [38] examined a solar-powered cylindrical dryer that utilizes a rotary column design. This particular dryer belongs to the category of indirect active dryers, where airflow is regulated through the use of fans. A diagram of the dryer is depicted in Fig. 12. While its main function is to dry apricots, it can also be used to dry other fruits and vegetables hygienically. The dryer comprises three central elements, the air blower section, solar collector, and rotating insulating chamber. The insulating chamber is made of wood, has a diameter of 1100 mm and is 1100 mm tall depending on the desired drying capacity. Wooden panels are assembled on a rotating shaft, which is powered by a 12 V Permanent magnet direct current (PMDC) motor through a pulley-belt system. The research showed that the moisture content of apricots decreased to 25% after eight days of sun drying in an open area. In contrast, the rotary dryer achieved the same moisture level in just four days. This indicates that the developed dryer significantly reduces drying time compared to traditional open sun drying methods.
Figure 12: The rotary drying system. Reprinted from Sarsilmaz et al. [38]
In the study, Benhamau et al. [39] simulated and evaluated the performance and evaluation of a forced-type convection dryer using an indirect active-type solar dryer as mentioned in Fig. 13. This solar dryer was designed by UDES (Unit Solar Energy Development in Bou Ismail). The purpose of the simulation was to evaluate the drying process of two substances: pomace and colocynth. Pomace is the by-product obtained after extracting olive oil. In this case, pomace was used as a fuel for heating purposes in the solar dryer. Colocynth, on the other hand, is an herbaceous plant that is native to North Africa. It is known for its climbing or creeping nature. Colocynth gourd is an herb traditionally used for its medicinal properties, particularly as a remedy for skin infections. To facilitate the drying process, the preheated air from a thermal collector is drawn into the system using a centrifugal pump located at the lower surface of the insulating chamber. The expanded and heated air flows from the bottom of the chamber to the top, drying the pomace and colocynth, and is eventually discharged outside.
Figure 13: Forced type convection dryer (a) thermal collector, (b) insulating chamber and (c) auxiliary fan. Adapted from Benhamau et al. [39]
The experiment aimed to measure various parameters related to the drying process of different products using a data acquisition system and a pyranometer to assess solar radiation. The experiment was conducted from 11 March to 19 May, 2012 at a site in Algeria with coordinates Latitude 36°39′ and Longitude 2°42′ East. A total of 2.6 kg of pomace was used for the experiment. The pomace initially contained 25% water. 1.5 kg of colocynth was used for the experiment. The colocynth initially contained 65% water. The temperature was measured using a Fluke Hydra data acquisition system, which recorded temperature readings at various subsystems of the dryer. The peak temperature achieved during the experiment was 62.6°C, with an ambient temperature of 23°C. The inner and outer relative humidity of the dryer was measured but the specific values or trends were not mentioned. The weight loss of the product during the drying process was measured, but the exact values or percentages were not provided. The global, direct, and diffused solar radiation was measured using a portable pyranometer. The product lost 75% moisture content in 7 h of continuous drying.
Gupta et al. [40] has designed an indirect-type dryer for drying agricultural products using natural convection airflow as shown in Fig. 14. The dryer includes a solar thermal collector, and the design was specifically developed for the Nagpur region in Maharashtra, India. According to the given formula, the angle of inclination or tilt (β) for the solar thermal collector is calculated as β = 10° + latitude. For Nagpur, with a latitude of 21.15°, the tilt angle would be 31.15°. To determine the total area required for the design of the solar thermal collector, the formula for mass flow rate (Ma) is used: Ma = va × ρa. However, the values for va (air velocity) and ρa (air density) are not provided in the given information, so it is not possible to calculate the total area required without this additional information.
Figure 14: Indirect type dryer for agriculture product. Adapted from Gupta et al. [40]
The area of the collector was 0.6 square meters with dimensions of 1 meter by 0.6 meters. The insulation surrounding the collector has a thickness of 3.54 centimeters. This insulation helps prevent heat loss during the drying process. The outer casing of the thermal collector and the insulating chamber were constructed using wooden material. This choice of material helps reduce heat loss. An aluminium sheet with a thickness of 1 millimeter was used as the absorber plate. The aluminium sheet was painted with backboard paint, which helps increase the air temperature inside the collector with the absorption of solar radiation. A transparent glass panel with a thickness of 5 millimeters was placed on top of the collector. This glass panel allows solar rays to enter the collector while protecting the interior from external elements.
Two reflecting mirrors are assembled on the collector at angles of 135°C and 120°C. These mirrors are used to concentrate solar radiation onto the thermal collector as shown in Fig. 15. The thickness of the two reflecting mirrors is 3.5 cm. Heatlon sheet is used as the insulation material for both the thermal collector and the insulating chamber. Heatlon sheet helps to retain the heat inside the chamber. An exhaust fan with a diameter of 6 mm and operating at 12 Volt direct current (VDC) is installed at the front top side of the insulating chamber. Its purpose is to remove humid air from the chamber, allowing for better drying conditions. Three trays made of steel net mesh, each measuring 48 cm × 48 cm, are installed inside the insulating chamber. These trays provide a platform for placing the fruits, vegetables, roots, and tuber crop chips for drying. A gap of 7 cm is maintained between the trays to allow for proper air circulation and heat distribution within the chamber. The insulating chamber can achieve a maximum temperature of 65°C when the ambient temperature is 35°C. This high temperature is beneficial for the efficient drying of food items. Author suggested that the developed solar based dryer is convenient for the drying of fruits, vegetables, roots and tuber crop chips.
Figure 15: Reflecting mirror and insulating chamber of indirect dryer. Adapted from Gupta et al. [40]
Sai Krishna Teja et al. [41] worked on the development of Indirect Solar Dryer (ITSD) (Fig. 16) for drying of red chilies and grapes and installed at Koneru Lakshmaiah Education Foundation (KLEF, Deemed to be University) at Vijayawada, Andhra Pradesh. The developed solar dryer comes in active type forced convection dryer category. The solar operated dryer was developed for the drying of fresh and ripened chilies (1 kg) and fresh grapes (1 kg). The solar collector area was calculated by using formula Ac = E/(Sr × ρ), where Ac is area of the collector, E is energy (kJ), Sr is solar radiation (kJ/m2) and ρ is efficiency (%). The calculated area for the solar collector is 0.245 m2 (0.7 m × 0.35 m).
Figure 16: Indirect solar dryer. Reprinted from Saikrishna Teja et al. [41]
The angle of inclination for the solar collector was calculated as 26.5° using the formula (Latitude + 10°). This angle is important for optimizing the collection of solar radiation. The insulating chamber has dimensions of 0.33 m (L) × 0.46 m (W) × 0.48 m (H). It is used to provide insulation and prevent heat loss during the drying process. The outer surface of both the solar thermal collector and the insulating chamber was fabricated using G.I. (galvanized iron) sheet. The solar radiation absorber plate in the thermal collector is made of G.I. with a thickness of 1mm and is colored black to enhance solar radiation absorption. The upper side of the thermal collector is covered with ordinary transparent glass with 5 mm in thickness to allow direct entry of solar radiation. Aluminium-coated rock wool with a thickness of 25 mm is used as an insulator for both the collector and the insulating chamber. This insulation helps to minimize heat loss during the drying process. Three trays, each measuring 300 mm × 300 mm, are fitted inside the insulating chamber for loading the red chilies and grapes. The trays are made of mild steel mesh. Various measurements were taken during the drying process. The temperatures at different sections of the dryer including the thermal collector inlet and outlet of the insulating chamber, ambient temperature were recorded using K-type thermocouples and temperature indicators. The relative humidity inside the insulating chamber was recorded using a hygrometer. Additionally, the weight of the loaded chilies and grapes was recorded at every 1-h interval. The peak temperature recorded in the thermal collector was 50°C, while the minimum temperature recorded was 35°C. These temperatures indicate the range of heat captured by the collector during the drying process.
Etim et al. [42] conducted a study on various models of indirect solar dryers to dry cooking bananas in Umudike, Nigeria. The study focused on the impact of the inlet air on the dryer’s performance. The authors found that there is no existing literature on the different sizes of air intake areas for solar dryers. To test their theories, they created four dryers namely square, rectangular, circular and triangular which are made of plywood. The inlet air vents varied in size and shape while the exhaust air vent remained uniform. Sawdust was used as an insulating material to prevent heat loss. The collector’s top cover was made of 3 mm thick transparent glass and the insulating chamber contained a single layer of trays for the banana slices. The dryer was based on the principle of forced convection, where air is circulated through the system to facilitate drying. The dryer was installed at a 10° North, and its size was 0.6 m in length and 0.4 m in width. There were 52 indirect solar dryers for experimental use. These dryers are 4.5 meters in length and 2.5 meters in width. The exploded view of the developed solar dryer is shown in Fig. 17.
Figure 17: Exploded view of solar dryer. Reprinted from Etim et al. [42]
When solar panels are connected in parallel, their voltages remain the same, but their current capacities increase. In your case, you have three solar panels, each with a power output of 150 W and a voltage of 12 V. When connected in parallel, the total power output remains the same (450 W), but the current capacity increases. However, they are connected through a charge controller to charge a 12 V, 200 Ah battery. The charge controller regulates the charging process to ensure that the battery is not overcharged and damaged. The battery is charged by a solar panel and used as an energy storage device in this system. It stores the extra power from solar panels during the day and provides a steady supply of DC power for operating the blower. The blower is connected to the battery and it helps increase the flow rate of air inside the dryers. By varying the air inlet area in the dryer, you observed that the drying efficiency increased up to a certain size. This can be attributed to the fact that increasing the air inlet area allows for more air to enter the dryer, leading to better circulation and faster evaporation of moisture from the drying material. However, there may be a point of diminishing returns, where increasing the air inlet area beyond a certain size doesn’t provide any significant improvement in drying efficiency. An experiment was conducted to investigate the impact of air inlet area on the efficiency of solar dryers. This information can be valuable for optimizing the design and the performance of solar based dryers in various applications.
Kolhe et al. [43] developed an innovative solar based dryer that uses indirect forced convection to dry agricultural products like red chili and potatoes. The dryer is made up of a thermal collector, trays, and an insulating chamber. The thermal collector has a surface area of 0.57 m2 and is designed to accumulate as much ambient air as possible. To facilitate this, a DC fan is installed at the entrance of the collector. By increasing the airflow, the relative humidity inside the insulating chamber is reduced which is ideal for the drying process. The trays used in the dryer are made of perforated steel sheets with 90% perforation and have an area of 0.96 m2 each. Four trays are placed inside the insulating chamber with equal spacing, providing efficient use of space for agricultural products. For a better understanding of the dryer’s design and configuration, an isometric view can be seen in Fig. 18.
Figure 18: Isometric view of developed solar dryer. Reprinted from Kolhe et al. [43]
The solar collector and insulating chamber together weighed 75.4 kg. In Raipur, India the experiment ran from 10:00 am to 05:00 pm and began with temperature and humidity readings taken hourly without any load to determine the maximum temperature in the insulating chamber. Wet and dry bulb temperatures were measured using the Sling psychrometer principle to assess moisture content and humidity levels. To calculate the solar dryer’s overall performance, load tests were conducted and compared to open sun drying. Hourly parameters were recorded for several samples with known moisture contents loaded onto trays. The average moisture content removed from the sample in the solar dryer was 0.414 kg out of a total sample weight of 0.507 kg indicating an effective moisture removal of approximately 81.65% on a wet basis (Wb). In comparison, open sun drying resulted in a moisture of 78.91% on a wet basis (Wb) for the same sample. Solar drying is a more efficient method of drying potatoes and chilies compared to open sun drying. The solar dryer was able to dry a certain weight of potato slices in 5 h, while open sun drying took 6 h to achieve the same task. The average temperatures in the collector and insulating chamber of the solar dryer were 58°C and 48°C, respectively, indicating its heating capacity and drying efficiency. When drying 2.5 kg of red chilies, the solar dryer achieved a moisture of 64.1% on a wet basis in 17 h, while open sun drying required 22 h to achieve a moisture content of 68.54% on a wet basis. Open sun drying also resulted in the inclusion of foreign materials, which negatively impacted the quality of the dried chilies. Therefore, the use of the solar dryer resulted in a shorter drying period, higher moisture content removal and improved product quality by avoiding the mixing of foreign materials.
Pakhare et al. [44] conducted research on a solar cabinet dryer that indirectly dried chili slices at Savitribai Phule Pune University. The objective was to improve the dryer’s efficiency by incorporating a method for storing thermal energy during the day and releasing it at night. The study explored three types of heat storage systems which are Sensible Heat Storage (SHS), Latent Heat Storage (LHS) and Thermal Chemical Storage (TCS). In the Sensible Heat Storage (SHS) method, thermal energy is stored by increasing the temperature of a solid or liquid material, which can be used later as needed. The Latent Heat Storage (LHS) approach uses a phase change material (PCM) to accumulate and release heat energy during a change of state, such as from solid to liquid. The study employed Paraffin wax as the PCM for heat energy storage. The solar dryer design included an insulating chamber, which played a critical role in the forced convection process. The experimental setup for the author’s solar dryer is depicted in Fig. 19 which shows the entire process of drying.
Figure 19: Solar dryer experimental setup. Adapted from Pakhare et al. [44]
The dryer was constructed using plywood and polystyrene (Thermocol) to create an insulating chamber. The dimensions of the chamber were 1 m (L) × 0.6 m (W) × 0.8 m (H). PCM trays were fabricated using aluminium material with sizes of 0.8 m (L) × 0.56 m (W) × 0.025 m (H). PCM is a material that can store and release heat energy during the phase change process. A flat plate collector was used to heat up the air before it entered the insulating chamber. Thermal collector had dimensions of 2 m (L) × 1 m (W) × 0.1 m (H) and contained an aluminium absorber plate (1.7 m (L) × 0.9 m (W)) painted with blackboard colour to enhance heat absorption. A centrifugal blower with a flow rate of 240 Cubic feet per minute (CFM) and 30 W AC power was installed at the corner of the thermal collector to facilitate forced convection and airflow. K type thermocouples were used to measure temperatures at different stages of the experiment. This helps in monitoring and evaluating the performance of the dryer. An anemometer (with a maximum speed of 30 m/s) was used to measure the airflow at the entry point of the insulating chamber. This allows for monitoring the flow of air through the system. The initial moisture of the chili was 79%, which was minimized to 15% at the end of the experiment. The drying process took 17 h in the developed solar based dryer, whereas it took 27 h in an open sun drying process, indicating improved efficiency. The efficiency of the solar based dryer was observed to be 22%. This efficiency is a measure of how effectively the system converts solar energy into usable heat for the drying process. It was noticed that the inside temperature of the insulating chamber was 6°C–9°C more than the ambient air temperature estimated 6–7 h during periods of non-sunshine. This temperature rise was attributed with the utilization of phase change materials, which can store and release heat energy. The paper also studied the variation in mass flow rate concerning temperature. This analysis helps understand the airflow dynamics and heat transfer within the system. Overall, the solar dryer with phase change materials showed improved drying efficiency and reduced drying time compared to open sun drying. The use of Phase change materials (PCM) contributed to maintaining higher temperatures inside the insulating chamber during non-sunny periods.
The study conducted by Vijayan et al. [45] focused on evaluating the exergy of an indirect forced convection solar dryer used for drying bitter gourd slices. Bitter gourd is a vegetable that spoils easily due to its high moisture content of 92% when calculated on a wet basis. Therefore, it is necessary to dry it in slices to preserve it for future use. To conduct the study, the authors created an experimental setup at the Solar Energy Research Centre in Coimbatore, Tamil Nadu, India, which included a solar thermal collector with a sensible heat storage system, a centrifugal blower and an insulating chamber. The solar thermal collector was 2 meters long, 1 meter wide and 150 mm high and had a black coated galvanized sheet above the heat storage medium to enhance solar radiation absorption. A 4 mm thick glass was installed 30 mm above the absorber plate to prevent heat leakage and minimize heat loss. Fig. 20 presents the findings of the study. To dry the materials, a blower was utilized to push air from the thermal collector into the insulated chamber. The airflow was regulated with a valve that required manual adjustment. Pebbles were layered between the absorber plate and the thermal collector’s lower plate each 30 mm thick. These pebbles were utilized to retain heat while the sun was out and then release it at night, which assisted in the drying process.
Figure 20: Schematic diagram and experimental setup of the forced indirect type solar dryer. Adapted from Vijayan et al. [45]
The thermal collector and insulating chamber were properly covered with glass wool (25 mm to 50 mm thickness) to minimize heat loss. The outer surface of the thermal collector and insulating chamber was covered with a 1.2 mm galvanized iron sheet. The insulating chamber had a size of 0.75 m × 0.75 m × 1.05 m and contained four perforated aluminium trays for holding the bitter gourd slices. Solar radiation was measured using a solar power meter and thermocouples of k-type were used at various levels to measure temperatures. Relative humidity of the ambient air, as well as the inlet-outlet of hot air was recorded using a hygrometer with 2% accuracy. Each tray initially contained 1 kg of bitter gourd slices. The experiment started at 9:00 am in the morning and remain continued until the product reached the slump point. To achieve a steady state, the empty dryer was allowed to run for 30 min before loading the bitter gourd slices. The moisture percentage of the product was calculated on both a wet as well as dry basis. The average solar radiation observed was 720 W/m2, with a maximum of 996 W/m2. During the peak of solar radiation, the thermal collector’s end/outlet point temperature was 45.3°C, and the absorbing plate temperature was 68.2°C. The weight of the bitter gourd slices was reduced from 3.65 kg (92% wet basis) to 0.35 kg (9% wet basis) of water in just 7 h using the indirect type solar dryer.
Güler et al. [46] conducted a study on the Computational Fluid Dynamics (CFD) analysis of an indirect type solar dryer that was designed with a low-cost mesh. The researchers used Ansys Fluent software to investigate the thermal behavior of the solar based dryer, which includes a solar thermal collector and an insulating chamber. The solar air collector was constructed with dimensions of 700 mm × 400 mm × 250 mm. The addition of the iron mesh was implemented in the design to observe the simulation results of the air collector. The researchers performed software analysis for both a flat double plate collector and a modified mesh double pass collector. Fig. 21 likely presents a visual representation or schematic of the flat double plate collector and the modified mesh double pass collector. This figure would illustrate the different configurations of the collectors and how they were implemented in the solar dryer.
Figure 21: Mesh configuration for collector (a) with a double pass (b) with modified mesh. Adapted from Güler et al. [46]
In their study likely shows the mesh that was created using the software for the CFD analysis. This mesh is a computational grid or discretization of the domain, allowing for the circulation of fluid flow and heat transfer within the solar dryer.
The geometry of the insulating chamber and mesh configuration can be seen in Fig. 22 and the analysis domain is displayed. Airflow was taken as fluid which was used for energy derivation, momentum and continuity equations. Velocity air contour can be observed at the middle part of both collectors which can be seen in Fig. 23.
Figure 22: Geometry view and mesh configuration of insulating chamber. Adapted from Güler et al. [46]
Figure 23: Velocity distributions in (a) without iron mesh and (b) with iron mesh collectors. Adapted from Güler et al. [46]
The addition of iron mesh at the bottom of the air collector decreases the velocity of air and air spends more time inside the collector. So air hated more and increased the solar dryer’s efficiency.
Table 2 describe the studies conducted on the development of indirect solar dryers for fruits, vegetables and other similar food products.
3.3 Mixed/ Combined Type Solar Dryer
A mixed type solar dryer consists of collectively direct and indirect solar drying combinations used for dehydrating agricultural products and foodstuffs. This dryer utilizes a thermal collector, an insulating chamber, and a chimney. The process works by absorbing direct solar radiation to heat the ambient air. The hot air is then blown through the thermal collector and enters the insulating chamber, where the dehydration of Agri-based products and foodstuffs takes place [47]. Fig. 24 likely illustrates a cross-sectional view of this solar dryer, showing how direct radiation passes through the insulating chamber from the top side [48]. This mixed type solar dryer offers advantages by combining the benefits of collectively direct and indirect solar drying methods. Direct solar radiation gives efficient heating, while the insulating chamber helps to maintain a controlled environment for drying the products. This approach can enhance the drying efficiency and quality of the dehydrated items [49].
Figure 24: Cross-sectional view of mixed solar dyer developed. Reprinted from Baniasadi et al. [48]
The study carried out by Erick César et al. [50] conducted a study that focused on analysing the thermal performance of a solar dryer used for dehydrating tomato slices. This dryer was classified as passive, meaning it did not require a motor or blower for air circulation. The main objective of the study was to compare the drying kinetics of tomato slices using two types of solar dryers: mixed and indirect. A diagram of the mixed/combined type solar dryer created by the authors is shown in Fig. 25 of the study. Various measuring instruments such as an anemometer, humidity sensor, pyranometer, temperature indicator and ambient temperature sensor were used on the dryer to measure relevant parameters. The upper side of the insulating chamber had 3.2 mm thick tempered glass, which helped in attracting direct solar radiation to enhance the heating process and improve the thermal efficiency of the dryer. On sunny days, the researchers recorded a temperature of 73.58°C inside the insulating chamber. The author developed a passive solar dryer that didn’t require a motor or blower and instead made use of tempered glass to enhance its thermal efficiency. This dryer was a mixed/combined type that fell under the category of indirect solar dryers.
Figure 25: Mixed/combined type solar dryer developed. Adapted from Erick César et al. [50]
The experiment was conducted for 3 days/26 h to minimize the moisture percentage of tomato slices from 93.36 WB to 15 WB.
The mixed/combined type solar dryer developed by Oosthuizen et al. [51] consisted of a solar collector, an insulating chamber and a provision of the chimney at the top of the insulating chamber as shown in Fig. 26. They used rice husk insulation to prevent heat loss during drying, and covered the insulating chamber and solar collector with transparent plastic sheets to allow solar radiation to heat up the grains and rice inside. The sheets also helped to trap the generated heat which makes the drying process more efficient. This mixed type solar dryer was specifically designed to make the most of solar energy with the insulation and transparent coverings playing a vital role in the process.
Figure 26: Isometric view of mixed type solar dryer developed. Adapted from Oosthuizen et al. [51]
Ekka et al. [52] worked on the development of a mixed/combined forced convection type solar cabinet dryer (MFCSCD) for the dehydration of cluster fig. The experimental setup of the developed dryer can be seen in Fig. 27.
Figure 27: Experimental setup of mixed type solar dryer developed. (a) Diagrammatic view and (b) Actual view of solar dryer. Reprinted from Ekka et al. [52]
The experiment conducted in October 2017 at IIT Guwahati, Assam, India focused on the drying of cluster figs. The setup used for the experiment is shown in Fig. 27 and two solar air heaters assembled in series for hot air flow. A blower was placed between the solar air heaters and an insulating chamber (cabinet) to facilitate air circulation. To minimize heat loss, a polyurethane insulation sheet was inserted at the bottom side of the air heating chamber. An insulating chamber made of pinewood had a dimension of 2.09 m × 0.89 m × 0.45 m. The top of the insulating chamber was covered with a 4 mm thick glass measuring 1.9 m × 0.9 m to capture solar radiation. An exhaust pipe was attached at the end of the insulating chamber to remove moisture and maintain airflow. Rectangular trays were fitted inside the insulating chamber at equal intervals, as depicted in the setup. The cluster figs used for the experiment were halved to facilitate dehydration. Each experiment loaded the dryer with 5 kg of cluster figs. Two different mass flow rates were employed to observe their effect on the drying process. The flow rates in terms of mass used were 3.72 kg/min and 1.08 kg/min for drying two separate samples of cluster figs. The experiment was conducted for 4 days. From the observation of the experiment, it was noted that an increase in the mass flow rate led to a higher percentage of moisture content being removed during the process of drying.
Ayua et al. [53] worked on the comparison of mixed/combined mode passive type solar dryer in direct mode for the African vegetables and processing the chili. The main aim of his research was to provide an easy way for drying of fresh agricultural produce like vegetables and chili without losing its nutritional parameters. The mixed mode passive type solar dryer developed by Ayua et al. can be seen in Fig. 28.
Figure 28: Mixed mode solar dryer developed. Reprinted from Ayua et al. [53]
The main components of above mentioned dryer were the thermal collector, insulating chamber and chimney. This dryer was modified from the design of a dryer developed by Ayua et al. [53] from the horticultural laboratory, Davis, CA, USA. The dryer includes thermal collectors, which are responsible for absorbing solar radiation along with heating the atmospheric air before it enters the drying chamber. In this case, three inclined thermal collectors are used to heat up the air. An insulating chamber is where the process of drying takes place. It is designed to retain heat and provide a controlled environment for drying vegetables. The chamber is made up of three trays named A, B and C. A chimney is assembled at the last of the insulating chamber to remove humid air. This helps to maintain proper ventilation and prevent moisture build-up during the process of drying. The bottom of the insulating chamber is covered with a black polythene sheet. This sheet is used to attract solar radiation and facilitate the heating of the chamber. The top of the insulating chamber is covered with a clear polythene sheet. This sheet serves multiple purposes: it protects the vegetables from dust, rain, and other foreign particles, allows uniform drying by allowing sunlight to pass through, and helps maintain a controlled environment within the chamber. The process involves placing the chili on the trays within the insulating chamber and exposing it to the heated air from the thermal collectors. As the heated air inside the chamber, it absorbs the amount of moisture from the chili, gradually reducing its moisture content. Once the desired moisture level is achieved (10% in this case), the chili is packed in airtight packaging to maintain its quality and prevent rehydration.
Zomorodian et al. [54] worked on the design of a mixed/combined mode solar dryer for drying of paddy rice in the Department of Agricultural Engineering, Shiraz University, Iran. The dryer was fabricated containing six air heaters, an insulating chamber, a heating passage, a blower and air carrying ducts. Fig. 29 illustrates the complete experimental setup of a mixed/combined mode solar dryer developed by Zomorodian et al.
Figure 29: Experimental set up of mixed/combined mode solar dryer. Reprinted from Zomorodian et al. [54]
The area of each thermal collector plate was 2 m2 to heat the ambient air. The electric heating channel (6 kW) was installed in between the air heater and insulating chamber to supply heated air during unfavorable weather conditions. The highest temperature of 55°C was maintained inside the insulating chamber. A total of 132 kg of rice was taken for the experiment and moisture was minimised from 27% (db) to 13% (db) in 3 h of drying duration.
Abubakar et al. [55] developed a mixed/combined mode solar dryer and studied it with and without thermal storage options. The experiment was conducted in meteorological conditions in Zaria, Nigeria.
Fig. 30 describes the isometric view of the mixed/combined mode solar dryer developed by Abubakar et al. for crops. The mixed/combined mode solar dryer consists of several components: a solar collector, an air duct, an insulating chamber, and chimneys to release humidified air. The air duct connects the solar collector and the insulating chamber. This design was tested during an experiment conducted between June and August 2016, comparing the dryer’s performance in with use and without use thermal storage materials. The addition of thermal storage materials improved the dryer’s efficiency by 13%. In this case, rocks were being used as the thermal/heat storage material. During the day, the rocks absorbed heat, and at night, they released the stored heat, contributing to the drying process. The solar thermal collector and the side of the cabinet were fitted with 4 mm transparent glass to allow sunlight to enter and heat the air inside. The drying cabinet was divided into three partitions, each with a separate chimney to allow the humidified air to escape. This mixed/combined mode solar dryer was designed specifically for the drying of 7 kg of yam, a root vegetable commonly cultivated in Nigeria. During the experiment, the moisture percentage of the yam was decreased from 80% to 17% using the dryer. The performance of dryer was improved by the addition of thermal storage materials, enabling more efficient and effective drying of the yam.
Figure 30: Mixed/combined mode solar dryer developed. Reprinted from Abubakar et al. [55]
Salauddin et al. [8] developed mixed type passive dryers for the drying of various agriculturally based products. The design of the solar dryer takes into consideration the geographical location in Abeokuta, Nigeria. It can achieve a maximum temperature of 60.50°C when the atmospheric temperature is 34.50°C. To prevent heat loss, wood (specifically gmelina) is used as an insulator for the outer body of the collector and the insulating chamber. The solar collector is installed in a north-south direction and tilted at an angle of 17.110° to the horizontal, which is done to maximize solar radiation absorption. Fig. 31 in the article likely depicts the side view of the developed mixed type passive dryer. The design of the solar collector is based on the ideal gas law (PV = nRT) and a total area of 0.239 m2 has been calculated for the collector.
Figure 31: Mixed type passive dryer. Adapted from Salauddin et al. [8]
Azam et al. [56] conducted research on the thermal analysis of a photovoltaic (PV) system and a solar collector integrated with a greenhouse dryer for drying tomatoes. The solar drying system they used falls under the category of a hybrid solar drying system. Hybrid solar systems typically combine different solar dryer types like direct and indirect solar dryers. The thermal collector used had an area of 1.2 square meters and a thickness of 0.2 meters. It was set at an inclination angle of 30 degrees to the horizontal surface. The greenhouse dryer was constructed with transparent plexiglass or polycarbonate sheets. Its dimensions were 2 meters in length, 1.0 meter in width, and 0.002 meters in thickness. The experiment was conducted at the College of Agricultural and Food Science, King Faisal University, Saudi Arabia. The airflow within the greenhouse chamber was regulated by a DC blower attached at the exit point. Airflow rates tested were 2.1, 3.12 and 4.18 cubic meters per minute (m3/min). It was determined that an airflow rate of 3.12 m3/min was optimal for drying tomatoes. The experiment involved placing 9 kg of halved fresh tomatoes on a single layer within the greenhouse dryer. The diagrammatic view of an experimental setup can be seen in Fig. 32. The parameters like temperature, relative humidity and airflow rate at various stages of collector and greenhouse dryers were calculated.
Figure 32: The fabricated experimental setup of the combined dryer. Reprinted from Azam et al. [56]
The study conducted by Tonui et al. [57]. Focused on the design, development and construction of a solar based dryer with an additional provision of a biomass burner for heating backup. The purpose of the dryer was to facilitate the maize grain drying in Kenya.
Fig. 33 in the study was of the indirect natural convection type which means that it utilized natural air movement for drying the maize grain. The solar based dryer was equipped with a 3.77 m2 of thermal collector area. This collector area was responsible for harnessing solar energy and transferring it to the drying chamber. The designed solar dryer could dry 100 kg of maize per batch. This means that each drying cycle could accommodate up to 100 kg of maize grain. The primary source of heat for the drying process was the solar energy captured by the thermal collector. In addition to the solar energy, the dryer also had a biomass burner as a heating backup. This burner could be used to provide heat in case of insufficient solar radiation or during nighttime when solar energy was not available. The biomass burner utilized biomass fuel to generate heat, thereby enabling continuous drying even in unfavorable weather conditions. The study also conducted a comparative analysis of different drying methods, including open sun drying, solar dryer alone, biomass burner alone, and the combination of solar dryer and biomass burner. Presumably, this comparison aimed to analyse the performance and effectiveness of each drying method in terms of energy efficiency, drying duration and better quality of the dried maize grain. Moisture content of maize grain in open sun drying was reduced from 19.3% to 13.2% in 6 days for daily 4 h of drying. The same moisture content reduction result was obtained in the solar dryer in 3 days for 4 h of daily drying. The moisture content of maize was reduced from 19.3% to 13.7% when the solar dryer was attached to a biomass burner for 3 days and 3 h daily drying. The thermal efficiency of only solar dryers was 39.9% and increased to 57.7% when assembled with biomass heat back-up in the dryer.
Figure 33: Isometric view of solar dryer. Reprinted from Tonui et al. [57]
Shimpy et al. [58] analyzed the Design, Performance and Economic Feasibility of Domestic Solar Dryers specifically used for small rural agricultural farming. Types of solar dryers had been briefed by the author in the review paper. The Natural Convection Solar Dryers (NCSD) like tray dryers, cabinet dryer, solar conduction dryer (SCD) and stair case shaped direct dryer were studied. The temperature range observed in these NCSD dryers typically varies from 46°C to 80°C. This temperature range is suitable for drying perishable fruits and vegetables efficiently while preserving their quality and nutritional content. These solar dryers offer sustainable and eco-friendly solutions for food preservation, particularly in regions where sunlight is abundant and traditional drying methods may not be feasible or efficient. The temperature at the absorbing plate of the solar cooker/cum dryer (SCCD) was measured at 140°C when there was no load and 98.6°C when there was. The SCCD’s performance had decreased due to sun tracking. Various forced convection solar dryers (FCSD) were studied with load and no-load conditions.
Fernandes et al. [59] conducted a study for review on various solar drying devices, heat transfer and types of insulating chambers. The author reviewed various types of available solar dryers in detail. Solar dryers have been classified based on the type of insulating chambers. The cabinet, staircase and tray chambers have been explained in details. Thermal energy storage options for hybrid solar dryers make them effective in the drying process. Gravel, marble, granite, limestone etc. can be used as a Sensible Heat Storage (SHS) option which gets heated during the day and release heat in the night. Latent heat Storage (LHS) is another option for heat storage. Phase change materials (PCM) like paraffin wax can be used for heat storage. The thermochemical Energy Storage (TCES) depends on the chemical to absorb or release heat. The energy can be stored by using high energy chemical process. The amount of heat storage depends upon the endothermic reaction of heat.
4 Development and Impact of Solar Dryers on Bee Pollen Preservation
The study conducted by Dunwoody et al. [60] involved the design of a natural convection type solar dryer specifically for drying bee pollen in Colombia. Beekeepers in Colombia were facing the challenge of drying 30–40 kg of bee pollen per week, which initially contained moisture contents ranging from 19% to 34%. To address this issue, a solar dryer was developed. The bee pollen dehydrator as illustrated in Fig. 34 was constructed entirely using plywood sheets. It was designed as a passive type solar dryer along with natural air convection. The capacity of the dryer allowed for the drying of 5 kg of bee pollen per batch. Throughout the day, the moisture percentage of the bee pollen decreased from the initial range of 18% to 34% to a final range of 4% to 6%. However, since the dryer was manufactured using plywood sheets, there were issues with air leakages in the insulating chamber. These air leakages negatively affected the efficiency of the dryer.
Figure 34: Bee pollen dehydrator. Adapted from Dunwoody et al. [60]
The study conducted by Barajas et al. [61]. Focused on investigating the effect of temperature on bee pollen during solar drying. The bee pollen samples were collected from two different zones in Colombia, namely La Calera and Zipaquira. La Calera is surrounded by mountains and native woods, while Zipaquira is known for its clean pastures and suitability for grazing domestic and wild animals. The average ambient temperature in both towns was recorded as 14°C. The researchers collected a total of 1 kg of bee pollen per week from two apiaries for their experiments. The study was conducted during July and August 2008. Initially, the bee pollen pallets were cleaned to remove leaves, stray chaffs, and bees. A tray dryer without air circulation was used for the drying process, and each tray was loaded with 500 g of bee pollen. The temperature inside the tray dryer was consistently maintained at 35°C and 45°C, as recommended by apiarists. The desired final moisture percentage of the bee pollen on a wet basis (Wb) was suggested to reach 12%. The drying area was calculated to be 0.07 m2 to accommodate the loading of drying trays. Each tray contained 250 g of bee pollen. Several important parameters were considered during the experiment, including water activity, carotene content, vitamin C, solubility index, moisture content, protein, fats, ash and fibre contents. These parameters were measured to evaluate the impact of solar drying on the nutritional composition of the bee pollen samples.
The drying process was performed for 297 min at 35°C and 45°C temperature for the bee pollen from La Calera and 210 min for samples from Zipaquira. Fig. 35 illustrates the drying curves for samples dried during an experiment. There was drastic change in moisture percentage with respect to time. The moisture content of samples from La Calera was tested and recorded at fresh, 35°C and 45°C and found to be 23.82%, 9.31% and 8.29%. The moisture content of samples from Zipaquira was recorded as 19.41%, 11.23% and 7.09%. Protein, fat, ash and fiber content of sample from La Calera was also recorded at Fresh, 35°C and 45°C and observed as (27.38%, 28.19% and 28.85%), (3.8%, 4.12% and 5.1%), (2.16%, 2.09% and 2.89%) and (0.48%, 0.46% and 0.43%). The same readings were recorded for samples from Zipaquira as (28.17%, 28.45% and 28.20%), (1.14%, 1.37% and 2.2%) and (3.0%, 3.33% and 3.20%). Vitamin C and carotene content for the sample from La Calera reduced from 40.22 mg/100 gm and 0.77 mg/100 gm to 27.35 mg/100 gm and 0.51 mg/100 gm. Finally, it was observed that the protein content (28%), fiber content (0.04%) and ash content (2%–3%) of the bee pollen samples were not affected in drying process.
Figure 35: Drying curve of bee pollen collected from La Calera and Zipaquira zone. Adapted from Barajas et al. [61]
Castellanos-Paez et al. [62] specifically focused on examining the impact of solar drying on the structural and thermodynamic characteristics of bee pollen collected from Viracacha Boyaca, Colombia. A sample of 250 g of bee pollen was taken for the experiment. Solar drying was carried out separately using both natural convection and forced convection solar dryers for 9 h, from 8 am to 5 pm. Parameters such as dry bulb temperature, relative humidity, etc., were recorded at three different points within the solar dryer using a thermos-hygrometer. This monitoring helped in assessing the environmental conditions during the drying process, which could affect the quality of the dried bee pollen. A natural Convection Solar Dryer with a stainless steel tray with dimensions of 1.5 meters in length and 1 meter in width was used. The bee pollen was spread in a 1 cm layer on the tray for drying. The stainless-steel tray had a perforation of 2 mm to facilitate the transfer of heated air. Drying occurs under natural convection, where heated air rises due to temperature differences without the use of any mechanical fans. Forced Convection Solar Dryer with stainless steel tray measuring 1.2 meters in length and 0.8 meters in width was used for other results. Similar to the natural convection setup, the bee pollen was spread in a 1 cm layer on the tray for drying. This setup utilized forced convection, where air circulation was facilitated by the use of mechanical fans, enhancing the drying rate. The morphology of fresh bee pollen and pollen dried in natural convection and forced convection solar dryers were studied. The moisture content decreased from 21.03% to 6.51% after being dried in a natural convection solar dryer. Moisture reduction in the forced convection solar dryer reduced from 21.01% to 3.74%. These findings indicate that both drying methods were effective in reducing the moisture content of the bee pollen. The forced convection solar dryer appears to have been slightly more efficient in removing moisture compared to the natural convection solar dryer.
Duran et al. [63] worked on the impact of the solar drying process on bioactive compounds and antioxidant activity in vitro of high Andean region bee pollen. The study utilized bee pollen samples collected from the Andean Forest and Paramus zones of Colombia. Two distinct drying methods, namely electric-operated forced convection cabin dryer and natural convection greenhouse dryer, were employed for the drying process. Through in vitro analysis, the study assessed the preservation of bioactive compounds and antioxidant activity in the dried bee pollen samples. Fig. 36 depicts a traditional electric-operated cabin dryer measuring 66.5 cm × 95 cm × 158.5 cm, with a 0.5 cm thick wooden wall and 1.5 cm of polyurethane foam insulation. A 1500 W electric heater is utilised to heat the air and keep the flow at 2 m/s using an axial flow blower. Moist air is expelled through the air exit as shown in the diagram. To obtain a more accurate result, the pollen sample was dried for 8 h. The chamber maintains an average temperature of 50°C. The diagrammatic view of tray dryer can be seen in Fig. 36.
Figure 36: (a) Schematic diagram of the single tray with plastic mesh and wood frame, (b) perforated tray dryer and (c) thermal collector. Adapted from Duran et al. [63]
For the second experiment, a solar-powered greenhouse dryer with 8 trays carrying 700 gm of bee pollen each was employed. The experiment ran for 8 h. It is observed from the experiment that the bioactive compound and antioxidant properties of the bee pollen were effectively maintained in the solar dryer as compared to the traditional electric dryer.
5 Conclusion and Further Scope of Work
• The review paper discussed different types of solar-based dryers covering direct, indirect, active, passive, natural air convection, forced air convection and mixed/combined type dryers. These dryers are considered as alternatives to address the limitations associated with open sun drying and electricity-operated oven drying for Agri-based food products and bee pollen.
• This paper also compared the results of open sun drying, electric oven drying and solar drying on Agri-based food products and bee pollen. By analysing and reviewing the comparative data, the authors identified the benefits and disadvantages of each drying method.
• Interestingly, the review paper highlighted that only four researchers, Barajas et al., Dunwoody et al., Castellanos-Paez et al. and Duran et al. from Colombia have worked on the design of solar dryers specifically for bee pollen. Alex’s solar dryer was of passive and natural convection type. Castellanos-Paez et al. and Duran et al. specifically worked on the impact study of solar dryers on various bioactive and antioxidant components of bee pollen. Since solar dryers for drying bee pollen haven’t been developed in India. Developing solar dryers specifically tailored for drying bee pollen from different regions of India could be a promising endeavor. Since bee pollen composition may vary depending on factors such as floral sources and environmental conditions, customizing solar dryers to suit the specific characteristics of each region’s bee pollen could optimize the drying process and preserve its bioactive and antioxidant components effectively. This approach could contribute to the sustainable harvesting and processing of bee pollen, potentially benefiting both the beekeeping industry and consumers interested in its nutritional and health-promoting properties. However, the paper suggests there is still room for improvement and development of new solar dryers for bee pollen drying.
• Specifically, the authors propose the design of indirect type solar dryer with forced air convection. This type of dryer would enable the maintenance of a temperature ranging from 50–60°C, which is considered suitable for drying bee pollen.
• Smart connected devices can be utilized to monitor and record parameters such as temperature and humidity in specific sections of the thermal collector and insulating chamber. These devices can collect real-time data and transmit it to a central system or a cloud-based platform for further analysis and monitoring. By implementing sensors at strategic locations within the solar dryer, the temperature and humidity levels can be measured accurately. These sensors can be connected to a microcontroller or a data acquisition system, which then transmits the data to a central server or cloud platform.
• Overall, the review paper provides insights into different types of solar dryers, compares their effectiveness with other drying methods, and identifies areas for further research and development in the field of solar drying, particularly for bee pollen.
Acknowledgement: The author expresses gratitude to the Rohit Sharma from Vellore Institute of Technology, Bhopal, India for their continuous support and guidance in preparing this review paper. Additionally, the author extends thanks to their parent institute, Mahatma Gandhi Institute for Rural Industrialization (MGIRI), Wardha, India, for granting access to their library resources for the preparation of this review paper.
Funding Statement: No external funding source was involved in the preparation of this paper.
Author Contributions: The contributions of the authors to this paper are as follows: Ganesh There-Conceptualization and preparation of the paper; Rohit Sharma-Guidance, research paper analysis, and study. All authors reviewed the results and approved the final version of the manuscript.
Availability of Data and Materials: Data relevant to this paper are included in the article.
Ethics Approval: Not applicable.
Conflicts of Interest: The authors declare that they have no conflicts of interest to report regarding the present study.
References
1. S. Singh, R. S. Gill, V. S. Hans, and M. Singh, “A novel active-mode indirect solar dryer for agricultural products: Experimental evaluation and economic feasibility,” Energy, vol. 222, pp. 1–10, Jan. 2021. doi: 10.1016/j.energy.2021.119956. [Google Scholar] [CrossRef]
2. A. Lingayat, V. P. Chandramohan, and V. R. K. Raju, “Energy and exergy analysis on drying of banana using indirect type natural convection solar dryer,” Heat Transf. Eng., vol. 41, no. 6–7, pp. 1–11, Jan. 2019. doi: 10.1080/01457632.2018.1546804. [Google Scholar] [CrossRef]
3. A. Fudholi and K. Sopian, “A review of solar air flat plate collector for drying application,” Renew. Sustain. Energ. Rev., vol. 102, pp. 333–345, Mar. 2019. doi: 10.1016/j.rser.2018.12.032. [Google Scholar] [CrossRef]
4. J. Gustavsson, C. Cederberg, and U. Sonesson, Global food losses and food waste. Düsseldorf: The Swedish Institute for Food and Biotechnology, May 2011. [Google Scholar]
5. S. Malakar, V. K. Arora, and P. K. Nema, “Design and performance evaluation of an evacuated tube solar dryer for drying garlic clove,” Renew. Energy, vol. 168, pp. 568–580, May 2021. doi: 10.1016/j.renene.2020.12.068. [Google Scholar] [CrossRef]
6. V. Belessiotis and E. Delyannis, “Solar drying,” Sol. Energy, vol. 85, no. 8, pp. 1665–1691, Aug. 2011. doi: 10.1016/j.solener.2009.10.001. [Google Scholar] [CrossRef]
7. R. K. Sahadev, “Open sun and greenhouse drying of agricultural and food product: A review,” Int. J. Eng. Res. Technol., vol. 3, no. 3, pp. 1053–1066, Mar. 2014. [Google Scholar]
8. O Salaudeen, Design, construction and testing of a solar dryer. Abeokuta, Nigeria: University of Agriculture, pp. 1–47, Jun. 2011. [Google Scholar]
9. O. Prakash, V. Laguri, A. Pandey, A. Kumar, and A. Kumar, “Review on various modelling techniques for the solar dryers,” Renew. Sustain. Energ. Rev., vol. 62, pp. 396–417, Sep. 2016. doi: 10.1016/j.rser.2016.04.028. [Google Scholar] [CrossRef]
10. O. V. Ekechukwu and B. Norton, “Review of solar-energy drying systems II: An overview of solar drying technology,” Energy Convers. Manag., vol. 40, no. 6, pp. 615–655, Apr. 1999. doi: 10.1016/S0196-8904(98)00093-4. [Google Scholar] [CrossRef]
11. M. Hanif, “Drying of grapes using a dish type solar air heater,” J. Agric. Res., vol. 50, no. 3, pp. 423–431, Dec. 2012. [Google Scholar]
12. U. Toshniwal and S. R. Karale, “A review paper on solar dryer,” Int. J. Eng. Res. Appl., vol. 3, no. 2, pp. 896–902, Apr. 2013. [Google Scholar]
13. S. Kapil, Agri share in GDP hit 20% after 17 years: Economic survey. New Delhi: Down to Earth, pp. 1–2, Jan. 29, 2021. [Google Scholar]
14. K. B. Koua, W. F. Fassinou, P. Gbaha, and S. Toure, “Mathematical modelling of the thin layer solar drying of banana, mango and cassava,” Energy, vol. 34, no. 10, pp. 1594–1602, Oct. 2009. doi: 10.1016/j.energy.2009.07.005. [Google Scholar] [CrossRef]
15. W. Chramsa-ard, S. Jindaruksa, C. Sirisumpunwong, and S. Sonsaree, “Performance evaluation of the desiccant bed solar dryer,” Energy Proc., vol. 34, pp. 189–197, 2013. doi: 10.1016/j.egypro.2013.06.747. [Google Scholar] [CrossRef]
16. A. Pujianto, S. D. P. Sidhi, and A. Nurfauzi, “Mathematical modeling of thin layer drying of salted yellowtail fish under open sun and in greenhouse dryer,” Russ J. Agric. Socioecon. Sci., vol. 91, no. 7, pp. 391–399, Jul. 2019. doi: 10.18551/rjoas.2019-07.46. [Google Scholar] [CrossRef]
17. S. I. Anwar and G. N. Tiwari, “Evaluation of convective heat transfer coefficient in crop drying under open sun drying conditions,” Energy Convers. Manag., vol. 42, no. 5, pp. 627–637, Mar. 2001. doi: 10.1016/S0196-8904(00)00065-0. [Google Scholar] [CrossRef]
18. İ. Doymaz, “Sun drying of seedless and seeded grapes,” J. Food Sci. Technol., vol. 49, no. 2, pp. 214–220, Apr. 2012. doi: 10.1007/s13197-011-0272-9. [Google Scholar] [PubMed] [CrossRef]
19. O. Ismail and E. Akyol, “Open-air sun drying: The effect of pretreatment on drying kinetic of cherry tomato,” Sigma J. Eng. Nat. Sci., vol. 34, no. 2, pp. 141–151, Mar. 2016. [Google Scholar]
20. O. İsmail, A. Kantürk Figen, and S. Pişkin, “Open sun drying of green bean: Influence of pretreatments on drying kinetics, colour and rehydration capacity,” Heat Mass Transf., vol. 53, no. 4, pp. 1277–1288, Apr. 2017. doi: 10.1007/s00231-016-1899-x. [Google Scholar] [CrossRef]
21. A. E. L. Khadraoui, I. Hamdi, S. Kooli, and A. Guizani, “Drying of red pepper slices in a solar greenhouse dryer and under open sun: Experimental and mathematical investigations,” Innov. Food Sci. Emerg. Technol., vol. 52, pp. 262–270, Mar. 2019. doi: 10.1016/j.ifset.2019.01.001. [Google Scholar] [CrossRef]
22. N. R. Pochont, M. N. Mohammad, B. T. Pradeep, and P. V. Kumar, “A comparative study of drying kinetics and quality of Indian red chilli in solar hybrid greenhouse drying and open sun drying,” Mater. Today Proc., vol. 21, pp. 286–290, 2020. doi: 10.1016/j.matpr.2019.05.433. [Google Scholar] [CrossRef]
23. A. Agrawal and R. M. Sarviya, “A review of research and development work on solar dryers with heat storage,” Int. J. Sustain. Energy, vol. 35, no. 6, pp. 583–605, Jul. 2016. doi: 10.1080/14786451.2014.930464. [Google Scholar] [CrossRef]
24. M. Seveda, “Design of a photovoltaic powered forced convection solar dryer in NEH region of India,” Int. J. Renew. Energy Res., vol. 3, no. 4, pp. 906–912, Jan. 2013. [Google Scholar]
25. H. S. EL-Mesery, A. I. EL-Seesy, Z. Hu, and Y. Li, “Recent developments in solar drying technology of food and agricultural products: A review,” Renew. Sustain. Energ. Rev., vol. 157, pp. 112070, Apr. 2022. doi: 10.1016/j.rser.2021.112070. [Google Scholar] [CrossRef]
26. D. E. Ogheneruona and M. O. L. Yusuf, “Design and fabrication of a direct natural convection solar dryer for tapioca,” Leonardo Electron. J. Pract. Technol., no. 18, pp. 95–104, Jun. 2011. [Google Scholar]
27. E. Hakizimana, “Design and optimization of domestic solar dryer,” Sci. J. Energy Eng., vol. 5, no. 6, pp. 130–135, Jan. 2017. doi: 10.11648/j.sjee.20170506.11. [Google Scholar] [CrossRef]
28. M. S. Sodha, A. Dang, P. K. Bansal, and S. B. Sharman, “An analytical and experimental study of open sun drying and a cabinet tyre drier,” Energy Convers. Manag., vol. 25, no. 3, pp. 263–271, Jan. 1985. doi: 10.1016/0196-8904(85)90042-1. [Google Scholar] [CrossRef]
29. H. Hallak, J. Hillal, F. Hilal, and R. Rahhal, “The staircase solar dryer: Design and characteristics,” Renew. Energy, vol. 7, no. 2, pp. 177–183, Feb. 1996. doi: 10.1016/0960-1481(95)00127-1. [Google Scholar] [CrossRef]
30. P. Gbaha, H. Yobouet Andoh, J. Kouassi Saraka, B. Kaménan Koua, and S. Touré, “Experimental investigation of a solar dryer with natural convective heat flow,” Renew. Energy, vol. 32, no. 11, pp. 1817–1829, Sep. 2007. doi: 10.1016/j.renene.2006.10.011. [Google Scholar] [CrossRef]
31. A. Borah, K. Hazarika, and S. M. Khayer, “Drying kinetics of whole and sliced turmeric rhizomes (Curcuma longa L.) in a solar conduction dryer,” Inf. Process. Agric., vol. 2, no. 2, pp. 85–92, Sep. 2015. doi: 10.1016/j.inpa.2015.06.002. [Google Scholar] [CrossRef]
32. A. Lotfalian, M. Ghazavi, and B. H. samani, “Comparing the performance of two type collectors on drying process of lemon and orange fruits through a passive and indirect solar dryer,” J. Am. Sci., vol. 6, no. 10, pp. 248–251, Jan. 2010. [Google Scholar]
33. M. A. Basunia and T. Abe, “Thin-layer solar drying characteristics of rough rice under natural convection,” J. Food Eng., vol. 47, no. 4, pp. 295–301, Mar. 2001. doi: 10.1016/S0260-8774(00)00133-3. [Google Scholar] [CrossRef]
34. G. Visavale, “Principles, classification and selection of solar dryers,” in C. L. Hii, S. P. Ong, S. V. Jangam, and A. S. Mujumdar (Eds.Solar drying: Funda-mentals, Applications and Innovations, Singapore: University of Nottingham, 2012, pp. 1–50. [Google Scholar]
35. B. Basumatary et al., “Design, construction and calibration of low cost solar cabinet dryer,” In. J. Environ. Eng. Manag., vol. 4, no. 4, pp. 351–358, Nov. 2013. [Google Scholar]
36. J. Singh, M. P. Singh, M. Akhtar, and A. Khajuria, “Design and performance analysis of an indirect type solar dryer,” Iaetsd J. Adv. Res. Appl. Sci., vol. 5, no. 2, pp. 553–568, Feb. 2018. [Google Scholar]
37. O. V. Ekechukwu and B. Norton, “Experimental studies of integral-type natural-circulation solar-energy tropical crop dryers,” Energy Convers. Manag., vol. 38, no. 14, pp. 1483–1500, Sep. 1997. doi: 10.1016/S0196-8904(96)00102-1. [Google Scholar] [CrossRef]
38. C. Sarsilmaz, C. Yildiz, and D. Pehlivan, “Drying of apricots in a rotary column cylindrical dryer (RCCD) supported with solar energy,” Renew. Energy, vol. 21, no. 2, pp. 117–127, Jan. 2000. doi: 10.1016/S0960-1481(00)00077-X. [Google Scholar] [CrossRef]
39. A. Benhamou, F. Fazouane, and B. Benyoucef, “Simulation of solar dryer performances with forced convection experimentally proved,” Phys. Proc., vol. 55, pp. 96–105, Jan. 2014. doi: 10.1016/j.phpro.2014.07.015. [Google Scholar] [CrossRef]
40. P. M. Gupta, A. S. Das, R. C. Barai, S. C. Pusadkar, and V. G. Pawar, “Design and construction of solar dryer for drying agricultural products,” Int. Res. J. Eng. Technol., vol. 4, no. 3, pp. 1946–1951, Mar. 2017. [Google Scholar]
41. M. Sai Krishna Teja, K. V. Narasimha Rao, and N. R. Pochont, “Design, development and experimental evaluation of solar dryer,” Int. J. Mech. Prod. Eng. Res. Dev., vol. 8, no. 3, pp. 949–968, Jun. 2018. doi: 10.24247/ijmperdjun2018101. [Google Scholar] [CrossRef]
42. P. J. Etim, A. Ben Eke, and K. J. Simonyan, “Design and development of an active indirect solar dryer for cooking banana,” Sci. Afr., vol. 8, pp. 1–9, Jun. 2020. doi: 10.1016/j.sciaf.2020.e00463. [Google Scholar] [CrossRef]
43. K. Kolhe and S. V. Jogdand, “Development of solar dryer for selected agricultural products,” Int. Res. J. Eng. Technol., vol. 7, no. 6, pp. 960–969, Jun. 2020. [Google Scholar]
44. V. V. Pakhare and S. P. Salve, “Design and development of solar dryer cabinet with thermal energy storage for drying chilies,” Int. J. Curr. Eng. Technol., pp. 2347–5161, Jun. 2016. doi: 10.14741/ijcet/22774106/spl.5.6.2016.67. [Google Scholar] [CrossRef]
45. S. Vijayan, T. V. Arjunan, and A. Kumar, “Exergo-environmental analysis of an indirect forced convection solar dryer for drying bitter gourd slices,” Renew. Energy, vol. 146, pp. 2210–2223, Aug. 2019. doi: 10.1016/j.renene.2019.08.066. [Google Scholar] [CrossRef]
46. H. Ö. Güler et al., “Experimental and CFD survey of indirect solar dryer modified with low-cost iron mesh,” Sol. Energy, vol. 197, pp. 371–384, Jan. 2020. doi: 10.1016/j.solener.2020.01.021. [Google Scholar] [CrossRef]
47. C. B. Pardhi and J. L. Bhagoria, “Development and performance evaluation of mixed-mode solar dryer with forced convection,” Int. J. Energy Environ. Eng., vol. 4, no. 1, pp. 2251–6832, Jan. 2013. doi: 10.1186/2251-6832-4-23. [Google Scholar] [CrossRef]
48. E. Baniasadi, S. Ranjbar, and O. Boostanipour, “Experimental investigation of the performance of a mixed-mode solar dryer with thermal energy storage,” Renew. Energy, vol. 112, pp. 143–150, May 2017. doi: 10.1016/j.renene.2017.05.043. [Google Scholar] [CrossRef]
49. B. O. Bolaji and A. P. Olalusi, “Performance evaluation of a mixed-mode solar dryer,” AU J. Technol., vol. 11, pp. 225–231, Apr. 2008. [Google Scholar]
50. L. V. Erick César, C. M. Ana Lilia, G. V. Octavio, P. F. Isaac, and B. O. Rogelio, “Thermal performance of a passive, mixed-type solar dryer for tomato slices (Solanum lycopersicum),” Renew. Energy, vol. 147, pp. 845–855, Sep. 2019. doi: 10.1016/j.renene.2019.09.018. [Google Scholar] [CrossRef]
51. P. H. Oosthuizen and H. Bashagur, “Numerical evaluation of a low cost mixed flow indirect solar rice dryer,” J. Eng. Int. Dev., vol. 2, pp. 20–27, Dec. 1995. [Google Scholar]
52. J. P. Ekka, P. Muthukumar, K. Bala, D. K. Kanaujiya, and K. Pakshirajan, “Performance studies on mixed-mode forced convection solar cabinet dryer under different air mass flow rates for drying of cluster fig,” Sol. Energy, vol. 229, pp. 39–51, Nov. 2021. doi: 10.1016/j.solener.2021.06.086. [Google Scholar] [CrossRef]
53. E. Ayua, V. Mugalavai, J. Simon, S. Weller, P. Obura and N. Nyabinda, “Comparison of a mixed modes solar dryer to a direct mode solar dryer for African indigenous vegetable and chili processing,” J. Food Process. Preserv., vol. 41, no. 6, pp. 1–7, Sep. 2016. doi: 10.1111/jfpp.13216. [Google Scholar] [CrossRef]
54. A. Zomorodian, D. Zare, and H. Ghasemkhani, “Optimization and evaluation of a semi-continuous solar dryer for cereals (Rice, etc),” Desalination, vol. 209, pp. 129–135, Apr. 2007. doi: 10.1016/j.desal.2007.04.021. [Google Scholar] [CrossRef]
55. S. Abubakar, S. Umaru, M. U. Kaisan, U. A. Umar, B. Ashok and K. Nanthagopal, “Development and performance comparison of mixed-mode solar crop dryers with and without thermal storage,” Renew. Energy, vol. 128, pp. 285–298, May 2018. doi: 10.1016/j.renene.2018.05.049. [Google Scholar] [CrossRef]
56. M. M. Azam, M. A. Eltawil, and B. M. A. Amer, “Thermal analysis of PV system and solar collector integrated with greenhouse dryer for drying tomatoes,” Energy, vol. 212, pp. 1–18, Sept. 2020. doi: 10.1016/j.energy.2020.118764. [Google Scholar] [CrossRef]
57. K. S. Tonui, E. B. K. Mutai, D. A. Mutuli, D. O. Mbuge, and K. V. Too, “Design and evaluation of solar grain dryer with a back-up heater,” Res. J. Appl. Sci. Eng. Technol., vol. 7, no. 15, pp. 3036–3043, April. 2014. doi: 10.19026/rjaset.7.639. [Google Scholar] [CrossRef]
58. Shimpy, M. Kumar, and A. Kumar, “Designs, performance and economic feasibility of domestic solar dryers,” Food Eng. Rev., vol. 15, no. 1, pp. 156–186, Oct. 2022. doi: 10.1007/s12393-022-09323-1. [Google Scholar] [CrossRef]
59. L. Fernandes and P. B. Tavares, “A review on solar drying devices: heat transfer, air movement and type of chambers,” Solar Acad. Open Access Publishing, vol. 4, pp. 15–42, Jan. 2024. [Google Scholar]
60. A. Dunwoody and L. Metrulas, “Bee polley dehydrator,” California, Jun. 2015, pp. 1–34. Accessed: May 21, 2024. [Online]. Available: https://www.idin.org/resources/student-papers/bee-pollen-dehydrator [Google Scholar]
61. J. Barajas, M. Cortes-Rodriguez, and E. Rodríguez-Sandoval, “Effect of temperature on the drying process of bee pollen from two zones of Colombia,” J. Food Process. Eng., vol. 35, no. 1, pp. 134–148, Dec. 2012. doi: 10.1111/j.1745-4530.2010.00577.x. [Google Scholar] [CrossRef]
62. B. A. Castellanos-Paez, A. Durán-Jiménez, C. A. Fuenmayor, M. C. Quicazán, and C. M. Zuluaga-Domínguez, “Effects of solar drying on the structural and thermodynamic characteristics of bee pollen,” Vitae, vol. 29, no. 3, pp. 1–12, Oct. 2022. doi: 10.17533/udea.vitae.v29n3a350572. [Google Scholar] [CrossRef]
63. A. Duran, M. C. Quicazán, and C. M. Zuluaga, “Effect of solar drying process on bioactive compounds and antioxidant activity in vitro of high Andean region bee pollen,” Chem. Eng. Trans., vol. 75, pp. 91–96, Nov. 2019. doi: 10.3303/CET1975016. [Google Scholar] [CrossRef]
Cite This Article
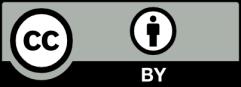
This work is licensed under a Creative Commons Attribution 4.0 International License , which permits unrestricted use, distribution, and reproduction in any medium, provided the original work is properly cited.