Open Access
ARTICLE
Surface Morphology and Thermo-Electrical Energy Analysis of Polyaniline (PANI) Incorporated Cotton Fabric
1 Automotive Engineering Research Group, Faculty of Mechanical and Automotive Engineering Technology, Universiti Malaysia Pahang Al-Sultan Abdullah, Pekan, Malaysia
2 Department of Textile Engineering, Khulna University of Engineering & Technology (KUET), Khulna, Bangladesh
3 Centre for Research in Advanced Fluid and Processes (CARIFF), Universiti Malaysia Pahang Al-Sultan Abdullah, Lebuhraya Tun Razak, Gambang, Malaysia
4 Mechanical Engineering Programme Area, Faculty of Engineering, Universiti Teknologi Brunei, Brunei, Darussalam
* Corresponding Author: Md. Mustafizur Rahman. Email:
Energy Engineering 2024, 121(1), 1-12. https://doi.org/10.32604/ee.2023.027472
Received 31 October 2022; Accepted 28 February 2023; Issue published 27 December 2023
Abstract
With the exponential development in wearable electronics, a significant paradigm shift is observed from rigid electronics to flexible wearable devices. Polyaniline (PANI) is considered as a dominant material in this sector, as it is endowed with the optical properties of both metal and semiconductors. However, its widespread application got delineated because of its irregular rigid form, level of conductivity, and precise choice of solvents. Incorporating PANI in textile materials can generate promising functionality for wearable applications. This research work employed a straightforward in-situ chemical oxidative polymerization to synthesize PANI on Cotton fabric surfaces with varying dopant (HCl) concentrations. Pre-treatment using NaOH is implemented to improve the conductivity of the fabric surface by increasing the monomer absorption. This research explores the morphological and structural analysis employing SEM, FTIR and EDX. The surface resistivity was measured using a digital multimeter, and thermal stability is measured using TGA. Upon successful polymerization, a homogenous coating layer is observed. It is revealed that the simple pre-treatment technique significantly reduces the surface resistivity of Cotton fabric to 1.27 kΩ/cm with increasing acid concentration and thermal stability. The electro-thermal energy can also reach up to 38.2°C within 50 s with a deployed voltage of 15 V. The modified fabric is anticipated to be used in thermal regulation, supercapacitor, sensor, UV shielding, antimicrobial and other prospective functional applications.Keywords
Nomenclature
nm | Nanometer |
µm | Micrometer |
Ω | Ohm |
GSM | Gram square per meter |
HCl | Hydrochloric acid |
Flexible electronics have recently drawn enormous interest from researchers worldwide [1]. Previously, the fundamental constituents of formulating these devices were metallic constituents like fillers/wires/particles [2,3]. Despite substantial advances, the use of metallic compounds for cutting-edge applications is constrained by their higher prices, availability, lack of biocompatibility, and mechanical vulnerability (such as corrosion) for human contact [4,5]. Additionally, due to their rigid characteristics and lack of comfort, metallic elements are of least interest in wearable performance [6,7]. When the term wearable and functional is considered, textile-based materials are given special consideration because of their superior flexibility, mechanical stability, breathability, and lightweight properties. Additionally, wearable devices, elements, and connections can be embedded within the fabric to draw less notice yet remain functional [8]. However, it is necessary to transform the electrically inert textile substrate into a conductive surface to make it suitable for practical applications [9,10]. Numerous conductive materials are used to perform this action [11,12]. The choice of conductive materials and properties primarily depends on the requisite functionality and application area [12]. Using metallic substances to induce conductivity on the textile surface was primarily used in earlier cases; however, their incorporation showed some detrimental effects on fabric pliability, increasing weight, and an overall decrease in comfort [13]. Conductive polymers (CPs) predominate due to their availability, simplicity in synthesis, integration with textile surfaces, and processability [14,15]. CP’s inherent conductivity comes from delocalized π-electrons in the conjugated backbone, transforming into a conduction band [16]. This band generates a continuous conducting layer on the material’s surface and throughout its structure [17].
Polyaniline, Polypyrrole, MXene, Graphene, PEDOT, Carbon nanotubes, and different metallic components are predominantly used among various conductive materials. Incorporating these conductive materials with textiles resulted in impressive outcomes [18]. However, critical issues like flexibility, stability, and washing durability after integrating with textile substrates remained challenging. As a result, widespread progress of these materials with textile surfaces was hindered [19]. In this regard, Polyaniline is selected due to its facile synthesis process, low-cost monomer, excellent electrochemical performance, compatibility with textile surfaces, doping-dedoping cycle, inherent conductivity, and outstanding environmental stability [20]. However, using PANI with other solvents poses several complexities in forming coherent bonds [21]. Moreover, for some advanced functionalization, enhanced conductivity is required. Polyaniline often suffers from lower conductivity with textiles, eventually restricting its extensive application [22]. Therefore, the advancement in electrical attributes of PANI is a prerequisite for innovative applications.
The morphology and thermoregulatory analysis needed to be explored for practical applications. Notably, thermogravimetric analysis helps to comprehend the thermal stability of a polymer under predefined temperatures and heating rates while in a heated condition. Moreover, it is anticipated that intriguing conventional textile fabric with conductive PANI can augment the inherent potentials of the material and trigger complex functional features like thermal heating pads and functional fabric for the advanced automotive sector [23]. Although conductive polymer, PANI-based materials are endowed with promising features to be used in thermoregulatory responsive materials, extensive research using PANI-based materials in this field is still inadequate. Therefore, it is necessary to explore materials with PANI inclusions to develop futuristic wearable electronics. Current research is focused on formulating PANI-coated Cotton fabric by in-situ polymerization, its morphological changes, thermal stability, electrical performance analysis, and the evaluation for thermo-electrical performance.
Untreated 100% Cotton fabric (woven, GSM 95) was procured from a local factory in Bangladesh (Noman Group). The primary chemicals were purchased from the mentioned condition and sources: Aniline (Sigma Aldrich, 99% Purity), Ammonium Persulphate (Sigma Aldrich, 98%), Sodium hydroxide (v/V 5%, RM Chemical, Malaysia), Hydrogen chloride (Conc. 37%, RM Chemical, Malaysia), Acetone (Conc. 99.5%, RM Chemicals, Malaysia). Double distilled water is used for the whole study.
2.2 Formulation of Polyaniline/Cotton Fabric
The raw Cotton fabric was cut into a dimension of 15 cm × 5 cm. Pretreatment on the fabric was performed to remove the adhered dirt and oil and improve the absorbency of the fabric. This process is performed by soaking the material in a Sodium hydroxide (5%) solution for 10 min. The treated fabric is then washed with distilled water, followed by drying (at 60°C) [15]. A monomer solution was prepared for this experiment by adding 2 ml of Aniline into 50 ml of HCl solution of varied concentrations (1, 2, and 3 M). 50 ml each of a 1, 2, and 3 M HCl solution and 5 g of ammonium persulphate (APS) were mixed, respectively. The polymerization process was commenced upon the addition of respective APS solution dropwise. This reaction is anticipated to be exothermic. Subsequently, to keep the reaction temperature under 5°C, the whole reaction media was performed in an ice bath [24]. The reaction continued for 1.5 h. Meantime, with the reaction progression, the color of the solution changes from Blue to Green. With the dropwise addition of APS (Oxidant), the Aniline mixed Dopant solution starts the first phase of the reaction by forming a Polyaniline emeraldine base (PANI-EB). It was Blue, and upon continuous addition of APS solution, the solution turned to emeraldine green. This color change indicates the successful formation of Polyaniline (PANI). A glass rod was occasionally stirred to maintain a homogeneous reaction throughout the reaction bath. The PANI-coated fabric was removed from the reaction bath after completing the polymerization. The solution is filtered, followed by subsequent drying (at 60°C) to collect the PANI particle for further characterization. Meanwhile, any residual unfixed Aniline monomers are removed using acetone and thoroughly washed with distilled water. As a final step, the fabric sample was dried (at 60°C) in a woven overnight and then ready for characterization.
The surface topology of both untreated Cotton fabric and PANI-coated fabric was examined using JEOL (JSM IT-200) with an accelerating voltage of 5 and 15 kV, respectively, and at magnification rates of 50×, 250×, 2000×, and 5000×. The same machine was used to analyze the elemental mapping. The surface resistivity of the PANI-treated Cotton fabric was assessed using a digital multimeter (UNI-T, UT61E+). While the thermogravimetric analysis (TGA) was carried out using a TGA analyzer (TGA Q500 V6.7 Build 203). FTIR spectra of control Cotton fabric and Polyaniline-treated Cotton fabric at different concentrations were evaluated using Thermo Fisher Scientific FTIR Spectrometer (Model: Nicolet iS5; 4.16 A, 12 V DC, USA) at 32 scans in the frequency region of 3500–400 cm−1.
From the PANI-coated Cotton fabric, noticeable differences can be observed with plain eyesight. Due to successful in-situ polymerization, the appearance of the treated fabric changes from white to emeraldine green. This color change signifies the successful impregnation of emeraldine salt on Cotton fabric [25]. The surface orientation can also be observed from the Scanning Electron Microscopic (SEM) image. The surface of the untreated Cotton is notably more smooth, cylindrical, and flat, as seen in Fig. 1a. In contrast, Fig. 1b reveals a different version of the surface with rough and irregular granular particles adhered to the fiber strand. It is further observed from the other magnified images.
Figure 1: SEM micrographs of (a) untreated Cotton fabric; (b) PANI-coated Cotton fabric (50×) magnification
Upon successful polymerization, the PANI particles adhere to the fabric surface in irregular granular forms. The shape of the PANI dendrites is not well defined. However, they flocked together on the fabric surface, as shown in Figs. 2b and 2c. Their formation can be justified compared to the pristine Cotton surface as in Fig. 2a. A dispersion of PANI was seen along the length of the fiber in the magnified SEM picture Fig. 2d. Additionally, the deposited PANI concentration is greater around the ridges and pores than on the surface. It can be understood that the synthesized PANI made it to the inner spaces of the fiber. Furthermore, Fig. 2d reveals a thin, film-like formation behind the PANI cluster formation, which affirms the continuous layer of PANI on the yarn strand. This was also validated by elemental analysis and electrical conductivity analysis.
Figure 2: SEM Micrographs of (a) untreated Cotton fabric (2000×) magnification; PANI-coated Cotton at (b) 250× (c) 2000× and (d) 5000× magnification
Additional size distribution analysis revealed the range of particle sizes using ImageJ software. The average size of the PANI is 91.98528 nm. The marked areas are depicted in Fig. 3a, and the Histogram is in Fig. 3b.
Figure 3: Particle size distribution of PANI incorporated fabric (a) chosen particles; (b) particle size distribution
From the elemental mapping (Fig. 4) presence of polymer substances and a uniform layer of distributed materials are observed throughout the outer layer of the Cotton fabric. The presence of N (Nitrogen) confirms the formation of PANI. Cl- is due to treatment with HCl. Although the main constituent is C, (Carbon) comes from the Cellulose structure. However, a homogenous distribution of other elements, specially N, is observed through the fabric surface. This signifies a successful polymerization and a coating layer.
Figure 4: Elemental mapping of the PANI-deposited fabric
To assess the functional group of the Cotton fabric and PANI-deposited Fabric, FTIR-ATR was performed on the samples. FTIR-ATR spectras (Fig. 5) show the chemical structure of Plain Cotton fabric and PANI-deposited Cotton fabric at varried dopant concentrations (1, 2, 3 M).
Figure 5: FTIR-ATR spectra of 100% Cotton fabric and PANI-deposited Cotton fabric at a different dopant concentration
The plain Cotton fabric displayed the board absorption peak at 3268–3332 cm−1 region, assigned to the O-H stretching band of Cellulose. Consequently, there were several regions, for example, vibration at 1111, 1160 cm−1 for C-O-C stretching, 2900 cm−1 subjected to C-H stretching band, 2900 cm−1 for C-H stretching band, and 1638 cm−1 assigned for H-O-H bending absorbed water molecules.
Upon PANI deposition, the peak at the 3268–3332 cm−1 region disappeared. Alongside, distinctive quinoid and benzenoid peaks are noticed at 1551 and 1474 cm−1, respectively. Peaks for secondary amines from PANI formation are also located at 1294 cm−1. Additional regions and distinctive peaks are also depicted in Table 1.
4.4 PANI Impregnation and Increase in Electroconductive Behavior
The conductive nature of the Cotton fabric is largely affected by the impregnation of PANI. With the PANI deposition, the surface becomes conductive. The resistivity measurement of the PANI-coated surface can justify this phenomenon.
Primarily, it is observed that the conductivity is significantly affected by the pretreatment process with NaOH. The alkaline process enhances the roundness of the surrounding fiber strand at the peripheral regions. As a result of penetration of the alkaline solution, the whole fiber strand is swollen, and the inner spiral formation of fiber got a more rounded shape and space [31] (Fig. 5a). As a result, the absorbency of the cellulosic fiber increased as well. This swollen structure housed a comparatively large number of PANI particle inside than non-treated ones, reducing the resistivity significantly.
The presence of conjugated lone pair in the PANI backbone does not make it conductive unless a charge carrier is introduced. Doping enables the electrons to flow due to the generation of conduction bands and making PANI conductive. Acid (HCl) doping protonates the imine nitrogen atoms without exchanging the electrons [32]. With the increase in dopant concentration, more protonation occurred in the nitrogen atom resulting in more conductivity of PANI-deposited fabric. It is observed that the resistivity with the lowering with the increase in dopant concentration (Fig. 6b). Significant improvement in resistivity was observed (15 folds) as the successful deposition conductive PANI.
Figure 6: (a) Swollen state of the PANI fiber upon treated with NaOH; (b) resistivity of PANI incorporated Cotton fabric at different dopant concentrations
Fig. 7a illustrates the repeatability of the resistivity value upon the continuous measurement from different parts of the coated fabric (3 M HCl). And from it, it can be presumed that the coating is well performed on the surface by creating a continuous, homogenous layer of PANI. From Figs. 7b and 7c, it can the flexibility of the treated fabric is retained in its original form.
Figure 7: PANI coated Cotton fabric (3 M HCl) (a) repeatability of the resistivity and flexibility of the (b) untreated sample, (c) treated sample
4.5 Thermogravimetric Analysis (TGA)
The thermal stability of the PANI-incorporated Cotton fabric was analyzed using TGA. From the curve (as shown in Fig. 8), a small initial weight loss (3%–7%) was observed at the temperature between 40°C~50°C; this could be attributed to the presence of moisture or solvents in the samples. All the samples exhibited prominent endothermic peaks at a temperature over 300°C, indicating the major weight loss started from this point. This might be due to the breakage of the Cellulose backbone of the Cotton [33]. This phase continues from the temperature of 370°C~380°C. Here, the peak for PANI grafted fabric slightly shifted to a lower temperature than pristine Cotton, implying a reduction in thermal stability. This might be ascribed to the effect of acid on the Cellulose structure of Cotton. Notably, the PANI with a 3 M dopant concentration showed the minimum of weight loss compared with other concentrations (e.g., 1, 2 M). Thus, 3 M dopant concertation exhibited better thermal stability among the rest concentration by shifting the degradation point from 365°C to 390°C (3 M). Moreover, the residue of the thermal degradation of PANI (3 M HCl)-Cotton fabric is the highest. This might be due to the structural changes caused by much stronger acid and larger surface area for oxidation [34].
Figure 8: TGA thermogram of (a) untreated Cotton; (b) PANI (3 M HCl) deposited Cotton fabric; (c) TGA curve at different dopant concentrations
4.6 Evaluation of Electro-Thermal Energy
The promising electrical conductivity and thermal stability of the PANI-coated fabric hold considerable potential to be used as a flexible thermo-regulated device. It is notable that upon providing a current supply to conductive substances, heat generation occurs upon the colloid of electrons and photons. This phenomenon is practiced via joule heating. The joule heating behavior of the treated Cotton fabric (3 M HCl, 3.5 cm2 × 1 cm2) was evaluated using a DC power supply operating at 1–15 V. The heat signature and surface distribution were examined using an inferred camera (FLIR E4). A progressive temperature rise is monitored with a gradual increase in the voltage depicted in Fig. 9. Consequently, upon the immediate release of the input voltage, the heat quickly declines to the initial ambient temperature. The surface temperature of the fabric elevated rapidly from ambient temperature to 30°C when a 5 V voltage was applied. Temperature raised from 30°C to 35°C within 15 s with the increase in voltage from 5 to 10 V. Within the next time span from 15–30 s, the temperature rose rapidly; at 15 V, the flexible thermos-electrical composite fabric provided elevated temperature that can exceed human skin temperature (38.2°C) and eventually reached a steady state of condition.
Figure 9: Electro-thermal characterization of PANI-coated fabric
The current study demonstrated a simple synthesis procedure to incorporate Polyaniline (PANI) into Cotton fabric and assessed its structural stability and thermographic stability. Additionally, SEM, Elemental Analysis, and EDX are used to evaluate surface morphology. The thermal stability and electrical performance of the PANI grafted cloth are also assessed. The pretreatment step (with NaOH) has been found to significantly enhance the textile material’s electrical properties. The Cotton fabric coated with 3 M HCl exhibited better conductivity than other dopant concentrations, demonstrating that the increase in doping concentration resulted in the formation of long and stable PANI chains, thus improving the conductivity and thermal stability. The electrical resistivity of the Cotton knitted fabric attenuated to 1.27 kΩ/cm for pre-treated fabric via in situ polymerization. The induced thermal energy can also reach up to 38.2°C within 50 s with a deployed voltage of 15 V. It is also projected that the flexible and lightweight nature of the conductive fabric will open a new frontier in cutting-edge thermoregulatory, supercapacitor, shielding, and many more potential applications. Moreover, the higher yield of synthesis implies bulk production-based implementation.
Acknowledgement: The authors would like to thank all the lab instructors and facilitators of Universiti Malaysia Pahang (UMP), Gambang, Malaysia, for providing necessary laboratory facilities, characterization, and financial support.
Funding Statement: This work is supported by the International Publication Research Grant No. RDU223301 and Postgraduate Research Grant Scheme, UMP, Malaysia (PGRS210370).
Author Contributions: Md. Shohan Parvez: Writing, Original draft, Conceptualization, Methodology, Investigation, Formal analysis, Data curation, Visualization. Md. Mustafizur Rahman and Mahendran Samykano: Conceptualization, Writing–review & editing, Supervision, Methodology, Investigation, Data curation, Validation. Mohammad Yeakub Ali: Writing–review & editing, Validation.
Availability of Data and Materials: Data will be made available on request.
Conflicts of Interest: The authors declare that they have no conflicts of interest to report regarding the present study.
References
1. Zhao, Y., Kim, A., Wan, G., Tee, B. C. K. (2019). Design and applications of stretchable and self-healable conductors for soft electronics. Nano Convergence, 6(1), 1–22. [Google Scholar]
2. Wu, W. (2019). Stretchable electronics: Functional materials, fabrication strategies and applications. Science and Technology of Advanced Materials, 20(1), 187–224. [Google Scholar]
3. Naghdi, S., Rhee, K. Y., Hui, D., Park, S. J. (2018). A review of conductive metal nanomaterials as conductive, transparent, and flexible coatings, thin films, and conductive fillers: Different deposition methods and applications. Coatings, 8(8), 278. [Google Scholar]
4. Stoppa, M., Chiolerio, A. (2014). Wearable electronics and smart textiles: A critical review. Sensors, 14(7), 11957–11992. [Google Scholar] [PubMed]
5. Sazonov, E., Daoud, W. A. (2021). Grand challenges in wearable electronics. Frontiers in Electronics, 2, 668619. [Google Scholar]
6. Ometov, A. (2021). A survey on wearable technology: History, state-of-the-art and current challenges. Computer Networks, 193, 108074. [Google Scholar]
7. Song, P., He, X., Xie, M., Tao, J., Shen, X. et al. (2020). Polyaniline wrapped graphene functionalized textile with ultrahigh areal capacitance and energy density for high-performance all-solid-state supercapacitors for wearable electronics. Composites Science Technology, 198, 108305. [Google Scholar]
8. Uddin, F. (2019). Introductory Chapter Textile manufacturing processes. In: Textile manufacturing processes, pp. 1–14. IntechOpen. [Google Scholar]
9. Tseghai, G. B. D., Mengistie, A., Malengier, B., Fante, K. A., Langenhove, L. V. (2020). PEDOT: PSS-based conductive textiles and their applications. Sensors, 20(7), 1881. [Google Scholar] [PubMed]
10. Kalaoglu-Altan, O. I., Kayaoglu, B. K., Trabzon, L. (2022). Improving thermal conductivities of textile materials by nanohybrid approaches. iScience, 25(3), 103825. [Google Scholar] [PubMed]
11. Repon, M. R., Mikučionienė, D. (2021). Progress in flexible electronic textile for heating application: A critical review. Materials, 14(21), 6540. [Google Scholar] [PubMed]
12. Barakzehi, M., Montazer, M., Sharif, F., Norby, T., Chatzitakis, A. (2019). A textile-based wearable supercapacitor using reduced graphene oxide/polypyrrole composite. Electrochimica Acta, 305, 187–196. [Google Scholar]
13. Muthukumar, N., Thilagavathi, G. (2012). Development and characterization of electrically conductive Polyaniline coated fabrics. Indian Journal of Chemical Technology, 19(6), 434–441. [Google Scholar]
14. Stejskal, J. (2020). Conducting polymers are not just conducting: A perspective for emerging technology. Polymer International, 69(8), 662–664. [Google Scholar]
15. Maity, S., Chatterjee, A. (2018). Conductive polymer-based electro-conductive textile composites for electromagnetic interference shielding: A review. Journal of Industrial Textiles, 47(8), 2228–2252. [Google Scholar]
16. Namsheer, K., Rout, C. S. (2021). Conducting polymers: A comprehensive review on recent advances in synthesis, properties and applications. RSC Advances, 11(10), 5659–5697. [Google Scholar]
17. Zhang, F., Feng, Y., Feng, W. (2020). Three-dimensional interconnected networks for thermally conductive polymer composites: Design, preparation, properties, and mechanisms. Material Science and Engineering, R: Reports, 142, 100580. [Google Scholar]
18. Omar, S. N. I., Ariffin, Z. Z., Zakaria, A., Safian, M. F., Mahat, M. M. (2020). Electrically conductive fabric coated with Polyaniline: Physicochemical characterisation and antibacterial assessment. Emergent Materials, 3(4), 469–477. [Google Scholar]
19. Harito, C., Utari, L., Putra, B. R., Yuliarto, B., Walsh, F. C. (2020). Review—The development of wearable polymer-based sensors: Perspectives. Journal of the Electrochemical Society, 167(3), 037566. [Google Scholar]
20. de Souza, F. G., Barradas, T. N., de Freitas Caetano, V., Lovera, A. B. (2021). Nanoparticles and polyaniline electrical conductivity. Qeios, 1–42. [Google Scholar]
21. Gupta, R., Singhal, M., Chaudhary, J. P. (2022). Regulation of nanostructured polyaniline synthesis and its properties through organic solvent in interfacial polymerization. Colloids and Surfaces A: Physicochemical and Engineering Aspects, 632, 127806. [Google Scholar]
22. Gicevicius, M., Cechanaviciute, I. A., Ramanavicius, A. (2020). Electrochromic textile composites based on polyaniline-coated metallized conductive fabrics. Journal of Electrochemical Society, 167(15), 155515. [Google Scholar]
23. Parvez, M. S., Rahman, M. M., Samykano, M., Ali, M. Y. (2023). Current advances in fabric-based airbag material selection, design and challenges for adoption in futuristic automobile applications. Materials Today: Proceedings. https://doi.org/10.1016/j.matpr.2023.09.081 [Google Scholar] [CrossRef]
24. Prasutiyo, Y. J., Manaf, A., Hafizah, M. A. E. (2020). Synthesis of Polyaniline by chemical oxidative polymerization and characteristic of conductivity and reflection for various strong acid dopants. Journal of Physics: Conference Series, 1442(10), 012003. [Google Scholar]
25. Roslan, N. C., Aizamddin, M. F., Omar, S. N. I., Jani, N. A., Halim, M. I. A. et al. (2020). Morphological and conductivity studies of polyaniline fabric doped phosphoric acid. Malaysian Journal of Analytical Sciences, 24(5), 698–706. [Google Scholar]
26. Ahmad, I., Kan, C., Yao, Z. (2019). Reactive Blue-25 dye/TiO2 coated cotton fabrics with self-cleaning and UV blocking properties. Cellulose, 26(4), 2821–2832. [Google Scholar]
27. Mousli, F., Khalil, A. M., Maurel, F., Kadri, A., Chehimi, M. M. (2020). Mixed oxide-polyaniline composite-coated woven cotton fabrics for the visible light catalyzed degradation of hazardous organic pollutants. Cellulose, 27(13), 7823–7846. [Google Scholar]
28. Parvez, M. S., Rahman, M. M., Samykano, M., Ali, M. Y. (2023). Electrochemical characterization and joule heating performance of Polyaniline incorporated cotton fabric. Journal of Physics, Chemistry and Earth, Parts A/B/C, 129, 103323. [Google Scholar]
29. Patil, A. J., Deogaonkar, S. C. (2012). A novel method of in situ chemical polymerization of Polyaniline for synthesis of electrically conductive cotton fabrics. Textile Research Journal, 82(15), 1517–1530. [Google Scholar]
30. Kang, E. (1998). Polyaniline: A polymer with many interesting intrinsic redox states. Progress in Polymer Science, 23(2), 277–324. [Google Scholar]
31. Azeem, F., Mao, N., Faisal, S. (2019). Effect of alkali treatment of lower concentrations on the structure and tensile properties of Pakistan’s coarse cotton fibre. The Journal of the Textile Institute, 110(10), 1499–1507. [Google Scholar]
32. Khalid, M., Honorato, A. M. B., Varela, H. (2018). Polyaniline: Synthesis methods, doping and conduction mechanism. In: Polyaniline-from synththesis to practical applications, pp. 1–17. IntechOpen. [Google Scholar]
33. Tissera, N. D., Wijesena, R. N., Rathnayake, S., de Silva, R. M., de Silva, K. M. N. (2018). Heterogeneous in situ polymerization of Polyaniline (PANI) nanofibers on cotton textiles: Improved electrical conductivity, electrical switching, and tuning properties. Carbohydrate Polymers, 186, 35–44. [Google Scholar] [PubMed]
34. Mahat, M. M., Aizamddin, M. F., Che Roslan, N., Kamarudin, M. A., Omar, S. N. I. et al. (2020). Conductivity, morphology and thermal studies of polyaniline fabrics. Journal of Mechanical Engineering, 9(1), 137–150. [Google Scholar]
Cite This Article
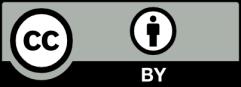
This work is licensed under a Creative Commons Attribution 4.0 International License , which permits unrestricted use, distribution, and reproduction in any medium, provided the original work is properly cited.