Open Access
ARTICLE
Experimental Analyses of Moderately High-Temperature Heat Pump Systems with R245fa and R1233zd(E)
1 State Key Laboratory of Fluid Power & Mechatronic Systems, Zhejiang University, Hangzhou, 310027, China
2 Clean Energy R&D Department, Korea Institute of Industrial Technology, Cheonan, 31056, Republic of Korea
* Corresponding Author: Oh Kyung Kwon. Email:
Energy Engineering 2022, 119(6), 2231-2242. https://doi.org/10.32604/ee.2022.021289
Received 06 January 2022; Accepted 23 May 2022; Issue published 14 September 2022
Abstract
With the limited production and use of R245fa, environmentally friendly refrigerant has attracted the attention of researchers. Due to the similar thermal characteristics, R1233zd(E) is considered to be an ideal substitute for R245fa in heat pump systems. In this study, the performance and economic analysis of heat pump systems with R245fa and R1233zd(E) as refrigerants are carried out. The results show that the total cost of R1233zd(E) system is more than 10% higher than that of R245fa system under the same heating load. With the increase of condensation temperature, the heating capacity of both systems decreases, and with the increase of evaporation temperature, the heating capacity increases. The variation trend of coefficient of performance (COP) of the two systems is similar to that of heating capacity. Under the same operating conditions, the COP of R1233zd(E) system is 19.2% higher than that of R245fa system, and the volumetric heat capacity of R1233zd(E) is 9.0%–13.9% lower than that of R245fa. The economic analysis results show that the investment cost of R1233zd(E) system is low under the same heat load.Keywords
Nomenclature
Ai | heat transfer area [m2] |
Cenv | CO2 emission penalty cost [$] |
Cim | investment and maintenance cost [$] |
Cop | operational cost [$] |
cp | specific heat [kJ kg−1K−1] |
total cost rate [$ s−1] | |
COP | coefficient of performance [−] |
CRF | capital recovery factor [−] |
i | interest rate [−] |
m | mass flow rate [kg h−1] |
n | system life time [year] |
P | pressure [kPa] |
Q | heat [kJ] |
T | temperature [°C] |
VHC | volumetric heat capacity [kJ m−3] |
W | electricity consumption [kW] |
Greek symbols | |
η | efficiency [−] |
μ | emission factor [−] |
ρ | density [kg m−3] |
τ | operation hours per year [h] |
φ | maintenance factor [−] |
Subscripts | |
comp | compressor |
cond | condenser |
evap | evaporator |
ev | expansion valve |
in | inlet |
out | outlet |
r | refrigerant |
rat | ratio |
sk | heat sink side |
sou | heat source side |
sub | subcooling |
suc | suction |
sup | superheating |
w | water |
wf | working fluid |
In industrial processes, heat is usually generated through fossil fuel combustion. Insufficient combustion will cause serious environmental pollution and energy waste, however. Mateu-Royo et al. [1] reported that about 2.8% of industrial energy is discharged as low-grade heat (below 100°C). The recovery of low-grade heat and its transformation into high-grade heat would reduce the use of primary energy and greenhouse gas emissions. In the traditional natural gas combined cycle power plant (CCPP), the cooling of steam at the steam turbine outlet needs to consume a lot of cooling water. The outlet temperature of the cooling water is about 40°C, and the low-grade waste heat storage in the cooling water is huge, which has a good recovery prospect.
In 2011, Rattner et al. [2] reported that fossil fuel usage can be significantly reduced by recovering and upgrading the waste energy. However, such large amounts of waste energy remain unrecovered owing to technical difficulties or economic considerations. A large portion of the waste heat energy is low-quality heat (below 60°C), which cannot be efficiently used unless it is upgraded. Therefore, developing high-efficiency high-temperature heat pump (HTHP) systems for waste heat recovery has attracted considerable attention [3–5]. Assaf et al. [6] experimentally studied the application of a heat pump in the food industry using a 50°C waste steam to produce a 75°C steam. Bamigbetan et al. [7] experimentally investigated a hydrocarbon HTHP using 30°C~60°C waste heat to produce heat up to 115°C. Mahdi et al. [8] evaluated the thermoeconomic and environmental feasibilities of an air source heat pump by recovering the waste heat of a data center under different conditions. Hu et al. [9] developed a high-efficient centrifugal heat pump for district heating and heat recovery. The testing results show that the COP of 500RT PSF(permanent-magnetic synchronous frequency-convertible) centrifugal heat pump could reach 7.1, and the total system COP of the district heating system reaches 4.8. Hybrid systems have been also investigated to improve the performances of heat pump systems. In the study of Liu et al. [10], an advanced absorption-compressor heat pump for recovering the sensible heat of flue gas is investigated. A 151.8°C high-temperature steam was produced by using flue gas below 150°C as a heat source. Farshi et al. [11] have introduced a cascaded compression-absorption heat pump for waste heat recovery application. The results show that the cascaded system performs better at high-temperature lifts than compression, absorption, and hybrid heat pumps.
R245fa, which has a global warming potential (GWP) of 858, has been widely used as a working fluid for HTHP [12–14]. Bobelin et al. [15] experimentally studied a blend of R245fa(ECO3) to provide heat up to 140°C. In addition, the feasibility of other refrigerants like R1234ze(E), R365mfc, R236fa, and R717 were studied theoretically. The Kigali Amendment to the Montreal Protocol that reached in 2016 had set a schedule of the consumption and production of HFCs. Considering the restrictions on HFCs (Hydrochlorofluorocarbons), alternatives with lower GWP are increasingly used. As a refrigerant with a GWP of 4.7~7 and almost no ODP, R1233zd(E) is considered the next-generation refrigerant is replacing R245fa [16]. Owing to their similarities in thermal properties, R1233zd(E) has the potential to replace R245fa directly without changes to existing systems [17]. The application of R1233zd(E) in heat pumps have been extensively studied [18–22]. Arpagaus et al. [23,24] investigated the performance of R1336mzz(Z), R1233zd(E), and R1224yd(Z) in a laboratory-scale HTHP with up to 150°C supply temperature. The experimental results showed that R1233zd(E) and R1336mzz(Z) are more efficient than R1224yd(Z) at 150°C heat sink temperature due to higher critical temperatures; the system with an internal heat exchanger is 15%~24% higher than that of the basic cycle; by increasing the temperature glide at heat sink side from 5K to 30K, a further 15% COP increase was achieved. A theoretical study on low GWP HFO and HCFO refrigerants with heat sink temperatures up to 160°C are conducted by Arpagaus et al. [25]. The results showed that R1336mzz(Z) achieved the highest COP in the temperature range from 120°C to 160°C. Studies on heat pump systems using R245fa or R1233zd(E) are listed in Table 1, and the main thermal properties of R245fa and R1233zd(E) are listed in Table 2.
From the above review, most research about R1233zd(E) heat pump was carried out theoretically, as the compressor for R1233zd(E) is not yet commercially available. However, the theoretical studies are usually developed by assuming that the compressor is well developed with a certain efficiency. It makes that theoretical results may differ from experimental ones a lot. The economic analysis of R1233zd(E) heat pump is hard to be conducted. Although few of them were experimentally investigated, they focused on the recovery of the high-temperature waste heat of 80°C–90°C, the recovery of waste heat with a temperature around 35°C by using R1233zd(E) heat pump has not been reported yet. The main objectives of this study are to test R245fa and R1233zd(E) heat pumps prototypes by using a commercial available compressor, and to present the experimental results of the heat pumps for low-temperature heat recovery. An economic comparison between R245fa and R1233zd(E) heat pump systems is also carried out.
2.1 Experimental Setup and Conditions
A schematic of the experimental setup is shown in Fig. 1a, and the experimental setup is shown in Fig. 1b. The heat pump system consists of a compressor, condenser, evaporator, receiver, and expansion valve. A water flow meter and several pressure and temperature transducers are installed in the loop. The compressor model is 2DES-3Y and is manufactured by Bitzer. The electrical expansion valve made by Danfoss is adopted, and two plate heat exchangers are adopted for the condenser and evaporator, respectively. An Oval type U mass flow meter is used to detect the mass flow rate of the heat pump cycle. Two water cycles are used as a heat source and heat sink, respectively. The system is well insulated with foam insulation materials.
Figure 1: (a) Schematic of the heat pump system; (b) Experimental set-up
Temperature is detected through resistance temperature detectors, which have an uncertainty of ±0.05°C. Pressure transducers have an accuracy of 0.13% and are used to detect pressure. The mass flow meter, which can measure two-phase flow, has an accuracy of ±0.1%. The transducers are connected to a data collector, connected to a computer. All experimental data are obtained under a stable operation of the system. And the experiment process is conducted as follows: when condensing temperature or evaporating temperature is changed, the mass flow rate of the heat pump cycle is adjusted to the same value.
The experimental conditions are listed in Table 3. The waste heat source is the cooling water of a conventional combined cycle power plant; it has a temperature of 40°C, and the evaporating temperature is set as 35°C. The compressor is operated at a frequency of 60 Hz. Two commercial plate heat exchangers (Alfa Laval AC70X-22MX) are used as evaporators and condensers. The commercially purchased Honeywell Solstice® R1233zd(E) is used in the present study.
An uncertainty analysis is carried out using
where f is the objective function and x, y, and z are the measured data.
The COP, defined as the ratio of the output and input energies, is calculated as
where W is the work input of the compressor and Qcond is the heat transfer of the condenser,
The volume of the HTHP system is a critical factor for practical applications. A smaller volume usually leads to a lower cost. In this regard, volumetric heating capacity (VHC) is defined as
where vsuc is the volumetric flow rate,
For a heat pump system, the total cost rate is calculated as
where
The investment and maintenance cost Cim is defined as
where φ is the maintenance factor, CRF is the capital recovery factor that can be assessed as
where i is the interest rate, and n is the system lifetime.
The evaporator and condenser in the present study are plate heat exchangers. The capital cost can be expressed as a function of its heat transfer area,
Capital cost of the compressor and expansion valve are calculated as
The operational cost is calculated as
The CO2 emission penalty cost is
where CCO2 is the cost of CO2 avoided, and the amount of CO2 emission mCO2 is obtained by
where
The maintenance factor is 1.06, the interest rate in South Korea is assumed to be 5%, the lifetime of the system is 15 years, and the annual operational hours are assumed to be 5000 h. The CO2 emission factor is considered to be 0.968 kg kWh−1, the electricity price is 0.101 $ kWh−1, and the cost of the CO2 avoided is 87 $ ton−1.
Fig. 2 shows the experimentally determined heating capacity variations with the condensation and evaporation temperatures. According to Eq. (1), the uncertainty of the heating capacity is smaller than 3%. For both R1233zd(E) and R245fa systems, the heating capacity tends to decrease with the increase in condensation temperature and increase with the evaporation temperatures. This can be explained as with the increase in condensation temperature, the evaporation temperature is maintained at 35°C. The temperature lifting is increased, and thus the compression ratio is also increased. Since the compressor is operated under a certain frequency of 60 Hz, the mass flow rate is reduced. When the evaporation temperature is increased, the difference between the evaporation and condensation temperatures is increased, which implies that the compression ratio is reduced. Thus a larger amount of refrigerant can be compressed. Under the same operation conditions, the heating capacity of R1233zd(E) is over 10% higher than that of the R245fa system. When the condensing temperatures of the heat pump systems are the same, R1233zd(E) refrigerant has a lower condensing pressure than R245fa. The lower condensing pressure means that the requirements for equipment are reduced under the same safe operation standard, which is conducive to cost-saving. At the same time, R1233zd(E) heat pump system can provide a higher heating capacity.
Figure 2: Heating capacity variation with different conditions
Fig. 3 shows the experimentally determined COP variations with the condensation and evaporation temperatures. According to Eq. (1), the uncertainty of the COP is smaller than 3%. The COP continues to decreases with the increase in condensation temperature in the range of 66°C to 74°C. Under the same operation conditions, the COP of the R1233zd(E)system, on average, is 19.2% higher than that of the R245fa system. Although the heating capacity significantly increases as the evaporation temperature increases, the electricity consumed by the compressor also increases. Considering the combined effect of increasing heating capacity and electricity consumption, the COP increases slightly. Mateu-Royo et al. [1] have also compared the COPs of R245fa and R1233zd(E) heat pump systems. They have reported similar results; the COP of the R1233zd(E)system was 5%–25% higher than that of the R245fa system. The figure shows that when the condensing temperature is 74°C, the COP of R1233zd(E) heat pump system is still close to 5, and the condensing temperature of the heat pump system has great potential to continue to increase.
Figure 3: COP variation with different conditions
The experimentally determined VHC variations with the condensation and evaporation temperatures are shown in Fig. 4. Disregarding the uncertainty of the refrigerant properties, the uncertainty of the VHC is smaller than 2%. The variation in VHC with the condensation temperature is tiny. The VHC of the R1233zd(E) system is reduced by 0.76%, while that of the R245fa system is increased by 3.2%. This indicates that the VHC is not sensitive to variations in condensation temperature. At a condensation temperature range of 66°C to 74°C, the VHC of the R1233zd(E) system is 9.0% lower than that of the R245fa system, mainly because of the density of R1233zd(E) sucked into the compressor is lower than that of R245fa. The thickness is calculated according to the measured pressure and temperature at the compressor inlet. With the increase in evaporation temperature in the range of 32°C to 38°C, the VHC increases for both R1233zd(E) and R245fa systems by 22.3% and 13.7%, respectively. This indicates that the VHC is sensitive to variations in condensation temperature. In the evaporation temperature range of 32°C to 38°C, the VHC of the R1233zd(E) system is 13.9% lower than that of the R245fa system, mainly because the density of R1233zd(E) sucked into the compressor is lower than that of R245fa. These results agree with those reported by Kondou et al. [20]. The high VHC value of the R1233zd system shows that the volume of the system can be further optimized, and the cost is expected to reach a lower level than the R245fa heat pump system.
Figure 4: VHC variation with different conditions
Figs. 5 and 6 show the variations of the total capital cost per hour and the total capital cost per MkW against condensation and evaporation temperatures. For both R1233zd(E) and R245fa systems, the total cost is increased with the increase in condensation and evaporation temperatures. At a condensation temperature range of 66°C to 74°C, the total cost of the R245fa system is 4.0% higher than that of the R1233zd(E) system. Since the same experimental equipment is used, the total cost difference is caused by the CO2 emission penalty and the operational cost. The CO2 emission penalty and the operational cost are determined by the electricity consumed by the compressor, which is slightly higher in the R245fa system. For the same heating load, which is 1000 kW in the present study, it is observed that the total cost of the R245fa system is considerably higher than that of the R1233zd(E) system. At a condensation temperature range of 66°C to 74°C, the total cost of the R245fa system is 17.6% higher than that of the R1233zd(E) system. At an evaporation temperature range of 32°C to 38°C, the total cost of the R245fa system is 12.4% higher than that of the R1233zd(E) system. It means that R1233zd(E) system would be a more economical alternative compared to the R245fa system.
Figure 5: Variations of the total cost with condensing temperature in R1233zd(E) and R245fa systems
Figure 6: Variations of the total cost with evaporating temperature in R1233zd(E) and R245fa systems
In this study, moderately HTHP systems using R245fa and R1233zd(E) were studied experimentally. The experimental data of R245fa and R1233zd(E) systems using low-temperature waste heat are presented. The economic differences between the R245fa and the R1233zd(E) systems are compared. Overall, the R1233zd(E) heat pump shows great potential to replace the R245fa heat pump for low-temperature heat recovery both economically and practically. The following main conclusions can be summarized:
1. Under the same operation conditions, the heating capacity of the R1233zd(E) heat pump system is over 10% higher than that of the R245fa system. When the condensing temperatures of the heat pump systems are the same, R1233zd(E) refrigerant has a lower condensing pressure than R245fa, which is conducive to cost-saving.
2. The COP of the R1233zd(E) system was higher than that of the R245fa. Therefore, The condensing temperature of the R1233zd(E) heat pump system has great potential to continue to increase.
3. The VHC of the R1233zd(E) system was lower than that of the R245fa system, it means that the volume of the R1233zd(E) system can be further optimized, and the cost is expected to reach a lower level than R245fa heat pump system.
4. The R1233zd(E) system is more economical than the R245fa system; the total cost of the R1233zd(E) system is over 10% higher than the R245fa system under the same heating load.
Funding Statement: This work was supported by the Korea Institute of Energy Technology Evaluation and Planning (KETEP) grant funded by the Korean Government (MOTIE) (No. 20202020900060, The development and application of operational technology in smart farm utilizing waste heat from particulates reduced smokestack).
Conflicts of Interest: The authors declare that they have no conflicts of interest to report regarding the present study.
References
1. Mateu-Royo, C., Navarro-Esbrí, J., Mota-Babiloni, A., Amat-Albuixech, M., Molés, F. (2019). Thermodynamic analysis of low GWP alternatives to HFC-245fa in high-temperature heat pumps: HCFO-1224yd(ZHCFO-1233zd(E) and HFO-1336mzz(Z). Applied Thermal Engineering, 15, 762–777. DOI 10.1016/j.applthermaleng.2019.02.047. [Google Scholar] [CrossRef]
2. Rattner, A., Garimella, S. (2011). Energy harvesting, reuse and upgrade to reduce primary energy usage in the US. Energy, 36(10), 6172–6183. DOI 10.1016/j.energy.2011.07.047. [Google Scholar] [CrossRef]
3. Jakobs, R., Cibis, D., Laue, H. (2010). Status and outlook: Industrial heat pumps. International Refrigeration and Air Conditioning Conference at Purdue, 2282. West Lafayette, USA. [Google Scholar]
4. Bor, V., Ferreira, I., Kiss, A. (2015). Low grade waste heat recovery using heat pumps and power cycles. Energy, 89, 864–873. DOI 10.1016/j.energy.2015.06.030. [Google Scholar] [CrossRef]
5. Little, A. B., Garimella, S. (2011). Comparative assessment of alternative cycles for waste heat recovery and upgrade. Energy, 36(7), 4492–4504. DOI 10.1016/j.energy.2011.03.069. [Google Scholar] [CrossRef]
6. Assaf, K., Zoughaib, A., Sapora, E., Peureux, J., Clodic, D. (2010). Experimental simulation of a heat recovery heat pump system in food industries. International Refrigeration and Air Conditioning Conference at Purdue, 2311. West Lafayette, USA. [Google Scholar]
7. Bamigbetan, O., Eikevik, T., Neksa, P., Bantle, M., Schlemminger, C. (2019). The development of a hydrocarbon high temperature heat pump for waste heat recovery. Energy, 173(3–4), 1141–1153. DOI 10.1016/j.energy.2019.02.159. [Google Scholar] [CrossRef]
8. Mahdi, D. D., Sajad, V. N. (2019). Thermoeconomic and environmental feasibility of waste heat recovery of a data center using air source heat pump journal of cleaner production. Journal of Cleaner Production, 219, 117–126. DOI 10.1016/j.jclepro.2019.02.061. [Google Scholar] [CrossRef]
9. Hu, B., Liu, H., Wang, R. Z., Li, H., Zhang, Z. et al. (2017). A high-efficient centrifugal heat pump with industrial waste heat recovery for district heating. Applied Thermal Engineering, 125(3), 359–365. DOI 10.1016/j.applthermaleng.2017.07.030. [Google Scholar] [CrossRef]
10. Liu, C., Jiang, Y., Han, W., Kang, Q. (2018). A high-temperature hybrid absorption-compression heat pump for waste heat recovery. Energy Conversion and Management, 172, 391–401. DOI 10.1016/j.enconman.2018.07.027. [Google Scholar] [CrossRef]
11. Farshi, L. G., Khalili, S., Mosaffa, A. H. (2018). Thermodynamic analysis of a cascaded compression–Absorption heat pump and comparison with three classes of conventional heat pumps for the waste heat recovery. Applied Thermal Engineering, 128, 282–296. DOI 10.1016/j.applthermaleng.2017.09.032. [Google Scholar] [CrossRef]
12. Mateu-Royo, C., Navarro-Esbrí, J., Mota-Babiloni, A., Amat-Albuixech, M., Molés, F. (2018). Theoretical evaluation of different high-temperature heat pump configurations for low-grade waste heat recovery. International Journal of Refrigeration, 90, 229–237. DOI 10.1016/j.ijrefrig.2018.04.017. [Google Scholar] [CrossRef]
13. Mounier, V., Mendoze, L. C., Schiffmann, J. (2017). Thermo-economic optimization of an ORC driven heat pump based on small scale turbomachinery and comparison with absorption heat pumps. International Journal of Refrigeration, 81, 96–110. DOI 10.1016/j.ijrefrig.2017.05.021. [Google Scholar] [CrossRef]
14. Chen, T., Bae, K. J., Kwon, O. K. (2018). Empirical correlation development of R245fa flow in electronic expansion valves. International Journal of Refrigeration, 88, 284–290. DOI 10.1016/j.ijrefrig.2018.01.014. [Google Scholar] [CrossRef]
15. Bobelin, D., Bourig, A. (2012). Experimental results of a newly developed very high temperature industrial heat pump (140°C) equipped with scroll compressors and working with a blend refrigerant. International Refrigeration and Air Conditioning Conference at Purdue, 1299. West Lafayette, USA. [Google Scholar]
16. Yang, J., Ye, Z., Yu, B., Ouyang, H., Chen, J. (2019). Simultaneous experimental comparison of low-GWP refrigerants as drop-in replacements to R245fa for Organic Rankine cycle application: R1234ze(ZR1233zd(Eand R1336mzz(E). Energy, 173(1), 721–731. DOI 10.1016/j.energy.2019.02.054. [Google Scholar] [CrossRef]
17. Datla, B. V., Brasz, J. J. (2014). Comparing R1233zd and R245fa for low temperature ORC applications, International Refrigeration and Air Conditioning Conference. http://docs.lib.purdue.edu/iracc/1524. [Google Scholar]
18. Ju, F., Fan, X., Chen, Y., Zhang, H., Wang, T. et al. (2017). Performance assessment of heat pump water heaters with R1233zd(E)/HCs binary mixtures. Applied Thermal Engineering, 123, 1345–1355. DOI 10.1016/j.applthermaleng.2017.05.137. [Google Scholar] [CrossRef]
19. Zuhlsdorf, B., Meesenburg, W., Ommen, T. S., Thorsen, J. E., Markussen, W. B. et al. (2018). Improving the performance of booster heat pumps using zeotropic mixtures. Energy, 154, 390–402. DOI 10.1016/j.energy.2018.04.137. [Google Scholar] [CrossRef]
20. Kondou, C., Koyama, S. (2015). Thermodynamic assessment of high-temperature heat pumps using Low-GWP HFO refrigerants for heat recovery. International Journal of Refrigeration, 53(1), 126–141. DOI 10.1016/j.ijrefrig.2014.09.018. [Google Scholar] [CrossRef]
21. Lee, S. J., Shon, B. H., Jung, W., Kang, Y. T. (2018). A novel type solar assisted heat pump using a low GWP refrigerant (R-1233zd(E)) with the flexible solar collector. Energy, 149(17), 386–396. DOI 10.1016/j.energy.2018.02.018. [Google Scholar] [CrossRef]
22. Arpagaus, C., Bless, F., Uhlmann, M., Schiffmann, J., Bertsch, S. S. (2018). High temperature heat pumps: Market overview, state of the art, research status, refrigerants, and application potentials. Energy, 152(1), 985–1010. DOI 10.1016/j.energy.2018.03.166. [Google Scholar] [CrossRef]
23. Arpagaus, C., Bertsch, S. S. (2019). Experimental results of HFO/HCFO refrigerants in a laboratory scale HTHP with up to 150°C supply temperature. 2nd Conference on High Temperature Heat Pumps, pp. 1–9. Copenhagen, Denmark. https://orbit.dtu.dk/en/publications/book-of-presentations-of-the-2nd-symposium-on-high-temperature-he. [Google Scholar]
24. Arpagaus, C., Kuster, R., Prinzing, M., Bless, F., Uhlmann, M. et al. (2019). High temperature heat pump using HFO and HCFO refrigerants-system design, simulation, and first experimental results. 25th IIR International Congress of Refrigeration, pp. 1–9. Montréal, Québec, Canada. DOI 10.18462/iir.icr.2019.242. [Google Scholar]
25. Arpagaus, C., Prinzing, M., Kuster, R., Bless, F., Uhlmann, M. et al. (2019). High temperature heat pumps-theoretical study on low GWP HFO and HCFO refrigerants. 25th IIR International Congress of Refrigeration, pp. 1–8. Montréal, Québec, Canada. DOI 10.18462/iir.icr.2019.259. [Google Scholar]
Cite This Article
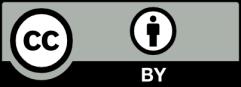
This work is licensed under a Creative Commons Attribution 4.0 International License , which permits unrestricted use, distribution, and reproduction in any medium, provided the original work is properly cited.