Open Access
REVIEW
The Metaverse Review: Exploring the Boundless Ream of Digital Reality
School of Computer Science and Technology, Zhoukou Normal University, Zhoukou, 466001, China
* Corresponding Author: Shi Dong. Email:
Computers, Materials & Continua 2024, 81(3), 3451-3498. https://doi.org/10.32604/cmc.2024.055575
Received 01 July 2024; Accepted 17 October 2024; Issue published 19 December 2024
Abstract
The metaverse has emerged as a prominent topic with growing interest fueled by advancements in Web 3.0, blockchain, and immersive technologies. This paper presents a thorough analysis of the metaverse, showcasing its evolution from a conceptual phase rooted in science fiction to a dynamic and transformative digital environment impacting various sectors including gaming, education, healthcare, and entertainment. The paper introduces the metaverse, details its historical development, and introduces key technologies that enable its existence such as virtual and augmented reality, blockchain, and artificial intelligence. Further this work explores diverse application scenarios, future trends, and critical challenges including data privacy, technological limitations, and integration issues that must be addressed for the metaverse to reach its full potential. The significance of this study lies in its comprehensive nature, providing insights not only for researchers and practitioners but also for policymakers aiming to navigate the complexities of the metaverse and leverage its capabilities for societal advancements. Finaly, the paper forecast the future where the metaverse plays an integral role in reshaping human interaction, commerce, and creativity, thus emphasizing the need for ongoing research and collaborative efforts to unlock its vast possibilities.Keywords
In today’s rapidly evolving society, emerging technologies like virtual reality, artificial intelligence, and blockchain are converging, driving the rise and advancement of a new digital realm known as the metaverse. The concept of the metaverse can be traced back to Neal Stephenson’s novel in 1992 [1]. The term “meta-” is derived from Greek, meaning “beyond,” while “verse” refers to the entirety of something. Therefore, etymologically, the metaverse represents a space that transcends our current reality, offering an immersive experience in another world. Metaverse, as a comprehensive concept that integrates multiple cutting-edge technologies such as virtual reality, augmented reality, blockchain, and artificial intelligence, is gradually moving from science fiction to real-world applications and becoming an important topic in the digital age.
The metaverse is a digital space consisting of virtual worlds, digital assets, and intelligent devices, creating a new environment for production and life as we embrace the digital age. Its emergence will bring about profound changes and impacts on human society, the economy, culture, and ways of life. Given these implications, conducting in-depth research on the concept, development process, key technologies, application scenarios, future trends, technological challenges, and development directions of the metaverse is valuable for promoting the advancement of the digital era and fostering the innovation and application of information technology.
However, the development of the metaverse is not smooth sailing. While pursuing technological innovation and application expansion, we are also facing many challenges. How can we protect user privacy and data security? How do we balance the relationship between the virtual and the real? How can we build a fair and sustainable metaverse ecosystem? These issues require us to think deeply and explore further. Therefore, the objective of this paper is to provide a comprehensive review of metaverse research. It aims to analyze the current status and future trends of metaverse-related technologies, explore the application scenarios of the metaverse across different fields, and serve as a valuable reference for further research and application in related domains.
The main contributions of this review are listed as follows:
• It describes the concept, characteristics and development of metaverse, and introduces the key technology about metaverse.
• It introduces the application scenarios of the metaverse. At the same time, some challenges and limitations of metaverse technologies are discussed in the paper.
• New trends and future directions are pointed out, with the aim of helping other researchers better understand the current state of research and the path ahead in the field.
The structure of the following paper: Section 2 introduces the overview of the metaverse. Section 3 discusses the key technology for constructing the metaverse. The application scenarios of the metaverse is introduced in the Section 4. Section 5 shows the technical challenges. Section 6 gives out the limitations of metaverse technologies. Section 7 presents the future development trends of the metaverse. Finally, conclusions are drawn in Section 8.
2.1 The Concept of the Metaverse
Since 2021, the term “metaverse” has gained significant attention and has become ingrained in our lives, particularly due to its association with the pandemic’s impact. Domestically and internationally, the metaverse has captured the interest of internet giants and industrial enterprises. Various stakeholders are attempting to define the metaverse or expand its concept based on its current development. However, as the metaverse is still in its early stages of development [2], there is currently no standardized or universally accepted definition. This article aims to provide an overview of different definitions proposed by scholars through a comprehensive literature review.
Kraus et al. [3] argue that the metaverse is not an entirely new concept in network technology but rather represents a new technological concept and trend. It signifies the culmination of various emerging technologies and heralds the next phase of the network era see for example how other technologies are being used Table 1. Specifically, the metaverse can be broadly defined as a vast virtual reality network world that integrates cutting-edge technologies such as blockchain, cloud computing, artificial intelligence, virtual reality, 3D, 5G, and potentially even 6G.
According to Yemenic [12], the metaverse can be defined as a three-dimensional digital society built on digital technology. In this society, individuals participate as digital identities, and the virtual and real worlds are seamlessly integrated.
Chen et al. [13] assert that the metaverse is an unavoidable progression toward social informatization and virtualization, marking what is seen as the final stage of internet evolution. The transition of the Internet’s core focus from information to people is driving ongoing advancements in media technology, which in turn are bringing about significant changes in contemporary society and paving the way for a new social structure in the future.
Chen and colleagues [14] state that the metaverse is the product of the cumulative development of numerous technologies to date, integrating various advanced technologies. Rather than being a completely new concept, it can be seen as a reimagining of a classic concept. The metaverse embodies the convergence of new technologies such as virtual reality, blockchain, cloud computing, digital twin, and artificial intelligence.
According to Freyermuth [15], the metaverse is a collection of digital social forms that arise from the deep integration of intelligent technology clusters. It is characterized by immersive experiences, integration of virtual reality, virtual avatars, and the establishment of independent ecosystems.
In summary, different scholars offer diverse definitions of the metaverse from various perspectives. They agree that the metaverse represents a virtual world where users can interact, socialize, and learn through key technologies such as virtual reality, interaction, and blockchain. This transformative concept will bring comprehensive changes to the development of human society. These scholars’ definitions indicate that the future metaverse will have a profound impact on our lives. In Table 2 the metaverse is compared with digital twin and traditional methods. It is poised to become a significant trend in digitization, as Wang Jue has comprehensively analyzed in their work [16].
The metaverse holds immense commercial value and development potential, finding applications in fields such as entertainment, social networking, education, and healthcare.
2.2 The Characteristics of the Metaverse
Based on the literature review, it is evident that there are diverse perspectives on the characteristics of the metaverse. The metaverse can be categorized into three types: technology facilitating interaction among all entities, technology enabling the fusion of virtual and real worlds, and technology promoting decentralization. American metaverse analyst Matthew Ball proposes six characteristics that the metaverse should possess: sustainability, real-time capabilities, unrestricted access, economic functionality, connectivity, and creativity. Dr. Gong Caichun, the author of the White Paper, identifies the core characteristics of the metaverse as immersive experiences, virtual avatars, the metaverse economy, and metaverse social governance, Fig. 1. Wu Tong and others argue that the metaverse represents an advanced form of the token economy characterized by sustainability, openness, autonomy, and immersion. In summary, the key characteristics of the metaverse can be roughly summarized as follows:
Figure 1: Characteristics of metaverse
The metaverse exhibits several distinctive characteristics. Firstly, in terms of hyper-spatiotemporal attributes, the metaverse is composed of vast amounts of data that represent the time and space within the virtual world. Users access the metaverse through digital avatars, essentially becoming “virtual humans,” enabling them to transcend the limitations of physical space and time and providing them with an immersive experience beyond reality.
Secondly, decentralization plays a crucial role in the metaverse, primarily implemented through blockchain technology. The metaverse strives for complete decentralization, ensuring that data is securely stored in separate blocks protected by algorithms. Encryption technology is utilized to enhance the security of data and address transactional security concerns for users within the metaverse.
Thirdly, open participation is a fundamental characteristic of the metaverse. It fosters an open ecosystem that encourages user-driven innovation and creativity. Users have the freedom to build metaverse modules and facilitate collaborative interconnections among different entities, promoting a diverse and dynamic metaverse environment.
Lastly, the metaverse demonstrates the fusion of virtual and real elements. This fusion encompasses various stages, including digital twins, virtual primitives, virtual and real symbiosis, and virtual and real linkage. With the continuous advancement of technologies and devices such as VR, AR, MR, and 3D restoration, the boundaries between the physical and virtual worlds blur. Sensory experiences encompassing vision, hearing, touch, taste, and smell are interconnected, and economic and cultural systems seamlessly integrate. As a result, users enter an immersive environment where the line between reality and virtuality becomes indistinguishable, providing them with an astonishing virtual reality experience within the metaverse.
2.3 The Development Course of the Metaverse
The concept of the metaverse, first introduced in Neal Stephenson’s 1992 science fiction novel “Snow Crash,” described a parallel virtual world alongside physical reality. A significant milestone occurred in 2003 with the launch of Second Life by Linden Lab, the first successful metaverse platform, allowing users to create and interact in a virtual environment.
Technology companies began actively developing the metaverse following this. Facebook’s acquisition of Oculus VR in 2014 demonstrated a commitment to virtual reality technology, and the release of “Fortnite” by Epic Games in 2017 highlighted the metaverse’s growing influence.
Recent advancements in technologies such as 5G, artificial intelligence, and blockchain have propelled the metaverse into a new phase. The year 2021 marked a significant increase in scholarly interest and research, indicating the metaverse’s expanding impact.
The metaverse’s development can be categorized into different stages. According to Ning et al. [22], there are three stages: the creation of a vast virtual world, the coexistence of virtual and physical beings, and the integration of virtual society into the physical world. Duan et al. [2] expanded on this by defining the metaverse’s stages as follows: the virtual stage with foundational technologies like extended reality and blockchain, the integration of physical senses into the virtual world, and the seamless perceptual integration of virtual and physical realms. Researchers [23] found that metaverse’s development can be divided into four stages:infancy stage, early stage, mature stage and final stage. The initial exploration focused on application and design, but the field still has room for breakthroughs and innovation.
3 Key Technologies for Constructing the Metaverse
Metaverse technology is a fusion of multiple digital technologies, as illustrated in Fig. 2. The key technologies include blockchain, human-computer interaction, artificial intelligence, networking, computing, the Internet of Things, and electronic gaming. Additionally, other significant technologies like digital twins, communication, high-performance computing, and cloud computing also play a role. Upon reviewing various articles on metaverse-related technologies, a connection was found between certain aspects, such as interaction, blockchain, artificial intelligence, and high-performance computing, leading to their integration. Based on previous works, this article primarily analyzes the applications of blockchain technology, interaction technology, artificial intelligence, digital twin technology, edge computing, the Internet of Things, and networking in the metaverse [24]. In addition to describing the roles that each of these technologies play in the metaverse, this article also provides definitions of the nine major technologies and includes relevant technical diagrams.
Figure 2: Technologies needed for metaverse to work efficiently
As with other technologies, the main users of the metaverse are humans. In fact, the metaverse will create its own virtual society, utilizing metaverse interfaces such as smart wearable devices. Human users can interact and control their avatars [25–28] for socializing, work, and play [29]. A mindmap is depicted in Fig. 3.The metaverse enables two-way interaction, allowing avatars to affect the real world. For example, an avatar can make a video call to their human friends or colleagues, purchase real-world goods and services, and more.
Figure 3: Human user functions in the metaverse
3.1.1 Human Digitization: Avatar
In the metaverse, human-like animated characters, known as avatars, are generated. These avatars are created by initially capturing photogrammetry scans of individuals and then processing them [30,31]. Before creating human avatars in the metaverse, it is essential to understand the psychological factors that influence user acceptance. Concepts like the Proteus Effect, Body Ownership (BO), and the Illusion of Virtual Body Ownership (IVBO) are key to this understanding. These ideas trace back to the famous 1998 rubber hand experiments, which revealed the phenomenon of BO-where individuals perceived a rubber hand as part of their own body [32]. By applying such findings, digital avatars in the metaverse can be designed to evoke a stronger sense of ownership and presence, enhancing user immersion and acceptance.
In the context of the metaverse, it is imperative to ensure that users experience the IVBO and BO effects for an immersive virtual reality experience. This is typically achieved through an action synchronization mechanism, where movements of the physical hand are mirrored in the virtual hand. For instance, when the physical hand moves, the virtual hand should replicate the same motion. Moreover, establishing visiomotor synchrony between the physical body and avatar imitation helps induce the IVBO effect, leading to the BO effect [25]. Feng et al. [33] demonstrate a process for capturing human subjects using commodity scanning hardware to generate digital characters from four poses, constructing 3D models, and registering them for animation within minutes. The resulting digital representations are suitable for simulations, games, and other virtual character applications. Visconti et al. [34] compare two real-time methods for conveying expressions in virtual reality through full-body avatars: one utilizing dedicated hardware for facial and eye tracking, and the other employing an algorithm that generates facial motion from audio. A user study found that facial tracking was more effective than lip sync in conveying sadness and disgust, with no significant differences observed for anger and surprise. Batnasan et al. [35] create a prototype avatar capable of mimicking Arabic sign language gestures for use in metaverse applications, enhancing research and educational opportunities for people with hearing loss and beyond. Min et al. [36] propose a framework for constructing a digital twin in the petrochemical industry, integrating IoT, machine learning, and a practice loop for information exchange to optimize production control. This framework addresses challenges such as high data dimensions and time lags. A case study demonstrates that this innovative approach effectively enhances intelligent manufacturing by enabling real-time adaptation to market changes and improving economic benefits. Gesslein et al. [31] present BodyDigitizer, an open-source, high-resolution photogrammetry-based 3D body scanner that uses up to 96 Raspberry Pi cameras. It is designed to provide an affordable solution for creating person-specific avatars for applications such as mixed reality health projects. Tong et al. [37] present a novel system for capturing 3D full human body models using multiple Microsoft Kinect devices. The approach effectively registers various body parts from different views while managing non-rigid deformations, resulting in quick and efficient generation of personalized avatars for applications in 3D animation and virtual reality. Villani et al. [38] proposed the concept of a “Physical Metaverse” facilitated by the “Avatarm.” This avatar is equipped with a hidden robotic arm, enabling physical manipulation of objects within the metaverse. The innovative interface aims to bridge the gap between digital and physical interactions, creating the illusion that avatars can directly manipulate objects. This enhancement is expected to improve user engagement and interaction in immersive virtual environments. Yoon et al. [39] presented a taxonomy for avatar-based interactions in virtual worlds, categorizing various scenarios according to avatar types, flexibility, fidelity, and interaction features. By incorporating recent enabling technologies, the study aimed to position current metaverse research and interaction platforms within this framework. This approach demonstrates the viability and utility of the proposed taxonomy for understanding and enhancing user interactions in the metaverse. Kato et al. [40] introduce the XR (Cross Reality) Telexperience Portal as a method to connect remote real spaces and past environments with the metaverse, thereby enhancing participation in business and entertainment. The study also addresses the need for highly realistic avatars in 3D metaverse communication by proposing a method for generating natural facial expressions, which improves the reliability of avatars as conversation partners. To explore further advancements in avatar creation research, interested readers can refer to the following works [41–43].
3.1.2 Social Interaction and Community Building
The metaverse is a social space where users can connect, collaborate, and build communities. Communication tools like voice chat, text, gestures, and even non-verbal cues are integral to fostering these interactions. Virtual spaces within the metaverse are designed to facilitate both casual and structured social interactions, from virtual coffee shops to collaborative workspaces and large-scale events.
3.1.3 Privacy, Security, and Ethical Considerations
The metaverse raises important questions about privacy, data security, and ethical use of information. Users need to be aware of how their data is collected, used, and shared, and they should have control over their digital identities. Ethical considerations also include addressing issues like cyberbullying, harassment, and ensuring safe and respectful environments for all users.
In the context of the metaverse, a digital twin refers to creating an exact replica of a physical object within a virtual world [36,44–46]. The term “digital twin” was initially introduced in a white paper in 2003 [47]. Digital twin technology stands at the forefront of the Industry 4.0 revolution. With advanced data analytics and the Internet of Things (IoT) leveraging high-band connectivity, it is set to revolutionize various domains such as manufacturing, healthcare, gaming, power systems [48] and more.
A significant milestone in digital twin research was marked by the National Aeronautics and Space Administration (NASA), which published an article titled “The Digital Twin Paradigm for Future NASA and U.S. Air Force Vehicles” [44]. This publication spurred a new research trend. NASA provides the following definition for a digital twin [49]: “A Digital Twin is an integrated multiphysics, multi-scale, probabilistic simulation of an as-built vehicle or system that uses the best available physical models, sensor updates, fleet history, etc., to mirror the life of its corresponding flying twin” [44]. Han et al. [50] introduce a dynamic hierarchical framework for the metaverse, which enables multiple virtual service providers (VSPs) to share digital twins (DTs) of physical objects while optimizing synchronization intensities to improve service quality. By employing an evolutionary game and a Stackelberg differential game approach, the authors demonstrate that incentivizing Internet of Things devices can enhance data sensing and control. Their study provides both theoretical and experimental evidence of the framework’s effectiveness compared to traditional methods. Nguyen et al. [51] present a metaverse framework for scalable control of robot swarms, which integrates the physical and virtual worlds through digital twins and control agents. By enabling human operators to manage swarms using a limited number of virtual agents, the approach simplifies swarm control. The effectiveness of this framework is demonstrated in a case study involving uncrewed ground vehicles (UGVs) and a virtual uncrewed aerial vehicle (UAV), revealing improved task performance with higher levels of autonomy. Chua et al. [52] address the challenges of real-time detection of adversarial patches in augmented reality (AR)-assisted driving by offloading the processing of captured physical scenes to a Metaverse Map Base Station (MMBS). They formulated an optimization problem aimed at maximizing detection accuracy while minimizing transmission latency and battery consumption. To solve this, they propose a Heterogeneous Action (HA) algorithm, which demonstrated improved performance over baseline models through extensive experiments. Jagatheesaperumal et al. [53] introduce a digital twin framework that integrates semantic communication with metaverse technologies to enhance performance in smart industrial applications. The effectiveness of this framework is demonstrated through a use case focused on shop floor management. Jagatheesaperumal et al. [54] proposed a service-oriented digital twin architecture that leverages metaverse-enabled platforms to enhance human interactions with cyber-physical systems (CPS) in Industry 5.0 scenarios. The study highlights the transformative potential of technologies such as the Internet of Everything (IoE), virtual/augmented reality (VR/AR), and extended reality (XR). Digital Twin is an active research area with lots of opportunities for improvements [55–57]. Digital Twin technology mainly includes the following core components.
3.2.1 Physical Entity and Digital Model
The actual object, system, or environment being mirrored in the digital world could be anything from a machine part or an entire factory to a human organ or a city. A virtual representation of the physical entity, often built using CAD models, simulation software, and advanced modeling techniques that capture the entity’s behavior and characteristics.
Real-time or near real-time data from the physical entity is fed into the digital model through sensors, IoT devices, and data streams. This data includes everything from operational parameters and environmental conditions to user interactions and external factors. The digital twin uses advanced analytics, machine learning, and AI to process the data, simulate different scenarios, predict future outcomes, and provide insights for decision-making. Digital twin technology is an advanced digital technology with broad application prospects, which brings unprecedented development opportunities to various industries by achieving seamless connection and real-time interaction between the physical world and the virtual world.
The physical infrastructure for the metaverse refers to the underlying hardware and systems that support the creation, maintenance, and operation of virtual worlds. The physical world supplies the metaverse with essential infrastructure, such as sensing, control, communication, and storage systems. The sensing and control infrastructure consists of smart objects such as sensors and actuators, which utilize various heterogeneous wireless or wired networks, including satellite communications, cellular communications, and unmanned aerial vehicle (UAV) communications [58]. While the metaverse technology encompasses powerful computing and storage infrastructure, it also leverages cloud-edge-end computing. Kai et al. [59] investigate a collaborative computing framework for mobile edge computing (MEC) that enables partial processing of tasks across mobile devices, edge nodes, and cloud centers to enhance processing efficiency. They propose a pipeline-based offloading scheme and formulate a sum latency minimization problem. By utilizing successive convex approximation, they transform the non-convex optimization into a convex one. Simulations demonstrate that this approach significantly outperforms existing offloading schemes. So we can see physical infrastructure consists of the following key components.
3.3.1 Hardware Equipment and Network Facilities
Virtual reality devices: Including head mounted displays (VR headsets), controllers, motion capture devices, etc., which allow users to immerse themselves in the metaverse world and interact with the virtual environment. VR headset is one of the most essential devices, which can provide 360 degree panoramic vision, allowing users to feel an immersive experience.
Augmented reality devices (AR): Although slightly different from VR, AR devices are also important tools for accessing the metaverse. They can overlay virtual information in the real world, providing users with a mixed reality experience.
High performance computing devices: The metaverse requires processing large amounts of data and complex computing tasks, so high-performance servers, workstations, and data centers are essential. These devices can ensure the stable operation and efficient data processing of the metaverse.
High speed communication networks: Including high-speed wireless communication networks such as 5G and 6G, as well as wired networks such as fiber optic broadband. These network facilities can provide low latency, high bandwidth data transmission capabilities, ensuring a smooth user experience in the metaverse.
Satellite Internet: In order to realize the global coverage and global access of the meta universe, satellite Internet is also one of the important infrastructures. It can provide stable network connections for users in remote areas, further promoting the popularization and development of the metaverse.
3.3.2 Data Acquisition and Storage
Data acquisition can be achieved through sensors and IoT technology.
Sensor technology: Sensors are the bridge between the real world and the metaverse, capable of sensing and collecting various information about the real world, such as environmental parameters, personnel location, object status, etc. These pieces of information can be used to construct more realistic and vivid virtual environments.
Internet of Things technology (IoT): Internet of Things technology can connect various devices to achieve data interoperability and sharing. In the metaverse, IoT technology can facilitate interactions between the virtual and real worlds, extending to areas like smart homes, smart cities, and beyond. Data storage can be accomplished using data centers and cloud computing.
Large data centers: The metaverse requires the storage and processing of massive amounts of data, including user information, virtual environment data, interaction data, and more. Large data centers can provide sufficient storage space and powerful computing power to support the demands of the metaverse.
Cloud computing platform: Through the cloud computing platform, the metaverse can achieve dynamic allocation and elastic expansion of resources. Users do not need to purchase and maintain expensive hardware devices, they only need to access the cloud computing platform through the network to enjoy the services of the metaverse.
A “metaverse engine” originally refers to the technology and software frameworks used to create, run, and manage virtual worlds, or the metaverse. These engines and technologies are the foundation for building the immersive, interactive, and persistent virtual worlds that make up the metaverse. However, the primary fuel for the metaverse is real-world data [1]. The metaverse takes input from real-world data and generates and maintains its virtual environment using human-computer interaction tools such as smart glasses and AI-enabled devices. AI-enabled devices, digital twins, and blockchain serve as the main sources and mediums for data input. For example, augmented reality and virtual reality enable users to experience the metaverse through human senses such as touch, hearing, and sight. Virtual Reality Modeling Language (VRML) is another technology that enables smooth interaction with the metaverse. While VRML was originally developed in the 1990s for creating 3D virtual experiences in web applications, it finds application in the metaverse as well. Brain-computer interfaces (BCI) are another invention that enhances users’ ability to perceive and interact with the virtual world more realistically. The virtual economy, coupled with digital avatars, provides an elevated level of experience. The virtual economy requires security technologies like blockchain. As a result, the metaverse introduces a new economic system wherein digital twin avatars can create and monetize digital assets replicated using digital twin technology. Thus, we can say that real-world data serves as the engine powering the metaverse. In Fig. 4, we have depicted how the metaverse engine interacts with real-world data and other components of metaverse technology to breathe life into it.
Figure 4: Metaverse engine works at the core of metaverse technology
Human-computer interaction technology serves as the gateway to the metaverse, providing device interfaces for entering the virtual world and offering users an immersive experience. Interactive immersion technology allows users to experience full sensory immersion in the metaverse, enabling them to control virtual avatars, create content, socialize, and participate in digital transactions. Virtual reality, utilizing computer simulation to create virtual environments, plays a crucial role in generating a sense of immersion in the virtual world.
XR, which stands for extended reality, encompasses immersive technologies used to access the metaverse. It represents a range of technologies that provide a sense of virtual reality. XR allows users to transition from the physical world to a completely virtual one. Based on the level of virtuality, XR can be categorized into three distinct bands [60].
• Augmented Reality (AR): AR provides a semi-real and semi-virtual experience, serving as an interface between the physical and virtual worlds. Users can distinguish between the virtual and physical elements. AR effects can be experienced through devices like smartphones, tablets, Realer devices, and more.
• Mixed Reality (MR): As defined by [60], mixed reality offers a more seamless integration of the virtual world with the real world compared to AR. For example, virtual objects can be occluded by physical objects. Devices such as Hololens, Magic Leap 1, and Lynx enable users to experience MR effects.
• Virtual Reality (VR): VR provides the highest level of immersion, where the virtual world closely resembles the physical world. Users can perceive audio and visual stimuli in both the virtual and physical realms. Devices like Meta Quest 2 and HTC Vive are designed for experiencing VR.
3.5.2 Interactive Basic Technology
The basic interactive technologies mainly include virtual reality (VR), augmented reality (AR), hybrid reality (MR), VR handle technology, spatial positioning technology, brain-computer interaction technology, line of sight tracking technology, etc. The application of VR technology, for example, involves companies creating virtual factories, where visitors wear VR glasses and perceive the environment as if they are physically present in the factory. Among these technologies, VR handle technology refers to a special handheld device that can sense user hand movements and translate them into corresponding movements in virtual scenes. Users can control characters, objects, etc., in the virtual environment using the VR handle. Spatial positioning technology enables user position tracking and motion capture in virtual environments through devices such as sensors and cameras, including rotation tracking based on gyroscopes and position tracking based on laser rangefinders. Brain-computer interaction technology monitors users’ brain waves through sensors, allowing for mental control and sensing in virtual environments. Eye tracking technology monitors the user’s line of sight using devices such as eye trackers, enabling gaze-based interaction in virtual environments.
He et al. [24] demonstrate a novel cutoff system for wearable devices currently used for VR scene interaction, which mostly rely on complex structures and external power sources, such as flexible gloves. The system integrates multimodal sensors and tactile feedback units for virtual reality applications. However, the challenge of complex structure has not been adequately addressed, highlighting the need for the development of new single-functional materials capable of detecting multiple stimuli simultaneously.
3.5.3 Interactive Technology Empowers the Metaverse
Virtual reality technology, also known as VR technology, utilizes computer technology and devices to simulate a virtual world, allowing users to immerse themselves and experience this virtual environment. Through headsets, controllers, and sensors, virtual reality technology can replicate users’ visual, auditory, tactile, and other sensory experiences, providing them with a truly immersive and engaging experience.
The applications of virtual reality technology in the metaverse are vast. Apart from offering immersive entertainment experiences, it has found its place in the field of education as well. Particularly during the outbreak of the epidemic, the education sector actively embraced online education and teaching activities. Utilizing virtual reality technology, three future online education scenarios were created, incorporating VR course resources, VR course design, and the integration of “5G+VR” technology. These initiatives aimed to establish an immersive online education model for students, providing them with interactive and engaging learning experiences [61]. In the architecture field, virtual reality technology, combined with digital modeling techniques and XR technology, has been leveraged for safety education inspections. Spitzer et al. [62] propose a decision-making framework to assist educators in Architecture, Engineering, and Construction (AEC) programs in selecting appropriate extended reality (XR) technologies-such as virtual reality, augmented reality, and mixed reality-for specific educational outcomes. Grounded in a comprehensive literature review, the framework addresses factors like budget, scalability, and space requirements, and was successfully validated at a summer camp, emphasizing the importance of context in choosing XR modalities to enhance student engagement and learning. Kwok et al. [63] demonstrate the use of VR headphones in conjunction with the Fuzzy Multi-Criteria Decision Making (MCDM) method. This approach was designed to identify crucial factors and parameters for product casing design by understanding customer preferences and engineers’ technical requirements. The visual representation of the universe within a virtual environment provided by the VR headphones facilitated a comprehensive evaluation process. By incorporating technical and aesthetic considerations, the proposed fuzzy MCDM method enhanced the robustness of product design, leading to better outcomes. Patel et al. [64] conduct research on interactive technology and collected body-related information through feedback devices worn on the wrist and ankle. This data, combined with AI calculations and 3D scanning technology, was utilized to gather the necessary information for virtual projection. Wang et al. [65] provide a comprehensive overview of immersive (XR) interaction technology, holographic communication technology, and sensory interconnection technology. Additionally, the article discussed the application of brain-computer interface technology in the medical field, focusing on detection and evaluation. Notable advancements have also been made in entertainment and focus training domains, with ongoing exploration required for further development. Yeong et al. [66] explore the utilization of AR and VR technology in video fusion to enhance the 360-degree autonomous direction selection in photography and videography. By enabling the selection of viewing angles during shooting, the author addressed the limitations of traditional TV shooting and display, allowing for a full field of view and multiple angles. This application of AR and VR technologies augmented the traditional advantages of television media, mitigated certain drawbacks, and introduced a novel viewing and interaction mode known as the “Metaverse Neighbor Viewing Mode.” This mode is expected to bring significant changes to various forms of video new media, including news dissemination, concerts, and live broadcasts. Yu et al. [67] develope a lightweight and cost-effective design for a self-sensing actuator called SenAct, which was showcased in the form of a tactile feedback glove. This glove enhances immersion and overall sensation, enabling users to experience a more realistic virtual reality environment. To ensure consistent and accurate force feedback, a closed-loop control strategy utilizing sensor features was proposed. This strategy leverages the relationship between capacitance (Cloud) and load (Flow), allowing for precise force feedback during interactions with virtual objects.
The relationship between output force and sensor capacitance with different loads is linear, and the fitting curve of
The output force of SenAct can be expressed as:
When SenAct comes into contact with the wearer, there is a preload on the SenAct, which can be calculated by:
Since
Based on the relationship and control strategy between preload, capacitance, and output force, the closed-loop control ensures the uniformity of the sensor’s output force under different loading conditions. According to Peter A. Kara et al. [68], VR, AR, and XR are not the only 3D visualization technologies benefiting from ongoing research. Light field technology, another type of 3D imaging, can be utilized in telepresence, social activities, education, cultural heritage, gambling, online dating, and more. The light field metaverse remains an important concern in terms of privacy compared to the traditional metaverse.
In summary, it can be observed that interaction technology does not exist in isolation and usually requires comprehensive application to achieve a more natural and efficient user interaction experience. At the same time, interaction technology also needs to cooperate with other elements such as hardware devices and software platforms to build a complete metaverse system.
Artificial intelligence technology is a key factor in achieving end-to-end intelligence in the metaverse, providing technical support for metaverse application scenarios and improving the operational efficiency and intelligence level of the virtual world. When it comes to intelligent agent technology, artificial intelligence is trained and optimized using techniques such as machine learning and deep learning, enabling it to learn independently and adapt to different scenarios. When applied to metaverse intelligent dialogue systems, it can act as a way for users to engage with virtual environments.
3.6.1 Basic Technology of Artificial Intelligence
An intelligent agent refers to a virtual role that performs tasks on behalf of users in the metaverse, and it has the ability to make decisions and act independently. In the metaverse, intelligent agents can play various roles, such as virtual guides, virtual salesmen, virtual customer service, and more. Users can interact with intelligent agents through voice commands, gestures, head movements, and other means to complete various tasks and operations. Intelligent agents can make independent decisions and take actions based on user input and the current environmental status, thereby providing more convenient and personalized services and experiences.
The core of intelligent agent technology lies in machine learning and deep learning [69]. Generally, intelligent agents require extensive training and optimization to achieve desirable results. Additionally, intelligent agents need to possess good human-computer interaction abilities to effectively communicate and interact with users. Overall, intelligent agent technology is a major component of the metaverse, providing users with more advanced and convenient virtual services and experiences. Machine learning is an artificial intelligence technology that enables machines to learn and improve algorithms automatically by analyzing and processing large amounts of data, thereby accomplishing various tasks.
Machine learning can be categorized into supervised learning [70], unsupervised learning [71], semi-supervised learning [72,73], and reinforcement learning [74]. In the metaverse, machine learning technology finds applications in numerous fields, including intelligent agents, virtual roles, speech recognition, image recognition, natural language processing [75], and more. For example, intelligent agents can utilize machine learning technology for training and optimization, enabling them to provide more intelligent and personalized services and experiences. Virtual characters can leverage machine learning technology to exhibit more realistic behavior and interaction. Speech recognition and image recognition can utilize machine learning technology to achieve more accurate recognition and understanding. In the metaverse, machine learning necessitates a large volume of data for effective training and optimization. Additionally, selecting appropriate algorithms and models for analysis and processing is crucial. Furthermore, machine learning also needs to address concerns related to data security and privacy protection.
Natural language processing (NLP) [76] is a technology that uses computer technology to process and analyze human natural language. NLP is an active research area [77–80] with a wide area of applications including metaverse. In the metaverse, natural language processing technology can be applied to speech recognition, text analysis, natural language generation, intelligent dialogue, and other fields to provide users with more natural, intelligent, and personalized interactions and services. For example, in the metaverse, users can engage in speech interaction through speech recognition technology, converting natural language into computer-recognized language. Text analysis technology can be used to analyze users’ comments and behaviors on social media, games, and other platforms, thereby providing more intelligent services and recommendations. Natural language generation technology such as Large Language Models can be used to generate answers and comments in intelligent conversations. Intelligent dialogue technology can achieve natural, smooth, and personalized conversations, providing a higher-quality user experience. With the minute recording of data from all aspect of life gave birth to big data which paved the path to success of deep neural networks, such as large language models. At the same time, the improvement of hardware equipment also provides natural language processing technology with stronger computing power and efficiency.
3.6.2 Artificial Intelligence Empowers the Metaverse
Huynh-The et al. [81] describe how the metaverse can establish a secure, scalable, and realistic virtual world on a dependable and continuously online platform by integrating artificial intelligence technology with AR/VR, blockchain, and network technology. By examining traditional ML algorithms and DL architectures, we can advance various metaverse support technologies, such as natural language processing, machine vision, blockchain, networking, digital twins, and neural interfaces. The modernization of built-in services and applications has demonstrated significant potential for the establishment and development of metaverse virtual worlds. Mu et al. [82] describe the techniques of object segmentation, object tracking, and attitude estimation in artificial intelligence, providing people with a hyper-spatial perception of past, present, and future metaverse scenes by capturing the ever-changing real world. In artificial intelligence NPC games, these NPC characters can simulate human behavior and thinking, making the game scenes more realistic and vivid.
3.6.3 Artificial Intelligence Secures the Metaverse
Intelligent monitoring and warning: Artificial intelligence can monitor user behavior, transaction activity, and system operation status in the metaverse in real time, identify abnormal behavior patterns such as fraud, malicious attacks, etc., through big data analysis, and issue timely warnings. By utilizing natural language processing (NLP) and image recognition technology, AI can analyze user chat content, images, and videos to detect potential threat information such as hate speech, pornography, or fraudulent advertising.
Automated defense and response: In response to detected security threats, artificial intelligence can automatically trigger defense mechanisms, such as blocking malicious accounts, isolating infected areas, or initiating emergency response processes. AI can also dynamically adjust defense strategies based on the severity and type of threats, improving the system’s adaptability and resilience.
Identity authentication and permission management: Through technologies such as biometric recognition and behavioral analysis, artificial intelligence can offer users a more secure and convenient method of identity verification, ensuring that only authorized individuals can access sensitive resources in the metaverse. At the same time, AI can dynamically adjust users’ access permissions based on their roles and permissions, preventing unauthorized access and data leakage.
Let’s consider a metaverse-based airport scenario where there are no human employees present on-site. Instead, employees work remotely from different locations, monitoring and authenticating passengers and their belongings. In such a scenario, if a large amount of data is sent from the airport to a remote server, there is a risk of important customer information leaking. To address this, multiple servers are installed near the airport, and data analysis is performed locally. Additionally, data may need to be encrypted before being sent to the remote cloud server. This paradigm is known as edge computing. Edge computing enables operators to perform processing, storage, and other functions on demand at the network edge, where data is generated.
As for the need for the metaverse, a network based on demand cloud computing technology such as edge computing is required [83–86]. Ashani et al. [87] provide a comprehensive overview of metaverse-as-a-service (MaaS) platforms, focusing on privacy, security, edge computing, and blockchain technology. The study discussed challenges, proposed solutions, and outlined future directions for secure, private, and efficient MaaS implementation across various layers of the metaverse. Wang et al. [88] survey how mobile edge computing (MEC) addresses computational, storage, and energy limitations in the metaverse by offloading tasks to network edges. The study examined architectures, technologies, and application scenarios, including BoundlessXR and CloudXR, and proposed future directions for metaverse system development.
Karunarathna et al. [89] present a practical approach for realizing the metaverse using fifth-generation (5G) and beyond 5G (B5G) technologies, emphasizing the roles of network slicing and multi-access edge computing in enabling immersive applications. The survey also addressed deployment challenges and outlined the technical requirements for successful implementation. Jamshidi et al. [90] presented a novel GSM triplexer designed for 5G-enabled IoT applications in sustainable smart grid edge computing and the metaverse. The triplexer demonstrated outstanding performance with minimal insertion losses and a compact layout optimized for 5G smart grid services. Dhelim et al. [8] propose a Fog-Edge hybrid computing architecture for metaverse applications, which offloads computationally intensive tasks such as collision detection and 3D physics simulations to edge devices. This approach reduces latency by 50% compared to traditional cloud-based architectures. Yu et al. [91] explored the bidirectional relationship between digital twins and multi-access edge computing in the metaverse. The study focused on the creation of digital twins on MEC servers and the development of a continual learning framework for resource allocation strategies that enhance synchronization and improve user experience.
3.7.1 Edge Computing Basic Technology
The edge computing system mainly consists of three components: the perception layer, internet gateway, and edge servers. The perception layer comprises IoT sensors, actuators, visio motor sensor devices, VR headsets, and other devices that directly capture user input and send it to the edge servers for processing. Edge servers are located near the source of the original data, and they receive input from the perception layer or other sources to perform processing, aggregation, and local computing tasks. Internet gateways are used to connect these edge computing processors to the Internet. In the metaverse, various activities such as identity modeling, real-world and virtual-world interactions, and interactions between multiple universes generate massive amounts of data. Processing this data efficiently and reducing latency is essential, and edge computing technology plays a crucial role in addressing these challenges [12]. Edge computing enables faster data processing and transmission, making it well-suited for handling the large data volumes in the metaverse. It is considered an effective alternative to cloud computing when it comes to processing large amounts of data. By leveraging edge computing technology [92], the computational power in the metaverse can be brought closer to the user’s equipment base station, thereby reducing latency. However, it’s important to note that if a user’s device moves, it can cause interruptions. To mitigate this, computing power can be pre-deployed to the next base station, enabling seamless migration among users while maintaining continuous computational support.
Edge computing plays a crucial role in the metaverse by addressing the following tasks and challenges:
• Edge computing helps address the data latency problem in the metaverse by providing timely data without the need to travel through long internet distances. This is particularly crucial for tasks such as physical emulation, graphics rendering, and real-time data streaming, which are significant concerns causing data availability and latency issues in the metaverse.
• Edge computing contributes to performance optimization in the metaverse by resolving computational bottlenecks, managing network traffic, and enabling higher-level tasks. It ensures efficient utilization of computing resources and improves overall system performance.
• With its proximity to data sources, edge computing enhances data authentication, ensures ubiquitous access to data, and addresses scalability challenges. It reduces the reliance on transmitting every generated data over the internet and facilitates secure and efficient data handling.
• Edge computing helps alleviate network congestion issues by processing data at the edge instead of sending it all to the cloud. This approach reduces unnecessary data storage and ensures that data is available where it is needed.
• By providing fast processing capabilities in close proximity to various head-mounted displays (HMDs), edge computing relieves the computational burden on these devices, enabling them to receive processed information quickly.
• Edge computing plays a crucial role in addressing user data privacy concerns in the metaverse. By processing data locally and sending only relevant information to the cloud, it reduces the risks associated with sending raw data over the internet.
• As a service provider, edge computing offers greater control and authentication capabilities over data compared to remote cloud servers. It enables monitoring of data privacy and facilitates the differentiation between private and public data.
• Edge computing provides increased data reliability compared to remote cloud computers. By processing and storing data at the edge, it reduces the dependency on distant servers and improves data availability and integrity.
• Additionally, edge computing helps reduce the cost of data transmission over the internet. It also mitigates the computational burden on HMDs, which would require more resources and incur higher costs if all processing were performed on the devices themselves.
Blockchain technology is a distributed ledger system that ensures immutability, decentralization, and secure transmission of user data in the metaverse. Blockchain includes hash algorithms, timestamp technology, consensus mechanisms, and distributed technology. Hashing algorithms and timestamp techniques offer users data traceability and confidentiality. Consensus mechanisms solve credit problems, and distributed networks constitute the core foundation and support for the operation of the entire economic system [6].
In the metaverse, blockchain technology can be utilized in areas like virtual currency, digital asset trading, and data privacy protection. It provides users with more secure, transparent, and trustworthy transactions and services. Users can use virtual currency for payments and transactions in the metaverse, with blockchain technology ensuring the security and credibility of these exchanges. Digital asset trading can leverage blockchain technology to achieve decentralized trading, avoiding the risks and problems associated with traditional centralized exchanges. Additionally, data privacy protection can utilize blockchain technology for decentralized identity authentication and data storage, thereby protecting user privacy and data security.
3.8.1 Blockchain Basic Technology
Blockchain technology is based on cryptography and distributed systems, with its core comprising blockchain data structures and consensus mechanisms. In blockchain networks, data is recorded in the form of blocks on the blockchain, and collaboration and verification among nodes are achieved through consensus mechanisms. The fundamental technology of blockchain encompasses various aspects. For instance, the basic data units in blockchain consist of multiple transaction records and block header information. The problem of cooperation and synchronization between nodes is resolved through distributed systems, and data distribution and storage are realized using peer-to-peer networks, distributed hash tables, and other technologies. Cryptographic techniques ensure the confidentiality and integrity of data during transactions. The decentralization and security aspects of blockchain are achieved through consensus mechanisms [93,94].
Smart contract technology enables automated transaction operations, which can be written and executed using programming languages and virtual machines on the blockchain platform. Smart contracts can be considered as automated contracts that can execute and validate transactions without intermediaries, providing high security and reliability. They can facilitate automatic operations in various scenarios, including automatic transfer, tamper-proof records, and digital identity verification. Therefore, smart contracts play a crucial role in the application scenarios of the metaverse.
In the metaverse, decentralized storage can be applied to store and share various types of data, including virtual assets, smart contracts, and user data. Simultaneously, decentralized storage also provides users with better privacy protection, allowing them to have control over their data and decide whether to share it with others. It ensures the openness and transparency of transactions, and the development of decentralized storage technology will provide better technical support for metaverse construction.
Overall, blockchain technology forms the foundation for implementing blockchain networks and needs to be designed and optimized in conjunction with specific application scenarios to achieve more efficient, secure, and reliable blockchain applications. Additionally, blockchain technology can be utilized to ensure the security and credibility of data in the metaverse, thereby ensuring fairness and transparency within the virtual economy.
3.8.2 Blockchain Technology Empowers the Metaverse
The fair exchange protocol proposed by researchers based on blockchain is often included in TTP-free protocols due to the encryption pairing operation, but it incurs high costs for the blockchain. Chen et al. [95] have introduced a new signature exchange protocol called DFSE. This protocol is decentralized, verifiable, efficient, and autonomous. Through on-site network testing, the feasibility of the protocol has been confirmed, and it also demonstrates low costs.
Wei [96] discusses the limitations of the traditional tourism industry and addresses the security of tourism data through the utilization of cryptocurrency payment technology in blockchain. By establishing trust orders through consensus mechanisms, it promotes the high-quality development of the tourism market. This method has revolutionized the tourism industry’s value chain by lowering transaction costs, enhancing transparency in the business environment, and thereby improving service efficiency. Xu et al. [97] propose a novel trustless architecture for blockchain-enabled metaverses, focusing on efficient resource integration and allocation through On-Demand Trusted Computing Environments (OTCE). By leveraging local trust evaluation and hypergraph-based representation, the architecture aims to enhance security and flexibility while mitigating the inherent risks of metaverse technologies. Yang et al. [98] explore the integration of Blockchain and Artificial Intelligence (AI) within the metaverse, examining cutting-edge studies on its components, digital currencies, and blockchain-empowered technologies. The study emphasizes the need for interdisciplinary collaboration between academia and industry to advance the fusion of AI and Blockchain, with the goal of creating an open, fair, and transformative metaverse. Mishra et al. [99] provide a comprehensive assessment of blockchain systems in the metaverse, highlighting their potential to address privacy and security concerns due to decentralization, immutability, and transparency. The paper explores the integration of blockchain with key metaverse technologies like artificial intelligence, big data, and the Internet of Things (IoT), and highlights the challenges and opportunities for future development in this evolving digital landscape.
3.8.3 Blockchain Technology Secures the Metaverse
Data immutability and transparency: Blockchain technology ensures that key information such as transaction data and user behavior records in the metaverse cannot be tampered with once they are on the chain through distributed ledgers and encryption algorithms, thereby improving the authenticity and credibility of data. This transparency also helps with public supervision and reduces the occurrence of fraud and corruption.
Smart Contracts and Automated Execution: Smart contracts on the blockchain can automatically execute preset rules and conditions without the need for third-party intervention, thereby reducing the risk and cost of human operation. In the metaverse, smart contracts can be used to manage virtual assets, execute transaction protocols, and ensure the authenticity and fairness of transactions.
Decentralization and Attack Prevention: The decentralized nature of blockchain enables the metaverse to no longer rely on a single centralized institution to maintain security, thereby reducing the risk of single point attacks. Meanwhile, nodes in the blockchain network can mutually verify and backup data, improving the stability and reliability of the system.
3.9 Network and Computing Technology
Cloud service technology enhances the metaverse experience by offering low-latency services, more efficient collaboration, and products built on cloud infrastructure that are readily accessible to users around the globe. The Internet of Things (IoT) aims to establish information carriers and telecommunications networks based on the Internet, enabling the interconnection of all independently addressable physical objects. In the metaverse, Chen et al. [100] highlight the need to process large amounts of data and information, which requires robust network and computational technologies. These technologies include 5G/6G networks, cloud computing, big data, and more. The 5G/6G network can be likened to airplanes and high-speed trains in real life, providing large-scale, high-speed, and low-latency access transmission channels in the metaverse, ensuring a real-time and smooth user experience [101]. Cloud computing, big data, and artificial intelligence technologies help achieve efficient data management and computing capabilities, enhancing the intelligence and automation of the metaverse. By combining 5G, cloud computing, and edge computing technologies, the metaverse can support more online users while ensuring low latency and seamless experiences. This integration also reduces user terminal requirements, facilitates the expansion of the user base, and accelerates the development of the metaverse ecosystem.
The metaverse demands high synchronization and low latency to deliver a real-time, smooth, and seamless experience. Testing data from Open Signal, an independent network testing agency, shows that 4G LTE can achieve end-to-end latency of 98 milliseconds, which is sufficient for interactive applications such as video conferencing and online classrooms. However, it falls short of meeting the metaverse’s strict requirements for low latency. One major issue with VR equipment is the latency caused by transmission delays. Its index is the delay from rotating the head to rotating the picture. The improvement of 5G bandwidth and transmission rate can effectively reduce the delay and alleviate latency.
According to data from Nokia Bell, 5G end-to-end latency can be kept under 10 milliseconds. The metaverse demands rapid transmission of large data volumes and depends on robust communication infrastructure. However, the actual transmission rate of 5G may fall short of its design potential due to the limited number of base stations. Japan and South Korea’s projections for 6G network technology suggest that its latency will be reduced to one-tenth of 5G, with transmission speeds anticipated to be 50 times greater. This advancement is anticipated to truly achieve the goal of low latency issue solution. The characteristics of immersive, intelligent, and fully integrated 6G in the future will deeply integrate various new technologies, fully unleash the potential of the metaverse, and drive its progress and transformation [102].
The Internet of Things (IoT) technology plays a crucial role in bridging the gap between the real world and the virtual world, offering a wealth of data and intelligent capabilities to support the metaverse. IoT devices and sensors enable the capture and transmission of physical data from the real world into the metaverse, allowing for a seamless connection between the two realms. By operating simultaneously in both the virtual environment of the metaverse and the physical world, IoT devices can control the behavior of physical devices, enabling a seamless integration of the real and virtual worlds. Moreover, IoT technology provides real-time monitoring and feedback for the metaverse, empowering users to better manage and control virtual environments.
In the future development of the metaverse, IoT technology will be indispensable, as it offers strong support for its implementation and application. Serving as a key technology for bridging the gap between the virtual and real worlds, the Internet of Things is crucial for transcending the boundaries between these two realms. It provides reliable technological guarantees for the interaction and coexistence of virtual and real entities in the metaverse, enhances the perception of external resources within the metaverse, and contributes to the inevitable growth and development of this immersive virtual world.
The Internet of Things technology provides reliable technical support for connecting all things in the metaverse and the coexistence of the virtual and real worlds, including the perception layer, network layer, and application layer. The perception layer and network layer can provide a technical foundation for the metaverse to perceive signals from the physical world. The application layer represents the ultimate objective of the Internet of Things, managing the big data obtained by device terminals to provide intelligent services for different business fields. It can link all things in the metaverse and manage them in an orderly manner, achieving virtual and real coexistence. Based on the analysis of key technologies in the metaverse, the advantages and disadvantages of the key technologies in the metaverse are described in Table 3.
4 Application Scenarios of the Metaverse
With the maturity and improvement of various information technologies, the metaverse is increasingly being promoted and applied in numerous segmented fields, with wider coverage. The metaverse is also being increasingly applied in different scenarios, including gaming, social media, education, healthcare [113,114], entertainment, and more. For example, transactions in games can be achieved through virtual currency on the blockchain, and immersive experiences can be realized through interactive technology. Communication between people can be facilitated through virtual reality platforms in social interactions, among other applications. Dwivedi et al. [115] describe the use of the metaverse as a tool to supplement the real world in various ways, enabling the completion of tasks that are difficult to accomplish in reality. It has replaced offices, social networks, face-to-face courses, and healthcare, making it possible to overcome the challenges of cost limitations. When the metaverse is targeted, it serves as a tool to reflect the real world. It is precise because of the existence of the metaverse that social communication and value have been discovered in the gaming and business fields, see Fig. 5 and Table 4. Given this, let’s outline the techniques used in various scenarios of the metaverse.
Figure 5: Metaverse applications scenarios
Digital technology related to games continuously drives technological progress in other fields, becoming a significant force in promoting social development [116]. Originally, the “graphics processor” was designed to enhance the quality of digital games, but its application gradually expanded from game rendering to the field of artificial intelligence training. Games in the metaverse can encompass various types, such as role-playing games, casual games, competitive games, and others. These games offer users a realistic and immersive experience, enabling them to explore, interact, collaborate, and compete [117–119]. Virtual items and currencies within games can be traded and utilized within the metaverse, offering additional trading opportunities and possibilities for game players and virtual item collectors. Another significant application scenario in the metaverse is virtual socializing [105,120–123]. Within the metaverse, users can engage in social interactions with others through virtual identities and characters [58].
Virtual social scenes within the metaverse can be diverse, encompassing chat rooms, virtual reality social platforms, virtual activities, and more. These scenes enable users to make new friends, share information and resources, organize and participate in various activities, and so on. The defining characteristic of virtual social scenes is their ability to transcend geographical and identity constraints present in the real world, allowing users to express themselves more freely and expand their social circles. Furthermore, virtual social scenes offer diverse and personalized social experiences. Users can choose their preferred virtual images and environments, participate in social activities aligned with their interests, and more.
Virtual social media represents a significant business application scenario within the metaverse. Virtual social platforms, for instance, can offer advertising and promotional services to users, as well as support and safeguards for virtual goods and virtual currency transactions. Virtual socializing also facilitates access to various virtual services and entertainment experiences, such as virtual tour guides, virtual fitness programs, virtual performances, and more.
A famous scientist, Thomas Alva Edison, once said that “motion pictures” would be a game-changer for education. Almost a century later, we can see that science and technology have provided even better advancements than just movies. As Edison predicted, this technology has the potential to replace traditional learning mediums such as textbooks and be 100% effective. Hopefully, the metaverse will bring a positive change to the education system as well, providing students with a real and immersive learning experience. For example, when studying marine animals, students can learn about their characteristics by feeling and seeing them in a virtual ocean environment. The same applies to history subjects, where students can better remember and make comparisons by virtually living in ancient civilizations such as Greek, Roman, Indian, Chinese, and others.
However, the question remains as to whether these educational media will be accessible and usable for all students around the world. In the book [60], the authors discuss past failures. For instance, two MIT professors planned to provide
Education is indeed another important application scenario of the metaverse. In the metaverse, educational scenarios can be diverse, encompassing online classrooms, virtual laboratories, virtual scene teaching, and more. Through the utilization of virtual technology, educational scenarios can provide a highly immersive learning experience, thereby introducing a new form of immersive education [124–126]. This allows students to develop a more comprehensive understanding of course material and practical skills. In the educational scene of the metaverse, students can actively participate in various virtual experiments, virtual exams, and virtual interactions through their virtual characters. They can engage in activities such as conducting scientific experiments, creating virtual products, simulating business decisions, and more. These virtual scenes greatly contribute to students’ comprehension and mastery of knowledge and skills while fostering their creativity and teamwork spirit. Hare et al. [127] introduce a technical framework that utilizes a hierarchical multi-agent reinforcement learning approach with experience sharing to enhance the intelligence of non-player characters in metaverse learning. The study demonstrated the framework’s effectiveness in personalizing education through the metaverse learning game Gridlock and through extensive simulations. Zheng et al. [128] explore the education metaverse by addressing key aspects of smart services, including the learning scene defined by learner, time, space, and learning events. They proposed a data-knowledge-driven group intelligence framework aimed at transforming data into knowledge for transaction and management services within this innovative educational context. Ktoridou et al. [129] investigate students’ and educators’ perceptions of the metaverse and its potential applications in education using a mixed-methods approach. The study revealed a general understanding and optimism about the future use of the metaverse, although opinions varied regarding the significance of the changes it may bring. Zhao et al. [130] demonstrate that the metaverse enhances emotional engagement and cognitive levels in learners through immersive VR/AR/MR environments. This leads to the development of a personalized learning space that positively affects learning engagement and outcomes, as evidenced by PPG signals and test data comparisons between metaverse and traditional classroom settings. Rospigliosi et al. [108] note that the shift towards virtual reality, exemplified by Facebook’s rebranding to Meta in 2021, marks a pivotal moment in recognizing the metaverse as a transformative force in interactive learning environments. This shift offers flexible learning opportunities while also raising concerns about potential disconnection from authentic experiences, as articulated by Baudrillard’s concept of simulacra.
Zhou et al. [131] propose a deep integration of real educational resources with virtual teaching in the metaverse through the establishment of an educational metaverse community. This approach aims to promote the bidirectional circular development of online and offline education, allowing for synergistic growth in both realms. Fu et al. [132] review the application of metaverse-based teaching and suggests that hybrid teaching modes integrating the metaverse and multi-scenario learning will be crucial for the future of vocational education, promoting digital transformation and innovation in talent training. Hwang [133] empirically examine the impact of using the metaverse in maker education, finding that integrating NFTs and virtual exhibitions into the curriculum enhances students’ creative cognition and sense of achievement. It proposes an updated educational model, TMIOS (Thinkering, Making, Improving, Owning, Sharing), to reflect these benefits. Asiksoy [134] systematically reviews empirical research on metaverse-based education, highlighting that while virtual reality is the most commonly used metaverse type, there is a lack of research involving mixed-reality technology and younger students (middle and primary school). The findings suggest that more empirical studies are needed, particularly focusing on student behavioral engagement, to better understand the potential of the metaverse in education. Nagao [106] explores the potential and future prospects of virtual reality (VR) in online education, focusing on how VR, combined with metaverse construction and artificial intelligence (AI), can enhance the learning experience. It discusses concepts such as virtual campuses offering on-demand VR lectures, AI-driven student evaluations, and the integration of multiple digital campuses. Mohamed et al. [135] highlight the metaverse’s potential to reduce inequalities and enhance access to educational opportunities. Samala et al. [136] provide a systematic review of research on metaverse technology in education from 2012 to 2022, revealing a significant rise in interest, with a peak in 2022. The review, based on the PRISMA framework, highlights that augmentation and simulation are the main applications in learning, and identifies AR and VR as the most commonly used technologies, with notable contributions in education sector. Kaddoura et al. [137] conduct a systematic review of the metaverse’s role in education, addressing a literature gap by exploring its impact on learning environments, enabled by technologies like XR and IoE, and highlighting key challenges and future research directions. Shu et al. [138] evaluate the effectiveness of a smart education model within the Edu-Metaverse, comparing it to traditional teaching methods for college English at Zhejiang Open University. Findings show that the Edu-Metaverse model, characterized by immersive and multimodal interaction, significantly improved students’ performance in various aspects of English learning. The study recommends enhancing teaching scenario design, focusing on core literacy assessments, and increasing teachers’ understanding of the Edu-Metaverse architecture to further support smart education. Hare et al. [127] explore Hierarchical Deep Reinforcement Learning (HRL) with experience sharing for optimizing the metaverse in educational contexts. It aims to enhance learning outcomes by using advanced HRL techniques to improve decision-making and resource allocation within the metaverse environment. Lee et al. [139] evaluate the potential of metaverse-based education services in transforming university education post-COVID, based on feedback from 120 Korean college students. Findings indicate a high intention to use such services, with preferences for features including personal character display methods, educational application areas, and service fees. Wu et al. [140] explore integrating metaverse technology into mathematics education by examining four technology types and their applications, using the “Pythagorean Theorem” as a case study. It aims to provide theoretical support for enhancing math teaching through the metaverse. Al-Marzouqi et al. [141] evaluate UAE university students’ perceptions of metaverse applications in medical education, using a model incorporating Technology Acceptance Model (TAM) and other constructs, alongside machine learning and structural equation modeling. It highlights user satisfaction as a key factor in adopting metaverse technology and provides insights for stakeholders and methodological advancements in Information Systems research. Lo et al. [142] introduce a novel 3D virtual reality architecture (VRAM) to enhance water resources education through immersive technology. A quasi-experimental study comparing VRAM-based learning with traditional methods showed that VRAM significantly improved students’ flow experience, motivation, interaction, self-efficacy, and presence. The interactive VRAM portfolios also enable big-data analysis to refine environmental conservation education in the future.
By leveraging metaverse technology, educational institutions can offer students higher-quality online education services, thereby enhancing the learning experience. Additionally, institutions can explore revenue streams through the trading of virtual goods and virtual currency transactions within the virtual education environment. Furthermore, virtual education has the capacity to foster diversified innovation and development within the education industry. The immersive and interactive nature of the metaverse opens up new avenues for educational content, instructional methods, and collaborative learning experiences. This encourages the exploration of novel approaches to teaching and learning, ultimately benefiting students and driving progress in the field of education.
The metaverse has indeed found extensive applications in the medical field, as highlighted by various studies [143–146]. For instance, Chengoden et al. [147] explore the integration of sensors, big data, and artificial intelligence in digital intelligent healthcare. Musamih et al. [148] explore how the metaverse can revolutionize healthcare by enabling applications like telemedicine, medical education, and healthcare management. It also discusses the challenges to its adoption and suggests future research directions. The metaverse, a 3D digital platform accessed through AR/VR/MR, has the potential to revolutionize psychiatry by enhancing medical education, brain stimulation, and biofeedback, but it also presents significant ethical and data security challenges [149]. Ali et al. [150] propose integrating artificial intelligence and blockchain into the metaverse to enhance healthcare services by creating a secure, immersive environment for doctor-patient interactions. Qayyum et al. [151] explore the potential applications of an AI-XR surgical metaverse in revolutionizing surgical science, highlighting the benefits of combining artificial intelligence with extended reality technologies. It addresses the challenges to realizing this vision and presents a case study demonstrating security threats, specifically focusing on an immersive surgical attack on incision point localization during preoperative planning.
While the world has focused on managing COVID-19, another pandemic-loneliness-has quietly emerged, affecting over a third of adults globally. This isolation is not only uncomfortable but also has severe consequences, as research indicates that strong social bonds can reduce mortality risk by 50% [152]. For the metaverse to effectively address loneliness, its development must prioritize human experience and emotional connections; otherwise, it risks replicating the shortcomings of existing communication platforms. Meinlschmidt et al. [153] establish a trust chain-driven bidirectional feedback mechanism for social network group decision-making (SN-GDM), enhancing opinion interaction and consensus building in the metaverse. It also presents a simulation analysis of the proposed model and demonstrates its effectiveness through an illustrative example within a virtual community decision-making context. Bashir et al. [144] explore the use of Federated Learning (FL) in metaverse healthcare systems, highlighting its potential to enhance privacy, scalability, data management, and security in healthcare. It also discusses various FL-enabled applications in metaverse healthcare, such as medical diagnosis and patient monitoring, while addressing the challenges and potential solutions for implementing FL in this context. Bansal et al. [145] provide a comprehensive survey of the latest developments in metaverse technology within the healthcare industry, covering seven key domains: telemedicine, clinical care, education, mental health, physical fitness, veterinary services, and pharmaceuticals. It reviews metaverse applications, discusses technical challenges and solutions, and highlights the hurdles that need to be addressed for the successful integration of the metaverse into healthcare systems. The rapid transition to a computer-based society, while offering many conveniences, has led to concerns about increased sedentary lifestyles and their negative health impacts [154]. While many embrace the benefits of technology, healthcare professionals point out the detrimental effects of increased computer usage, such as a more sedentary lifestyle, which has been linked to serious health risks, including coronary heart disease and stroke, as noted in a 2019 study on augmented reality [154]. Wang et al. [146] explore how a metaverse focused on medical technology and AI (MeTAI) can enhance medical practice through virtual scanning, data sharing, and augmented regulatory science, while addressing challenges such as privacy and security, and outlining actions to improve healthcare quality and accessibility. Researchers like Ying et al. [155] introduce an innovative education platform called VREX (Virtual Reality based Education eXpansion), which integrates online and offline learning to enhance curriculum development and teaching experiences. Athar et al. [156] outline its potential future directions, emphasizing its role in addressing various issues such as virtual health, mental health, and access to care across geographical barriers. Additionally, it discusses the main challenges that may arise in the implementation of metaverse technology in healthcare moving forward. VREX leverages virtual reality (VR) to create immersive environments, helping students grasp abstract concepts more intuitively. Key contributions include: (1) the creation of an open, immersive O2O classroom using VR and internet technologies, potentially transforming traditional classrooms, (2) a distributed mode allowing students to engage in interactive learning anytime and anywhere, and (3) application across various educational levels and disciplines, demonstrated through practical cases like the “Marine Life” module. Wu et al. [157] conducted an extensive literature review and discovered that the integration of augmented reality (AR) and virtual reality (VR) has significant applications in education and clinical training, particularly noting that VR studies outnumber AR studies in emergency medicine. The metaverse roadmap highlights that lifelogging and mirror worlds are still developing areas within this digital landscape.
Firstly, the metaverse enables the provision of advanced medical equipment and surgical simulation systems, allowing doctors and medical students to engage in surgical simulations and training within a virtual environment. This enhances their surgical skills and medical knowledge, reducing the occurrence of medical accidents and improving overall medical efficiency and quality.
Secondly, the metaverse technology facilitates the delivery of intelligent and personalized medical services to patients. Through virtual doctors and virtual reality technology, patients can access online consultations, remote diagnosis, and rehabilitation treatments in virtual scenes. This not only improves the accessibility of high-quality medical services for patients but also reduces medical expenses and time costs.
Moreover, virtual medical services can also bring significant benefits and business opportunities to medical institutions and insurance companies. By offering virtual healthcare services, such as telemedicine and remote consultations, medical institutions can expand their reach and provide convenient access to healthcare for patients. This not only enhances patient satisfaction but also opens up new revenue streams for medical institutions. Insurance companies can also explore virtual health insurance models that cater to the changing dynamics of healthcare delivery.
The metaverse has indeed introduced exciting possibilities for the entertainment industry. Virtual reality movies and concerts are examples of how users can immerse themselves in these experiences from the comfort of their homes. This technology allows for a more realistic and engaging entertainment experience, blurring the boundaries between the virtual and physical worlds. For example Swami et al. [158] aim to explore how the metaverse, through technologies like AR, VR, AI, and blockchain, is enhancing user experiences in gaming and entertainment, while also addressing future opportunities and challenges related to privacy, security, and inclusivity. Kommareddy et al. [159] investigate the impact of the metaverse on the entertainment and business industries, focusing on how its core concepts, including virtual and augmented reality, and related techniques, influence economic outcomes and industry practices. It aims to provide insights into the metaverse’s real-world evaluation and its potential effects on these sectors. Hu [160] explores SM Entertainment’s successful metaverse marketing strategy and evaluates its potential as a model for other entertainment companies, using the 4Is of marketing mix theory and SWOT analysis. It finds that SM’s unique approach, emphasizing interest and interaction, has proven effective, but suggests that other companies would need to develop their own distinctive strategies to compete successfully. Putra et al. [161] examine how innovations in media technology, such as CGI, VR, and AR, have transformed the entertainment industry by overcoming previous limitations in content production, distribution, and consumption, enabling the creation of more immersive and engaging experiences. Furthermore, the metaverse itself can be a form of entertainment, offering various activities such as virtual racing where players can compete against each other. The metaverse’s versatility and potential for innovation make it an exciting prospect for further advancements in the entertainment industry.
The metaverse holds great potential for application in various fields beyond entertainment, games, socializing, education, and healthcare. It can bring about significant advancements and innovative solutions in several areas.
Smart cities can use the metaverse to create virtual models of urban environments, improving planning, management, and resource optimization. This can lead to more efficient and sustainable cities. Similarly, commerce can benefit from the metaverse by providing virtual shopping experiences, enabling users to browse and purchase products without physical limitations. Virtual advertisements can also be incorporated, enhancing the reach and impact of marketing campaigns. For example Nike-Roblox case study highlights that virtual platforms, content services, and consumer behavior are crucial components of the Nikeland experience for visitors in the Nike-Roblox partnership. As the metaverse grows in popularity among major global brands, its significant expansion is anticipated when regional and local brands begin to engage with this virtual space [162].
Transportation is another field that can benefit from the metaverse. Virtual simulations and models can aid in designing and optimizing transportation systems, improving efficiency and safety. For example, Zhou et al. [163] introduce the concept of “Vetaverse” (Vehicular-Metaverse), envisioned as a blended immersive realm that connects vehicular industries with the metaverse, encompassing two subcategories: the TS-Metaverse for large transportation systems focused on interconnection and management, and the IV-Metaverse for personalized infotainment services within individual vehicles, while also discussing enabling technologies, unresolved challenges, and future research directions. Virtual tourism offers the opportunity to explore various destinations and experience different cultures through virtual reality technology, opening up new possibilities for immersive travel experiences.
The metaverse can revolutionize the way businesses operate by providing virtual offices and workspaces, enabling remote collaboration and offering realistic work experiences. Additionally, virtual real estate allows users to own and customize virtual properties, fostering creativity and social interactions within the metaverse.
Overall, the metaverse offers a vast array of applications across different fields, ranging from smart cities to commerce, transportation, culture, and more. Its virtual nature fosters innovation and immersive experiences, influencing the future of various industries.
These are the challenges that researchers need to address in order to further advance the metaverse [164].
As hardware and equipment, such as Head-Mounted Displays (HMDs), play a key role in experiencing the metaverse, the problems related to these devices must be tackled first. For example, the cost of these devices is still high, which makes them unfeasible for many people, especially if they are intended to be implemented in fields that impact the majority, such as the education sector. As these devices require significant computing power to render, create, transmit, and receive audio-visual signals, they also require improved battery life. Battery life is an important issue because wearable devices cannot be plugged in like laptops or computers [164]. The compactness and ease of wearing the device can be improved in the future as wearing these devices for long periods may not suit all users. These devices should have advanced features such as eye tracking, emotion understanding, and touch sensing. They need further improvement to provide a more immersive experience to the user. Additionally, there is a need to develop these devices for unhealthy or physically disabled people, enabling them to experience a better quality of life. This is feasible by improving device sensing mechanisms such as touch, voice recognition, and eye-tracking. Sensors should be calibrated in a personalized fashion according to users’ preferences. These devices should not harm individuals or the environment in which people live. Therefore, energy consumption and carbon emissions should also be considered during their development.
5.2 Metaverse as a Service & Plateform
Metaverse platform development and deployment are still considered difficult due to various tangible and intangible factors. For example, providing real-time interaction among avatars requires a high-performance internet connection and high-capacity rendering devices. It demands significant computing power and resources to handle real-time user-generated data. Building a secure infrastructure is crucial to establish trust among users and protect their sensitive data from potential hackers.
Avatars play a crucial role in the metaverse experience. Avatar creation is a critical aspect as it should closely resemble the real user, including facial features, color tone, voice, and bodily characteristics, to create a more realistic feel. Manual creation of avatars by new users can be challenging and may affect their interest in the metaverse experience. Therefore, there is a need for automated tools that can generate users’ avatars, such as AI-generated avatars. Utilizing AI-based models can result in more realistic avatars.
Another important aspect is the interaction of avatars in the virtual world, which should align with the users’ intentions. Any time lag in receiving responses or the avatar not responding as desired by the user can impact user interest. Since users may not provide all their details while creating a user profile, the system should autonomously learn from user behavior and mimic the virtual world accordingly, enhancing the overall experience.
Rendering is indeed a crucial factor in providing users with immersive experiences in the metaverse. The metaverse platform should be capable of producing accurate and real-time rendering, faithfully reproducing various natural and physical environments in the virtual world. It should also be scalable to handle multiple users interacting in real-time scenarios. Real-time avatar interaction in a 3D environment requires significant computational power and network bandwidth.
Simulating real-time lighting effects in different atmospheres is a computationally demanding task, necessitating high computational power and rendering capacity. Furthermore, for a truly realistic effect, the metaverse’s virtual environment should incorporate and express physics effects, such as the laws of motion and elasticity, which require substantial computing power.
To address rendering challenges, various solutions have been proposed and are actively being researched. These include leveraging parallel computing power, utilizing ray tracing mechanisms, AI-assisted rendering, and traditional algorithm-based approaches. Among these, AI-assisted methods are often considered more economical and efficient.
By employing AI-assisted rendering techniques, the metaverse platform can optimize computational resources and enhance the rendering process, resulting in more realistic and immersive visual experiences for users.
The metaverse stores a large amount of data, including users’ personal privacy information. Protecting the security and privacy of this data is a critical issue that needs to be addressed.
The current interaction mode in the metaverse primarily relies on traditional input devices such as keyboards, mouse, and controllers. Exploring more natural interaction modes, such as gesture-based, speech-based, and eye movement control, is an important area for research.
5.7 Security of Virtual Reality Technology
The rise of the metaverse brings about security concerns. With users being highly connected within the metaverse, social networks become targets for malware authors. This exposes users to ransomware attacks, compelling them to engage in activities they wouldn’t willingly do in the physical world. Therefore, enhancing user security while enjoying immersive experiences is an urgent issue that requires attention.
5.7.1 Content Creation and Management
The metaverse is still in its early stages, and many people remain unaware of its potential. Simply relying on Internet promotion and academic publications is insufficient. The metaverse requires a vast amount of engaging content to attract users, but creating and managing such content demands significant manpower and resources. Finding ways to reduce content production costs and enhance content quality poses an important technical challenge.
The avatar should be able to move freely within one session and sometimes across sessions. Interconnecting different sessions or virtual worlds presents many challenges, such as identity management and currency management. One feasible solution for this is to use blockchain technology [165–167]. In May 2022, the World Economic Forum officially recognized the metaverse as a future challenge and opportunity. They called upon individuals from academia, industry, and government to take the initiative in addressing the forthcoming surge of the metaverse. This meeting aimed to create an economically viable, safe, interoperable, equitable, and inclusive metaverse1.
Session management is indeed a crucial aspect of the metaverse. Determining the duration and resource allocation for each session, as well as the amount of data to collect and store for future users, are important considerations. With potentially millions of users joining a session, it becomes essential to optimize computational, spatial, and network usage.
Managing session duration and resource allocation involves balancing the needs of different users and their data requirements. Some user data may need to persist for longer periods than others, requiring efficient storage and retrieval mechanisms. Additionally, real-time data generated by users during the session must be processed and stored, which further adds to the computational and network requirements.
Ensuring real-time effects and interactions within the metaverse necessitates the efficient processing and communication of the vast volume of data generated per session. Furthermore, as avatars move across different sessions in interoperable metaverse platforms, data communication between sessions becomes crucial.
Researchers are actively working to address these challenges and improve session management in the metaverse. By optimizing computational resources, spatial utilization, and network capabilities, they aim to enhance the overall metaverse experience for end users.
5.7.4 User-to-User Interaction
User-to-user interaction inside a metaverse poses similar challenges to real-world interaction, along with additional vulnerabilities. In the real world, it is important to ensure a secure and safe environment, such as protecting a company’s managerial policies from being revealed to employees or outsiders. This prevents hackers and competitors from exploiting sensitive company information. Similarly, in the metaverse, it is possible for one avatar to stalk another, obtain private details, and engage in harassment or unlawful activities. Voice propagation should be limited to avatars present in meetings or rooms, rather than being audible to everyone in the session. Following real-world physics, the audibility of voices should decrease as avatars move further apart. Physical visibility and voice audibility should be restricted to authorized avatars, addressing important privacy concerns in the metaverse.
Cultural, language and racial barriers should be avoided within the metaverse. Language barriers can be overcome with the assistance of AI-driven translation tools. Similarly to the real world, avatars may encounter disputes and conflicts, necessitating the establishment of a governing body to resolve such issues. Inclusive metaverse hosting and management programs should be designed to accommodate people from different backgrounds. Monitoring the number of participants in each session and conducting previous history checks are necessary for maintaining a safe metaverse environment.
Hani et al. [164] come with these questions that need to be addressed for successful metaverse applications. Since the metaverse aims to provide an autonomous replica of the real world, there should also be a virtual economic system in place, which presents several challenges to solve. For instance, how should computational and spatial resources be allocated to businesses? Should all businesses be allowed in each session? What type of economy will the metaverse support? Will it mirror the real-world economy or establish a separate virtual economy exclusive to the metaverse? Additionally, should goods and services be limited to the metaverse or be exchangeable with the real world, where users can purchase items in the metaverse and have them delivered physically?
These questions also raise concerns about shipping goods across different countries where users may be participating in the session. Another important aspect is the management of the tax system in the metaverse. How will personalized advertisements be implemented in the metaverse? Furthermore, it is crucial to address user privacy concerns while still providing personalized recommendations. Who will manage user purchase details and transactions within the metaverse? These are all significant considerations that metaverse-providing platforms need to tackle before offering the metaverse as a service.
User-object interaction in the metaverse indeed requires significant computing and spatial resources. The dynamic nature of allowing users to add their own objects within a session raises questions about visibility to other avatars, which adds complexity to object management. This can result in a crowded environment, placing additional computational and spatial burdens on rendering and object management [104].
Ownership of objects within the metaverse, as digital assets, presents challenges related to issues such as theft. To create an immersive experience, objects should appear natural and be responsive to physical actions. They should adhere to the laws of physics, such as being pushed and moved according to their weight. This also means that other avatars may be able to move or potentially steal objects. Managing the consistent movement of multiple objects within a session, especially with multiple avatars and across different metaverse services, poses a challenging task with limited computing power. Digital ownership and privacy, including theft prevention, are active research areas for researchers.
The question of whether a user can carry a digital asset across various metaverse sessions and whether ownership persists beyond the current session is important to address. Advanced machine learning algorithms can be employed to deliver on-demand resources to users, including computing power, space, digital assets, and environments. Considering the current challenges of user-object interaction, blockchain technology can play a role in providing solutions. Advanced sensors are also necessary to evaluate object dimensions, volume, mass, and respond accordingly. For the creation of dynamic objects and environments, ML-based generative models are required.
6 Limitations of Metaverse Technologies
The limitations of the metaverse can indeed impact its wide acceptability among users. Here are some key limitations to consider.
Accessing the metaverse through devices like Head Mounted Displays (HMDs) can impose additional cognitive burden on users, particularly during extended usage. This can lead to user fatigue and discomfort.
Extended usage of XR technologies, even for relatively short periods of 10-15 minutes, can cause discomfort and motion sickness in some users. This can be a limitation when considering applications that require prolonged user engagement, especially for larger participant groups like students.
Current XR technologies may not be suitable for all individuals, considering factors such as age, visual impairments, and physical limitations. Users who wear glasses may find it uncomfortable to wear HMDs, and individuals with limited hand movements may face challenges in using controllers or interacting with the metaverse.
One of the limitations of the metaverse is the affordability of XR technologies, particularly HMDs. These devices can be costly, making them inaccessible for users with limited financial resources. Additionally, the requirement for high-speed internet connectivity further adds to the affordability challenge. Users in areas with limited internet infrastructure may not be able to fully utilize XR technologies.
The metaverse relies on user-generated and real-world data to deliver personalized experiences. While this enables a more immersive user experience, it also raises concerns about privacy and safety. Storing and tracking user data creates potential vulnerabilities that hackers can exploit for unlawful activities, such as identity theft, stalking, or manipulation. Addressing user comfort, health considerations, and accessibility is crucial for the development and adoption of metaverse technologies. By investing in research and advancements, we can mitigate the limitations associated with cognitive load, time constraints, and accessibility issues. User comfort should be prioritized to prevent cognitive burden and fatigue during prolonged usage of XR technologies. Additionally, efforts should be made to improve the user experience and reduce the potential for motion sickness or discomfort.
Furthermore, promoting accessibility in the metaverse involves ensuring that XR technologies cater to diverse user needs. This encompasses considerations for users with visual impairments, hearing impairments, mobility limitations, and other accessibility requirements. By designing inclusive interfaces, providing alternative interaction methods, and accommodating different abilities, we can create a more inclusive metaverse experience for all users.
Ultimately, by addressing these limitations and prioritizing user comfort, health, and accessibility, we can enhance the acceptance and adoption of metaverse technologies, making them more accessible and enjoyable for a wider range of users.
7 The Future Development Trends of the Metaverse
7.1 Technological Development Trends
In addition to entertainment, games, virtual socializing, education, and healthcare, the metaverse can also be applied in many other fields, such as smart cities [168,169], commerce [162], transportation [163], and culture. For example, virtual tourism [170,171] is applied in the metaverse, where users can personally experience tourist attractions around the world and learn about different cultures and customs through virtual reality technology. The metaverse can help improve businesses by providing virtual advertisements for products [172]. In virtual shopping, the metaverse can provide a virtual shopping mall where users can choose and purchase products without leaving their homes. In the application of virtual real estate in the metaverse, users can purchase virtual properties, design and decorate their own virtual houses, and communicate and share with other users. The application of a virtual office in the metaverse can provide a virtual workspace for remote workers, thereby achieving a more realistic work experience [173]. In short, as a virtual world, the metaverse can provide many new application scenarios in various fields.
In terms of interaction technology, the interaction within the future metaverse will be more natural and efficient. XR technology, being the core of the metaverse, has been implemented in both domestic and foreign countries in recent years. It encompasses not only headband systems but also gloves, wristbands, and other types of devices. This body-sensing technology can also be applied to other types of devices such as body-sensing clothing and handles, and it is compatible with AR glasses or VR headsets. Although XR technology has been applied and popularized to a certain extent, it is still in the early stages of development, and VR devices available in the market offer different functionalities. By improving the innovative new functions of devices, chips, and technology, the user’s visual and tactile experience can be enhanced.
As for holographic technology, there are certain challenges to address. Firstly, cost is an important consideration. Additionally, there are still many difficulties and limitations in holographic communication. Moreover, holographic communication involves the use of important privacy information, such as facial recognition. Therefore, ensuring the security and confidentiality of data transmission is particularly important, and further advancements in information technology are needed to achieve the widespread application of holographic communication in professional fields, such as medical treatment.
In terms of sensory interconnection technology, high bandwidth and low latency are required for network technology to carry multidimensional sensory signals, ensuring consistency and real-time transmission. These technological requirements are crucial to provide a seamless and immersive experience within the metaverse. Blockchain technology can offer an open, decentralized environment for the metaverse, as well as a fair and equitable system for distributing digital assets. The unique advantages of decentralization will drive the design and development of free and decentralized network access infrastructure. In terms of communication and computing resource management mechanisms, the need for consensus among multiple parties in blockchain can lead to significant communication overhead, necessitating the use of new communication and computer resource management mechanisms. Encryption identity frameworks play a crucial role in regulation and privacy protection. As a new field, the metaverse needs to establish its own security and regulatory framework to govern user behavior. Moreover, in the metaverse, supervision of virtual assets is as important as offline supervision of physical assets. Therefore, a regulatory strategy is urgently needed to address future privacy protection issues.
Furthermore, future hardware devices will be more intelligent, ergonomic, and capable of providing a more realistic virtual reality experience. These advancements in hardware technology will enhance user immersion and overall virtual reality experience.
7.2 Application Development Trends
With technological advancements and the broadening of application scenarios, the metaverse will encompass a wider range of fields, including enterprises, governments, and the military. As an emerging concept, the metaverse still has ample room for growth and development in terms of its applications and directions. As various technologies continue to evolve and improve, the adoption of the metaverse will become more widespread. Metaverse application development has attracted considerable interest from both industry and academia, driving advancements in underlying technologies and showcasing a thriving trend. This has propelled the progress of technologies such as blockchain, virtual reality, cloud computing, and network communication. The trajectory of application development in the upcoming years is expected to be increasingly intense. This paper provides a comprehensive overview of the future of the metaverse across six dimensions, including socialization, decentralization, interactivity, and cross-platform, as outlined in Table 5.
This article offers a comprehensive overview of the metaverse, including its concept, development history, technology, application scenarios, and future trends. First, we introduce the concept, characteristics, and development course of the metaverse. Next, we discuss the key technologies for constructing the metaverse. The article examines the role of blockchain in securing data and enhancing interoperability within the metaverse, offering technical insights into how blockchain can address challenges related to data privacy, sharing, and so on. We also present various application scenarios of the metaverse, such as education-where immersive virtual worlds foster critical 21st-century skills-entertainment, and other fields, with expectations for its broader application in the future. Additionally, we synthesize the challenges and limitations of metaverse technologies and outline future research directions for its development.
Acknowledgement: The authors would like to thank the anonymous reviewers for their helpful comments to improve the technical quality of the paper. We also want to acknowledge that we have used software tools such as ChatGPT for language correction and restructuring.
Funding Statement: This paper is supported by Key Scientific Research Projects of Colleges and Universities in Henan Province (Grant No. 23A520054), Henan Provincial Science and Technology Research and Development Program Joint Fund (Application Research) (Grant No. 232103810045), Henan Province High end Foreign Expert Introduction Program Project (Grant No. HNGD2024034), and Open Foundation of State Key Laboratory of Networking and Switching Technology (Beijing University of Posts and Telecommunications) (Grant No. SKLNST-2020-2-01).
Author Contributions: Draft manuscript preparation: Shi Dong, Mengke Liu; Study conception and design: Khushnood Abbas. All authors reviewed the results and approved the final version of the manuscript.
Availability of Data and Materials: Not applicable.
Ethics Approval: Not applicable.
Conflicts of Interest: The authors declare no conflicts of interest to report regarding the present study.
1https://www.weforum.org/reports/interoperability-in-the-metaverse (accessed on 10 August 2024).
References
1. M. Xu et al., “A full dive into realizing the edge-enabled metaverse: Visions, enabling technologies, and challenges,” IEEE Commun. Surv. Tutorials, vol. 25, no. 1, pp. 656–700, Jan. 2023. doi: 10.1109/COMST.2022.3221119. [Google Scholar] [CrossRef]
2. H. Duan et al., “Metaverse for social good: A university campus prototype,” in Proc. 29th ACM Int. Conf. Multimed., New York, NY, USA: Association for Computing Machinery, 2021, pp. 153–161. [Google Scholar]
3. S. Kraus, D. K. Kanbach, P. M. Krysta, M. M. Steinhoff, and N. Tomini, “Facebook and the creation of the metaverse: Radical business model innovation or incremental transformation?” Int. J. Entrep. Behav. Res., vol. 28, no. 9, pp. 52–77, 2022. doi: 10.1108/IJEBR-12-2021-0984. [Google Scholar] [CrossRef]
4. P. Himangi and M. Singla, “Investigating role of deep learning in metaverse,” Int. J. New Pract. Manag. Eng., vol. 11, no. 1, pp. 53–60, 2022. [Google Scholar]
5. K. G. Nalbant, Ş. Uyanık, “Computer vision in the metaverse,” J. Metaverse, vol. 1, no. 1, pp. 9–12, 2021. [Google Scholar]
6. T. Huynh-The et al., “Blockchain for the metaverse: A review,” Future Gener. Comput. Syst., vol. 143, pp. 401–419, 2023. doi: 10.1016/j.future.2023.02.008. [Google Scholar] [CrossRef]
7. D. Mourtzis, J. Angelopoulos, and N. Panopoulos, “Blockchain integration in the era of industrial metaverse,” Appl. Sci., vol. 13, no. 3, 2023, Art. no. 1353. doi: 10.3390/app13031353. [Google Scholar] [CrossRef]
8. S. Dhelim et al., “Edge-enabled metaverse: The convergence of metaverse and mobile edge computing,” 2022. doi: 10.48550/ARXIV.2205.02764. [Google Scholar] [CrossRef]
9. D. K. Murala and S. K. Panda, “The internet of things in developing metaverse,” in Metaverse and Immersive Technologies: An Introduction to Industrial, Business and Social Applications. USA: John Wiley & Sons, Ltd., pp. 437–465, 2023. [Google Scholar]
10. K. Li et al., “When internet of things meets metaverse: Convergence of physical and cyber worlds,” IEEE Internet Things J., vol. 10, no. 5, pp. 4148–4173, 2022. doi: 10.1109/JIOT.2022.3232845. [Google Scholar] [CrossRef]
11. M. Gattullo, E. Laviola, A. Evangelista, M. Fiorentino, and A. E. Uva, “Towards the evaluation of augmented reality in the metaverse: Information presentation modes,” Appl. Sci., vol. 12, no. 24, 2022, Art. no. 12600. doi: 10.3390/app122412600. [Google Scholar] [CrossRef]
12. A. D. Yemenici, “Entrepreneurship in the world of metaverse: Virtual or real?” J. Metaverse, vol. 2, no. 2, pp. 71–82, 2022. doi: 10.57019/jmv.1126135. [Google Scholar] [CrossRef]
13. D. Chen and R. Zhang, “Exploring research trends of emerging technologies in health metaverse: A bibliometric analysis,” SSRN Electron. J., 2022. doi: 10.2139/ssrn.3998068. [Google Scholar] [CrossRef]
14. Z. Chen, “Metaverse office: Exploring future teleworking model,” Kybernetes, vol. 53, no. 6, pp. 2029–2045, 2024. doi: 10.1108/K-10-2022-1432. [Google Scholar] [CrossRef]
15. G. S. Freyermuth, “Vegas, disney, and the metaverse,” in Playful Materialities: The Stuff That Games Are Made of, vol. 14, pp. 17–98, 2022. doi: 10.14361/9783839462003-002. [Google Scholar] [CrossRef]
16. M. Wang, H. Yu, Z. Bell, and X. Chu, “Constructing an edu-metaverse ecosystem: A new and innovative framework,” IEEE Trans. Learn. Technol., vol. 15, no. 6, pp. 685–696, 2022. doi: 10.1109/TLT.2022.3210828. [Google Scholar] [CrossRef]
17. M. Weinberger, “What is metaverse?—A definition based on qualitative meta-synthesis,” Future Internet, vol. 14, no. 11, 2022, Art. no. 310. doi: 10.3390/fi14110310. [Google Scholar] [CrossRef]
18. G. Riva and B. K. Wiederhold, “What the metaverse is (really) and why we need to know about it,” Cyberpsychol. Behav., Soc. Netw., vol. 25, no. 6, pp. 355–359, 2022. doi: 10.1089/cyber.2022.0124. [Google Scholar] [PubMed] [CrossRef]
19. E. VanDerHorn and S. Mahadevan, “Digital twin: Generalization, characterization and implementation,” Decis. Support Syst., vol. 145, 2021, Art. no. 113524. doi: 10.1016/j.dss.2021.113524. [Google Scholar] [CrossRef]
20. M. Ball, The Metaverse: And How It Will Revolutionize Everything. UK: Routledge, 2023, vol. 25, pp. 98–101. [Google Scholar]
21. A. Abilkaiyrkyzy, A. Elhagry, F. Laamarti, and A. Elsaddik, “Metaverse key requirements and platforms survey,” IEEE Access, vol. 11, pp. 117765–117787, 2023. doi: 10.1109/ACCESS.2023.3325844. [Google Scholar] [CrossRef]
22. H. Ning et al., “A survey on the metaverse: The state-of-the-art, technologies, applications, and challenges,” IEEE Internet Things J., vol. 10, no. 16, pp. 14671–14688, 2023. doi: 10.1109/JIOT.2023.3278329. [Google Scholar] [CrossRef]
23. Q. L. Pu, Y. Pang, B. Peng, C. J. Hu, and A. Y. Zhang, “Metaverse report-future is here: Global XR industry insight,” Mar. 2022. Accessed: Aug. 10, 2024. [Online]. Available: https://www2.deloitte.com/content/dam/Deloitte/cn/Documents/technology-media-telecommunications/deloitte-cn-tmt-metaverse-report-en-220321.pdf [Google Scholar]
24. G. He, Y. He, H. Liu, and Z. Guo, “Engineering integrated multimodal sensing and feedback ring system for advanced interactive metaverse platform,” Sci. Bull., vol. 68, no. 2, pp. 133–135, 2023. doi: 10.1016/j.scib.2023.01.009. [Google Scholar] [PubMed] [CrossRef]
25. M. E. Latoschik et al., “The effect of avatar realism in immersive social virtual realities,” in Ser. VRST’17, Gothenburg, Sweden: Association for Computing Machinery, 2017. [Google Scholar]
26. J. Romero, D. Tzionas, and M. J. Black, “Embodied hands: Modeling and capturing hands and bodies together,” ACM Trans. Graph., vol. 36, no. 6, pp. 245:1–245:17, 2017. doi: 10.1145/3130800.3130883. [Google Scholar] [CrossRef]
27. S. Saito, J. Yang, Q. Ma, and M. J. Black, “Scanimate: Weakly supervised learning of skinned clothed avatar networks,” in IEEE Conf. Comput. Vis. Pattern Recognit., CVPR 2021, Computer Vision Foundation/IEEE, 2021, pp. 2886–2897. [Google Scholar]
28. G. Pavlakos et al., “Expressive body capture: 3D hands, face, and body from a single image,” in IEEE Conf. Comput. Vis. Pattern Recognit., CVPR 2019, Long Beach, CA, USA: Computer Vision Foundation/IEEE, 2019, pp. 10975–10985. [Google Scholar]
29. L. Heller and L. Goodman, “What do avatars want now? Posthuman embodiment and the technological sublime,” in 22nd Int. Conf. Virtual Syst. Multimed. (VSMM), 2016, pp. 1–4. doi: 10.1109/VSMM.2016.7863165. [Google Scholar] [CrossRef]
30. D. Gajic, S. Mihic, D. Dragan, V. Petrovic, and Z. Anisic, “Simulation of photogrammetry-based 3D data acquisition,” Int. J. Simul. Model., vol. 18, no. 1, pp. 59–71, Mar. 2019. doi: 10.2507/IJSIMM18(1)460. [Google Scholar] [CrossRef]
31. T. Gesslein, D. Scherer, and J. Grubert, “BodyDigitizer: An open source photogrammetry-based 3D body scanner,” 2017. doi: 10.48550/arXiv.1710.01370. [Google Scholar] [CrossRef]
32. M. Botvinick and J. Cohen, “Rubber hands ‘feel’ touch that eyes see,” Nature, vol. 391, no. 6669, 1998, Art. no. 756. doi: 10.1038/35784. [Google Scholar] [PubMed] [CrossRef]
33. A. Feng et al., “Rapid avatar capture and simulation using commodity depth sensors,” in SIGGRAPH ’14: ACM SIGGRAPH 2014 Talks, Vancouver, Canada: Association for Computing Machinery, 2014. [Google Scholar]
34. A. Visconti, D. Calandra, and F. Lamberti, “Comparing technologies for conveying emotions through realistic avatars in virtual reality-based metaverse experiences,” Comput. Animat. Virtual Worlds, vol. 34, no. 3–4, 2023. doi: 10.1002/cav.2188. [Google Scholar] [CrossRef]
35. G. Batnasan, M. Gochoo, M. Otgonbold, F. Alnajjar, and T. K. Shih, “Ars1211: Arabic sign language letter dataset benchmarking and an educational avatar for metaverse applications,” in IEEE Glob. Eng. Educ. Conf., EDUCON 2022, Tunis, Tunisia: IEEE, 2022, pp. 1814–1821. [Google Scholar]
36. Q. Min, Y. Lu, Z. Liu, C. Su, and B. Wang, “Machine learning based digital twin framework for production optimization in petrochemical industry,” Int. J. Inf. Manag., vol. 49, pp. 502–519, 2019. doi: 10.1016/j.ijinfomgt.2019.05.020. [Google Scholar] [CrossRef]
37. J. Tong, J. Zhou, L. Liu, Z. Pan, and H. Yan, “Scanning 3D full human bodies using kinects,” IEEE Trans. Vis. Comput. Graph., vol. 18, no. 4, pp. 643–650, 2012. doi: 10.1109/tvcg.2012.56. [Google Scholar] [PubMed] [CrossRef]
38. A. Villani et al., “Avatarm: An avatar with manipulation capabilities for the physical metaverse,” in 2023 IEEE Int. Conf. Robot. Autom. (ICRA), IEEE, 2023, pp. 11626–11632. [Google Scholar]
39. H. Yoon, Y. Lee, and C. Shin, “Avatar-based metaverse interactions: A taxonomy, scenarios and enabling technologies,” J. Multim. Inf. Syst., vol. 9, no. 4, pp. 293–298, 2022. doi: 10.33851/jmis.2022.9.4.293. [Google Scholar] [CrossRef]
40. R. Kato, Y. Kikuchi, V. Yem, and Y. Ikei, “Reality avatar for customer conversation in the metaverse,” in Human Interface and the Management of Information: Applications in Complex Technological Environments. Cham: Springer, 2022, vol. 13306, pp. 131–145. [Google Scholar]
41. K. Lam et al., “Human-avatar interaction in metaverse: Framework for full-body interaction,” in Proc. 4th ACM Int. Conf. Multimedia Asia, MMAsia 2022, Tokyo, Japan: ACM, 2022, pp. 10:1–10:7. [Google Scholar]
42. J. Yang, Y. Zhou, H. Huang, H. Zou, and L. Xie, “MetaFi: Device-free pose estimation via commodity wifi for metaverse avatar simulation,” 2022. doi: 10.48550/arXiv.2208.10414. [Google Scholar] [CrossRef]
43. K. Yang, Z. Zhang, T. Youliang, and J. Ma, “A secure authentication framework to guarantee the traceability of avatars in metaverse,” IEEE Trans. Inf. Forensics Secur., vol. 18, pp. 3817–3832, 2023. doi: 10.1109/TIFS.2023.3288689. [Google Scholar] [CrossRef]
44. A. Fuller, Z. Fan, C. Day, and C. Barlow, “Digital twin: Enabling technologies, challenges and open research,” IEEE Access, vol. 8, pp. 108952–108971, 2020. doi: 10.1109/ACCESS.2020.2998358. [Google Scholar] [CrossRef]
45. Y. Zheng, S. Yang, and H. Cheng, “An application framework of digital twin and its case study,” J. Ambient Intell. Humaniz. Comput., vol. 10, no. 3, pp. 1141–1153, 2019. doi: 10.1007/s12652-018-0911-3. [Google Scholar] [CrossRef]
46. C. Cimino, E. Negri, and L. Fumagalli, “Review of digital twin applications in manufacturing,” Comput. Ind., vol. 113, 2019. doi: 10.1016/j.compind.2019.103130. [Google Scholar] [CrossRef]
47. M. Grieves, “Digital twin: Manufacturing excellence through virtual factory replication,” Nov. 2015. Accessed: Aug. 10, 2024. [Online]. Available: https://www.researchgate.net/publication/275211047_Digital_Twin_Manufacturing_Excellence_through_Virtual_Factory_Replication [Google Scholar]
48. C. Menezes, B. Frana, and Y. Lopes, “A survey on extended reality, digital twins, and metaverse applications in power systems,” IEEE Internet Things J., 2024. doi: 10.1109/JIOT.2024.3450813. [Google Scholar] [CrossRef]
49. E. Glaessgen and D. Stargel, “The digital twin paradigm for future nasa and us air force vehicles,” in 53rd AIAA/ASME/ASCE/AHS/ASC Struct., Struct. Dyn. Mater. Conf., Honolulu, Hawaii, 2012. doi: 10.2514/6.2012-1818. [Google Scholar] [CrossRef]
50. Y. Han et al., “A dynamic hierarchical framework for iot-assisted digital twin synchronization in the metaverse,” IEEE Internet Things J., vol. 10, no. 1, pp. 268–284, 2023. doi: 10.1109/JIOT.2022.3201082. [Google Scholar] [CrossRef]
51. H. T. Nguyen, A. Hussein, M. A. Garratt, and H. A. Abbass, “Swarm metaverse for multi-level autonomy using digital twins,” Sensors, vol. 23, no. 10, 2023, Art. no. 4892. doi: 10.3390/s23104892. [Google Scholar] [PubMed] [CrossRef]
52. T. J. Chua, W. Yu, and J. Zhao, “Mobile edge adversarial detection for digital twinning to the metaverse: A deep reinforcement learning approach,” IEEE Trans. Wirel. Commun., vol. 12, pp. 316–322, 2023. doi: 10.1109/ICC45041.2023.10279064. [Google Scholar] [CrossRef]
53. S. K. Jagatheesaperumal et al., “Semantic-aware digital twin for metaverse: A comprehensive review,” IEEE Wirel. Commun., vol. 30, no. 4, pp. 38–46, 2023. doi: 10.1109/MWC.003.2200616. [Google Scholar] [CrossRef]
54. S. K. Jagatheesaperumal and M. Rahouti, “Building digital twins of cyber physical systems with metaverse for Industry 5.0 and beyond,” IT Prof., vol. 24, no. 6, pp. 34–40, 2022. doi: 10.1109/MITP.2022.3225064. [Google Scholar] [CrossRef]
55. X. Wang, J. Yang, J. Han, W. Wang, and F. Wang, “Metaverses and demetaverses: From digital twins in CPS to parallel intelligence in CPSS,” IEEE Intell. Syst., vol. 37, no. 4, pp. 97–102, 2022. doi: 10.1109/MIS.2022.3196592. [Google Scholar] [CrossRef]
56. Z. Lv, L. Qiao, Y. Li, Y. Yuan, and F. Wang, “BlockNet: Beyond reliable spatial digital twins to parallel metaverse,” Patterns, vol. 3, no. 5, 2022, Art. no. 100468. doi: 10.1016/j.patter.2022.100468. [Google Scholar] [PubMed] [CrossRef]
57. P. Antonijevic, M. Iqbal, G. Ubakanma, and T. Dagiuklas, “The metaverse evolution: Toward future digital twin campuses,” in Hum.-Centered Cogn. Syst., HCCS 2022, Shanghai, China: IEEE, 2022, pp. 1–8. [Google Scholar]
58. Y. Wang et al., “A survey on metaverse: Fundamentals, security, and privacy,” IEEE Commun. Surv. Tutorials, vol. 25, no. 1, pp. 319–352, Jan. 2023. doi: 10.1109/COMST.2022.3202047. [Google Scholar] [CrossRef]
59. C. Kai, H. Zhou, Y. Yi, and W. Huang, “Collaborative cloud-edge-end task offloading in mobileedge computing networks with limited communication capability,” IEEE Trans. Cogn. Commun. Netw., vol. 7, no. 2, pp. 624–634, 2020. doi: 10.1109/TCCN.2020.3018159. [Google Scholar] [CrossRef]
60. D. Pimentel et al., “An introduction to learning in the metaverse,” in Meridian Treehouse, 2022. Accessed: Aug. 10, 2024. [Online]. Available: https://www.meridiantreehouse.com/metaverse-education-guide [Google Scholar]
61. L. Dawley and C. Dede, “Situated learning in virtual worlds and immersive simulations,” in Handbook of Research on Educational Communications and Technology, 2014, pp. 723–734. doi: 10.1007/978-1-4614-3185-5. [Google Scholar] [CrossRef]
62. B. O. Spitzer et al., “Framework for the use of extended reality modalities in AEC education,” Buildings, vol. 12, no. 12, 2022, Art. no. 2169. doi: 10.3390/buildings12122169. [Google Scholar] [CrossRef]
63. C. P. Kwok and Y. M. Tang, “A fuzzy MCDM approach to support customer-centric innovation in virtual reality (VR) metaverse headset design,” Adv. Eng. Inform., vol. 56, no. 3, 2023, Art. no. 101910. doi: 10.1016/j.aei.2023.101910. [Google Scholar] [CrossRef]
64. V. Patel, A. Chesmore, C. M. Legner, and S. Pandey, “Trends in workplace wearable technologies and connected-worker solutions for next-generation occupational safety, health, and productivity,” Adv. Intell. Syst., vol. 4, no. 1, 2022, Art. no. 2100099. doi: 10.1002/aisy.202100099. [Google Scholar] [CrossRef]
65. Z. Wang et al., “Vision, application scenarios, and key technology trends for 6G mobile communications,” Sci. China Inf. Sci., vol. 65, no. 5, 2022, Art. no. 151301. doi: 10.1007/s11432-021-3351-5. [Google Scholar] [CrossRef]
66. D. J. Yeong, G. Velasco-Hernandez, J. Barry, and J. Walsh, “Sensor and sensor fusion technology in autonomous vehicles: A review,” Sensors, vol. 21, no. 6, 2021, Art. no. 2140. doi: 10.3390/s21062140. [Google Scholar] [PubMed] [CrossRef]
67. M. Yu et al., “A self-sensing soft pneumatic actuator with closed-loop control for haptic feedback wearable devices,” Mater. Des., vol. 223, no. 6, pp. 111–149, 2022. doi: 10.1016/j.matdes.2022.111149. [Google Scholar] [CrossRef]
68. P. A. Kara et al., “Connected without disconnection: Overview of light field metaverse applications and their quality of experience,” Displays, vol. 78, no. 21, 2023, Art. no. 102430. doi: 10.1016/j.displa.2023.102430. [Google Scholar] [CrossRef]
69. S. Dong, P. Wang, and K. Abbas, “A survey on deep learning and its applications,” Comput. Sci. Rev., vol. 40, no. 1, 2021, Art. no. 100379. doi: 10.1016/j.cosrev.2021.100379. [Google Scholar] [CrossRef]
70. S. B. Kotsiantis, “Supervised machine learning: A review of classification techniques,” in Emerging Artificial Intelligence Applications in Computer Engineering-Real Word AI Systems with Applications in eHealth, HCI, Information Retrieval and Pervasive Technologies, Ser. Frontiers in Artificial Intelligence and Applications, I. Maglogiannis, K. Karpouzis, M. Wallace, J. Soldatos, Eds., IOS Press, 2007, vol. 160, pp. 3–24. [Google Scholar]
71. F. Hu et al., “Unsupervised feature learning via spectral clustering of multidimensional patches for remotely sensed scene classification,” IEEE J. Sel. Top. Appl. Earth Obs. Remote. Sens., vol. 8, no. 5, pp. 2015–2030, 2015. doi: 10.1109/JSTARS.2015.2444405. [Google Scholar] [CrossRef]
72. R. Sheikhpour, M. A. Sarram, S. Gharaghani, and M. A. Z. Chahooki, “A survey on semi-supervised feature selection methods,” Pattern Recognit., vol. 64, no. Suppl 1, pp. 141–158, 2017. doi: 10.1016/j.patcog.2016.11.003. [Google Scholar] [CrossRef]
73. J. E. van Engelen and H. H. Hoos, “A survey on semi-supervised learning,” Mach. Learn., vol. 109, no. 2, pp. 373–440, 2020. doi: 10.1007/s10994-019-05855-6. [Google Scholar] [CrossRef]
74. W. Chen et al., “Deep reinforcement learning for internet of things: A comprehensive survey,” IEEE Commun. Surv. Tutorials, vol. 23, no. 3, pp. 1659–1692, 2021. doi: 10.1109/COMST.2021.3073036. [Google Scholar] [CrossRef]
75. J. Oh, R. Iida, J. Kloetzer, and K. Torisawa, “BERTAC: Enhancing transformer-based language models with adversarially pretrained convolutional neural networks,” in Proceedings of the 59th Annual Meeting of the Association for Computational Linguistics and the 11th International Joint Conference on Natural Language Processing, Association for Computational Linguistics, 2021, pp. 2103–2115. [Google Scholar]
76. T. Young, D. Hazarika, S. Poria, and E. Cambria, “Recent trends in deep learning based natural language processing,” IEEE Comput. Intell. Mag., vol. 13, no. 3, pp. 55–75, 2018. doi: 10.1109/MCI.2018.2840738. [Google Scholar] [CrossRef]
77. M. Daniluk, T. Rocktschel, J. Welbl, and S. Riedel, “Frustratingly short attention spans in neural language modeling,” in 5th Int. Conf. Learn. Represent., ICLR 2017, Toulon, France, 2017. [Google Scholar]
78. K. Benes, M. K. Baskar, and L. Burget, “Residual memory networks in language modeling: Improving the reputation of feed-forward networks,” in 18th Ann. Conf. Int. Speech Commun. Assoc. (Interspeech 2017), Stockholm, Sweden, 2017, pp. 284–288. [Google Scholar]
79. N. Pham, G. Kruszewski, and G. Boleda, “Convolutional neural network language models,” in Proc. 2016 Conf. Empir. Methods Nat. Lang. Process., EMNLP 2016, Austin, TX, USA: Association for Computational Linguistics, 2016, pp. 1153–1162. [Google Scholar]
80. J. A. F. Thompson, M. Schnwiesner, Y. Bengio, and D. Willett, “How transferable are features in convolutional neural network acoustic models across languages?” in IEEE Int. Conf. Acoust., Speech Signal Process., ICASSP 2019, Brighton, UK: IEEE, 2019, pp. 2827–2831. [Google Scholar]
81. T. Huynh-The et al., “Artificial intelligence for the metaverse: A survey,” Eng. Appl. Artif. Intell., vol. 117, pp. 105–581, 2023. [Google Scholar]
82. X. Mu et al., “Fashion intelligence in the metaverse: Promise and future prospects,” Artificial Intelligence Review, vol. 57, no. 3, pp. 1573–7462, 2024. doi: 10.1007/s10462-024-10703-8. [Google Scholar] [CrossRef]
83. Y. Wang and J. Zhao, “Mobile edge computing, metaverse, 6G wireless communications, artificial intelligence, and blockchain: Survey and their convergence,” in 2022 IEEE 8th World Forum Internet Things (WF-IoT), IEEE, 2022, pp. 1–8. [Google Scholar]
84. N. C. Luong et al., “Edge computing for semantic communication enabled metaverse: An incentive mechanism design,” 2022. doi: 10.48550/ARXIV.2212.06463. [Google Scholar] [CrossRef]
85. C. Liu, Y. Wang, and J. Zhao, “Mobile edge computing for the metaverse,” 2022. doi: 10.48550/arXiv.2212.09229. [Google Scholar] [CrossRef]
86. T. J. Chua, W. Yu, and J. Zhao, “Unified, user and task (UUT) centered artificial intelligence for metaverse edge computing,” 2022. doi: 10.48550/arXiv.2212.09295. [Google Scholar] [CrossRef]
87. V. Ahsani, A. Rahimi, M. Letafati, and B. H. Khalaj, “Unlocking metaverse-as-a-service the three pillars to watch: Privacy and security, edge computing, and blockchain,” 2023. doi: 10.48550/arXiv.2301.01221. [Google Scholar] [CrossRef]
88. Y. Wang and J. Zhao, “A survey of mobile edge computing for the metaverse: Architectures, applications, and challenges,” in 8th IEEE Int. Conf. Collab. Int. Comput., CIC 2022, Atlanta, GA, USA: IEEE, 2022, 1–9. [Google Scholar]
89. S. Karunarathna et al., “The role of network slicing and edge computing in the metaverse realization,” IEEE Access, vol. 11, pp. 25502–25530, 2023. doi: 10.1109/ACCESS.2023.3255510. [Google Scholar] [CrossRef]
90. M. Jamshidi et al., “A super-efficient GSM triplexer for 5G-enabled iot in sustainable smart grid edge computing and the metaverse,” Sensors, vol. 23, no. 7, 2023, Art. no. 3775. doi: 10.3390/s23073775. [Google Scholar] [PubMed] [CrossRef]
91. J. Yu, A. Alhilal, P. Hui, and D. H. K. Tsang, “Bi-directional digital twin and edge computing in the metaverse,” IEEE Internet Things Mag., vol. 7, no. 3, pp. 106–112, 2024. doi: 10.1109/IOTM.001.2300173. [Google Scholar] [CrossRef]
92. Y. Jararweh et al., “Software-defined system support for enabling ubiquitous mobile edge computing,” Comput. J., vol. 60, no. 10, pp. 1443–1457, 2017. doi: 10.1093/comjnl/bxx019. [Google Scholar] [CrossRef]
93. M. J. M. Chowdhury, A. Colman, M. A. Kabir, J. Han, and P. Sarda, “Blockchain versus database: A critical analysis,” in 2018 17th IEEE Int. Conf. Trust, Secur. Privacy Comput. Commun./12th IEEE Int. Conf. Big Data Sci. Eng. (TrustCom/BigDataSE), IEEE, 2018, pp. 1348–1353. [Google Scholar]
94. M. Niranjanamurthy, B. Nithya, and S. Jagannatha, “Analysis of blockchain technology: Pros, cons and swot,” Cluster Comput., vol. 22, no. S6, pp. 14743–14757, 2019. doi: 10.1007/s10586-018-2387-5. [Google Scholar] [CrossRef]
95. J. Chen, H. Xiao, M. Hu, and C. -M. Chen, “A blockchain-based signature exchange protocol for metaverse,” Future Gener. Comput. Syst., vol. 142, no. 1, pp. 237–247, 2023. doi: 10.1016/j.future.2022.12.031. [Google Scholar] [CrossRef]
96. D. Wei, “Gemiverse: The blockchain-based professional certification and tourism platform with its own ecosystem in the metaverse,” Int. J. Geoheritage Parks, vol. 10, no. 2, pp. 322–336, 2022. doi: 10.1016/j.ijgeop.2022.05.004. [Google Scholar] [CrossRef]
97. M. Xu et al., “A trustless architecture of blockchain-enabled metaverse,” High-Confid. Comput., vol. 3, no. 1, 2023, Art. no. 100088. doi: 10.1016/j.hcc.2022.100088. [Google Scholar] [CrossRef]
98. Q. Yang et al., “Fusing blockchain and AI with metaverse: A survey,” IEEE Open J. Comput. Soc., vol. 3, no. 3, pp. 122–136, 2022. doi: 10.1109/OJCS.2022.3188249. [Google Scholar] [CrossRef]
99. S. Mishra, H. Arora, G. Parakh, and J. Khandelwal, “Contribution of blockchain in development of metaverse,” in 2022 7th Int. Conf. Commun. Electron. Syst. (ICCES), IEEE, 2022, pp. 845–850. [Google Scholar]
100. Z. Chen, J. Wu, W. Gan, and Z. Qi, “Metaverse security and privacy: An overview,” 2022. doi: 10.48550/arXiv.2211.14948. [Google Scholar] [CrossRef]
101. L. Chang et al., “6G-enabled edge ai for metaverse: Challenges, methods, and future research directions,” J. Commun. Inf. Netw., vol. 7, no. 2, pp. 107–121, 2022. doi: 10.23919/JCIN.2022.9815195. [Google Scholar] [CrossRef]
102. P. Bhattacharya et al., “Towards future internet: The metaverse perspective for diverse industrial applications,” Mathematics, vol. 11, no. 4, 2023, Art. no. 941. doi: 10.3390/math11040941. [Google Scholar] [CrossRef]
103. A. W. Feng, D. Casas, and A. Shapiro, “Avatar reshaping and automatic rigging using a deformable model,” in Proc. 8th ACM SIGGRAPH Conf. Motion Games, MIG 2015, Paris, France: ACM, 2015, pp. 57–64. [Google Scholar]
104. D. Van Huynh, S. R. Khosravirad, A. Masaracchia, O. A. Dobre, and T. Q. Duong, “Edge intelligence-based ultra-reliable and low-latency communications for digital twin-enabled metaverse,” IEEE Wirel. Commun. Lett., vol. 11, no. 8, pp. 1733–1737, 2022. doi: 10.1109/LWC.2022.3179207. [Google Scholar] [CrossRef]
105. R. Cheng, N. Wu, M. Varvello, S. Chen, and B. Han, “Are we ready for metaverse?: A measurement study of social virtual reality platforms,” in Proc. 22nd ACM Internet Meas. Conf., IMC 2022, Nice, France, 2022, pp. 504–518. [Google Scholar]
106. K. Nagao, “Virtual reality campuses as new educational metaverses,” IEICE Trans. Inf. Syst., vol. 106, no. 2, pp. 93–100, 2023. doi: 10.1587/transinf.2022ETI0001. [Google Scholar] [CrossRef]
107. J. Fan, “Theory and method for evaluating the importance of college course teaching for future education: From virtual reality to metaverse,” J. Intell. Fuzzy Syst., vol. 44, no. 4, pp. 5893–5919, 2023. doi: 10.3233/JIFS-220931. [Google Scholar] [CrossRef]
108. P. A. Rospigliosi, “Metaverse or simulacra? Roblox, minecraft, meta and the turn to virtual reality for education, socialisation and work,” Interact. Learn. Environ., vol. 30, no. 1, pp. 1–3, 2022. doi: 10.1080/10494820.2022.2022899. [Google Scholar] [CrossRef]
109. Y. Yang, K. Siau, W. Xie, and Y. Sun, “Smart health intelligent healthcare systems in the metaverse, artificial intelligence, and data science era,” J. Organ. End User Comput., vol. 34, no. 1, pp. 1–14, 2022. doi: 10.4018/joeuc.308814. [Google Scholar] [CrossRef]
110. D. Crevier, AI: The Tumultuous History of the Search for Artificial Intelligence. New York, USA: Basic Books, Inc., 1993, pp. 1–386. [Google Scholar]
111. Z. Qu, S. Yu, and M. Fu, “Motion background modeling based on context-encoder,” in 2016 Third Int. Conf. Artif. Intell. Pattern Recognit. (AIPR), IEEE, 2016, pp. 1–5. [Google Scholar]
112. L. Xu, Y. Li, Y. Wang, and E. Chen, “Temporally adaptive restricted boltzmann machine for background modeling,” in Twenty-Ninth AAAI Conf. Artif. Intell., 2015. [Google Scholar]
113. T. H. Hur et al., “Iss2Image: A novel signal-encoding technique for CNN-based human activity recognition,” Sensors, vol. 18, no. 11, 2018, Art. no. 3910. doi: 10.3390/s18113910. [Google Scholar] [PubMed] [CrossRef]
114. T. Huynh-The, C. Hua, N. A. Tu, and D. Kim, “Physical activity recognition with statistical-deep fusion model using multiple sensory data for smart health,” IEEE Internet Things J., vol. 8, no. 3, pp. 1533–1543, 2021. doi: 10.1109/JIOT.2020.3013272. [Google Scholar] [CrossRef]
115. Y. K. Dwivedi et al., “Metaverse beyond the hype: Multidisciplinary perspectives on emerging challenges, opportunities, and agenda for research, practice and policy,” Int. J. Inf. Manag., vol. 66, no. 3, pp. 102–542, 2022. doi: 10.1016/j.ijinfomgt.2022.102542. [Google Scholar] [CrossRef]
116. J. Holmström, “The consequences of information: Institutional implications of technologicalchange,” Info. Technol. People, vol. 21, pp. 205–207, 2008. doi: 10.1108/ITP.2008.21.2.205.1. [Google Scholar] [CrossRef]
117. P. Mirza-Babaei et al., “Games and the metaverse,” in Extended Abstracts of the Annual Symposium on Computer-Human Interaction in Play, CHI PLAY 2022. Bremen, Germany: ACM, 2022, pp. 318–319. [Google Scholar]
118. J. Xu, “From augmented reality location-based games to the real-world metaverse,” in Extended Abstracts Annu. Symp. Comput.-Human Interact. Play, CHI PLAY 2022, Bremen, Germany: ACM, Nov. 2–5, 2022, pp. 364–366. [Google Scholar]
119. S. Jian, X. Chen, and J. Yan, “From online games to “metaverse”: The expanding impact of virtual reality in daily life,” in Cult. Comput.: 10th Int. Conf. 2022, vol. 13324, pp. 34–43. [Google Scholar]
120. D. Sundaram, V. Sadovykh, G. Peko, and K. Craig, “Introduction to the minitrack on the social and economic dynamics of the metaverse, smart contracts, and non-fungible tokens,” in 56th Hawaii Int. Conf. Syst. Sci., HICSS 2023, Maui, HI, USA: ScholarSpace, 2023, p. 4535. [Google Scholar]
121. Y. Wang, Z. Su, and M. Yan, “Social metaverse: Challenges and solutions,” IEEE Internet Things Mag., vol. 6, no. 3, pp. 144–150, 2023. doi: 10.1109/iotm.001.2200266. [Google Scholar] [CrossRef]
122. B. Chen and D. Yang, “User recommendation in social metaverse with VR,” in Proc. 31st ACM Int. Conf. Inform Knowl. Manag., Atlanta, GA, USA: ACM, 2022, pp. 148–158. [Google Scholar]
123. B. Guidi and A. Michienzi, “Social games and blockchain: Exploring the metaverse of decentraland,” in 42nd IEEE Int. Conf. Distrib. Comput. Syst., ICDCS, Bologna, Italy: IEEE, 2022, 199–204. [Google Scholar]
124. G. Hwang and S. Chien, “Definition, roles, and potential research issues of the metaverse in education: An artificial intelligence perspective,” Comput. Educ. Artif. Intell., vol. 3, 2022, Art. no. 100082. doi: 10.1016/j.caeai.2022.100082. [Google Scholar] [CrossRef]
125. A. Tlili et al., “Is metaverse in education a blessing or a curse: A combined content and bibliometric analysis,” Smart Learn. Environ., vol. 9, no. 1, 2022, Art. no. 24. doi: 10.1186/s40561-022-00205-x. [Google Scholar] [CrossRef]
126. R. Bathla, S. Gupta, and T. S. Manivasagam, “Applications and case studies in metaverse healthcare,” in Examining the Metaverse in Healthcare: Opportunities, Challenges, and Future Directions. USA: IGI Global, 2024, pp. 103–128. [Google Scholar]
127. R. Hare and Y. Tang, “Hierarchical deep reinforcement learning with experience sharing for metaverse in education,” IEEE Trans. Syst. Man Cybern. Syst., vol. 53, no. 4, pp. 2047–2055, 2023. doi: 10.1109/tsmc.2022.3227919. [Google Scholar] [CrossRef]
128. W. Zheng, L. Yan, W. Zhang, L. Ouyang, and D. Wen, “D→k→i: Data-knowledge-driven group intelligence framework for smart service in education metaverse,” IEEE Trans. Syst. Man Cybern. Syst., vol. 53, no. 4, pp. 2056–2061, 2023. doi: 10.1109/tsmc.2022.3228849. [Google Scholar] [CrossRef]
129. D. Ktoridou, E. Epaminonda, and L. Efthymiou, “Is education ready to embrace metaverse?” in IEEE Global Eng. Educ. Conf., EDUCON 2023, Kuwait, Kuwait: IEEE, 2023, pp. 1–5. [Google Scholar]
130. Z. Zhao, B. Zhao, Z. Ji, and Z. Liang, “On the personalized learning space in educational metaverse based on heart rate signal,” Int. J. Inf. Commun. Technol. Educ., vol. 18, no. 2, pp. 1–12, 2022. doi: 10.4018/ijicte.314565. [Google Scholar] [CrossRef]
131. B. Zhou, “Building a smart education ecosystem from a metaverse perspective,” Mob. Inf. Syst., vol. 2022, no. 3, pp. 1–10, 2022. doi: 10.1155/2022/1938329. [Google Scholar] [CrossRef]
132. W. Fu and J. Pan, “Exploration of classroom teaching reform in higher vocational colleges from the perspective of metaverse,” in 2022 Int. Conf. Cloud Comput., Big Data Internet Things (3CBIT), IEEE, 2022, pp. 178–181. [Google Scholar]
133. Y. Hwang, “When makers meet the metaverse: Effects of creating NFT metaverse exhibition in maker education,” Comput. Educ., vol. 194, no. 3, 2023, Art. no. 104693. doi: 10.1016/j.compedu.2022.104693. [Google Scholar] [CrossRef]
134. G. Asiksoy, “Empirical studies on the metaverse-based education: A systematic review,” Int. J. Eng. Pedagog., vol. 13, no. 3, pp. 120–133, 2023. doi: 10.3991/ijep.v13i3.36227. [Google Scholar] [CrossRef]
135. E. S. Mohamed, T. A. Naqishbandi, and G. Veronese, “Metaverse!: Possible potential opportunities and trends in e-healthcare and education,” Int. J. E Adopt., vol. 15, no. 2, pp. 1–21, 2023. doi: 10.4018/IJEA. [Google Scholar] [CrossRef]
136. A. D. Samala et al., “Metaverse technologies in education: A systematic literature review using PRISMA,” Int. J. Emerg. Technol. Learn., vol. 18, no. 5, pp. 231–252, 2023. doi: 10.3991/ijet.v18i05.35501. [Google Scholar] [CrossRef]
137. S. Kaddoura and F. A. Husseiny, “The rising trend of metaverse in education: Challenges, opportunities, and ethical considerations,” PeerJ Comput. Sci., vol. 9, no. 3, 2023, Art. no. e1252. doi: 10.7717/peerj-cs.1252. [Google Scholar] [PubMed] [CrossRef]
138. X. Shu and X. Gu, “An empirical study of A smart education model enabled by the edu-metaverse to enhance better learning outcomes for students,” Systems, vol. 11, no. 2, 2023, Art. no. 75. doi: 10.3390/systems11020075. [Google Scholar] [CrossRef]
139. J. Lee and S. Jang, “Metaverse-based education service adoption and preference study using conjoint analysis,” in Int. Conf. Comput., Netw. Commun., ICNC 2023, Honolulu, HI, USA, IEEE, Feb. 20–22, 2023, pp. 273–277. [Google Scholar]
140. X. Wu, Y. Chen, and Y. Wu, “Exploration of mathematics education by metaverse technology,” in 12th IEEE Int. Conf. Educ. Inf. Technol., ICEIT 2023, Chongqing, China: IEEE, 2023, 173–178. [Google Scholar]
141. A. Al-Marzouqi, A. Aburayya, and S. A. Salloum, “Prediction of user’s intention to use metaverse system in medical education: A hybrid SEM-ML learning approach,” IEEE Access, vol. 10, no. 5, pp. 43421–43434, 2022. doi: 10.1109/ACCESS.2022.3169285. [Google Scholar] [CrossRef]
142. S. Lo and H. Tsai, “Design of 3d virtual reality in the metaverse for environmental conservation education based on cognitive theory,” Sensors, vol. 22, no. 21, 2022, Art. no. 8329. doi: 10.3390/s22218329. [Google Scholar] [PubMed] [CrossRef]
143. T. Zhang, J. Shen, C. Lai, S. Ji, and Y. Ren, “Multi-server assisted data sharing supporting secure deduplication for metaverse healthcare systems,” Future Gener. Comput. Syst., vol. 140, no. 1, pp. 299–310, 2023. doi: 10.1016/j.future.2022.10.031. [Google Scholar] [CrossRef]
144. A. K. Bashir et al., “Federated learning for the healthcare metaverse: Concepts, applications, challenges, and future directions,” IEEE Internet Things J., vol. 10, no. 24, pp. 21873–21891, 2023. doi: 10.1109/JIOT.2023.3304790. [Google Scholar] [CrossRef]
145. G. Bansal, K. Rajgopal, V. Chamola, Z. Xiong, and D. Niyato, “Healthcare in metaverse: A survey on current metaverse applications in healthcare,” IEEE Access, vol. 10, pp. 119914–119946, 2022. doi: 10.1109/ACCESS.2022.3219845. [Google Scholar] [CrossRef]
146. G. Wang et al., “Development of metaverse for intelligent healthcare,” Nat. Mac. Intell., vol. 4, no. 11, pp. 922–929, 2022. doi: 10.1038/s42256-022-00549-6. [Google Scholar] [PubMed] [CrossRef]
147. R. Chengoden et al., “Metaverse for healthcare: A survey on potential applications, challenges and future directions,” IEEE Access, vol. 11, no. 1, pp. 12765–12795, 2023. doi: 10.1109/ACCESS.2023.3241628. [Google Scholar] [CrossRef]
148. A. Musamih et al., “Metaverse in healthcare: Applications, challenges, and future directions,” IEEE Consumer Electron. Mag., vol. 12, no. 4, pp. 33–46, 2023. doi: 10.1109/MCE.2022.3223522. [Google Scholar] [CrossRef]
149. T. J. Ford et al., “Taking modern psychiatry into the metaverse: Integrating augmented, virtual, and mixed reality technologies into psychiatric care,” Front. Digit. Health, vol. 5, 2023, Art. no. 1146806. doi: 10.3389/fdgth.2023.1146806. [Google Scholar] [PubMed] [CrossRef]
150. S. Ali et al., “Metaverse in healthcare integrated with explainable AI and blockchain: Enabling immersiveness, ensuring trust, and providing patient data security,” Sensors, vol. 23, no. 2, 2023, Art. no. 565. doi: 10.3390/s23020565. [Google Scholar] [PubMed] [CrossRef]
151. A. Qayyum et al., “Can we revitalize interventional healthcare with ai-xr surgical metaverses?” in 2023 IEEE Int. Conf. Metaverse Comput., Netw. Appl. (MetaCom), IEEE, 2023, pp. 496–503. [Google Scholar]
152. B. K. Wiederhold, “(mental) healthcare consumerism in the metaverse: Is there a benefit?” Cyberpsychol. Behav. Soc. Netw., vol. 26, no. 3, pp. 145–146, 2023. doi: 10.1089/cyber.2023.29269.editorial. [Google Scholar] [PubMed] [CrossRef]
153. G. Meinlschmidt et al., “Mental health and the metaverse: Ample opportunities or alarming threats for mental health in immersive worlds?” in CHI EA’ 2023: Extended Abstracts 2023 CHI Conf. Human Factors Comput. Syst., Hamburg, Germany: ACM, 2023, pp. 525:1–525:5. [Google Scholar]
154. B. K. Wiederhold, “Metaverse games: Game changer for healthcare?” Cyberpsychol. Behav. Soc. Netw., vol. 25, no. 5, pp. 267–269, 2022. doi: 10.1089/cyber.2022.29246.editorial. [Google Scholar] [PubMed] [CrossRef]
155. L. Ying, Z. Jiong, S. Wei, W. Jingchun, and G. Xiaopeng, “VREX: Virtual reality education expansion could help to improve the class experience (VREX platform and community for VR based education),” in 2017 IEEE Front. Educ. Conf. (FIE), IEEE, 2017, pp. 1–5. [Google Scholar]
156. A. Athar, S. M. Ali, M. A. I. Mozumder, S. Ali, and H. Kim, “Applications and possible challenges of healthcare metaverse,” in 25th Int. Conf. Adv. Commun. Technol., ICACT 2023, Pyeongchang, Republic of Korea: IEEE, 2023, pp. 328–332. [Google Scholar]
157. T. -C. Wu and C. -T. B. Ho, “A scoping review of metaverse in emergency medicine,” Australasian Emergency Care, vol. 26, no. 1, pp. 75–83, 2023. doi: 10.1016/j.auec.2022.08.002. [Google Scholar] [PubMed] [CrossRef]
158. P. Swami, “Metaverse: Transforming the user experience in the gaming and entertainment industry,” Res., Innov., Ind. Impacts Metaverse, pp. 115–128, 2024. doi: 10.4018/979-8-3693-2607-7.ch007. [Google Scholar] [CrossRef]
159. R. V. Rodriguez, K. H., A. Majumdar, and R. Basu, 1st International Conference, ‘Resonance’: on Cognitive Approach, Social Ethics and Sustainability. London, UK: Routledge, 2024. doi: 10.4324/9781003397175. [Google Scholar] [CrossRef]
160. X. Hu, “Analysing the new marketing model for entertainment companies: A case study of SM. entertainment,” SHS Web Conf., vol. 181, no. 3, 2024, Art. no. 01007. doi: 10.1051/shsconf/202418101007. [Google Scholar] [CrossRef]
161. A. Putra, A. Firnanda, M. A. F. Gunawan, and S. Batubara, “Media technology innovation in the transformation of the entertainment industry,” J. Inf. Technol., Comput. Sci. Electr. Eng., vol. 1, no. 2, pp. 60–62, 2024. [Google Scholar]
162. S. Hollensen, P. Kotler, and M. O. Opresnik, “Metaverse-the new marketing universe,” J. Bus. Strategy, vol. 44, no. 3, pp. 119–125, 2023. doi: 10.1108/JBS-01-2022-0014. [Google Scholar] [CrossRef]
163. P. Zhou et al., “Vetaverse: Technologies, applications, and visions toward the intersection of metaverse, vehicles, and transportation systems,” 2022. doi: 10.48550/ARXIV.2210.15109. [Google Scholar] [CrossRef]
164. H. Sami et al., “The metaverse: Survey, trends, novel pipeline ecosystem & future directions,” 2023. doi: 10.48550/arXiv.2304.09240. [Google Scholar] [CrossRef]
165. R. Belchior, A. Vasconcelos, S. Guerreiro, and M. Correia, “A survey on blockchain interoperability: Past, present, and future trends,” ACM Comput. Surv., vol. 54, no. 8, Oct. 2021. doi: 10.1145/3471140. [Google Scholar] [CrossRef]
166. M. Madine et al., “Appxchain: Application-level interoperability for blockchain networks,” IEEE Access, vol. 9, pp. 87777–87791, 2021. doi: 10.1109/ACCESS.2021.3089603. [Google Scholar] [CrossRef]
167. R. Jabbar, N. Fetais, M. Krichen, and K. Barkaoui, “Blockchain technology for healthcare: Enhancing shared electronic health record interoperability and integrity,” in 2020 IEEE Int. Conf. Inf., IoT, Enabling Technol. (ICIoT), 2020, pp. 310–317. [Google Scholar]
168. J. Wang and G. Medvegy, “Exploration the future of the metaverse and smart cities,” in Int. Conf. Electronic Bu., ICEB 20222, Bangkok, Thailand: Association for Information Systems, 2022, p. 12. [Google Scholar]
169. K. Kuru, “MetaOmniCity: Toward immersive urban metaverse cyberspaces using smart city digital twins,” IEEE Access, vol. 11, pp. 43844–43868, 2023. doi: 10.1109/ACCESS.2023.3272890. [Google Scholar] [CrossRef]
170. E. C. Ciliberti, M. Fiore, and M. Mongiello, “Development of a metaverse platform for tourism promotion in apulia,” in 2023 IEEE Int. Conf. Metaverse Comput. Netw. Appl. (MetaCom), IEEE, 2023, pp. 680–681. [Google Scholar]
171. T. Yoon, J. K. Do, and S. C. Jeong, “Developing songjeong metaverse surfing village: Development of metaverse-based platform specialized for marine tourism,” in 7th IEEE/ACIS Int. Conf. Big Data Cloud Comput. Data Sci., BCD 2022, Danang, Vietnam: IEEE, 2022, pp. 276–278. [Google Scholar]
172. H. Du, D. Niyato, C. Miao, J. Kang, and D. I. Kim, “Optimal targeted advertising strategy for secure wireless edge metaverse,” in GLOBECOM 2022—2022 IEEE Global Commun. Conf., IEEE, 2022, pp. 4346–4351. [Google Scholar]
173. L. Oppermann, F. Buchholz, and Y. Uzun, “Industrial metaverse: Supporting remote maintenance with avatars and digital twins in collaborative XR environments,” in CHI EA’ 2023: Extended Abstracts 2023 CHI Conf. Human Factors Comput. Syst., Hamburg, Germany, April 23–28, 2023, pp. 178:1–178:5. [Google Scholar]
Cite This Article
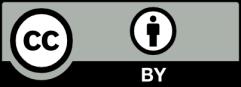
This work is licensed under a Creative Commons Attribution 4.0 International License , which permits unrestricted use, distribution, and reproduction in any medium, provided the original work is properly cited.