Open Access
ARTICLE
Th-Shaped Tunable Multi-Band Antenna for Modern Wireless Applications
1 Department of Telecommunication Engineering, University of Engineering and Technology, Mardan, Pakistan
2 Electrical Engineering Department, College of Engineering, Najran University, Najran, Saudi Arabia
3 Department of Electrical Engineering, University of Engineering and Technology, Mardan, 23200, Pakistan
4 Faculty of Maritimes Studies, King Abdulaziz University, Jeddah, Saudi Arabia
5 Ports & Maritime Transportation, Faculty of Maritimes Studies, King Abdulaziz University, Jeddah, Saudi Arabia
6 Natural Science Department, Faculty of Maritimes Studies, King Abdulaziz University, Jeddah, Saudi Arabia
* Corresponding Author: Fazal Muhammad. Email:
Computers, Materials & Continua 2023, 74(2), 2517-2530. https://doi.org/10.32604/cmc.2023.031833
Received 28 April 2022; Accepted 24 June 2022; Issue published 31 October 2022
Abstract
A compact, reconfigurable antenna supporting multiple wireless services with a minimum number of switches is found lacking in literature and the same became the focus and outcome of this work. It was achieved by designing a Th-Shaped frequency reconfigurable multi-band microstrip planar antenna, based on use of a single switch within the radiating structure of the antenna. Three frequency bands (i.e., 2007–2501 MHz, 3660–3983 MHz and 9341–1046 MHz) can be operated with the switch in the ON switch state. In the OFF state of the switch, the antenna operates within the 2577–3280 MHz and 9379–1033 MHz Bands. The proposed antenna shows an acceptable input impedance match with Voltage Standing Wave Ratio (VSWR) less than 1.2. The peak radiation efficiency of the antenna is 82%. A reasonable gain is obtained from 1.22 to 3.31 dB within the operating bands is achieved. The proposed antenna supports Universal Mobile Telecommunication System (UMTS)-1920 to 2170 MHz, Worldwide Interoperability and Microwave Access (WiMAX)/Wireless Broadband/(Long Term Evolution) LTE2500–2500 to 2690 MHz, Fifth Generation (5G)-2500/3500 MHz, Wireless Fidelity (Wi-Fi)/ Bluetooth-2400 to 2480 MHz, and Satellite communication applications in X-Band-8000 to 12000 MHz. The overall planar dimension of the proposed antenna is 40 × 20 mm2. The antenna was designed, along with the parametric study, using Electromagnetic (EM) simulation tool. The antenna prototype is fabricated for experimental validation with the simulated results. The proposed antenna is low profile, tunable, lightweight, cheap to fabricate and highly efficient and hence is deemed suitable for use in modern wireless communication electronic devices.Keywords
Advancement in the domain of wireless communication requires a single portable electronic device to serve multiple wireless services. Commonly used wireless services for communication and space applications used by European Space Agency plus their ranges are summarized in Tab. 1.
As every wireless technology has its own requirements, multiband antennas with the reconfigurable ability (Frequency, Pattern and Polarization) are the preferred choice in recent wireless systems. These reconfigurable antennas operate on multiple resonant frequencies whereas reconfigurable techniques enhanced its performance in terms of efficiency, battery power, and cost. In a conventional multiband antenna system, the radiation happens to be on multiple frequencies without giving due consideration to the end users’ preferences. Hence more battery power is consumed and degrades overall antenna performance (efficiency). Due to the flexible and attractive features of reconfigurable antennas’ researchers have proposed different designs [1–4]. Reconfigurable mechanisms increase the performance of the system and tuning to desirable operating frequency saves battery power as compared to conventional multiband antenna-based systems which may be radiating unnecessary power at unused bands. Researchers have designed an optically reconfigured monopole antenna for Wireless applications using a truncated ground plane [5].
The arc-shaped slot is investigated in [6] having five PIN diodes in a circular patch antenna for Digital Cellular System 1800 (DCS1800), Universal Mobile Telecommunication System (UMTS), Wireless Broadband (Wi-bro) and Bluetooth wireless applications. In addition, a circular patch antenna comprising of two patches i.e., one inside and the other outside connected using a silicon switch of photoconductive nature is made to get frequency reconfigurability while a hole has been created using optical fiber laser light, which is coupled together with a switch [7]. Now antenna properties would improve once the illumination or power is increased from laser light [8]. Reconfigurability using Radio frequency microelectromechanical system (RF-MEMS) switches was reported in [9–11]. PIN diodes are operated on the quad-band mobile phones performing operations at UMTS, Global System for Mobile Communications (GSM)-1900, GSM-1800, and GSM-800 [12]. Inductors (chip-based) are used with PIN diodes for UMTS and Wireless Local Area Network (WLAN) applications [13]. Three PIN diodes are utilised in the antenna; their control is managed by a Direct Current (DC) line to achieve frequency reconfiguration for Fourth Generation (4G) Long Term Evolution (LTE) and GSM wireless services [14]. Reconfigurable antenna for space applications was reported in [15]. Researchers investigated the use of frequency reconfigurability by connecting three parasitic patches with a main radiating patch through PIN diodes [16]. Hence seven different operating bands are obtained by changing surface current distribution with the help of switch states. To achieve overall effective performance researchers have employed different techniques within radiating structure in past decade, in unique shapes, i.e., Dual Y-Shaped [17], C-Shaped [18], F-Shaped [19] and 7-Shaped [20].
A slot antenna using PIN diodes to achieve frequency reconfiguration for four bands was implemented in [21]. A frequency reconfigurable antenna was designed for 2.2 to 6 GHz with switching performed up to 10 frequency bands [22]. A Bow-tie antenna was proposed with frequency reconfigurable characteristics for wireless applications [23,24]. Reconfigurability achieved using folded slots with PIN diode was reported using inkjet-printing technology [25]. Reference [26] depicts the reconfigurable patch frequency design for multiband applications using RF PIN diodes while a hexagonal-shaped printed reconfigurable antenna is being designed in [27] covering four frequency bands in sub 6 GHz range. In [28], Three-pin diodes were used to achieve the successful design of a frequency reconfigurable antenna, having operational bands within the range of 1.8 to 5.54 GHz. The works [29–31] report a frequency reconfigurable antenna that uses three switches, to cover the frequencies within the sub-6 GHz 5G bands, achieving peak gains up to 3.6 dBi. A frequency reconfigurable monopole antenna is designed in [32], for 5.6 and 2.4 GHz Unlicensed Industrial, Scientific, and Medical (ISM) bands, depending upon the state of the switch.
In the literature, it can be observed that researchers have used different reconfiguration approaches. Electrical reconfiguration techniques are easy to implement and have a comparatively low cost; however, they require a biasing circuit, which complicates the overall antenna structure. Optical reconfiguration does not require biasing lines, but it is lossy in nature and has a complex activation mechanism. Mechanical reconfiguration does not require a biasing system or active elements, but the antennas are comparatively slow in response and need a dedicated power source. Smart material-based reconfiguration, albeit lighter in weight, leads to lower efficiency and limited applications [33].
Antenna size is one of the key parameters for feasible integration in electronic devices. For multiband operation, the size of the antenna becomes an issue. Researchers have designed various antennas of different sizes to achieve optimum frequency bands for wireless communication [34,35]. Large antennas need more space requirements, which might not be desirable in most wireless systems. Hence, a compact antenna that can support multiple applications and frequency bands in a handheld device, with a minimum number of switches, while offering higher gain and supporting larger bandwidths than its competitors, could address the requirements and any potential shortcomings from previous designs. In addition, as discussed above, considering the overall advantages of electrical reconfiguration techniques, specifically of PIN diodes, and the disadvantages of other reconfiguration techniques, the PIN diode was selected as the switching technique for the proposed antenna. The above-identified shortcomings acted as the motivation for this work.
In this paper, a novel, Th-shaped, frequency switchable, a monopole antenna, using a PIN diode switch, is proposed. To enhance the performance of the reconfigurable antenna, the truncated ground plane is used, while to reduce its footprint, the antenna size is kept small. To simulate PIN diode behavior in CST Microwave Studio, lumped elements are used. In the switch OFF state, a high resistance value is used to block the flow of current in specific portions of the radiating structure. A minimum value of resistance is used in the switch ON state, to allow full flow of current. The performance of the antenna is evaluated in both these states. These bands supported by the proposed antenna can be utilized for wireless services that include Worldwide Interoperability and Microwave Access (WIMAX), LTE2500, WLAN and various satellite applications.
The rest of the paper is organized as follows: the methodology and design geometry of the reconfigurable planar monopole antenna proposed is explained in Section 2. The Measured and Simulation results are discussed and presented in Section 3. Finally, the findings are concluded in Section 4.
Fig. 1 represents the geometrical construction of a slotted Th-Shaped switchable multi-band monopole antenna for WIMAX, Bluetooth, Wi-Fi, LTE2500 and satellite applications. The antenna is printed on FR-4 substrate, with thickness of 1.6 mm, loss tangent of 0.019 and relative permittivity of 4.5, matched to 50 Ω feed. The front view in Fig. 1a clarifies the top antenna radiating part having a shape that looks like “Th”. There is a slot in the top part of “T” and an embedded switch between “T” and “h”.
Figure 1: Geometry of Th-shaped frequency reconfigurable monopole antenna (a) Front view (b) Back view
Tab. 2 summarizes the dimensional parameters of the proposed antenna. The theory of the Transmission Line Model (TLM) is used to deduce the effective resonant lengths for the intended [36]. The proposed resonant length is written in terms of guided wavelength
A Pin diode is used for switching, which works as adjustable resistor in the radio frequency range. Tunability is obtained with the help of a pin diode which, like any other diode, acts as an open and short circuit under certain operating conditions. When the pin diode is in ON state, the effective length of the current path increases, leading to the antenna resonating within lower frequency bands. On the other hand, when the pin diode is in its OFF state, the effective length of the current path becomes shortened. This leads to the antenna resonating at comparatively higher frequency bands, as shown in Figs. 5a and 5b. Thus, frequency reconfigurability is achieved by varying the effective resonant lengths.
Fig. 2 illustrates the model of the equivalent circuit and pin diode model. In the OFF state, it behaves like a RLC equivalent circuit, wherein an inductor “L” is in series with parallel combination of a capacitor “C” and a high-value resistor “RH”. On the other hand, in the ON state, it acts as RL series circuit with an inductor “L” and low-value resistor “RL”. In this paper, SMP-1345079LF model pin diode is used. The pin diode can be designed from its datasheet in CST Microwave Studio as L = 0.7 nH, RL = 1.5 Ω and C = 0.15 pF. Fig. 3 shows the prototype of the fabricated antenna as well as the biasing circuit used to control the operation of the Pin diode switch.
Figure 2: Equivalent circuit model of PIN diode: (a) ON state; (b) OFF state
Figure 3: Photographs of fabricated model with biasing setup for PIN diode switch
The designed procedure and simulations are performed on the CST Microwave Studio (CST MWS). A waveguide port with the optimum size is used for excitation. The parameters i.e., gain, reflection coefficient, E-field and H-field are analyzed using optimum boundary conditions and field monitors in the CST microwave studio.
The radiation patterns of the proposed antenna is measured in the set up as shown in Fig. 4. During the measurement process, a horn antenna is used as a transmitting antenna, while the proposed antenna as a receiving antenna. The receiving antenna is placed horizontally at the top of the turntable. In order to provide a reflection free environment, the walls of anechoic chamber were covered with the radio frequency absorber. The data were taken from anechoic chamber by exciting the port.
Figure 4: Anechoic chamber set up for radiation pattern measurement
3.1 Impedance Bandwidth and Reflection Coefficient
The simulation-based reflection coefficient of the proposed antenna is analyzed using CST Microwave Studio whereas measured results are obtained by utilizing a Vector Network Analyzer (VNA) and anechoic chamber facility. As per simulation, the Th-Shaped antenna shows tri-band behavior in the switch ON state, operating within 2007–2501 MHz, 3660–3983 MHz and 9341–1046 MHz bands, as shown in Fig. 5. The measured results in the switch ON state show dual-band characteristics, one larger band (due to a combination of first and second bands), having an operating range of 1085–4145 MHz and the other band of 8735–1009 MHz Furthermore, the proposed antenna shows dual-band characteristics when the Switch is in the OFF state. According to the simulation results, the operating frequency ranges are 2577–3280 MHz and 9379–1033 MHz while the switch is in the OFF state. When the measurements were made through the Vector Network analyzer, a dual-band characteristic is realized within operating frequency ranges 2700–3805 MHz and 8990–1043 MHz bands. Hence, simulated and measured results show similar operating frequency ranges in both states of the switch, with a slight shift. The reflection coefficient of the slotted, Th-Shaped frequency reconfigurable multiband monopole is shown in Fig. 5, while switching modes and dual-band characteristics are summarized in Tab. 3.
Figure 5: Reflection coefficient as a function of frequency (a) ON-state of switch (b) OFF-state of the switch
3.2 Far-field Radiation Pattern
Gain patterns of the proposed antenna structure are analyzed in both the ON and OFF states of the switch. The h-plane pattern reveals that the proposed structure is radiating in all directions. At an angle of 90° in the E-plane pattern, nulls are observed. E-Plane Gain patterns of the proposed structure, in both the ON and OFF states of the switch, for the respective resonant frequencies, are shown in Figs. 6–9 show the H-plane gain patterns in both states of the switch.
Figure 6: Comparison of simulated and measured gain (E-Plane) in switch ON-mode
Figure 7: Comparison of simulated and measured gain (E-Plane) in switch OFF-mode
Figure 8: Comparison of simulated and measured gain (H-Plane) in switch ON-mode
Figure 9: Comparison of simulated and measured gain (H-Plane) in switch OFF-mode
While the switch is in ON mode, the values of gains are 1.39, 1.61 and 3.31 dBi at 2.19, 3.81 and 9.72 GHz respectively. In contrast, when the switch is in the OFF state, the resonant frequency shifts to dual-band operation i.e., 2.90 and 9.79 GHz, with respective gain values of 1.22 and 1.83 dBi.
The surface current distribution over the radiating structure is investigated for both states of the switch (ON/OFF). The surface current contributions/distributions, in both the ON and OFF states, are shown in Figs. 10 and 11 respectively.
Figure 10: Surface currents in switch ON-mode (a) 2.19 GHz (b) 3.81 GHz (c) 9.72 GHz
Figure 11: Surface currents in switch OFF-mode (a) 2.90 GHz (b) 9.79 GHz
In the Switch ON state, it is observed that the whole part of the central/main radiating structure, including “T” portion, is contributing in radiating at 2.19 GHz. At 3.81 GHz, the central part of “h” portion and some positions of the main radiating structure are involved in radiation. It is also observed that some specific parts of the whole structure are contributing to radiation at resonant frequency of 9.72 GHz.
In the Switch OFF state, the “T” part is no more responsible for radiation and a portion of the radiator below the switch radiates at frequencies 2.90 GHz and at 9.79 GHz.
Antenna size is one of the key parameters in designing, fabricating, and integrating in an electronic device. Researchers have designed various antennas of different sizes to optimize them for wireless communication. Large size antennas need more space requirements which might not be desirable in most wireless systems. The proposed frequency reconfigurable multi-band monopole antenna is miniaturized (40 × 20 × 1.6 mm3) to occupy less space. The addition of reconfigurability enhances its performance, with a wide frequency range to support multiple wireless services i.e., UMTS, Wi-Fi, Bluetooth, WiMAX, LTE2500 and X-band satellite applications.
The overall summary of the performance of our proposed Th-Shaped frequency reconfigurable monopole structure is given in Tab. 5.
3.4 Comparison with Relevant Work
The comparison of this research work with the existing relevant state of the art works is listed in Tab. 4. It is obvious that our proposed model has a very small size as compared to other works, and it can operate at five different wireless bands with acceptable directivity, gain, and bandwidth performance. Even though the PIN diode technique requires a biasing line, we achieved reconfiguration using only one PIN diode.
A Th-Shaped, planar monopole, multi-band, frequency reconfigurable antenna is proposed for UMTS, LTE2500, 5G, Wi-Fi, WIMAX, Bluetooth and satellite applications is proposed in this work. The discrete switch is used to enable frequency reconfigurability to enable its operation on various wireless services depending upon the state of the switch (ON/OFF). The antenna reveals triple-band nature and covers a range of frequencies (2007–2501 MHz), (3660–3983 MHz) and (9341–1046 MHz) in the ON state of the switch. However, in this state, the third frequency band (9341–1046 MHz) is the third harmonic resonance of the second band (3660–3983 MHz) with satisfactory radiation properties. Similarly, dual-band characteristics with operating frequency ranges (2577–3280 MHz) and (9379–1033 MHz) are observed in the OFF state of the switch. In the OFF state, the second band is the third harmonic resonance of the 2577–3280 MHz frequency band with optimum radiation characteristics. The proposed antenna is quite small however it has radiation efficiency of up to 82%. The nearly Omnidirectional radiation pattern of the proposed antenna within the far-field increases its applicability for short-range broadcast applications. The antenna covers a specific range of frequencies used in different wireless services and can be a prospective candidate to be used in devices that include laptops, smartwatches, mobile phones, and autonomous vehicles.
Funding Statement: The authors acknowledge the support from the Deanship of Scientific Research, Najran University. Kingdom of Saudi Arabia, for funding this work under the Research Collaborations funding program Grant Code Number (NU/RC/SERC//11/5).
Conflicts of Interest: The authors declare that they have no conflicts of interest to report regarding the present study.
References
1. X. Meng, “Small-size eight-band frequency reconfigurable antenna loading a mems switch for mobile handset applications,” International Journal of Antennas and Propagation, vol. 2, no. 1, pp. 1–6, 2014. [Google Scholar]
2. A. Roy, S. Anand, P. K. Choudhury, P. P. Sarkar and S. Bhunia, “Compact multi-frequency patch antenna with spur-lines for WLAN/WiMAX applications,” International Journal of Electronics & Communication Technology, vol. 5, no. 2, pp. 84–86, 2014. [Google Scholar]
3. M. Choi, H. Wi, B. Mun, Y. Yoon, H. Lee et al., “A compact frequency reconfigurable antenna for lte mobile handset applications,” International Journal of Antennas and Propagation, vol. 1, no. 1, pp. 1–10, 2015. [Google Scholar]
4. D. Rodrigo, B. A. Cetiner and L. Jofre, “Frequency radiation pattern and polarization reconfigurable antenna using a parasitic pixel layer,” IEEE Transactions on Antennas and Propagation, vol. 62, no. 6, pp. 3422–3427, 2014. [Google Scholar]
5. S. A. A. Shah, M. F. Khan, S. Ullah, A. Basir, U. Ali et al., “Design and measurement of planar monopole antennas for multi-band wireless applications,” IETE Journal of Research, vol. 6, no. 3, pp. 194–204, 2017. [Google Scholar]
6. Y. Chen, L. Ye, J. Zhuo, Y. Liu, L. Zhang et al., “Frequency reconfigurable circular patch antenna with an arc-shaped slot ground controlled by pin diodes,” International Journal of Antennas and Propagation, vol. 1, no. 1, pp. 1–7, 2017. [Google Scholar]
7. Y. Tawk, A. R. Albrecht, S. Hemmady, G. Balakrishnan and C. G. Christodoulou, “Optically pumped frequency reconfigurable antenna design,” IEEE Antennas and Wireless Propagation Letters, vol. 9, no. 2, pp. 280–283, 2010. [Google Scholar]
8. A. Andy, P. Alizadeh, K. Z. Rajab, T. Kreouzis and R. Donnan, “An optically-switched frequency reconfigurable antenna for cognitive radio applications,” in 10th European Conf. on Antennas and Propagation (EuCAP), Davos, Switzerland, pp. 1–4, 2016. [Google Scholar]
9. K. Topalli, E. Erdil, O. A. Civi, S. Demir, S. Koc et al., “Tunable dual-frequency RF MEMS rectangular slot ring antenna,” Sensors and Actuators A: Physical, vol. 156, no. 2, pp. 373–380, 2009. [Google Scholar]
10. A. Grau, J. Romeu, M. J. Lee, S. Blanch, L. Jofre et al., “A dual-linearly-polarized mems-reconfigurable antenna for narrowband mimo communication systems,” IEEE Transactions on Antennas and Propagation, vol. 58, no. 1, pp. 4–17, 2010. [Google Scholar]
11. D. A. Anagnostou, G. Zheng, L. Feldner, M. T. Chryssomallis, J. C. Lyke et al., “Silicon-etched re-configurable self-similar antenna with RF-MEMS switches,” IEEE Antennas and Propagation Society Symposium, vol. 2, no. 1, pp. 1804–1807, 2004. [Google Scholar]
12. Y. K. Park and Y. Sung, “A reconfigurable antenna for quad-band mobile handset applications,” IEEE Transactions on Antennas and Propagation, vol. 60, no. 6, pp. 3003–3006, 2012. [Google Scholar]
13. R. Gonçalves, P. Pinho and N. B. Carvalho, “Compact frequency reconfigurable, printed mono.pole antenna,” International Journal of Antennas and Propagation, vol. 2, no. 1, pp. 1–6, 2012. [Google Scholar]
14. H. T. Chattha, M. Hanif, X. Yang, Q. H. Abbasi and I. E. Rana, “Frequency reconfigurable patch antenna for 4G lte applications,” Progress in Electromagnetics Research M, vol. 69, no. 1, pp. 1–13, 2018. [Google Scholar]
15. C. G. Christodoulou, Y. Tawk, S. A. Lane and S. R. Erwin, “Reconfigurable antennas for wireless and space applications,” Proceedings of the IEEE, vol. 100, no. 7, pp. 2250–2261, 2012. [Google Scholar]
16. R. Baruah and N. S. Bhattacharyya, “A frequency reconfigurable antenna with consistent radiation characteristics,” in Int. Conf. on Innovations in Electronics, Signal Processing and Communication (IESC), Shillong, India, pp. 177–180, 2017. [Google Scholar]
17. H. Zhai, Q. Gao, Z. Ma and C. Liang, “Dual y-shaped monopole antenna for dual-band WLAN/WiMAX operations,” International Journal of Antennas and Propagation, vol. 2, no. 2, pp. 1–7, 2014. [Google Scholar]
18. F. Li, L. S. Ren, G. Zhao and Y. C. Jiao, “Compact triple-band monopole antenna with C-shaped and S-shaped meander strips for WLAN/WiMAX applications,” Progress in Electromagnetics Research, vol. 15, no. 2, pp. 107–116, 2010. [Google Scholar]
19. J. R. Panda and R. S. Kshetrimayum, “A printed F-shaped dual-band monopole antenna for RFID and WLAN applications,” in Int. Conf. on Computer and Communication Technology (ICCCT), Allahabad, India, pp. 789–791, 2010. [Google Scholar]
20. S. A. A. Shah, M. F. Khan, S. Ullah and J. A. Flint, “Design of a multi-band frequency reconfigurable planar monopole antenna using truncated ground plane for Wi-Fi, WLAN and WiMAX applications,” in Int. Conf. on Open Source Systems Technologies, Lahore, Pakistan, pp. 151–155, 2014. [Google Scholar]
21. D. Peroulis, K. Sarabandi and L. P. B. Katehi, “Design of reconfigurable slot antennas,” IEEE Transactions on Antennas and Propagation, vol. 53, no. 2, pp. 645–654, 2005. [Google Scholar]
22. Y. I. Abdulraheem, G. A. Oguntala, A. S. Abdullah, H. J. Mohammed, R. A. Ali et al., “Design of frequency reconfigurable multiband compact antenna using two PIN diodes for WLAN/WiMAX applications,” IET Microwaves, Antennas & Propagation, vol. 11, no. 8, pp. 1098–1105, 2017. [Google Scholar]
23. T. Li, H. Zhai, X. Wang, L. Li and C. Liang, “Frequency-reconfigurable bow-tie antenna for bluetooth, WIMAX, and WLAN applications,” IEEE Antennas and Wireless Propagation Letters, vol. 14, no. 1, pp. 171–174, 2015. [Google Scholar]
24. Y. Chen, L. Ye, J. Zhuo, Y. Liu, L. Zhang et al., “Frequency reconfigurable circular patch antenna with an arc-shaped slot ground controlled by pin diodes,” International Journal of Antennas and Propagation, vol. 2, no. 3, pp. 1–6, 2017. [Google Scholar]
25. S. A. Saeed, C. A. Balanis and C. R. Birtcher, “Inkjet-printed flexible reconfigurable antenna for conformal WLAN/WiMAX wireless devices,” IEEE Antennas and Wireless Propagation Letters, vol. 15, no. 1, pp. 1979–1982, 2016. [Google Scholar]
26. S. Saikia, P. Dutta and K. Borah, “Design of a frequency reconfigurable microstrip patch antenna for multiband applications,” in 5th Int. Conf. on Computers & Management Skills (ICCM 2019), Arunachal Pradesh, India, pp. 15–16, 2019. [Google Scholar]
27. S. Ullah, I. Ahmad, Y. Raheem, S. Ullah, T. Ahmad et al., “Hexagonal shaped cpw feed based frequency reconfigurable antenna for wlan and sub-6 GHz 5G applications,” in Int. Conf. on Emerging Trends in Smart Technologies (ICETST), Karachi, Pakistan, pp. 26–27, 2020. [Google Scholar]
28. A. Iqbal, A. Smida, L. F. Abdulrazak, O. A. Saraereh, N. K. Mallat et al., “Low-profile frequency reconfigurable antenna for heterogeneous wireless systems,” Electronics, vol. 8, no. 9, pp. 1–11, 2019. [Google Scholar]
29. I. A. Shah, S. Hayat, A. Basir, M. Zada, S. A. A. Shah et al., “Design and analysis of a hexa-band frequency reconfigurable antenna for wireless communication,” AEU-International Journal of Electronics and Communications, vol. 98, no. 1, pp. 80–88, 2019. [Google Scholar]
30. H. Dildar, F. Althobiani, I. Ahmad, W. U. R. Khan, S. Ullah et al., “Design and experimental analysis of multiband frequency reconfigurable antenna for 5G and sub-6 GHz wireless communication,” Micromachines, vol. 12, no. 1, pp. 1–15, 2021. [Google Scholar]
31. M. T. Khan, M. T. Jilani, A. M. Khan, F. Hafeez and A. K. Memon, “Effects of defected ground structure slot tuning on frequency and circuit parameters of bandpass filter,” Journal of Optoelectronics and Advanced Materials, vol. 20, no. 9–10, pp. 479–485, 2018. [Google Scholar]
32. A. L. Muttairy and M. J. Farhan, “Frequency reconfigurable mono.pole antenna with harmonic suppression for IoT applications,” Telkomnika, vol. 18, no. 1, pp. 10–18, 2020. [Google Scholar]
33. N. P. Ojaroudi, H. B. Jahanbakhsh, Y. I. Al-Yasir, A. M. Abdulkhaleq and R. A. Abd-Alhameed, “Reconfigurable antennas: Switching techniques-A survey,” Electronics, vol. 9, no. 2, pp. 1–14, 2020. [Google Scholar]
34. S. Ullah, S. Hayat, A. Umar, U. Ali, F. A. Tahir et al., “Design fabrication and measurement of triple band frequency reconfigurable antennas for portable wireless communications,” AEU-International Journal of Electronics and Communications, vol. 81, no. 2, pp. 236–242, 2017. [Google Scholar]
35. I. A. Shah, S. Hayat, I. Khan, I. Alam, S. Ullah et al., “A compact, tri-band and 9-shape reconfigurable antenna for WIFI, wimax, and wlan applications,” International Journal of Wireless and Microwave Technologies (IJWMT), vol. 6, no. 5, pp. 45–53, 2016. [Google Scholar]
36. S. Rahman, R. Xin-cheng, A. Ahsan, M. Irfan, M. Abdullah et al., “Nature inspired mimo antenna system for future MMwave technologies,” Micromachines, vol. 11, no. 12, pp. 1–11, 2020. [Google Scholar]
Cite This Article
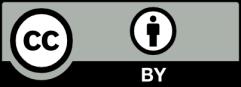