Open Access
REVIEW
Transcatheter Pulmonary Valve Implantation: A State of the Art Review
1 Paediatric Cardiology Unit, Department of Women’s and Child’s Health, University of Padua, Padua, 35129, Italy
2 PhD School in Developmental Medicine and Health Planning Sciences, University of Padua, Padua, 35129, Italy
* Corresponding Author: Biagio Castaldi. Email:
Congenital Heart Disease 2024, 19(5), 513-533. https://doi.org/10.32604/chd.2025.058053
Received 03 September 2024; Accepted 17 December 2024; Issue published 31 December 2024
Abstract
Congenital heart disease (CHD) affects about 1% of live births. Among them, about 20% will undergo one or more surgical or percutaneous maneuvers on the right ventricle outflow tract or pulmonary valve. Transcatheter pulmonary valve implantation is a recently available less invasive alternative to surgery for treatment of right ventricular outflow tract dysfunction. Thus, residual dysfunction can be treated early and with a lower risk profile. This narrative review aimed to describe the state of the art of percutaneous pulmonary valve implantation.Keywords
Congenital heart disease (CHD) affects approximately 1% of live births [1]. Among these, about 20% will undergo one or more surgical or percutaneous procedures involving the right ventricle outflow tract (RVOT) or pulmonary valve (PV). The most common CHDs involved are Tetralogy of Fallot (ToF), pulmonary valve stenosis (PVS), pulmonary atresia with ventricular septal defect (PA-VSD), or without ventricular septal defect (PA-IVS), truncus arteriosus, some subtypes of double outlet right ventricle (DORV), and transposition of great arteries (TGA). Additionally, some left heart CHDs, where the pulmonary valve might be used as an autograft (like the Ross procedure and further variants) might also be included.
The surgical approach to these pathologies has undergone significant changes over the past four decades Until the 1980s pulmonary valvectomy (with or without RVOT enlargement using a trans-annular patch) was the most common procedure. Although this approach was effective in the short and mid-term follow-up, patients developed progressive RV dysfunction in adulthood. Thus, surgical PV repair or replacement became part of the treatment strategy of these patients [2].
The long-term durability of surgical correction is influenced by several factors, including the patient’s age at the time of surgery, the underlying congenital heart defect the severity of PV-RVOT stenosis and/or dysplasia, the type of surgical approach, the presence of infections, and other patient-specific variables, some of which remain poorly understood. Despite advancements in surgical techniques, the durability and functional outcomes of surgically manipulated pulmonary valves are generally inferior to those of native PV. The need for multiple re-interventions is common, the type of RVOT dysfunction (stenosis, regurgitation, or mixed diseases) is closely related to the initial surgical procedure: regurgitation and RV volume overload are the most common sequelae following RVOT patching, monocusp patch and PV valvectomy or commissurotomy; whereas stenosis and increased RV afterload are more frequently observed with valved conduit and biologic prosthetic valves [3].
Transcatheter pulmonary valve implantation (TPVI) is a relatively recent less invasive alternative to surgery for the treatment of RVOT/PV dysfunction. This percutaneous technique allows for the early treatment of residual dysfunction with a lower risk profile. This narrative review aims to provide an overview of the current state of the art of percutaneous pulmonary valve replacement.
The history of TPVI dates back to 2000 when Bonhoeffer et al. first reported a case series of TPVI in a lamb model [4]. Later that year, the same group published the first-in-human” TPVI in a 12-year-old boy TOF with severe conduit stenosis [5]. This landmark procedure marked the introduction of Melody®® Valve (Medtronic, Inc.; Minneapolis, MN, USA) which became the first commercially available percutaneous valve. The favorable outcomes associated with the Melody valve played a pivotal role in advancing the development of percutaneous aortic valve replacement techniques. Following minor improvement, the Melody valve received the CE Marking and Health Canada approval, in 2006 and then the U.S. Food and Drug Administration (FDA) approval in 2010 [6].
Since its initial clinical application, early trials conducted both in Europe and the United States demonstrated substantial reductions in conduit pressure gradients, with an immediate restoration of valve competence [7,8]. Among the most frequently reported post-implant complications were stent fractures and recurrent stenosis due to the “hammock effect”. The stent fractures were primarily a result of high radial stress from the stenotic conduit, residual RVOT muscularization, and the pulsatile force exerted by the aortic beat on the stent. These fractures were markedly reduced through systematic conduit rehabilitation and pre-stenting before Melody valve implantation. With this technique, the two stents increased exponentially the resistance to fractures. The “hammock effect” which arises from blood accumulation between the stent and the conduit, leads to stenosis in the mid-segment of the valve. To prevent this, the conduit was sutured to the stent not only at the ends but also for the length of the stent. In patients showing the hammock effect, a valve-in-valve procedure was consistently effective [9].
Concurrently the same research group began exploring devices for percutaneous aortic valve interventions [10]. In 2002, Alain Cribier performed the first human implantation of a custom-made device in aortic position [11]. The evolution of that valved device led to the development of the commercially available Edwards SAPIEN transcatheter heart valve (THV) (Edwards Lifesciences LLC, Irvine, CA, USA [12]. In 2008, the COMPASSION trial was initiated to assess the outcomes of the Sapien valve implanted in the pulmonary position, demonstrating an excellent safety/efficacy profile [13,14].
Driven by marketing factors, the portfolio of aortic valves has expanded exponentially since 2010. For TPVI, the choice of devices was initially limited to the Melody valve (for annular diameters between 16 and 22 mm), and Sapien valve (for diameters between 23 and 29 mm). These devices underwent incremental technical improvements, including enhancements to the valve, delivery sheath, and specific maneuvers to facilitate valve accommodation [15,16].
In other words, following the initial experience with Melody valve, the main Companies were mainly focused on developing devices to implant in the aortic position rather than developing devices for the rarer CHDs. Consequently, pediatric cardiologists were left to adapt a device originally designed and conceived for aortic valve replacement, by modifying valve orientation for the crimping on the balloon.
After 15 years without significant innovations, a self-expandable device (previously used for aortic valve replacement since 2006) became available also for pulmonary position. These included the Venus-P-Valve™ in Europe, the Alterra adaptive pre-stent™, and Harmony®® trans-catheter pulmonary valve in the USA; and the Pulsta trans-catheter pulmonary valve®® in Asia. The availability of self-expandable valves significantly expanded the pool of eligible patients for TPVR, particularly those with native or dilated RVOTs.
Balloon-Expandable Valves
Balloon expandable valves were the first devices widely used for TPVI. Over the past 20 years, thousands of devices have been successfully implanted, demonstrating an excellent safety and efficacy profile. These valves are mounted on a stent with high radial force, which allows them to be used in both stenotic and regurgitant lesions. Typically, pre-stenting is performed before device deployment, to stabilize the landing zone, address multi-site stenosis, and minimize the risk of stent fractures and device embolization. Currently, two balloon expandable valves are available for TPVI; the Melody valve and the S3 Sapien valve (Table 1).
Melody®® TPV (Medtronic Inc., Minneapolis, MN, USA
European CE mark obtained in 2006, US FDA mark: 2010
Melody was the first percutaneous valve implanted in humans. Currently available the Melody®® TPV valve consists of a tri-leaflet bovine jugular valve segment (16 or 18 mm), which is first fixed in glutaraldehyde under pressure and then without pressure. The valve is sutured to a 34 mm CP stent (NuMED, Inc., Hopkinton, New York, NY, USA) frame by frame, using a blue suture at the distal end of the valve and a white suture on the proximal end of the device. This color coding assist in ensuring correct valve orientation during crimping on the dedicated delivery system, which has a blue carrot tip on the end and a white shaft (“blue with blue and white with white”) [17].
The device is available in two sizes, 20 and 22 mm. The stent used is identical in both cases; the difference lies in the conduit diameter. The smaller 16 mm valve is designed to accommodate an inner diameter of up to 20 mm, while the 18 mm valve can be dilated to a diameter of 22–24 mm [17]. The valve can be crimped on an 18, 20, or 22 mm Ensamble delivery system, which consists of a nose tip, that optimizes system trackability; a BiB balloon, for valve dilation; and a sheath, with a larger distal portion to cover the valve during placement maneuvers. The valve can be used also for smaller diameters, by using smaller balloon catheters and long sheaths for delivery in RVOT.
Once the valve is in position the sheath is retracted to uncover the valve. Deployment is completed by sequentially inflating the inner balloon first, followed by inflation of the outer balloon. The ensemble is 22 Fr large. It can be effectively used bare in the vein, over a stiff exchange guidewire (Fig. 1). Once the valve is delivered, an 18 Fr sheath can replace it without risk of local bleeding. Alternatively, it can be delivered through a 24 or 26 Fr Gore®® Dry Seal long-sheath.
Figure 1: Melody valve implantation—step-by-step procedure. (A) Conduit ballon interrogation, aortic root, and non-selective coronary interrogation by using a 22 mm × 40 mm atlas gold balloon; (B) presenting of RVOT with a CP stent mounted on a 22 mm × 40 mm atlas gold balloon; (C) 18–10 Melody valve deployment, mounted on a 22 mm ensemble; (D) aortic angiography after valve deployment, demonstrating no regurgitation; (E) selective coronary angiography after valve deployment, no coronary compression; (F) pulmonary angiography demonstrating no regurgitation or paravalvular leak
Melody®® TPV is currently approved for the treatment of at least moderate dysfunction (stenosis and/or regurgitation) of RV-PA surgical conduit or biologic prosthetic valves. Additionally, it might also be used safely in native dysfunctional RVOT if a landing zone with a diameter of less than 22 mm of diameter can be achieved [6]. Pre-stenting of the RVOT is strongly recommended to reduce the risk of valve dysfunction due to stent fractures.
SAPIEN XT THV and SAPIEN 3 THV (Ewards Lifesciences LLC, Irvine, CA, USA)
European CE mark obtained in 2016 (SAPIEN XT), 2021 (SAPIEN 3 THV)
US FDA mark in 2016 (SAPIEN XT), 2020 (SAPIEN 3 THV)
Since the first implant in 2002, Sapien valves were progressively improved, increasing the size availability (actually 20-23-26-29 mm), modifying the stent material (from steel to chrome-cobalt) lengthening the stent and reducing the size of the delivery sheath (from 24 Fr to 16 Fr). However, these improvements were initially tailored to the aortic valve anatomy and were later adapted for TPVI. The valve is handmade sutured in the stent, by using pericardial bovine tissue. Sapien XT received its first CE mark approval for TPVI in 2016; and Sapien S3 in 2020. Sapien Ultra is still not on-label for TPVI. Sapien XT is currently out of production, thus, Sapien S3 is the only version available for TPVI [18,19].
Sapien S3 valves can range from diameters between 20–29 mm. Compared to the Melody valve, it is designed for larger diameters, and it is significantly shorter in length. Compared to XT, the S3 is longer due to the addition of a proximal skirt which helps to prevent potential para-valvar leaks. In addition, it can be used in a DrySeal long sheath. Therefore, S3 might be implanted in RVOT without pre-stenting in selected cases [20]. However, pre-stenting is still performed in approximately 80% of cases before S3 implantation Unlike the Melody valve, the device size is referred to as the external diameter. Additionally, S3 can also be over-dilated to fit a 1–2 mm diameter larger than the nominal size, still preserving leaflet coaptation, some elastic recoil might be observed at larger diameters [21].
Sapien valves are crimped using a dedicated system and should be used by certified operators. The valve is mounted outside of the balloon, to reduce the whole delivery sheath size. After introduction via the femoral access, the balloon (which features both proximal and distal radio-opaque marker) is advanced through the RVOT and aligned to the stent. The valve is delivered within the sheath up to the deployment site. To enhance the system trackability and to reduce the risk of iatrogenic tricuspid valve injury, the valve is advanced in a 26 Fr Dry-Seal long-sheath (WL Gore, Flagstaff, AZ, USA). Once the valve reaches the RVOT, the sheath is retracted to expose the valve. The ensemble can be angulated using a flex wheel to facilitate the crossing of kinking or acute angles but before deployment, the system should be straightened again and the valve fully uncovered before balloon inflation (Fig. 2).
Figure 2: Sapien S3 valve implantation. (A) RVOT angiography demonstrating landing zone on the distal trunk, 23 mm large (B). (C) Aortic root angiography during balloon interrogation, no significant aortic root distortion or coronary compression were detected. (D) Pre-stenting by using a 57 mm Andra stent XL, was implanted from the left pulmonary artery, intentionally jailing the right pulmonary artery, by using a 18 mm × 60 mm Atlas Gold Balloon. The stent was dilated proximally by using a 26 × 40 atlas gold balloon; Finally, a 29 mm Sapien S3 valve was deployed (E). (F) Final aortic root angiography after valve deployment demonstrated no complication on the aorta and coronary tree
Sapien valves can be used both in native RVOT, and RV-PA conduits and for valve-in-valve procedures [22].
Self-Expandable Valves
Although self-expandable valves were available in the early era of percutaneous aortic valve replacement, their use in the pulmonary position represents an innovation of this decade. Self-expandable valves have expanded the procedural feasibility, particularly for large native or surgically patched RVOT [23]. While balloon-expandable valves have a cylindrical design, self-expandable valves feature a dual funnel shape. The stent is made of Nitinol, which facilitates easier crimping and subsequently adaptation to the anatomy of dilated native RVOT. Once deployed, these valves often adopt an elliptic shape, owing to their low radial force. On the other hand, self-expandable valves are contraindicated in cases of stenosis and should only be used in patients with severe pulmonary valve regurgitation [19]. To date, the prostheses available on the market are Venus Valve, Harmony Valve, Alterra Adaptive Prestent, and Pulsta Valve (Table 2).
Venus Pulmonary Valve (Venus Medtech, Shanghai, China)
European CE mark in 2022, US FDA mark: under investigation
The Venus Pulmonary Valve (Venus Medtech, Shanghai, China) consists of a self-expandable Nitinol multi-level support frame with a tri-leaflet porcine pericardial tissue valve sutured with PTFE sutures to the frame. The valve is constructed with porcine pericardial tissue which is fabricated from three equal sections of buffered glutaraldehyde to fully crosslink the tissue while preserving its flexibility and strength [24].
Anchorage is achieved through a flared, uncovered distal end, which ensures adequate perfusion of pulmonary artery branches in the event of jailing. The valve is typically deployed in or just below pulmonary artery bifurcation, with the symmetric proximal end, covered to close possible paravalvular leaks. The proximal landing zone should ideally be positioned near (or better above) the native (or former) pulmonary valve annulus. The valve is available in five sizes, corresponding to the smallest (valved) stent diameter, ranging from 28 to 36 mm in 2 mm increments. The edges of the device are about 10 mm larger than the valve diameter. In addition, there are two lengths available for any diameter, 25, and 30 mm, indicating the length of the central (cylindrical) segment of the device. The cylindrical segment of the valve is marked with six radiopaque markers, to facilitate the deployment maneuvers [25]. The funnel-shaped edges are both 15 mm in height. The valve must be loaded into the delivery system using a dedicated crimping system. To improve the ductility of the device, it should be manipulated in an iced sterile saline solution, to facilitate crimping maneuvers. The valve is secured to three hooks located on the proximal edge of the stent similar to the S3 Sapien valve, this valve is advanced in a 26 Fr Gore®® DrySeal long-sheath (Fig. 3). Once deployment is started, the valve can be retrieved when up to 75% of the device is deployed.
Figure 3: Venus P valve implantation. Virtual reality simulation of device implantation based on CT, in right anterior oblique (A) and lateral (B) views. (C) RVOT angiography demonstrating a narrowest diameter of 27.5 mm. (D) A 34 mm × 25 mm Venus P valve was implanted. (E) Final RVOT angiography demonstrated an effective device function. AO: Aorta, LPA: Left pulmonary artery, RPA: Right pulmonary artery, RV: Right ventricle, RA: Right atrium
Harmony®® TPV (Medtronic Inc., Minneapolis, MN, USA)
European CE mark under investigation to date, US FDA mark: 2021
The Harmony TPV is constructed from a porcine pericardial tissue which is treated with alpha-amino oleic acid anti-mineralization treatment to minimize leaflet calcification. The stent is self-expanding and made in nitinol, with the frame covered in a polyester knit fabric. The valve is available in two sizes: 22 and 25 mm with the diameter referring to the valve size. In both sizes, the central segment is cylindrical and the proximal edge is larger than the proximal one:
-Harmony ®®TPV 22 mm: 41 mm inflow diameter, 32 mm outflow diameter; total length 55 mm
-Harmony®® TPV 25 mm: 54 mm inflow diameter, 43 mm outflow diameter; total length 51 mm
The valve can be easily crimped by the operator. It is secured to the delivery cable through 9 suture loops, which have to be attached to the delivery system by a spiral system. Once loaded the valve, is advanced in a 26 Fr Gore®® DrySeal sheath. Upon reaching the desired landing zone it can be easily deployed. The valve might be potentially retrieved and re-adjusted during the deployment phase if the suture loops are still secured to the delivery system when a DrySeal is used [26].
To date, the Harmony valve is the largest valve available for TPVI. Unlike the Venus Valve, it is fully covered. Thus, the operators should take care to avoid jailing pulmonary branches. On the other hand, the covered distal edge might reduce or abolish paravalvular leaks in huge RVOTs.
The Alterra Adaptive Pre-stent (Edwards Lifesciences, Irvine, California)
European CE mark under investigation to date, US FDA mark: 2021
Alterra Adaptive Pre-stent was designed as an “infundibular reducer” to expand the feasibility of TPVI using Sapien devices by reducing and reconfiguring RVOT morphology, thus creating a favorable landing zone for the SAPIEN 3 THV 29 mm.
The Alterra system is a self-expanding, radiopaque, nitinol frame assembly with a fabric covering of polyethylene terephthalate (PET). It features distinct inflow and outflow ends both flared, the final shape of the device is a double funnel with the diameter of both ends measuring 41 mm. The central landing zone is 27 mm in diameter; the total device length is 48 mm. The inflow section is differentiated from the outflow section by two key features: first the presence of two triangular tabs which are used for attachment to the delivery system, and a circumferential covering of all cells. Like in the Venus-P valve, the distal part of the stent is uncovered, to avoid pulmonary artery branches jailing.
The Alterra Adaptive Pre-stent comes pre-loaded in a 22 Fr dedicated delivery system. The use of a 26 Fr DrySeal to accommodate the whole system in RVOT is strongly suggested. The delivery system is designed to provide slow controlled deployment and allows the operator to recapture two times the pre-stent using a one-handed rotational knob [27]. Once the Alterra device is deployed, a Sapien valve can be used to complete TPVI.
Pulsta Valve
Pulsta Valve (TaeWoong Medical Co, Gimpo, Republic of Korea) is a 3-leaflet decellularized porcine pericardial valve attached to a self-expandable Nitinol stent. The valve’s size refers to the narrowest diameter of the stent, ranging from 18 to 32 mm, with 2 mm increments. The edges are 4 mm larger than the valve size, regardless of the chosen diameter. The total length ranges from 28 and 38 mm depending on the stent diameter. The first row of cells and the two most distal rows are uncovered, while one row of proximal cells and two rows of distal cells are left uncovered to avoid obstructing flow through the pulmonary branches.
The valve can be crimped by the operator itself, without any specific device. It is attached to the delivery system through three hooks. The valve can be retrieved when at least 33% of the device is still in the delivery sheath. To date, the largest experience is limited to Asian countries, because this device is still awaiting CE and FDA approvals [28–30].
4 Imaging for Right Ventricular Outflow Tract (RVOT)
Imaging in RVOT diseases has a double purpose: to assess the impact of the residual defect on heart function (indicating treatment) and to provide detailed anatomical information when treatment is indicated. Several imaging tools are available, each one with tips and tricks [31,32]. A comprehensive understanding of this option is essential to approach these patients and to create an effective diagnostic flowchart [33,34]; Additionally, periodical updates on eligibility criteria should be shared between hub and spoke centers to screen candidates for TPVI [35].
To thoroughly assess RVOT anatomy the experienced cardiologist may therefore choose among different imaging modalities, including echocardiography (Echo), cardiac magnetic resonance imaging (CMR), and computed tomography (CT) [36]. The selection of appropriate imaging techniques depends on the clinical scenario, patients’ characteristics, and the specific information required. A multi-modality imaging approach is generally preferred to overcome the limitations of individual techniques enabling a more comprehensive and reproducible assessment of RVOT anatomy before TPVI. Pre-procedural imaging provides detailed information about TPVI contraindication, especially the relationship between RVOT and coronary artery anatomy [37]. Furthermore, the integration of artificial intelligence (AI) in clinical practice has promoted the evolution of cardiac imaging with accordingly customized treatment strategies for each patient’s cardiac morphology [38]. Computational sciences and automated software can merge information derived from multimodality imaging, providing image segmentation and three-dimensional (3D) reconstruction which facilitate catheter-based interventions and optimize risk assessment during invasive procedures [39].
Echocardiography
The conotruncal region is routinely and longitudinally assessed with color and spectral Doppler Echo. It provides real-time images to assess instantaneously ventricular function, pulmonary valve motion, and blood flow, without safety concerns due to its non-invasive nature.
The widespread availability of Echo makes it particularly well-suited for bedside evaluations in critically ill patients. A poor acoustic window is the most frequent limitation, because of prior sternotomies or RVOT calcifications. However, the assessment of volumes and RV function is now feasible bedside. Thanks to advancements in probes and software technology widened the availability of this new tool. Recent studies demonstrated a good agreement between echo and CMR data [40]. Nevertheless, the acoustic window in these patients might be challenging, due to its peculiar shape, anterior position, and post-surgical scars.
Native pulmonary valve, RVOT conduit, and bio-prosthetic valve stenosis can be effectively evaluated by echo, either for stenosis [41,42] or to assess for regurgitation [43–45].
In conclusion, the Echo remains the first tool to quantify right heart valve dysfunction. The latest 3D probes further enhanced the role of Echo in RV volume quantification. However, once a clinically relevant RVOT dysfunction is suspected, second-level imaging is always recommended.
Cardiac Magnetic Resonance
CMR is a recognized tool for both structural and functional RVOT assessment, spanning from the prenatal period to adulthood [46–50]. The four-dimensional flow CMR (4D-Flow CMR) imaging technique establishes a comprehensive assessment of blood flow in a single free-breathing acquisition, enabling dynamic blood flow visualization and quantification, which is relevant when assessing PR [51–53].
In the case of RVOT obstruction, CMR analyzes the impact of this hemodynamic burden on the right ventricle [54]. It remains the gold standard for RV volumes and ejection fraction assessment, as well as for pulmonary valve regurgitation. Despite its advantages, CMR still relies on the practitioner’s expertise. Operators should be adequately trained to calculate volumes in post-surgical anatomies and to collect helpful and comprehensive RV and RVOT views, including pulmonary branches and aneurysms in the region of interest. Unfortunately, metallic materials like stents, clip devices, or calcifications, might compromise the collection of anatomic details.
Furthermore, the role of CMR in the assessment of coronary arteries before TPVI is still debated. Malone et al. highlighted that CMR is not the dedicated imaging modality to evaluate any potential coronary artery compression after TPVI [37]. Concerns about CMR are its limited availability, high costs, need for experienced cardiologists, and long time needed for a comprehensive evaluation. Additionally, patients implanted with cardiac devices, and suffering from claustrophobia may be not suitable for CMR imaging. Some studies demonstrated potential toxic effects in patients with kidney diseases and brain accumulation in patients undergoing repeated exams [55]. However, a contrast medium is not necessary to follow RV function and volumes and to study RVOT anatomy.
Computed Tomography
Computed tomography (CT) plays a pivotal role in RVOT assessment, providing high spatial resolution images. It is the first-choice technique when there are CMR contraindications or when comprehensive coronary artery assessment is required before performing invasive procedures [31,37]. CT is the current standard for the detection and quantification of calcifications (such as the Agatson score) [56], which is crucial for planning the optimal strategy for TPVI. Moreover, ECG-gated image acquisition minimizes artifacts and further increases spatial resolution. Finally, by using end systolic and end diastolic ECG-gated sequences, it is now possible to add functional data to anatomic data.
In contrast to CMR, CT is much faster and less operator-depending. Thus, it is the preferred imaging tool to plan TPVI. CT scan acquisitions can be easily 3D reconstructed and post-processed. Unlike CMR, which requires dedicated views and slices, CT protocols are simpler and might be demanded by spoke centers, facilitating more efficient pre-operative RVOT assessment.
Ideally, a CT scan for pre-operative RVOT assessment should be performed with ECG gating and recorded at least in two phases: systolic phase (30% of the cardiac cycle) and end-diastolic phase (90% of the cardiac cycle) [57,58]. The systolic phase provides the maximum RVOT diameters and the relationship between RVOT (and any associated calcifications) with the aorta and coronary arteries. The diastolic phase helps assess tissue: a fixed diameter, with tiny differences between systole and diastole, indicates a stiffer landing zone (due to calcium, scars, or prosthetic rings), while larger differences in diameter might indicate a compliant, and distensible tissue, that should be further studied by an invasive balloon sizing [59,60].
Despite the advancements in newer machines that reduce radiation exposure ionizing radiation is the main limitation of CT. Recent papers raised warnings on the extensive use of ionizing radiation and the higher rate of cancer in CHD patients, especially when performed at younger ages [61]. Therefore, its use should be carefully evaluated, because of the low repeatability of this exam. The iodine contrast medium is mandatory for RVOT assessment [57]. It should be carefully used in case of impaired renal function and in case of proven or suspected allergy to iodine contrast medium. Finally, CT cannot assess valvular function, neither for stenosis nor for regurgitation [62].
In pediatric patients, TPVI is performed under general anesthesia. Although adults may sometimes be performed under local anesthesia, the procedure might take longer than expected and be painful, so many centers prefer to perform (at least the interventional maneuvers) in deep sedation or general anesthesia.
The procedure requires a large venous access (22 to 26 Fr) Additionally a second venous sheath and an arterial access should be achieved, rather on the contralateral groin or on the right arm.
For Melody valve, the minimum weight for a percutaneous approach is 20 kg, whereas, for Gore®® 26 Fr DrySeal sheath, patients weighing more than 30 kg are typically preferred. However, most of the procedures are performed on adolescents and adults.
The use of contrast medium should be carefully weighed, particularly due to the repeated administration of both invasive and non-invasive imaging tools. Therefore, the operators should rely upon CT or MRI scans to determine the optimal plane to approach RVOT, minimizing the need for angiographies as much as possible. Once the reference images are obtained, a stiff guidewire should be placed, there are no pre-fixed rules about which guide wire to use, or where to accommodate it. Generally, a Lunderquist or a Back-up Meier 0.035” guidewires are the stiffest ones and most used.
The left pulmonary branch, which is positioned more cranially, usually presents a close angle with the hilum, when the guidewire is positioned distally in the lower lobe. This angle might create friction during the deployment maneuvers and could cause guidewire displacement; however, the vertical course of the guidewire may facilitate the crossing of the infundibulum, particularly when calcifications and stenosis produce some friction to advance long-sheath or the device ensemble. In contrast, the right pulmonary branch is more caudal, the vessel is longer and the curvature is more homogeneous, resulting in a lower risk of contra-lateral branch jailing and easier guidewire manipulation with less risk of displacement. Unfortunately, the left-convex curvature on the RVOT may create a significant impingement point on the infundibulum, when wall anomalies (pseudo-aneurysms, hypertrophic trabeculae, calcifications, etc.) are present.
Regarding patient-specific characteristics, the selection should consider factors such as the size of the pulmonary branches, the presence of proximal stenosis, distal wall injuries, risk of bleeding, concomitant maneuvers to perform before valve placement, the presence of any stent, clips, and any other surgical material that may be at risk of damage or may impede or damage the balloon during inflation. Of note, the operator might choose to change the guidewire position during the procedure, especially, before stent or valve implantation, if he finds the position unstable during the earliest interventional steps.
Regardless of the valve type to be implanted and the pre-procedural non-invasive anatomic assessment, the RVOT sizing is recommended before stent or device implantation; the purpose of sizing is to confirm the diameter of the landing zone. Differently from procedures on the aorta where the landing zone is delimited by the native valve annulus, in CHDs with native RVOT obstruction, the pulmonary annulus is widened and RVOT enlarged by surgical patch, the prosthetic valve landing zone may be found at various levels of the pulmonary trunk according to individual anatomical characteristics. Moreover, patched RVOT shows large diameter variations between systole and diastole.
A large balloon can simulate the accommodation of the valve. Once balloon stability is achieved, a right ventricular injection should be performed to confirm the stop flow (stability might be due to the impingement in a pulmonary branch). For large RVOT, when considering self-expandable valves, the sizing can be just performed with low-pressure and very compliant balloons, like a 34 mm Amplatzer Sizing Balloon or 40 mm PTS-X balloon. In case of RVOT stenosis, or before implanting a balloon-expandable valve, the sizing procedure should be performed with a high-pressure balloon, to simulate the radial force generated by the stent on the surrounding structures, mostly aortic valve, ascending aorta, and coronary arteries. Once checked stop flow on the RVOT, the angiography should be repeated in the aortic root to exclude new onset of aortic valve regurgitation and/or coronary compression, a potential contraindication to TPVR.
Once RVOT balloon interrogation is completed self-expandable valves can be implanted without further maneuvers. The selected valve size should guarantee both device stability and valve competence with the device being 2–4 mm larger than the waist size. For the Pulsta Valve and Venus Valve, the diameter is referred to as the central waist. For Alterra and Harmony devices, the retention is warranted by the edges because the central part is smaller.
Regarding balloon-expandable valves, the operator should decide whether to perform a direct implant or to proceed with an RVOT pre-stenting. The direct implant option might be considered when a safe landing zone is detected, and when the risk of device under-expansion and residual stenosis is low. For Melody valve, it might be considered in valve-in-valve procedures, when the expected final annulus will result adequate to the patient’s size. For the Sapien, it might be preferred either in valve-in-valve procedures or when it is possible to oversize the valve diameter 10%–15% more than the landing zone diameter. In case of stenotic conduits, diffuse calcifications, irregular RVOT profile, and borderline landing-zone sizes, an RVOT rehabilitation with a pre–stenting should be considered to straighten and to get a uniform caliper of the vessel. The most used stents are CP stent and Andra Stent (XL or XXL sizes). Covered stents might be preferred to pre-dilate stenotic conduits, bare metal stents represent the first option in the remaining cases.
After device deployment, final pulmonary angiography and aortic root injection should confirm the effectiveness of the device and the absence of iatrogenic coronary or aortic injuries. Despite an appropriate RVOT balloon interrogation, coronary compression and new-onset aortic valve regurgitation might be found in 2%–5% of cases. Aortic valve distortion and coronary stenosis can be resolved with surgical device removal. On the other hand, coronary occlusion might represent a life-threatening problem. Thus, in case of progressive stenting dilatation, coronary artery patency should be checked before any further step.
Hybrid Procedures
In challenging anatomies, hybrid procedures have emerged as a less invasive option, offering the potential to reduce surgical risks, enhance procedural feasibility, and improve overall outcomes [63]. Percutaneous implantation of the pulmonary valve through peripheral vascular access may be limited by factors such as poor venous access, low patient weight hemodynamic or rhythmic instability, and huge RVOT sizes [64]. In such cases, a hybrid approach might be considered to mitigate procedural risk, when re-do surgery might result in challenging or contraindicated (Fig. 4).
Figure 4: Hybrid procedure to implant a 25 Harmony TPV. (A) RVOT size by CT data. (B) Virtual renderings, CT based, of Harmony deployment. (C) Device deployment. The valve was secured through a suture on the distal part of the stent by a left anterior thoracotomy, while the proximal edge of the device was still anchored to the suture loops. (D) Final fluoroscopy after complete device deployment
Indications of hybrid approaches can be different, and a multidisciplinary team assessment (including cardiologists, imaging experts, interventional cardiologists, cardiac surgeons, and anesthesiologists) is always recommended.
Dedicated hybrid operating rooms should be prioritized, to facilitate the cooperation of different equips. Surgical access is planned based on the patient’s size, intended procedure, and bleeding risk [65]. In general, the objectives of hybrid procedures are to perform off-pump procedures, to minimize surgical invasiveness, and to shorten overall surgical times [65,66]; on the other hand, interventional cardiologists expand the procedural feasibility by utilizing various devices. In literature, hybrid approaches are described either for balloon expandable and for self-expandable valves; in case RVOT size is too large, other surgical maneuvers are planned, or in case of inadequate venous access to deploy the device [67–69].
Despite self-expandable valves expanding the feasibility spectrum of the procedure to huge native RVOT, some patients still present diameters too large for the classical percutaneous approach. In this setting, hybrid procedures by pulmonary trunk plication and percutaneous device implantation are becoming an emerging option [70].
Long Term Complications
The most common complications impacting TPVR outcome are infective endocarditis, stent fracture, and device deterioration.
Bacterial Infective Endocarditis
The incidence of infective endocarditis (IE) is about 16–27 in 1000 patients per year. Known risk factors for endocarditis are previous endocarditis, residual RVOT stenosis with invasive residual gradient >15 mmHg, and stenotic and calcified conduits [71–73]. Currently, evidence is just limited to balloon-expandable valves, because the follow-up of self-expandable devices is too short to evaluate this outcome.
Several studies underlined that the incidence of endocarditis is higher for Melody ®® TPV than for the Sapien valve. A meta-analysis published by Machanahalli Balakrishna et al. pooled IE incidence of 8.5% after the implantation of the Melody valve and pooled incidence of 2.1% after the implant of the Sapien valve [74–76]. Bovine jugular xenograft appears to be more prone to infections than handmade devices by using bovine pericardial tissue, because of the different tissue fixation processes [77,78]. However, some confounding factors might explain potential statistical bias. First, the Melody valve is smaller than the Sapien valve; they are mainly used in stenotic settings and calcific conduits, and the risk of residual RVOT gradient is higher. Second, differently from left-sided endocarditis, infections in RVOT evolve more frequently in stenosis and extensive calcifications. Thus, some conduit or patch degeneration might be due to undiagnosed past endocarditis. Third, the Melody valve is longer, and the stent is covered for all its length, by creating a larger zone isolated from the blood flow. Both these characteristics might get more favorable bacterial proliferation and lower the antibiotic concentration in that area.
The diagnosis can be suspected in case of rapidly evolving valve dysfunction (stenosis and/or regurgitation) or unexplained or recurrent fever. Blood cultures might identify the pathogenic bacteria and address the most appropriate antibiotic treatment. In patients without hemodynamic instability, the treatment of IE is based on the use of e.v. Antibiotics for 8–12 weeks. In case of refractory heart failure or when the infection is poorly controlled, urgent/emergent surgery might be considered [73].
In a large multicenter study (n = 663 Melody ®® TPV), the overall incidence of IE was 9,9% (n = 66 cases), in 41% of them surgery was needed acutely or in the short-term follow-up. Among non-invasive variables, an echocardiographic RVOT peak gradient >47 mmHg, or an increase in peak gradient across the valve of > 24 mmHg at the diagnosis were associated with surgical valve removal [79].
Stent Fractures
Stent fracture can be observed on the valve or the bare stent. These fractures might be classified as single frame fractures (type I), not impacting on stent or valve function; linear fractures (type II), impacting on stent or valve shape; and stent breaking (type III), in two or more fragments. If type I fractures might be incidentally found during fluoroscopy or chest X-ray, types II and III fractures might be suspected in case of rapid or sudden valve dysfunction. Fractures are usually due to kinking, aortic beats on the stent, impingement of the stent between the aorta and sternum, and muscular squeezing in RVOT [80,81]. In challenging anatomies, CP stents offer the highest resistance to fractures. Thus, in patients at risk for stent fractures, pre-stenting with one or more bare stents before TPVR might reduce the risk of valve dysfunction [82,83].
Treatment of stent fracture might be percutaneous, through a re-stenting, if the final expected inner diameter will result adequate for the patient’s size. If the conduit should result too small, or the risk of re-fracture remains high, surgery should be preferred.
Few data are available on self-expandable devices. Recently, Gillespie et al. reported a case series of 6 patients is the vascular protrusion of the Alterra adaptive, on a CT surveillance. The stent is used in native RVOT as a pre-stenting to create a landing zone with a useful diameter to implant a Sapien S3 valve of 29 mm. In some cases, the extravascular portion of the device can be seen contacting or extending into adjacent vascular structures including the aorta, the left pulmonary vein, and the left atrial appendage. In the patients described the vascular protrusion did not cause any complication, but it nevertheless has the potential to be clinically relevant [84].
Post-Implant Ventricular Arrhythmias
Arrhythmias represent the most common cause of morbidity and hospitalization in adult patients with CHD. Between all, conotruncal defects are particularly prone to ventricular arrhythmias. Pressure overload, volume overload, scars, infundibular patches, and right ventricular dysfunction are common triggers for arrhythmias in these patients. Barfuss et al. demonstrated that, after TPVI, native RVOT were more prone to post-procedural ventricular arrhythmias (OR 4.8, p = 0.02) [85]. The availability of self-expandable valves raised the issue of the risk stratification of arrhythmias before TPVI. In standard-risk patients, despite post-TPVI arrhythmias might occur when a stent or valve protrudes below the native pulmonary annulus. In these cases, it is usually transient and can be easily managed conservatively [86]. In the mid-term follow-up, once preload and afterload improve, the arrhythmic risk might improve [87]. On the other hand, patients with suspected or documented arrhythmic triggers should be studied and treated before or contextually to TPVI, because that focus might result in not reachable after device implantation [88].
Self-expandable valves are longer than balloon-expandable valves and the current problem is the stent protrusion below the pulmonary annulus. Several reports indicated a sub-annular implant as an independent risk factor for post-procedural arrhythmias. Fortunately, this problem is usually managed conservatively, through a short-term administration of beta-blocker [89,90]. However, longer follow-up studies should confirm the risk profile of a sub-annular implant.
TPVI has deeply changed the outcome of conotruncal CHD. The availability of a low-risk procedure compared to surgery has allowed for a timely improvement in pulmonary valve function and subsequent preservation of RV and tricuspid valve function. Therefore, following the best available evidence, these patients could preserve exercise capacity and quality of life and improve the long-term complication rate profile. The range of devices has expanded significantly over the last decade. Thus, the operators should be aware and skilled about the tips and tricks of these devices, to choose the one that best fits the anatomy of the individual patient.
The availability of new devices, custom-made devices, and lower-profile delivery systems might further improve and widen the use of TPVI in these patients.
The learning curve and the follow-up length with self-expandable devices are still short. Future studies should compare the effectiveness and freedom from adverse events of self-expandable devices with balloon-expandable devices and with surgery.
Acknowledgement: None.
Funding Statement: The authors received no specific funding for this study.
Author Contributions: Study conception and design: Biagio Castaldi; devices section: Francesco Prati, Biagio Castaldi; non-invasive section: Alice Pozza, Irene Cattapan, Jennifer Fumanelli; procedural details: Biagio Castaldi, Domenico Sirico; manuscript review: Giovanni Di Salvo. All authors reviewed the results and approved the final version of the manuscript.
Availability of Data and Materials: Not applicable.
Ethics Approval: Not applicable.
Conflicts of Interest: The authors declare no conflicts of interest to report regarding the present study.
References
1. Krasuski RA, Bashore TM. Congenital heart disease epidemiology in the United States: blindly feeling for the charging elephant. Circulation. 2016 Jul;134(2):110–3. doi:10.1161/CIRCULATIONAHA.116.023370. [Google Scholar] [PubMed] [CrossRef]
2. Vricella LA, Kanani M, Cook AC, Cameron DE, Tsang VT. Problems with the right ventricular outflow tract: a review of morphologic features and current therapeutic options. Cardiol Young. 2004 Oct;14(5):533–49. doi:10.1017/S1047951104005116. [Google Scholar] [PubMed] [CrossRef]
3. Davlouros PA, Karatza AA, Gatzoulis MA, Shore DF. Timing and type of surgery for severe pulmonary regurgitation after repair of tetralogy of Fallot. Int J Cardiol. 2004 Dec;97:91–101. doi:10.1016/j.ijcard.2004.08.013. [Google Scholar] [PubMed] [CrossRef]
4. Bonhoeffer P, Boudjemline Y, Saliba Z, Hausse AO, Aggoun Y, Bonnet D, et al. Transcatheter implantation of a bovine valve in pulmonary position: a lamb study. Circulation. 2000 Aug;102(7):813–6. doi:10.1161/01.cir.102.7.813. [Google Scholar] [PubMed] [CrossRef]
5. Bonhoeffer P, Boudjemline Y, Saliba Z, Merckx J, Aggoun Y, Bonnet D, et al. Percutaneous replacement of pulmonary valve in a right-ventricle to pulmonary-artery prosthetic conduit with valve dysfunction. Lancet Lond Engl. 2000 Oct;356(9239):1403–5. doi:10.1016/S0140-6736(00)02844-0. [Google Scholar] [PubMed] [CrossRef]
6. Boshoff DE, Cools BL, Heying R, Troost E, Kefer J, Budts W, et al. Off-label use of percutaneous pulmonary valved stents in the right ventricular outflow tract: time to rewrite the label? Catheter Cardiovasc Interv. 2013 May;81(6):987–95. doi:10.1002/ccd.24594. [Google Scholar] [PubMed] [CrossRef]
7. Khambadkone S, Coats L, Taylor A, Boudjemline Y, Derrick G, Tsang V, et al. Percutaneous pulmonary valve implantation in humans: results in 59 consecutive patients. Circulation. 2005 Aug;112(8):1189–97. doi:10.1161/CIRCULATIONAHA.104.523266. [Google Scholar] [PubMed] [CrossRef]
8. McElhinney DB, Hellenbrand WE, Zahn EM, Jones TK, Cheatham JP, Lock JE, et al. Short- and medium-term outcomes after transcatheter pulmonary valve placement in the expanded multicenter US melody valve trial. Circulation. 2010 Aug;122(5):507–16. doi:10.1161/CIRCULATIONAHA.109.921692. [Google Scholar] [PubMed] [CrossRef]
9. Zahn EM, Hellenbrand WE, Lock JE, McElhinney DB. Implantation of the melody transcatheter pulmonary valve in patients with a dysfunctional right ventricular outflow tract conduit early results from the U.S. clinical trial. J Am Coll Cardiol. 2009 Oct;54(18):1722–9. doi:10.1016/j.jacc.2009.06.034. [Google Scholar] [PubMed] [CrossRef]
10. Boudjemline Y, Bonhoeffer P. Steps toward percutaneous aortic valve replacement. Circulation. 2002 Feb;105(6):775–8. doi:10.1161/hc0602.103361. [Google Scholar] [PubMed] [CrossRef]
11. Cribier A, Eltchaninoff H, Bash A, Borenstein N, Tron C, Bauer F, et al. Percutaneous transcatheter implantation of an aortic valve prosthesis for calcific aortic stenosis: first human case description. Circulation. 2002 Dec;106(24):3006–8. doi:10.1161/01.cir.0000047200.36165.b8. [Google Scholar] [PubMed] [CrossRef]
12. Webb JG, Pasupati S, Humphries K, Thompson C, Altwegg L, Moss R, et al. Percutaneous transarterial aortic valve replacement in selected high-risk patients with aortic stenosis. Circulation. 2007 Aug;116(7):755–63. doi:10.1161/CIRCULATIONAHA.107.698258. [Google Scholar] [PubMed] [CrossRef]
13. Leon MB, Smith CR, Mack M, Miller DC, Moses JW, Svensson LG, et al. Transcatheter aortic-valve implantation for aortic stenosis in patients who cannot undergo surgery. N Engl J Med. 2010 Oct;363(17):1597–1607. doi:10.1056/NEJMoa1008232. [Google Scholar] [PubMed] [CrossRef]
14. Kenny D, Hijazi ZM, Kar S, Rhodes J, Mullen M, Makkar R, et al. Percutaneous implantation of the Edwards SAPIEN transcatheter heart valve for conduit failure in the pulmonary position: early phase 1 results from an international multicenter clinical trial. J Am Coll Cardiol. 2011 Nov;58(21):2248–56. doi:10.1016/j.jacc.2011.07.040. [Google Scholar] [PubMed] [CrossRef]
15. Holzer RJ, Hijazi ZM. Transcatheter pulmonary valve replacement: state of the art. Catheter Cardiovasc Interv. 2016 Jan;87(1):117–28. doi:10.1002/ccd.26263. [Google Scholar] [PubMed] [CrossRef]
16. Castaldi B, Di Candia A, Cuppini E, Sirico D, Reffo E, Padalino M, et al. Percutaneous approach to residual pulmonary bifurcation stenosis in conotruncal diseases. Cardiol Young. 2024 Jan;34(1):24–31. doi:10.1017/S1047951123000999. [Google Scholar] [PubMed] [CrossRef]
17. McElhinney DB, Hennesen JT. The Melody®® valve and Ensemble®® delivery system for transcatheter pulmonary valve replacement. Ann NY Acad Sci. 2013 Jul;1291(1):77–85. doi:10.1111/nyas.12194. [Google Scholar] [PubMed] [CrossRef]
18. Webb JG, Altwegg L, Masson JB, Al Bugami S, Al Ali S, Boone AS. A new transcatheter aortic valve and percutaneous valve delivery system. J Am Coll Cardiol. 2009 May;53(20):1855–8. doi:10.1016/j.jacc.2008.07.075. [Google Scholar] [PubMed] [CrossRef]
19. Kagiyama Y, Kenny D, Hijazi ZM. Current status of transcatheter intervention for complex right ventricular outflow tract abnormalities. Glob Cardiol Sci Pract. 2024 Jan;2024(1):e202407. doi:10.21542/gcsp.2024.7. [Google Scholar] [PubMed] [CrossRef]
20. Amat-Santos IJ, Dahou A, Webb J, Dvir D, Dumesnil JG, Allende R, et al. Comparison of hemodynamic performance of the balloon-expandable SAPIEN 3 versus SAPIEN XT transcatheter valve. Am J Cardiol. 2014 Oct;114(7):1075–82. doi:10.1016/j.amjcard.2014.07.019. [Google Scholar] [PubMed] [CrossRef]
21. Shivaraju A, Kodali S, Thilo C, Ott I, Schunkert H, von Scheidt W, et al. Overexpansion of the SAPIEN 3 transcatheter heart valve: a feasibility study. JACC Cardiovasc Interv. 2015 Dec;8(15):2041–3. doi:10.1016/j.jcin.2015.10.006. [Google Scholar] [PubMed] [CrossRef]
22. Hascoët S, Bentham JR, Giugno L, Betrián-Blasco P, Kempny A, Houeijeh A, et al. Outcomes of transcatheter pulmonary SAPIEN 3 valve implantation: an international registry. Eur Heart J. 2024 Jan;45(3):198–210. doi:10.1093/eurheartj/ehad663. [Google Scholar] [PubMed] [CrossRef]
23. Cao QL, Kenny D, Zhou D, Pan W, Guan L, Ge J, et al. Early clinical experience with a novel self-expanding percutaneous stent-valve in the native right ventricular outflow tract. Catheter Cardiovasc Interv. 2014 Dec;84(7):1131–7. doi:10.1002/ccd.25544. [Google Scholar] [PubMed] [CrossRef]
24. Promphan W, Prachasilchai P, Siripornpitak S, Qureshi SA, Layangool T. Percutaneous pulmonary valve implantation with the venus P-valve: clinical experience and early results. Cardiol Young. 2016 Apr;26(4):698–710. doi:10.1017/S1047951115001067. [Google Scholar] [PubMed] [CrossRef]
25. Morgan G, Prachasilchai P, Promphan W, Rosenthal E, Sivakumar K, Kappanayil M, et al. Medium-term results of percutaneous pulmonary valve implantation using the venus P-valve: international experience. EuroIntervention J Eur Collab Work Group Interv Cardiol Eur Soc Cardiol. 2019 Jan;14(13):1363–70. doi:10.4244/EIJ-D-18-00299. [Google Scholar] [PubMed] [CrossRef]
26. Benson LN, Gillespie MJ, Bergersen L, Cheatham SL, Hor KN, Horlick EM, et al. Three-year outcomes from the harmony native outflow tract early feasibility study. Circ Cardiovasc Interv. 2020 Jan;13(1):e008320. doi:10.1161/CIRCINTERVENTIONS.119.008320. [Google Scholar] [PubMed] [CrossRef]
27. Zahn EM, Chang JC, Armer D, Garg R. First human implant of the alterra adaptive Prestent™: a new self-expanding device designed to remodel the right ventricular outflow tract. Catheter Cardiovasc Interv. 2018 May;91(6):1125–9. doi:10.1002/ccd.27581. [Google Scholar] [PubMed] [CrossRef]
28. Kim AY, Jung JW, Jung SY, Shin JI, Eun LY, Kim NK, et al. Early outcomes of percutaneous pulmonary valve implantation with pulsta and melody valves: the first report from Korea. J Clin Med. 2020 Aug;9(9):2769. doi:10.3390/jcm9092769. [Google Scholar] [PubMed] [CrossRef]
29. Lee SY, Kim GB, Kim SH, Jang SI, Choi JY, Kang IS, et al. Mid-term outcomes of the Pulsta transcatheter pulmonary valve for the native right ventricular outflow tract. Catheter Cardiovasc Interv. 2021 Nov;98(5):E724–32. doi:10.1002/ccd.29865. [Google Scholar] [PubMed] [CrossRef]
30. Odemis E, Yenidogan I, Kizilkaya MH. Early results of Pulsta®® transcatheter heart valve in patients with enlarged right ventricular outflow tract and severe pulmonary regurgitation due to transannular patch. Cardiol Young. 2023 Oct;33(10):1926–34. doi:10.1017/S1047951122003511. [Google Scholar] [PubMed] [CrossRef]
31. Han BK, Moga FX, Overman D, Carter C, Lesser JR. Diagnostic value of contrast-enhanced multiphase computed tomography for assessment of percutaneous pulmonary valve obstruction. Ann Thorac Surg. 2016 Apr;101(4):e115–116. doi:10.1016/j.athoracsur.2015.09.096. [Google Scholar] [PubMed] [CrossRef]
32. Canan A, Ocazionez-Trujillo D, Vargas D, Foley TA, Cabalka AK, Rajiah PS. Pre- and postprocedure imaging of transcatheter pulmonary valve implantation. Radiogr Rev Publ Radiol Soc N Am Inc. 2022;42(4):991–1011. doi:10.1148/rg.210160. [Google Scholar] [PubMed] [CrossRef]
33. Saremi F, Ho SY, Cabrera JA, Sánchez-Quintana D. Right ventricular outflow tract imaging with CT and MRI: part 2, function. AJR. 2013 Jan;200(1):W51–61. doi:10.2214/AJR.12.9334. [Google Scholar] [PubMed] [CrossRef]
34. Kaczyńska AE, Kosiński A, Bobkowska K, Zajączkowski MA, Kamiński R, Piwko GM, et al. Clinical anatomy of the spatial structure of the right ventricular outflow tract. Adv Clin Exp Med. 2022 Jan;31(1):33–40. doi:10.17219/acem/131752. [Google Scholar] [PubMed] [CrossRef]
35. Castaldi B, Butera G, Chessa M, Galletti L, Giamberti A, Giugno L, et al. Transcatheter pulmonary valve implantation in clinical practice: a nationwide survey of cardiological implanting and non-implanting physicians. Int J Cardiol Congenit Heart Dis. 2023 Dec;14:100478. doi:10.1016/j.ijcchd.2023.100478. [Google Scholar] [PubMed] [CrossRef]
36. Sengupta PP, Narula J. RV form and function: a piston pump, vortex impeller, or hydraulic ram? JACC Cardiovasc Imaging. 2013 May;6(5):636–9. doi:10.1016/j.jcmg.2013.04.003. [Google Scholar] [PubMed] [CrossRef]
37. Malone L, Fonseca B, Fagan T, Gralla J, Wilson N, Vargas D, et al. Preprocedural risk assessment prior to PPVI with CMR and cardiac CT. Pediatr Cardiol. 2017 Apr;38(4):746–53. doi:10.1007/s00246-017-1574-0. [Google Scholar] [PubMed] [CrossRef]
38. Pozza A, Zanella L, Castaldi B, Di Salvo G. How will artificial intelligence shape the future of decision-making in congenital heart disease? J Clin Med. 2024 May;13(10):2996. doi:10.3390/jcm13102996. [Google Scholar] [PubMed] [CrossRef]
39. Chessa M, Van De Bruaene A, Farooqi K, Valverde I, Jung C, Votta E, et al. Three-dimensional printing, holograms, computational modelling, and artificial intelligence for adult congenital heart disease care: an exciting future. Eur Heart J. 2022 Jul;43(28):2672–84. doi:10.1093/eurheartj/ehac266. [Google Scholar] [PubMed] [CrossRef]
40. Ferraro AM, Bonello K, Sleeper LA, Lu M, Shea M, Marx GR, et al. A comparison between the apical and subcostal view for three-dimensional echocardiographic assessment of right ventricular volumes in pediatric patients. Front Cardiovasc Med. 2023;10:1137814. doi:10.3389/fcvm.2023.1137814. [Google Scholar] [PubMed] [CrossRef]
41. Stout KK, Daniels CJ, Aboulhosn JA, Bozkurt B, Broberg CS, Colman JM, et al. 2018 AHA/ACC guideline for the management of adults with congenital heart disease. J Am Coll Cardiol. 2018;73(12):e81–192. doi:10.1016/j.jacc.2018.08.1029. [Google Scholar] [PubMed] [CrossRef]
42. Valente AM, Cook S, Festa P, Ko HH, Krishnamurthy R, Taylor AM, et al. Multimodality imaging guidelines for patients with repaired tetralogy of fallot: a report from the american society of echocardiography. J Am Soc Echocardiogr. 2014 Feb;27(2):111–41. doi:10.1016/j.echo.2013.11.009. [Google Scholar] [PubMed] [CrossRef]
43. Lancellotti P, Tribouilloy C, Hagendorff A, Popescu BA, Edvardsen T, Pierard LA, et al. Recommendations for the echocardiographic assessment of native valvular regurgitation: an executive summary from the European association of cardiovascular imaging. Eur Heart J-Cardiovasc Imaging. 2013 Jul;14(7):611–44. doi:10.1093/ehjci/jet105. [Google Scholar] [PubMed] [CrossRef]
44. Dellas C, Kammerer L, Gravenhorst V, Lotz J, Paul T, Steinmetz M. Quantification of pulmonary regurgitation and prediction of pulmonary valve replacement by echocardiography in patients with congenital heart defects in comparison to cardiac magnetic resonance imaging. Int J Cardiovasc Imaging. 2018 Apr;34(4):607–13. doi:10.1007/s10554-017-1267-z. [Google Scholar] [PubMed] [CrossRef]
45. Van Berendoncks A, Van Grootel R, McGhie J, van Kranenburg M, Menting M, Cuypers JAAE, et al. Echocardiographic parameters of severe pulmonary regurgitation after surgical repair of tetralogy of Fallot. Congenit Heart Dis. 2019 Jul;14(4):628–37. doi:10.1111/chd.12762. [Google Scholar] [PubMed] [CrossRef]
46. Karsenty C, Alattar Y, Mousseaux E, Marcilhacy G, Gencer U, Craiem D, et al. 4D flow magnetic resonance imaging to assess right ventricular outflow tract in patients undergoing transcatheter pulmonary valve replacement. Rev Espanola Cardiol. 2023 Oct;76(10):793–802. doi:10.1016/j.rec.2023.02.010. [Google Scholar] [PubMed] [CrossRef]
47. Horowitz MJ, Kupsky DF, El-Said HG, Alshawabkeh L, Kligerman SJ, Hsiao A. 4D flow MRI quantification of congenital shunts: comparison to invasive catheterization. Radiol Cardiothorac Imaging. 2021 Apr;3(2):e200446. doi:10.1148/ryct.2021200446. [Google Scholar] [PubMed] [CrossRef]
48. Chelu RG, Horowitz M, Sucha D, Kardys I, Ingremeau D, Vasanawala S, et al. Evaluation of atrial septal defects with 4D flow MRI—multilevel and inter-reader reproducibility for quantification of shunt severity. Magn Reson Mater Phys Biol Med. 2019 Apr;32(2):269–79. doi:10.1007/s10334-018-0702-z. [Google Scholar] [PubMed] [CrossRef]
49. Moscatelli S, Pozza A, Leo I, Ielapi J, Scatteia A, Piana S, et al. Importance of cardiovascular magnetic resonance applied to congenital heart diseases in pediatric age: a narrative review. Children. 2024 Jul;11(7):878. doi:10.3390/children11070878. [Google Scholar] [PubMed] [CrossRef]
50. Pozza A, Reffo E, Castaldi B, Cattapan I, Avesani M, Biffanti R, et al. Utility of fetal cardiac resonance imaging in prenatal clinical practice: current state of the art. Diagnostics. 2023 Nov;13(23):3523. doi:10.3390/diagnostics13233523. [Google Scholar] [PubMed] [CrossRef]
51. Shaaban M, Salama M, Alsaied A, Elsheikh R, Elmasry M. Assessment of flow pattern of right ventricle outflow and pulmonary arteries in surgically corrected tetralogy of Fallot patients by four-dimensional cardiac magnetic resonance flow. Egypt Heart J. 2020 Sep;72(1):57. doi:10.1186/s43044-020-00092-y. [Google Scholar] [PubMed] [CrossRef]
52. Elsayed A, Gilbert K, Scadeng M, Cowan BR, Pushparajah K, Young AA. Four-dimensional flow cardiovascular magnetic resonance in tetralogy of Fallot: a systematic review. J Cardiovasc Magn Reson. 2021 May;23(1):59. doi:10.1186/s12968-021-00745-0. [Google Scholar] [PubMed] [CrossRef]
53. Kozak MF, Redington A, Yoo SJ, Seed M, Greiser A, Grosse-Wortmann L. Diffuse myocardial fibrosis following tetralogy of Fallot repair: a T1 mapping cardiac magnetic resonance study. Pediatr Radiol. 2014 Apr;44(4):403–9. doi:10.1007/s00247-013-2840-9. [Google Scholar] [PubMed] [CrossRef]
54. Kohi MP, Ordovas KG, Naeger DM, Meadows AK, Foster E, Higgins CB. CMR assessment of right ventricular function in patients with combined pulmonary stenosis and insufficiency after correction of tetralogy of Fallot. Acta Radiol Stockh Swed 1987. 2013 Dec;54(10):1132–7. doi:10.1177/0284185113491565. [Google Scholar] [PubMed] [CrossRef]
55. Vymazal J, Rulseh AM. MRI contrast agents and retention in the brain: review of contemporary knowledge and recommendations to the future. Insights Imaging. 2024 Jul;15(1):179. doi:10.1186/s13244-024-01763-z. [Google Scholar] [PubMed] [CrossRef]
56. Hecht HS, Blaha MJ, Kazerooni EA, Cury RC, Budoff M, Leipsic J, et al. CAC-DRS: coronary artery calcium data and reporting system. An expert consensus document of the society of cardiovascular computed tomography (SCCT). J Cardiovasc Comput Tomogr. 2018 May;12(3):185–91. doi:10.1016/j.jcct.2018.03.008. [Google Scholar] [PubMed] [CrossRef]
57. Soszyn N, Schweigert J, Franco SR, Morgan GJ, Mitchell M, Zablah JE. Computed tomography-derived normative values of right ventricular outflow tract structures in the pediatric population. Pediatr Cardiol. 2024 Mar. doi:10.1007/s00246-024-03456-2. [Google Scholar] [PubMed] [CrossRef]
58. Gać P, Trejtowicz-Sutor A, Witkowski K, Poręba R. Role of computed tomography before transcatheter pulmonary valve implantation in patients with dysfunctional native right ventricular outflow tract. Diagnostics. 2023 Oct;13(20):3231. doi:10.3390/diagnostics13203231. [Google Scholar] [PubMed] [CrossRef]
59. Zucker EJ. Cardiac computed tomography in congenital heart disease. Radiol Clin North Am. 2024 May;62(3):435–52. doi:10.1016/j.rcl.2023.12.015. [Google Scholar] [PubMed] [CrossRef]
60. Schievano S, Capelli C, Young C, Lurz P, Nordmeyer J, Owens C, et al. Four-dimensional computed tomography: a method of assessing right ventricular outflow tract and pulmonary artery deformations throughout the cardiac cycle. Eur Radiol. 2011 Jan;21(1):36–45. doi:10.1007/s00330-010-1913-5. [Google Scholar] [PubMed] [CrossRef]
61. Secinaro A, Ait-Ali L, Curione D, Clemente A, Gaeta A, Giovagnoni A, et al. Recommendations for cardiovascular magnetic resonance and computed tomography in congenital heart disease: a consensus paper from the CMR/CCT working group of the Italian Society of Pediatric Cardiology (SICP) and the Italian College of Cardiac Radiology endorsed by the Italian Society of Medical and Interventional Radiology (SIRM) Part I. Radiol Med. 2022 Jul;127(7):788–802. doi:10.1007/s11547-022-01490-9. [Google Scholar] [PubMed] [CrossRef]
62. Broncano J, Hanneman K, Ghoshhajra B, Rajiah PS. Cardiac computed tomography of native cardiac valves. Radiol Clin North Am. 2024 May;62(3):399–417. doi:10.1016/j.rcl.2023.12.004. [Google Scholar] [PubMed] [CrossRef]
63. Kalfa D, Torres AJ. Indications and results for hybrid interventions in patients with congenital heart disease. Arch Cardiovasc Dis. 2020 Feb;113(2):96–103. doi:10.1016/j.acvd.2019.06.006. [Google Scholar] [PubMed] [CrossRef]
64. Thalmann R, Merkel EM, Akra B, Bombien R, Kozlik-Feldmann RG, Schmitz C. Evaluation of hybrid surgical access approaches for pulmonary valve implantation in an acute swine model. Comp Med. 2019 Aug;69(4):299–307. doi:10.30802/AALAS-CM-18-000062. [Google Scholar] [PubMed] [CrossRef]
65. Breatnach CR, McGuinness J, Ng LY, Franklin O, Redmond M, Nölke L, et al. Procedural technique for hybrid pulmonary valve replacement in infants and small children. Eur J Cardio-Thorac Surg Off J Eur Assoc Cardio-Thorac Surg. 2021 Apr;59(4):823–30. doi:10.1093/ejcts/ezaa410. [Google Scholar] [PubMed] [CrossRef]
66. Mahadevan VS, Jenkins P, Vohra A, Hasan R, Andreas Hoschtitzky J. Hybrid right atrial approach for trans-catheter pulmonary valve implantation. Int J Cardiol. 2015 Jan;178:44–5. doi:10.1016/j.ijcard.2014.10.160. [Google Scholar] [PubMed] [CrossRef]
67. Holoshitz N, Ilbawi MN, Amin Z. Perventricular melody valve implantation in a 12 kg child. Catheter Cardiovasc Interv. 2013 Nov;82(5):824–7. doi:10.1002/ccd.25044. [Google Scholar] [PubMed] [CrossRef]
68. Mejia E, George RS, Lozier JS, Bocks ML. Perventricular transcatheter pulmonary valve implantation in a symptomatic 3-year-old child with repaired tetralogy of fallot. JACC Case Rep. 2021 May;3(5):712–4. doi:10.1016/j.jaccas.2020.12.050. [Google Scholar] [PubMed] [CrossRef]
69. Lugones I, Barbosa JD, Schvartz G, Ackerman J, Laudani V, Vitorino AM, et al. A hybrid strategy for geometrical reshaping of the main pulmonary artery and transcatheter pulmonary valve replacement. World J Pediatr Congenit Heart Surg. 2021 Sep;12(5):643–8. doi:10.1177/21501351211024994. [Google Scholar] [PubMed] [CrossRef]
70. Shibbani K, Aboulhosn J, Levi D, Mohammad Nijres B, Blais B, et al. Hybrid approach for harmony transcatheter pulmonary valve replacement. Catheter Cardiovasc Interv. 2023 Jan;101(1):135–9. doi:10.1002/ccd.30504. [Google Scholar] [PubMed] [CrossRef]
71. Østergaard L, Valeur N, Ihlemann N, Bundgaard H, Gislason G, Torp-Pedersen C, et al. Incidence of infective endocarditis among patients considered at high risk. Eur Heart J. 2018 Feb;39(7):623–9. doi:10.1093/eurheartj/ehx682. [Google Scholar] [PubMed] [CrossRef]
72. Steckelberg JM, Wilson WR. Risk factors for infective endocarditis. Infect Dis Clin North Am. 1993 Mar;7(1):9–19. doi:10.1016/S0891-5520(20)30502-X. [Google Scholar] [CrossRef]
73. Nordmeyer J, Ewert P, Gewillig M, AlJufan M, Carminati M, Kretschmar O, et al. Acute and midterm outcomes of the post-approval MELODY Registry: a multicentre registry of transcatheter pulmonary valve implantation. Eur Heart J. 2019 Jul;40(27):2255–64. doi:10.1093/eurheartj/ehz201. [Google Scholar] [PubMed] [CrossRef]
74. McElhinney DB, Zhang Y, Aboulhosn JA, Morray BH, Biernacka EK, Qureshi AM, et al. Multicenter study of endocarditis after transcatheter pulmonary valve replacement. J Am Coll Cardiol. 2021 Aug;78(6):575–89. doi:10.1016/j.jacc.2021.05.044. [Google Scholar] [PubMed] [CrossRef]
75. Houeijeh A, Batteux C, Karsenty C, Ramdane N, Lecerf F, Valdeolmillos E, et al. Long-term outcomes of transcatheter pulmonary valve implantation with melody and SAPIEN valves. Int J Cardiol. 2023 Jan;370:156–66. doi:10.1016/j.ijcard.2022.10.141. [Google Scholar] [PubMed] [CrossRef]
76. Lehner A, Haas NA, Dietl M, Jakob A, Schulze-Neick I, Dalla Pozza R, et al. The risk of infective endocarditis following interventional pulmonary valve implantation: a meta-analysis. J Cardiol. 2019 Sep;74(3):197–205. doi:10.1016/j.jjcc.2019.04.007. [Google Scholar] [PubMed] [CrossRef]
77. Wilson WM, Benson LN, Osten MD, Shah A, Horlick EM. Transcatheter pulmonary valve replacement with the edwards sapien system: the toronto experience. JACC Cardiovasc Interv. 2015 Dec;8(14):1819–27. doi:10.1016/j.jcin.2015.08.016. [Google Scholar] [PubMed] [CrossRef]
78. Davtyan A, Guyon PW, El-Sabrout HR, Ponder R, Ramchandar N, Weber R, et al. Selective valve removal for melody valve endocarditis: practice variations in a multicenter experience. Pediatr Cardiol. 2022 Apr;43(4):894–902. doi:10.1007/s00246-021-02801-z. [Google Scholar] [PubMed] [CrossRef]
79. Nordmeyer J, Khambadkone S, Coats L, Schievano S, Lurz P, Parenzan G, et al. Risk stratification, systematic classification, and anticipatory management strategies for stent fracture after percutaneous pulmonary valve implantation. Circulation. 2007 Mar;115(11):1392–7. doi:10.1161/CIRCULATIONAHA.106.674259. [Google Scholar] [PubMed] [CrossRef]
80. McElhinney DB, Bergersen L, Marshall AC. In situ fracture of stents implanted for relief of pulmonary arterial stenosis in patients with congenitally malformed hearts. Cardiol Young. 2008 Aug;18(4):405–14. doi:10.1017/S1047951108002424. [Google Scholar] [PubMed] [CrossRef]
81. Cardoso R, Ansari M, Garcia D, Sandhu S, Brinster D, Piazza N. Prestenting for prevention of melody valve stent fractures: a systematic review and meta-analysis. Catheter Cardiovasc Interv. 2016 Feb;87(3):534–9. doi:10.1002/ccd.26235. [Google Scholar] [PubMed] [CrossRef]
82. Morgan GJ, Sadeghi S, Salem MM, Wilson N, Kay J, Rothman A, et al. SAPIEN valve for percutaneous transcatheter pulmonary valve replacement without pre-stenting: a multi-institutional experience. Catheter Cardiovasc Interv. 2019 Feb;93(2):324–9. doi:10.1002/ccd.27932. [Google Scholar] [PubMed] [CrossRef]
83. Gillespie MJ, Maschietto N, Aboulhosn JA, Balzer DT, Qureshi AM, McElhinney DB. Extravascular protrusion of the Alterra adaptive prestent identified on surveillance computed tomography imaging. Catheter Cardiovasc Interv. 2024 Aug;104(2):256–63. doi:10.1002/ccd.31147. [Google Scholar] [PubMed] [CrossRef]
84. Simmons MA, Elder RW, Shabanova V, Hellenbrand W, Asnes J. Ventricular arrhythmias immediately following transcatheter pulmonary valve implantation: a cause for concern? Catheter Cardiovasc Interv. 2018 Apr;91(5):920–6. doi:10.1002/ccd.27454. [Google Scholar] [PubMed] [CrossRef]
85. Barfuss SB, Samayoa JC, Etheridge SP, Pilcher TA, Asaki SY, Ou Z, et al. Ventricular arrhythmias following balloon-expandable transcatheter pulmonary valve replacement in the native right ventricular outflow tract. Catheter Cardiovasc Interv. 2023 Jan. doi:10.1002/ccd.30560. [Google Scholar] [PubMed] [CrossRef]
86. Nguyen HH, Shahanavaz S, Van Hare GF, Balzer DT, Nicolas R, Avari Silva JN. Percutaneous pulmonary valve implantation alters electrophysiologic substrate. J Am Heart Assoc. 2016 Sep;5(10):e004325. doi:10.1161/JAHA.116.004325. [Google Scholar] [PubMed] [CrossRef]
87. Abu-Anza O, Ochoa L, McLennan D, Porayette P, Maldonado JR, Law IH, et al. The safety and efficacy of transcatheter pulmonary valve replacement combined with electrophysiology procedures. Pediatr Cardiol. 2021 Feb;42(2):289–93. doi:10.1007/s00246-020-02481-1. [Google Scholar] [PubMed] [CrossRef]
88. Lindsay I, Nik-Ahd F, Aboulhosn JA, Moore JP. Electrophysiology and structural interventions in adults with congenital heart disease: comparison of combined versus separate procedures. J Cardiovasc Electrophysiol. 2018 Sep;29(9):1280–6. doi:10.1111/jce.13637. [Google Scholar] [PubMed] [CrossRef]
89. Taylor A, Yang J, Dubin A, Chubb MH, Motonaga K, Goodyer W, et al. Ventricular arrhythmias following transcatheter pulmonary valve replacement with the harmony TPV25 device. Catheter Cardiovasc Interv. 2022 Nov;100(5):766–73. doi:10.1002/ccd.30393. [Google Scholar] [PubMed] [CrossRef]
90. Goldstein BH, McElhinney DB, Gillespie MJ, Aboulhosn JA, Levi DS, Morray BH, et al. Early outcomes from a multicenter transcatheter self-expanding pulmonary valve replacement registry. J Am Coll Cardiol. 2024 Apr;83(14):1310–21. doi:10.1016/j.jacc.2024.02.010. [Google Scholar] [PubMed] [CrossRef]
Cite This Article
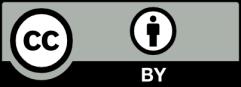
This work is licensed under a Creative Commons Attribution 4.0 International License , which permits unrestricted use, distribution, and reproduction in any medium, provided the original work is properly cited.