Open Access
REVIEW
Sodium-Glucose Cotransporter 2 Inhibitors in Adult and Pediatric Congenital Heart Disease: Review of Emerging Data and Future Directions
1 Division of Cardiovascular Medicine, Department of Internal Medicine, The Ohio State University, Columbus, OH 43210, USA
2 The Heart Center, Nationwide Children’s Hospital, Columbus, OH 43205, USA
* Corresponding Author: William H. Marshall V. Email:
Congenital Heart Disease 2024, 19(4), 419-433. https://doi.org/10.32604/chd.2024.056608
Received 26 July 2024; Accepted 18 September 2024; Issue published 31 October 2024
Abstract
Heart failure (HF) is common in patients with congenital heart disease (CHD) and there are limited medical therapies. Sodium-glucose cotransporter 2 inhibitors (SGLT2i) are a proven medical therapy in patients with acquired HF, though data are limited in patients with CHD. The aim of this review is to summarize the current evidence for use of SGLT2i in patients with CHD and identify future directions for study. In available publications, SGLT2i in patients with CHD seem to be well tolerated, with similar side effect profile to patients with acquired HF. Improvement in functional capacity and natriuretic peptides are mixed, though there is a signal for potential reduction in HF hospitalizations. One prospective study in patients with systemic right ventricles showed an improvement in systolic function for patients already on maximal HF medical therapy. Though limited, there is emerging data on use of SGLT2i in pediatric patients with CHD and HF. Future prospective studies are needed to evaluate for clinically meaningful endpoints, including HF hospitalization, as well as evaluate the hemodynamic impact in subtypes of CHD at high risk for HF.Keywords
Supplementary Material
Supplementary Material FileNomenclature
BNP | Brain natriuretic peptide |
ccTGA | Congenitally corrected transposition of the great arteries |
CHD | Congenital heart disease |
CKD | Chronic kidney disease |
GFR | Glomerular filtration rate |
HF | Heart failure |
HFrEF | Heart failure with reduced ejection fraction |
HFpEF | Heart failure with preserved ejection fraction |
SGLT2i | Sodium-glucose cotransporter 2 inhibitors |
sRV | Systemic right ventricle |
TGA | Transposition of the great arteries |
Heart failure (HF) is the leading cause of mortality and morbidity in patients with congenital heart disease (CHD) [1,2] and is complex, with multiple phenotypes [3,4]. Compared to patients with acquired HF, patients with CHD have more hospital admissions and greater costs of care [5]; thus, as the CHD population continues to grow [6], therapies are needed to improve long-term outcomes. In patients with acquired HF, medical therapy has shown improvements in morbidity and mortality, such that there are clear guidelines on appropriate HF medical therapy [7]. However, studies of HF medical therapy in patients with CHD have not shown a significant reduction in hard clinical endpoints [8,9]. This is likely at least in part due to the phenotypic heterogeneity in HF with CHD [3,4], as compared to patients with acquired HF. As such, the adult CHD guidelines do not endorse specific HF medical therapies [10]. Despite this, pharmacological therapy is an important component of HF management in patients with CHD and is often utilized. Diuretics are used to control symptoms in patients with fluid overload, and standard HF treatments such as angiotensin-converting enzyme inhibitors, angiotensin receptor blockers, angiotensin receptor neprilysin inhibitors (ARNI), beta-blockers, and mineralocorticoid receptor antagonists are frequently used, although their efficacy may vary depending on the underlying CHD [11].
Sodium-glucose cotransporter 2 inhibitors (SGLT2i) are the latest “pillar” of HF medical therapy [7], showing clinically meaningful improvements in patients with acquired HF and both reduced and preserved ejection fraction [12–15]. While the evidence for the use of SGLT2i in patients with CHD is limited in comparison to patients with acquired HF, use is often suggested in patients with CHD and HF [16]. The first report of SGLT2i use in a patient with CHD was in 2022 [17], and a literature search (outlined in Fig. 1, detailed in the Supplementary Materials) identified 10 studies [18–27] (Table 1) and 1 conference abstract [28] on the use of SGLT2i in patients with CHD. As such, the aim of this review is to summarize the current evidence for the use of SGLT2i in adult and pediatric patients with CHD and HF and identify areas for future study.
Figure 1: Literature search. * = detailed in Supplementary Materials. CHD = congenital heart disease; SGLT2i = sodium-glucose cotransporter 2 inhibitor
2 Background on SGLT2i Use in Acquired Heart Failure
SGLT2i were initially studied in patients with type 2 diabetes and high cardiovascular risk, with results showing improvement in composite major adverse cardiovascular events, including reduction in HF hospitalizations [29–31]. These results were followed by randomized controlled trials evaluating the impact of SGLT2i in patients with HF and reduced ejection fraction (HFrEF), showing patients receiving SGLT2i were at lower risk for cardiovascular death and worsening HF, independent of diabetes status [12,14]. After this, SGLT2i were incorporated into the acquired HF guidelines in addition to beta blockers, mineralocorticoid antagonists, and angiotensin-converting enzyme inhibitors/angiotensin receptor blockers or ARNIs [7].
Next, SGLT2i were evaluated in patients with HF and preserved ejection fraction (HFpEF), a more heterogenous population of patients with fewer options for efficacious medical therapy. While the reduction in mortality seen in patients with HFrEF was not reproduced in the trials of patients with HFpEF, there were reductions in HF hospitalization [13,15], and subsequently, SGLT2i were incorporated into the guidelines for the treatment of HFpEF [7]. Though guidelines make a class recommendation for SGLT2i in patients with HF [7], currently, only dapagliflozin and empagliflozin have specific dosing indications for HF without diabetes [32], with dosing described in Table 2.
A complete discussion on the mechanism of action by which SGLT2i improves cardiovascular outcomes is beyond the scope of this review and is still under active investigation. However, a brief discussion of the proposed mechanism is warranted. SGLT2 is a protein in the proximal convoluted tubule in the renal nephron which is responsible for the reabsorption of 90% filtered glucose [33]. The simplified mechanism of glucose lowering with SGLT2i in diabetes is to increase urinary glucose excretion [33]. Though originally thought to augment both diuresis and natriuresis in HF, this mechanism is insufficient to explain the long-term cardiovascular effects [34], and recent evidence in patients with HF has brought into question this paradigm of osmotic diuresis [35]. SGLT2i likely provides improvement in cardiovascular outcomes through multiple mechanisms, including improvement in cardiac contractility through reduced sarcoplasmic reticulum calcium leak, reduction in sympathetic nervous system overdrive, possible improvement in myocardial efficiency, reduction in inflammation and oxidative stress and the metabolic effects of lowered hemoglobin A1c, improved blood pressure, weight loss and improvements in vascular stiffness [33]. Another hypothesis is that inhibition of SGLT2 causes the up-regulation of cytoprotective nutrient deprivation signaling pathways, which affect both the heart and the kidneys [36].
In addition to the impact on cardiovascular outcomes, SGLT2i have shown consistent benefits in renal outcomes. In patients with HFrEF, there was a slower decline in renal function than in placebo [14]. Though caution should be used in patients where the glomerular filtration rate (GFR) is less than 30 mL/min/1.73 m2 [7], in trials of SGLT2i in patients with chronic kidney disease (CKD), cardiovascular benefits were similar with fewer HF hospitalizations and cardiovascular deaths, and renal outcomes such as doubling of serum creatinine and development of end stage kidney disease or death from renal causes were improved [29,37]. Importantly, in patients with HFrEF there was a small but statistically significant decline in GFR after initiation of dapagliflozin, especially in those with older age, lower ejection fraction, and type 2 diabetes. However, this was not associated with long-term decline in GFR or adverse events [38].
While the cardiovascular and renal benefits of SGLT2i are significant, there are a few notable side effects that have been reported. The trials of empagliflozin have shown an excess of genitourinary infections compared to placebo [13,14] (1%–2% in empagliflozin compared to <1% in placebo) which were not seen with dapagliflozin [12,15]. While not reported in the landmark trials, subsequent analyses have shown an increase in euglycemic diabetic ketoacidosis in patients receiving SGLT2i, though the overall risk is low [39]. Discontinuation of SGLT2i for any side effect in the landmark trials was 10%–14%, though notably, it was similar for the placebo [12,13].
In summary, despite the potential side effects and evolving understanding of the specific mechanism of action, SGLT2i have shown improvement in cardiovascular and renal outcomes in large clinical trials in patients with and without diabetes. As such, they have been incorporated into the guidelines for HF medical therapy and utilization is increasing in patients with acquired HF.
3.1 Rational for the Use of SGLT2i in CHD
Though patients with complex CHD were excluded from the landmark trials of SGLT2i, the use of SGLT2i in patients with CHD is increasing. This is not surprising, given the burden of HF in patients with CHD, and the mortality benefit and improvement in hospitalizations for HF with SGLT2i in acquired HF. However, there are other potential benefits that make these medications attractive. For example, in patients with liver disease from Fontan circulation, the positive metabolic effects of SGLT2i may to help minimize the occurrence of concomitant metabolic associated liver disease. Furthermore, patients with CHD often have concomitant renal disease [40], such that the renal protective effects of SGLT2i are intriguing. Finally, given inflammation may play a key role in outcomes in CHD [41], multiple mechanisms of SGLT2i have the potential to improve outcomes in this growing population of patients. Given this, next the available data on the safety of SGLT2i in patients with CHD will be reviewed, along with emerging data on the impact on heart failure hospitalizations, biomarkers, ventricular function, and functional status (Fig. 2).
Figure 2: Summary of sodium-glucose cotransporter 2 Inhibitors (SGLT2i) in patients with congenital heart disease (CHD) and heart failure (HF). FAC = fractional area change; NYHA = New York Heart Association; sRV = systemic RV. Summary based on References [18–27]. Created in Biorender. Left lower heart diagram courtesy of Dr. Curt J. Daniels
The majority of studies utilizing SGLT2i in patients with CHD report adverse events, including genitourinary infections. While some studies reported no adverse events leading to discontinuation [18,20,22,24], others report a few cases of genitourinary infections or other complaints, some leading to discontinuation of the medication [19,21]. The best “real world” evidence on adherence in patients with CHD is from a multicenter observational cohort of 174 adult patients, with 10.3% reporting some side effect, and 6.9% discontinuing the medication, including 1 patient with non-insulin dependent diabetes and obesity who developed Fournier’s gangrene [23]. As noted above, this is similar to the reported rate of discontinuation in patients with acquired HF.
One specific consideration in patients with CHD and the use of SGLT2i is the risk of genitourinary infections in patients with asplenia or functional asplenia with heterotaxy syndromes. Unfortunately, there is no data published specifically studying the risk of genitourinary infectious with SGLT2i in patients with asplenia. However, in the available reports of SGLT2i use in patients with CHD, one case series reports 3 patients with left or right atrial isomerism, with no infectious complications in follow-up (range 4–24 months) [18].
Notably, in the published reports there has not been a case of ketoacidosis from SGLT2i in a patient with CHD, though again this is a rare complication. However, given this risk, it is recommended SGLT2i be held for 3 days before elective surgery [42]. While this is ideal, many patients listed for heart transplant would benefit from SGLT2i use, but adequate advanced planning is not possible to stop the medication in these cases. As such, some heart transplant programs discontinue SGLT2i for patients listed for transplant, though recent emerging data may challenge this practice. One adult study reported 163 patients undergoing heart transplant, including 40 receiving SGLT2i, with no ketoacidosis in the cohort and a signal for increased survival in those receiving SGLT2i [43]. Furthermore, a study of 22 pediatric patients receiving SGLT2i who underwent heart transplant (including 12 patients with CHD) were matched with historic controls and reported no ketoacidosis and no difference in mortality or length of stay [27]. Given these small series, the potential benefits of SGLT2i, and the increasing waitlist time for transplant, this will be an area for further prospective study in patients with both acquired and CHD HF.
A few studies have reported the change in brain natriuretic peptide (BNP) or N terminal pro BNP (NT-Pro BNP) after initiation of SGLT2i in patients with CHD [17,18,20–22,24,25]. Both baseline and temporal changes in BNP have been associated with all-cause mortality independent of type of CHD and systemic ventricular function [44], as such it is a reasonable surrogate outcome for studies limited by power. Again, published cases report a reduction in BNP or NT-Pro BNP in short-term follow-up [17,18,21,22]. The first pediatric cohort reported BNP reduction overall but did not report specifically on those with CHD [20], and two larger single-center adult cohorts reported a significant reduction in NT-Pro BNP [19,25]. However, the only prospective study of SGLT2i in patients with CHD and systemic right ventricles (sRV), described in detail below, did not show a reduction in NT-Pro BNP compared to controls [24]. Furthermore, while natriuretic peptides may be a reasonable surrogate marker, the impact on clinical outcomes, including HF hospitalization, is more clinically meaningful.
The first few cases of SGLT2i use in patients with CHD included patients with a significant number of prior HF hospitalizations, after which there were no hospitalizations in short-term follow-up [17,18]. The previously mentioned large multicenter study of SGLT2i in patients with CHD showed a reduction in HF hospitalization and included a diverse cohort of adult patients (Table 1), of which 70% had at least moderate systemic ventricular dysfunction and were already on background therapy with other HF medications [23]. This study found a significant reduction in HF hospitalizations when comparing the 6 months before SGLT2i initiation to 6 months after (n = 36 HF hospitalizations in 6 months before SGLT2i, vs. n = 9 HF hospitalizations in 6 months after SGLT2i, relative rate 0.30 [95% CI 0.14–0.62], p = 0.001). While these findings must be put into the context of the retrospective observational study design and relatively few HF hospitalizations overall, this is a promising signal warranting further prospective study.
3.5 Functional Status & Exercise Capacity
In patients with acquired HF, the impact of SGLT2i on functional status has been mixed [45]. Patients with HFrEF and HFpEF had significant improvement in quality of life as assessed by the Kansas City Cardiomyopathy Questionnaire. Improvement in 6-min walk distance has been reported in HFrEF, and while results have varied in patients with HFpEF, patients with greater impairment in functional capacity may derive more benefit [46–48]. Thus far, studies reporting a change in functional status with SGLT2i use in patients with CHD are limited. One pediatric study reported a patient with single ventricle Fontan circulation had significant improvement in hemodynamics and ventricular function with SGLT2i, in addition to other medical therapy [21]. Two single-center cohorts of adults with CHD reported change in New York Heart Association function class; one with 18 patients and 6 months of follow-up reported no significant difference [26]. The second with 24 patients and >12 months follow-up reported a significant improvement in the proportion of patients in New York Heart Association functional classes III & IV from 58% (n = 14) at medication initiation to 25% (n = 6) at last follow up [25]. The only other studies reporting objective changes in functional status after SGLT2i therapy are in patients with biventricular CHD and sRV, which includes D-transposition of the great arteries (TGA) who have undergone atrial switch and congenitally corrected TGA (ccTGA), which are discussed below.
Two populations at increased risk for HF are patients with biventricular CHD and sRV and those with single ventricle CHD [4,49,50]. The first report of SGLT2i in a patient with CHD was a patient with ccTGA [17], and since that time the impact of SGLT2i in the population has been studied in a more robust manner. One retrospective study of 10 patients with sRV on optimal medical therapy reported improvement in functional capacity at 6 months in some patients within the cohort, as well as improvement in NT-Pro BNP [22]. However, there was no change in ventricular function by echocardiography at follow-up.
The only prospective randomized trial of SGLT2i in patients with CHD included only patients with sRV [24]. In this study, 25 patients were randomized to dapagliflozin (mean age 38 years) and 25 to placebo (mean age 34 years). All patients were on optimal medical therapy with ARNI or angiotensin converting enzyme inhibitors and had evidence of sRV systolic dysfunction with an sRV fractional area change of <35%. After 1 year of follow-up, there were no differences between the groups in systolic blood pressure, NT-Pro-BNP levels, or 6-min walk distance. However, there was a slight decrease in GFR in the treatment group (mean 111 mL/min/1.73 m2 vs. 93 mL/min/1.73 m2, p = 0.01) and a small but significant improvement in sRV fractional area change (mean increase 3.5% vs. 0.13%, p < 0.001) and sRV free wall global longitudinal strain (mean decrease of −1.6% vs. −0.1, p = 0.0005).
Prior studies in patients with sRV have not shown a significant reduction in hard clinical endpoints with HF medical therapies [8], as have been seen in patients with acquired HF. However, recent studies report some promising results with ARNIs [51,52]. While the main positive finding in this prospective study of dapagliflozin in patients with sRV is a small but significant improvement in sRV function by echocardiography, a strength of the study is that this improvement occurred with the majority of patients already on ARNI therapy [24]. In addition, as hypotension can limit HF medical therapy in this population of patients without concomitant hypertension, it is noteworthy that the addition of SGLT2i did not significantly lower the systolic blood pressure. Finally, the reduction in GFR in this study was similar to patients with acquired HF [38], and in the context of the known renal benefits of SGLT2i therapy discussed above, this should not prevent further use.
Though the improvement in sRV systolic function is encouraging, clinical endpoints are more meaningful. While studies in patients with sRV only did not show reductions in HF hospitalizations, in the large multicenter retrospective cohort discussed previously [23], a significant proportion (33%) of the patients had biventricular CHD with sRV, suggesting this population of patients at least has the potential for HF hospitalization reduction with SGLT2i therapy.
Between 7 studies [18,20,21,23,25,27,28], a total of 48 adult and pediatric patients with single ventricle CHD have been reported receiving SGLT2i therapy. While the outcomes reported in all of the cases are different, it seems the reduction in natriuretic peptides and the potential for reduction in HF hospitalization are consistent in this subgroup of patients. In addition, renal dysfunction is common in patients with Fontan circulation [53], so the potential to slow the progression of kidney disease is intriguing. However, HF in patients with single ventricle CHD, specifically those who have undergone Fontan palliation, has multiple phenotypes and is an insidious process complicated by multiple organ system complications, including liver disease and lymphatic dysfunction such as protein losing enteropathy and plastic bronchitis [3]. Thus, given the heterogenous nature of what is collectively called Fontan circulatory failure, larger studies of the potential long-term benefits of SGLT2i in this population are needed.
3.7 Pediatric Patients with CHD
Though the majority of reports of SGLT2i in patients with CHD are in adults, HF can occur across the lifespan of CHD [54]. Given this, there is growing interest in the use of SGLT2i in pediatric patients with HF. While empagliflozin has been used for other indications [55], data on use in pediatric HF are limited to 3 published studies that utilized dapagliflozin, detailed in Table 1 [20,21,27]. The main consideration for SGLT2i use is related to dosing, with dapagliflozin initiated at 0.1–0.2 mg/kg once daily, with a target dose of 0.2 mg/kg daily in patients less than 50 kg [20,21] (Table 2). For patients over 50 kg, the standard 10 mg dose was administered. Regarding safety and tolerability, of the 52 pediatric patients who were treated with SGLT2i, the medication was stopped in 9 for various reasons, and similar to adults, there were non-significant reductions in GFR after starting the medications [20,21]. Thus, while not yet part of current pediatric HF guidelines [56], many centers are incorporating SGLT2i as a fourth-line agent for pediatric patients with HF [28]. Therefore, there will likely be new data on the horizon for this population.
While there are ongoing trials to assess outcomes of SGLT2i in patients with CHD [57], further prospective multicenter studies are needed to evaluate the impact of SGLT2i on meaningful clinical outcomes. Though studies powered to evaluate for mortality are unlikely, given the signal for HF hospitalization reduction, this should be evaluated further as it has the potential to be the first medication with a positive effect on a meaningful clinical outcome. Future and ongoing studies with SGLT2i (and other HF medical therapy) in patients with CHD should also evaluate objective changes in exercise capacity in different subsets of patients with CHD.
HF in patients with CHD is often related to a residual anatomic defect or change in valve function; as such, patients may have improvement in symptoms after surgical or catheter-based interventions [58]. Furthermore, while the evidence for cardiac resynchronization therapy in HF from CHD is not as robust as it is for acquired HF [59], this is another possible therapy which is often utilized. However, these interventions take time for planning, and there may be a delay in referring the patient to a proceduralist experienced in CHD. One potential future area of study could be to evaluate the impact on symptoms and duration of recovery with early use SGLT2i (and other HF medical therapies) to “bridge” patients with HF symptoms awaiting cardiac intervention.
In patients with single ventricle physiology, higher Fontan pressures are associated with worse outcomes [60,61], and some patients with “normal” resting pressures have significant elevation in Fontan pressure with exercise [62]. Similarly, the presence of pulmonary hypertension in patients with sRV is a poor prognostic sign [63,64]. Therefore, future studies of SGLT2i in patients with CHD should evaluate for changes in invasive hemodynamics in these high-risk sub-populations of patients with CHD. Finally, if possible, a change in ventricular function utilizing cardiac magnetic resonance imaging could be revealing, especially given the potential limitations of echocardiography in assessing sRV function.
The evidence for SGLT2i in patients with acquired HF is robust, and thus far studies on use in the CHD population are limited (Fig. 2). The use of SGLT2i in patients with CHD seems to be well tolerated, with a similar side effect profile, including a small reduction in GFR, to patients with acquired HF. Improvement in functional capacity and natriuretic peptides are mixed [17,18,20–22,24–26], but there is a signal from a large retrospective study indicating a potential for reduction in HF hospitalizations in adult patients with CHD [23], and emerging data on the use of SGLT2i in pediatric patients with CHD and HF [20,21,27]. Finally, there is a potential for improvement in sRV function in patients already on maximal medical therapy [24]. These early studies of SGLT2i in patients with CHD HF have shown this medication class has the potential to improve outcomes in an understudied population and have hopefully provided momentum for future randomized prospective studies.
Acknowledgement: The authors would like to thank Curt J. Daniels, MD for use of the diagram for Fig. 2.
Funding Statement: The authors received no specific funding for this study.
Author Contributions: The authors confirm contribution to the paper as follows: study conception and design: William H. Marshall V; literature review: William H. Marshall V; draft manuscript preparation: William H. Marshall V and Lydia K. Wright. All authors reviewed the results and approved the final version of the manuscript.
Availability of Data and Materials: There is no data for this study. Full literature review is cited.
Ethics Approval: Not applicable.
Conflicts of Interest: The authors declare that they have no conflicts of interest to report regarding the present study.
Supplementary Materials: The supplementary material is available online at https://doi.org/10.32604/chd.2024.056608.
References
1. Bergh N, Skoglund K, Fedchenko M, Bollano E, Eriksson P, Dellborg M, et al. Risk of heart failure in congenital heart disease: a nationwide register-based cohort study. Circulation. 2023;147(12):982–4. doi:10.1161/CIRCULATIONAHA.122.061546. [Google Scholar] [PubMed] [CrossRef]
2. Diller G-P, Kempny A, Alonso-Gonzalez R, Swan L, Uebing A, Li W, et al. Survival prospects and circumstances of death in contemporary adult congenital heart disease patients under follow-up at a large tertiary centre. Circulation. 2015;132(22):2118–25. doi:10.1161/CIRCULATIONAHA.115.017202. [Google Scholar] [PubMed] [CrossRef]
3. Book WM, Gerardin J, Saraf A, Marie Valente A, Rodriguez F. Clinical phenotypes of fontan failure: implications for management. Congenit Heart Dis. 2016;11(4):296–308. doi:10.1111/chd.12368. [Google Scholar] [PubMed] [CrossRef]
4. Van De Bruaene A, Hickey EJ, Kovacs AH, Crean AM, Wald RM, Silversides CK, et al. Phenotype, management and predictors of outcome in a large cohort of adult congenital heart disease patients with heart failure. Int J Cardiol. 2018;252:80–7. doi:10.1016/j.ijcard.2017.10.086. [Google Scholar] [PubMed] [CrossRef]
5. Burstein DS, Rossano JW, Griffis H, Zhang X, Fowler R, Frischertz B, et al. Greater admissions, mortality and cost of heart failure in adults with congenital heart disease. Heart. 2021;107(10):807–13. doi:10.1136/heartjnl-2020-318246. [Google Scholar] [PubMed] [CrossRef]
6. Gilboa SM, Devine OJ, Kucik JE, Oster ME, Riehle-Colarusso T, Nembhard WN, et al. Congenital heart defects in the United States: estimating the magnitude of the affected population in 2010. Circulation. 2016;134(2):101–9. doi:10.1161/CIRCULATIONAHA.115.019307. [Google Scholar] [PubMed] [CrossRef]
7. Heidenreich PA, Bozkurt B, Aguilar D, Allen LA, Byun JJ, Colvin MM, et al. 2022 AHA/ACC/HFSA guideline for the management of heart failure: executive summary: a report of the American college of cardiology/American heart association joint committee on clinical practice guidelines. Circulation. 2022;145(18):e876–94. [Google Scholar] [PubMed]
8. Zaragoza-Macias E, Zaidi AN, Dendukuri N, Marelli A. Medical therapy for systemic right ventricles: a systematic review (Part 1) for the 2018 AHA/ACC guideline for the management of adults with congenital heart disease: a report of the American college of cardiology/American heart association task force on clinical practice guidelines. Circulation. 2019;139(14):e801–13. [Google Scholar] [PubMed]
9. Stout KK, Broberg CS, Book WM, Cecchin F, Chen JM, Dimopoulos K, et al. Chronic heart failure in congenital heart disease. Circulation. 2016;133(8):770–801. doi:10.1161/CIR.0000000000000352. [Google Scholar] [PubMed] [CrossRef]
10. Stout KK, Daniels CJ, Aboulhosn JA, Bozkurt B, Broberg CS, Colman JM, et al. 2018 AHA/ACC guideline for the management of adults with congenital heart disease: executive summary: a report of the American college of cardiology/American Heart Association task force on clinical practice guidelines. J Am Coll Cardiol. 2019;73(12):1494–563. doi:10.1016/j.jacc.2018.08.1028. [Google Scholar] [PubMed] [CrossRef]
11. Egbe AC, Miranda WR, Pellikka PA, DeSimone CV, Connolly HM. Prevalence and prognostic implications of left ventricular systolic dysfunction in adults with congenital heart disease. J Am Coll Cardiol. 2022;79(14):1356–65. doi:10.1016/j.jacc.2022.01.040. [Google Scholar] [PubMed] [CrossRef]
12. McMurray JJV, Solomon SD, Inzucchi SE, Køber L, Kosiborod MN, Martinez FA, et al. Dapagliflozin in patients with heart failure and reduced ejection fraction. N Engl J Med. 2019;381(21):1995–2008. doi:10.1056/NEJMoa1911303. [Google Scholar] [PubMed] [CrossRef]
13. Anker SD, Butler J, Filippatos G, Ferreira JP, Bocchi E, Böhm M, et al. Empagliflozin in heart failure with a preserved ejection fraction. N Engl J Med. 2021;385(16):1451–61. doi:10.1056/NEJMoa2107038. [Google Scholar] [PubMed] [CrossRef]
14. Packer M, Anker SD, Butler J, Filippatos G, Pocock SJ, Carson P, et al. Cardiovascular and renal outcomes with empagliflozin in heart failure. N Engl J Med. 2020;383(15):1413–24. doi:10.1056/NEJMoa2022190. [Google Scholar] [PubMed] [CrossRef]
15. Solomon SD, McMurray JJV, Claggett B, de Boer RA, DeMets D, Hernandez AF, et al. Dapagliflozin in heart failure with mildly reduced or preserved ejection fraction. N Engl J Med. 2022;387(12):1089–98. doi:10.1056/NEJMoa2206286. [Google Scholar] [PubMed] [CrossRef]
16. Vaikunth S, Sundaravel S, Saef J, Ortega-Legaspi J. Novel therapeutic strategies in heart failure in adult congenital heart disease: of medicines and devices. Curr Heart Fail Rep. 2023;20(5):401–16. doi:10.1007/s11897-023-00621-1. [Google Scholar] [PubMed] [CrossRef]
17. Egorova AD, Nederend M, Tops LF, Vliegen HW, Jongbloed MRM, Kiès P. The first experience with sodium-glucose cotransporter 2 inhibitor for the treatment of systemic right ventricular failure. ESC Heart Fail. 2022;9(3):2007–12. doi:10.1002/ehf2.13871. [Google Scholar] [PubMed] [CrossRef]
18. Muneuchi J, Sugitani Y, Kobayashi M, Ezaki H, Yamada H, Watanabe M. Feasibility and safety of sodium glucose cotransporter-2 inhibitors in adults with heart failure after the fontan procedure. Case Rep Cardiol. 2022;2022:5243594. doi:10.1155/2022/5243594. [Google Scholar] [PubMed] [CrossRef]
19. Saef J, Sundaravel S, Ortega-Legaspi J, Vaikunth S. Safety and treatment experience with sodium/glucose cotransporter-2 inhibitors in adult patients with congenital heart disease. J Card Fail. 2023;29(6):974–5. doi:10.1016/j.cardfail.2023.03.011. [Google Scholar] [PubMed] [CrossRef]
20. Newland DM, Law YM, Albers EL, Friedland-Little JM, Ahmed H, Kemna MS, et al. Early clinical experience with dapagliflozin in children with heart failure. Pediatr Cardiol. 2023;44(1):146–52. doi:10.1007/s00246-022-02983-0. [Google Scholar] [PubMed] [CrossRef]
21. Konduri A, West C, Lowery R, Hunter T, Jarosz A, Yu S, et al. Experience with SGLT2 inhibitors in patients with single ventricle congenital heart disease and fontan circulatory failure. Pediatr Cardiol. 2023. doi:10.1007/s00246-023-03332-5. [Google Scholar] [PubMed] [CrossRef]
22. Neijenhuis RML, Nederend M, Jongbloed MRM, Kiès P, Rotmans JI, Vliegen HW, et al. The potential of sodium-glucose cotransporter 2 inhibitors for the treatment of systemic right ventricular failure in adults with congenital heart disease. Front Cardiovasc Med. 2023;10:1093201. doi:10.3389/fcvm.2023.1093201. [Google Scholar] [PubMed] [CrossRef]
23. Neijenhuis RML, MacDonald ST, Zemrak F, Mertens BJA, Dinsdale A, Hunter A, et al. Effect of sodium-glucose cotransporter 2 inhibitors in adults with congenital heart disease. J Am Coll Cardiol. 2024;83(15):1403–14. doi:10.1016/j.jacc.2024.02.017. [Google Scholar] [PubMed] [CrossRef]
24. Fusco F, Scognamiglio G, Abbate M, Merola A, Grimaldi N, Ciriello GD, et al. Dapagliflozin in patients with a failing systemic right ventricle: results from the DAPA-SERVE trial. JACC Heart Fail. 2024;12(4):789–91. doi:10.1016/j.jchf.2024.01.006. [Google Scholar] [PubMed] [CrossRef]
25. Karnakoti S, Andi K, Miranda WR, Jain CC, Burchill LJ, Jokhadar M, et al. Outcomes of sodium-glucose cotransporter 2 inhibitor use in adults with congenital heart disease. CJC Pediatr Congenit Heart Dis. 2024;3(3):115–6. doi:10.1016/j.cjcpc.2024.02.001. [Google Scholar] [PubMed] [CrossRef]
26. Kheiwa A, Ssembajjwe B, Chatta P, Nageotte S, Abramov D. Safety of SGLT-2 inhibitors in the management of heart failure in the adult congenital heart disease patient population. Int J Cardiol Congen. 2024;15:100495. [Google Scholar]
27. Newland DM, Law YM, Albers EL, Ali R, Friedland-Little JM, Hartje-Dunn C, et al. Dapagliflozin use in children with advanced heart failure undergoing heart transplantation: a matched case-control study. Pediatr Cardiol. 2024. doi:10.1007/s00246-024-03604-8. [Google Scholar] [PubMed] [CrossRef]
28. Butts R, Nandi D, Hong B, Lorts A, Spinner J. (1115) SGLT2 inhibitor use in pediatric heart failure. J Heart Lung Transplant. 2023;42:S479. doi:10.1016/j.healun.2023.02.1326. [Google Scholar] [CrossRef]
29. Neal B, Perkovic V, Mahaffey KW, de Zeeuw D, Fulcher G, Erondu N, et al. Canagliflozin and cardiovascular and renal events in type 2 diabetes. N Engl J Med. 2017;377(7):644–57. doi:10.1056/NEJMoa1611925. [Google Scholar] [PubMed] [CrossRef]
30. Zinman B, Wanner C, Lachin JM, Fitchett D, Bluhmki E, Hantel S, et al. Empagliflozin, cardiovascular outcomes, and mortality in type 2 diabetes. N Engl J Med. 2015;373(22):2117–28. doi:10.1056/NEJMoa1504720. [Google Scholar] [PubMed] [CrossRef]
31. Wiviott SD, Raz I, Bonaca MP, Mosenzon O, Kato ET, Cahn A, et al. Dapagliflozin and cardiovascular outcomes in type 2 diabetes. N Engl J Med. 2019;380(4):347–57. doi:10.1056/NEJMoa1812389. [Google Scholar] [PubMed] [CrossRef]
32. Jaswaney R, Sokoloff S, Rakita V, Rubin DJ. SGLT-2 inhibitors in heart failure and chronic kidney disease: a review for internists. Cleve Clin J Med. 2024;91(7):415–23. doi:10.3949/ccjm.91a.23093. [Google Scholar] [PubMed] [CrossRef]
33. Zelniker TA, Braunwald E. Mechanisms of cardiorenal effects of sodium-glucose cotransporter 2 inhibitors: JACC state-of-the-art review. J Am Coll Cardiol. 2020;75(4):422–34. doi:10.1016/j.jacc.2019.11.031. [Google Scholar] [PubMed] [CrossRef]
34. Packer M. SGLT2 inhibition: neither a diuretic nor a natriuretic. J Am Coll Cardiol. 2024;83(15):1399–402. doi:10.1016/j.jacc.2024.02.028. [Google Scholar] [PubMed] [CrossRef]
35. Marton A, Saffari SE, Rauh M, Sun R-N, Nagel AM, Linz P, et al. Water conservation overrides osmotic diuresis during SGLT2 inhibition in patients with heart failure. J Am Coll Cardiol. 2024;83(15):1386–98. doi:10.1016/j.jacc.2024.02.020. [Google Scholar] [PubMed] [CrossRef]
36. Packer M. Critical reanalysis of the mechanisms underlying the cardiorenal benefits of SGLT2 inhibitors and reaffirmation of the nutrient deprivation signaling/autophagy hypothesis. Circulation. 2022;146(18):1383–405. doi:10.1161/CIRCULATIONAHA.122.061732. [Google Scholar] [PubMed] [CrossRef]
37. Heerspink HJL, Stefánsson BV, Correa-Rotter R, Chertow GM, Greene T, Hou F-F, et al. Dapagliflozin in patients with chronic kidney disease. N Engl J Med. 2020;383(15):1436–46. doi:10.1056/NEJMoa2024816. [Google Scholar] [PubMed] [CrossRef]
38. Adamson C, Docherty KF, Heerspink HJL, de Boer RA, Damman K, Inzucchi SE, et al. Initial decline (Dip) in estimated glomerular filtration rate after initiation of dapagliflozin in patients with heart failure and reduced ejection fraction: insights from DAPA-HF. Circulation. 2022;146(6):438–49. doi:10.1161/CIRCULATIONAHA.121.058910. [Google Scholar] [PubMed] [CrossRef]
39. Chow E, Clement S, Garg R. Euglycemic diabetic ketoacidosis in the era of SGLT-2 inhibitors. BMJ Open Diabetes Res Care. 2023;11(5):e003666. doi:10.1136/bmjdrc-2023-003666. [Google Scholar] [PubMed] [CrossRef]
40. El Sayegh S, Ephrem G, Wish JB, Moe S, Lim K. Kidney disease and congenital heart disease: partnership for life. Front Physiol. 2022;13:970389. doi:10.3389/fphys.2022.970389. [Google Scholar] [PubMed] [CrossRef]
41. Wienecke LM, Cohen S, Bauersachs J, Mebazaa A, Chousterman BG. Immunity and inflammation: the neglected key players in congenital heart disease? Heart Fail Rev. 2022;27(5):1957–71. doi:10.1007/s10741-021-10187-6. [Google Scholar] [PubMed] [CrossRef]
42. Handelsman Y, Henry RR, Bloomgarden ZT, Dagogo-Jack S, DeFronzo RA, Einhorn D, et al. American association of clinical endocrinologists and american college of endocrinology position statement on the association of SGLT-2 inhibitors and diabetic ketoacidosis. Endocr Pract. 2016;22(6):753–62. doi:10.4158/EP161292.PS. [Google Scholar] [PubMed] [CrossRef]
43. Raven LM, Muir CA, Deveza RC, Iglesias CK, Bart NK, Muthiah K, et al. Effect of sodium glucose cotransporter 2 inhibition immediately prior to heart transplantation. JHLT Open. 2024;5:100088. doi:10.1016/j.jhlto.2024.100088. [Google Scholar] [CrossRef]
44. Yumita Y, Xu Z, Diller G-P, Kempny A, Rafiq I, Montanaro C, et al. B-type natriuretic peptide levels predict long-term mortality in a large cohort of adults with congenital heart disease. Eur Heart J. 2024;45(23):2066–75. doi:10.1093/eurheartj/ehae254. [Google Scholar] [PubMed] [CrossRef]
45. Banner K, Silverman DN. Will the SGLT2i responders please stand up? Circ Heart Fail. 2023;16(11):e011124. [Google Scholar]
46. Abraham WT, Lindenfeld J, Ponikowski P, Agostoni P, Butler J, Desai AS, et al. Effect of empagliflozin on exercise ability and symptoms in heart failure patients with reduced and preserved ejection fraction, with and without type 2 diabetes. Eur Heart J. 2021;42(6):700–10. doi:10.1093/eurheartj/ehaa943. [Google Scholar] [PubMed] [CrossRef]
47. Lewis GD, Gosch K, Cohen LP, Nassif ME, Windsor SL, Borlaug BA, et al. Effect of dapagliflozin on 6-minute walk distance in heart failure with preserved ejection fraction: PRESERVED-HF. Circ Heart Fail. 2023;16(11):e010633. [Google Scholar] [PubMed]
48. Kosiborod MN, Bhatt AS, Claggett BL, Vaduganathan M, Kulac IJ, Lam CSP, et al. Effect of dapagliflozin on health status in patients with preserved or mildly reduced ejection fraction. J Am Coll Cardiol. 2023;81(5):460–73. doi:10.1016/j.jacc.2022.11.006. [Google Scholar] [PubMed] [CrossRef]
49. Broberg CS, van Dissel A, Minnier J, Aboulhosn J, Kauling RM, Ginde S, et al. Long-term outcomes after atrial switch operation for transposition of the great arteries. J Am Coll Cardiol. 2022;80(10):951–63. doi:10.1016/j.jacc.2022.06.020. [Google Scholar] [PubMed] [CrossRef]
50. van Dissel AC, Opotowsky AR, Burchill LJ, Aboulhosn J, Grewal J, Lubert AM, et al. End-stage heart failure in congenitally corrected transposition of the great arteries: a multicentre study. Eur Heart J. 2023;44(34):3278–91. doi:10.1093/eurheartj/ehad511. [Google Scholar] [PubMed] [CrossRef]
51. Fusco F, Scognamiglio G, Merola A, Iannuzzi A, Palma M, Grimaldi N, et al. Safety and efficacy of sacubitril/valsartan in patients with a failing systemic right ventricle: a prospective single-center study. Circ Heart Failure. 2022;16(2):e009848. [Google Scholar] [PubMed]
52. Nederend M, Kiès P, Regeer MV, Vliegen HW, Mertens BJ, Robbers-Visser D, et al. Tolerability and beneficial effects of sacubitril/valsartan on systemic right ventricular failure. Heart. 2023;109(20):1525–32. doi:10.1136/heartjnl-2022-322332. [Google Scholar] [PubMed] [CrossRef]
53. Khuong JN, Wilson TG, Grigg LE, Bullock A, Celermajer D, Disney P, et al. Fontan-associated nephropathy: predictors and outcomes. Int J Cardiol. 2020;306:73–7. doi:10.1016/j.ijcard.2020.01.014. [Google Scholar] [PubMed] [CrossRef]
54. Dykes JC, Rosenthal DN, Bernstein D, McElhinney DB, Chrisant MRK, Daly KP, et al. Clinical and hemodynamic characteristics of the pediatric failing Fontan. J Heart Lung Transplant. 2021;40(12):1529–39. doi:10.1016/j.healun.2021.07.017. [Google Scholar] [PubMed] [CrossRef]
55. Lava SAG, Laurence C, Di Deo A, Sekarski N, Burch M, Della Pasqua O. Dapagliflozin and empagliflozin in paediatric indications: a systematic review. Paediatr Drugs. 2024;26(3):229–43. doi:10.1007/s40272-024-00623-z. [Google Scholar] [PubMed] [CrossRef]
56. Amdani S, Conway J, George K, Martinez HR, Asante-Korang A, Goldberg CS, et al. Evaluation and management of chronic heart failure in children and adolescents with congenital heart disease: a scientific statement from the american heart association. Circulation. 2024;150(2):e33–50. [Google Scholar] [PubMed]
57. Moceri P. SGLT2 inhibitors in adult patients with heart failure related to congenital heart disease. Available from: https://clinicaltrials.gov/study/NCT05897489. [Accessed 2024]. [Google Scholar]
58. Crossland DS, Van De Bruaene A, Silversides CK, Hickey EJ, Roche SL. Heart failure in adult congenital heart disease: from advanced therapies to end-of-life care. Can J Cardiol. 2019;35(12):1723–39. doi:10.1016/j.cjca.2019.07.626. [Google Scholar] [PubMed] [CrossRef]
59. Ramdat Misier NL, Moore J, Nguyen HH, Lloyd MS, Dubin AM, Mah DY, et al. Long-term outcomes of cardiac resynchronization therapy in patients with repaired tetralogy of fallot: a multicenter study. Circ Arrhythm Electrophysiol. 2024;17(3):e012363. [Google Scholar] [PubMed]
60. Miranda WR, Borlaug BA, Hagler DJ, Connolly HM, Egbe AC. Haemodynamic profiles in adult Fontan patients: associated haemodynamics and prognosis. Eur J Heart Fail. 2019;21(6):803–9. doi:10.1002/ejhf.2019.21.issue-6. [Google Scholar] [CrossRef]
61. Inai K, Inuzuka R, Ono H, Nii M, Ohtsuki S, Kurita Y, et al. Predictors of long-term mortality among perioperative survivors of Fontan operation. Eur Heart J. 2022;43(25):2373–84. doi:10.1093/eurheartj/ehab826. [Google Scholar] [PubMed] [CrossRef]
62. Miranda WR, Jain CC, Borlaug BA, Jaffe AS, Connolly HM, Burchill LJ, et al. Exercise capacity, NT-proBNP, and exercise hemodynamics in adults post-fontan. J Am Coll Cardiol. 2023;81(16):1590–600. doi:10.1016/j.jacc.2023.02.031. [Google Scholar] [PubMed] [CrossRef]
63. Van De Bruaene A, Toh N, Hickey EJ, Benson L, Horlick E, Granton JT, et al. Pulmonary hypertension in patients with a subaortic right ventricle: prevalence, impact and management. Heart. 2019;105(19):1471–8. doi:10.1136/heartjnl-2019-314756. [Google Scholar] [PubMed] [CrossRef]
64. Chaix M-A, Dore A, Mercier L-A, Mongeon F-P, Marcotte F, Ibrahim R, et al. Late onset postcapillary pulmonary hypertension in patients with transposition of the great arteries and mustard or senning baffles. J Am Heart Assoc. 2017;6(10):e006481. doi:10.1161/JAHA.117.006481. [Google Scholar] [PubMed] [CrossRef]
Cite This Article
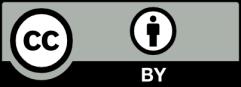
This work is licensed under a Creative Commons Attribution 4.0 International License , which permits unrestricted use, distribution, and reproduction in any medium, provided the original work is properly cited.