Open Access
ARTICLE
Risk Stratification and Prognosis of Pulmonary Arterial Hypertension Associated with Congenital Heart Disease
1 Department of Cardiology, The First People’s Hospital of Tianshui, Tianshui, 741000, China
2 Department of Cardiology, Pulmonary Vascular Disease Center, Gansu Provincial Hospital, Lanzhou, 730000, China
3 Heart, Lung and Vessels Center, Sichuan Provincial People’s Hospital, University of Electronic Science and Technology of China, Chengdu, 610072, China
* Corresponding Authors: Hongling Su. Email: ; Yunshan Cao. Email:
(This article belongs to the Special Issue: Interventional therapy of congenital heart disease: past, now, and future)
Congenital Heart Disease 2024, 19(3), 325-339. https://doi.org/10.32604/chd.2024.052267
Received 28 March 2024; Accepted 01 July 2024; Issue published 26 July 2024
Abstract
Background: Current guidelines for managing pulmonary arterial hypertension (PAH) recommend a risk stratification approach. However, the applicability and accuracy of these strategies for PAH associated with congenital heart disease (PAH-CHD) require further validation. This study aims to validate the reliability and predictive accuracy of a simplified stratification strategy for PAH-CHD patients over a three-year follow-up. Additionally, new prognostic variables are identified and novel risk stratification methods are developed for assessing and managing PAH-CHD patients. Methods: This retrospective study included 126 PAH-CHD patients. Clinical and biochemical variables across risk groups were assessed using Kruskal-Wallis and Fisher’s exact tests. Independent risk factors were identified using ordered logistic regression, while Kaplan-Meier and Cox proportional hazards regression analyses evaluated their impact on all-cause mortality. A new stratification model for the PAH-CHD population was constructed based on these analyses. Results: Significant survival differences across stratified risk groups were observed (p < 0.001), validating the effectiveness of the simplified risk stratification method in PAH-CHD patients. Prothrombin activity was a strong independent predictor of adverse outcomes of PAH-CHD patients (Hazard ratio 0.95, p < 0.001, C-index 0.70). A model combining N-terminal pro-brain natriuretic peptide, prothrombin activity, albumin, and right atrial area achieved an area under the curve of 0.89 and a C-index of 0.85. Conclusions: The simplified risk stratification method is applicable to PAH-CHD patients. Prothrombin activity is a strong independent predictor of adverse outcomes. A comprehensive risk stratification approach, incorporating both established and novel biomarkers, enhances accessibility and offers predictive efficacy during follow-up for PAH-CHD patients, comparable to established models.Graphic Abstract
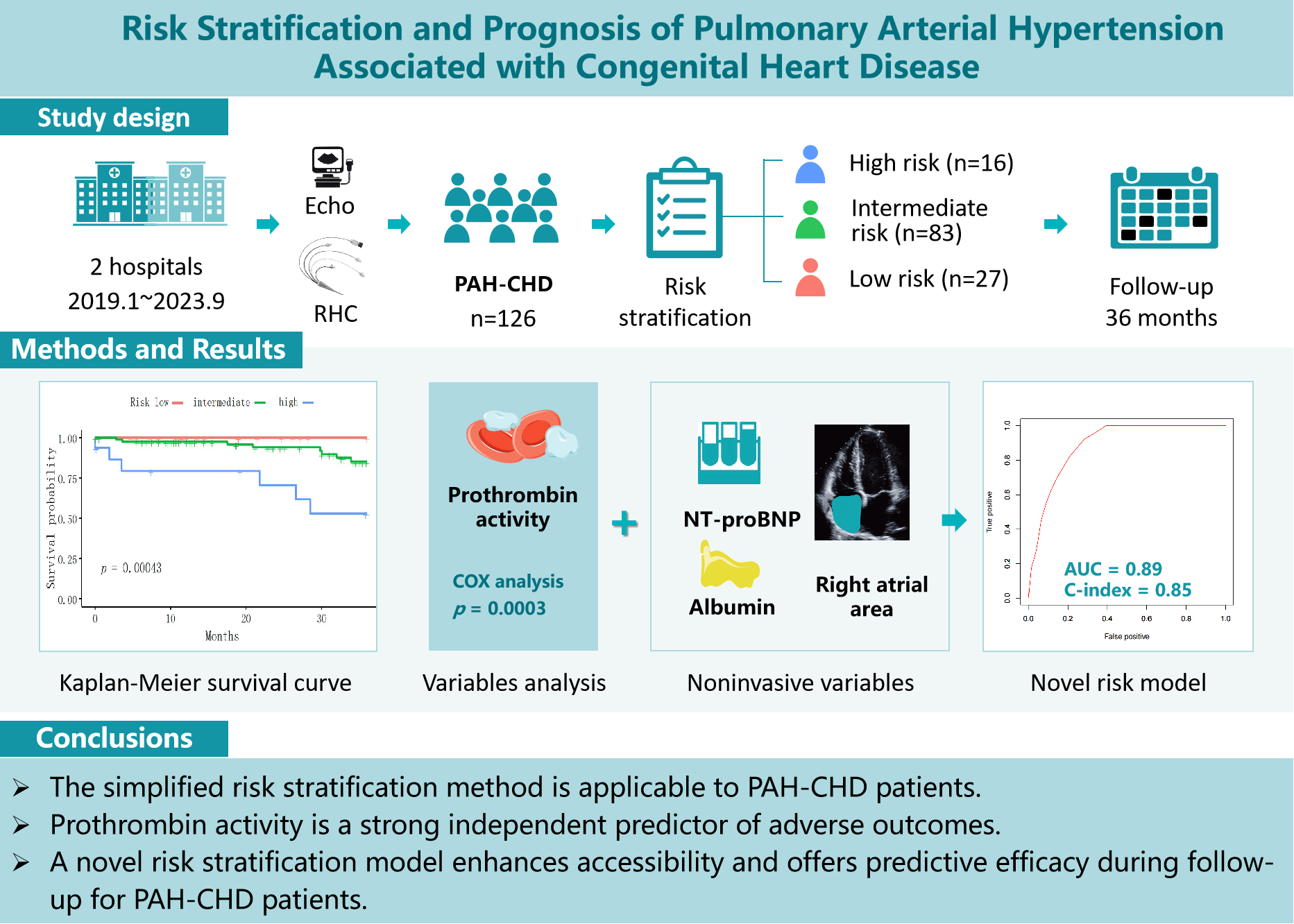
Keywords
Supplementary Material
Supplementary Material FilePulmonary arterial hypertension (PAH), classified within Group 1 pulmonary hypertension (PH), is a progressive and potentially fatal disease affecting individuals with varied conditions, including congenital heart disease (CHD) [1,2]. Characterized by the obstruction of small pulmonary arteries, PAH leads to increased pulmonary vascular resistance (PVR) and right heart failure [3]. If left untreated, this progression is often fatal. Despite significant advancements in targeted pharmacotherapy improving prognosis, PAH continues to impose substantial health burdens, presenting persistent risks of morbidity and mortality. Current guidelines recommend a goal-oriented therapeutic approach for managing PAH, underscoring the utility of multiparametric risk stratification to make decisions and improve patient outcomes [1,4]. Although risk stratification has been validated as effective in some studies, patients with PAH-associated with CHD (PAH-CHD), are often underrepresented or entirely absent from these cohorts. Consequently, the applicability of this stratification method to effectively manage PAH-CHD remains fraught with challenges. A seminal study involving 31 centers in China provided pivotal insights: it identified that nearly half (49.5%) of PAH cases were PAH-CHD, a rate substantially higher than those reported in European populations [5–8].
The study aimed to assess the reliability of the simplified stratification strategy specifically for the PAH-CHD population. Over a three-year follow-up, it evaluated the strategy’s predictive accuracy, analyzed clinical data using stratified methods, identified superior prognostic variables, and developed a novel risk stratification model tailored for managing PAH-CHD patients.
This study retrospectively reviewed 146 patients newly diagnosed with CHD using echocardiography and underwent right heart catheterization (RHC) in the Pulmonary Vascular Disease Center, Gansu Provincial Hospital, and the Department of Cardiology, The First People’s Hospital of Tianshui, between January 2019 and September 2023. The inclusion criteria included: PAH was diagnosed according to guidelines using RHC, defined by a mean pulmonary arterial pressure (mPAP) >20 mmHg, pulmonary arterial wedge pressure (PAWP) ≤15 mmHg and PVR > 2 Wood units [1]. Exclusion criteria included patients with significant comorbid conditions such as advanced liver or renal disease, cancer, and severe systemic infections. By following these criteria, 126 patients were ultimately considered for further analysis. The study was approved by the Ethics Committee of Gansu Provincial Hospital (Approval No. 2022-333) and the Ethics Committee of the First People’s Hospital of Tianshui (Approval No. 2024-07). Informed consent was not required due to the retrospective nature of the study.
2.2.1 Baseline Characteristics and Clinical Phenotypes
Baseline demographic data were collected from patient medical records, including age, gender, and body mass index (BMI). Clinical classification of PAH-CHD was categorized into four types: Eisenmenger’s syndrome, PAH with prior systemic-to-pulmonary shunts, PAH with small/coincidental defects, and PAH following defect correction [1]. Additionally, we also classified patients based on distinctions between pre- and post-tricuspid shunts and their complexity [9].
2.2.2 Collection of Additional Stratification Variables
Additional variables were collected for potential use in risk stratification. Complete blood count was assessed using an automated analyzer (XN-10, Sysmex, Kobe, Japan). Serum biochemical markers, including liver enzymes, kidney function tests, lipid profile, electrolytes, cardiac enzymes and N-terminal pro-brain natriuretic peptide (NT-proBNP), were measured with a biochemistry analyzer (Cobas e602, Roche, Basel, Switzerland), along with cardiac troponin T (Beckman Coulter DXL800, Brea, CA, USA). Coagulation profiles were analyzed using a coagulation analyzer (EVOLUTION, Stago, Asnières-sur-Seine, France). Arterial blood gases were determined using a blood gas analyzer (GEM5000, Werfen, Bedford, MA, USA). Echocardiographic parameters were obtained via transthoracic echocardiography using a high-resolution EPIQ 5 machine (Philips, Eindhoven, Netherlands), evaluating cardiac structures and functions such as right atrial area (RAA), right ventricular area, ejection fraction, tricuspid annular plane systolic excursion (TAPSE), and inferior vena cava collapse index. RHC was performed using a Swan-Ganz catheter (Edwards Lifesciences, Irvine, CA, USA) and a IntelliVue MP50 (Philips, Eindhoven, Netherlands) hemodynamic monitoring system. Measurements included mPAP and PAWP. PVR was calculated from cardiac output using the Fick principle [10].
In this study, we employed a simplified risk stratification method adapted from the European Society of Cardiology and European Respiratory Society guidelines for PAH [1]. The risk stratification utilized multiple variables including World Health Organization functional class (WHO-FC), 6-minute walk distance (6MWD), and NT-proBNP. Right atrial pressure (RAP), cardiac index (CI), and mixed venous oxygen saturation (SvO2) which were measured by RHC. We collected them into a scoring system to categorize patients into low-, intermediate-, and high-risk groups. Each variable was scored based on established thresholds from previous studies [6,7]. To categorize patients into risk groups—low-, intermediate-, and high—scores for each patient were summed and then averaged by dividing by the number of variables assessed. This average was rounded to the nearest whole number to determine the risk category [4,6,7] (Table S1).
Follow-up was performed through outpatient visits, phone calls, or online meetings. The follow-up team consisted of attending physicians, cardiology residents, and trained nurses, ensuring comprehensive and consistent patient monitoring. The main outcome measured was the duration from PAH-CHD diagnosis to all-cause mortality. For patients who had not undergone surgery at the initiation of the follow-up period but subsequently received surgical treatment, their follow-up was terminated on the day of surgery.
Continuous variables were presented as mean ± standard deviation (SD) or median with interquartile range (IQR), based on their distribution. Categorical variables were shown as frequencies and percentages (%). We used the Kruskal-Wallis H-test to compare continuous variables among the risk groups (low, medium, high risk), followed by pairwise comparisons with the Mann-Whitney U test, adjusting for multiple comparisons with the Bonferroni method (Table S2). Fisher’s exact test was applied to categorical variables. Variables that were significant in the univariate analysis were further analyzed in an ordered logistic regression model to examine their independent effects on risk classification. Survival analysis was conducted using the Kaplan-Meier method, with the Log-Rank test comparing survival across risk groups. The performance of different models in predicting outcomes was assessed with time-dependent ROC curves. All analyses were performed using R software (version 3.4.3), with p < 0.05 considered statistically significant.
3.1 Patient Characteristics and Risk Stratification
In this study involving 126 PAH-CHD patients, we classified them into three risk categories according to the simplified risk stratification guidelines: low risk (n = 27), intermediate risk (n = 83), and high risk (n = 16). Our analysis revealed no significant differences in age, gender distribution, or BMI among the different risk groups. However, significant distinctions were found in key stratification indicators including WHO-FC, 6MWD, NT-proBNP, and SvO2 and CI, both measured by RHC (Table 1).
126 PAH patients were categorized into low-, intermediate-, and high-risk groups. A range of hematological indices, including white and red blood cell counts, hemoglobin, and platelet counts, along with differential white cell counts, were examined. No significant differences were found in these parameters across the risk groups (p > 0.05) (Table 2).
In our examination of 126 PAH-CHD patients categorized by risk level (low, intermediate, high), biochemical parameters were compared. The analysis revealed statistically significant differences across risk categories in total protein (p = 0.013), albumin (p = 0.006), total bilirubin (p = 0.031), direct bilirubin (p = 0.014), high-density lipoprotein cholesterol (HDL-C, p = 0.015), lactate dehydrogenase (LDH, p = 0.022), and calcium (p = 0.021). These findings indicated that patients with high-risk status exhibited poorer nutritional status and liver function compared to the individuals in the low- and intermediate-risk groups (Table 3).
3.4 Coagulation Function Parameters
In our analysis of coagulation function parameters among PAH-CHD patients, we examined prothrombin activity, international normalized ratio (INR), activated partial thromboplastin time, fibrinogen, thrombin time, D-dimer, and fibrinogen degradation products, comparing across low-, intermediate-, and high-risk categories. The findings revealed significant differences in prothrombin activity (p = 0.004) and INR among the risk groups (p = 0.001), with the high-risk group displaying lower prothrombin activity and higher INR compared to the low- and intermediate-risk groups (Table 4).
3.5 Echocardiographic and Arterial Blood Gas Analysis Parameters
In the analysis of echocardiographic and arterial blood gas parameters among the three-risk groups, significant findings included right atrial and ventricular enlargement in the intermediate- and high-risk groups (p = 0.019 and p = 0.007, respectively), coupled with a decline in TAPSE (p = 0.028). Hypoxemia was also more severe in these groups (PO2 p = 0.011, SO2 p < 0.001), suggesting increased gas exchange inefficiency (Table 5).
3.6 Predictors in PAH-CHD Risk Stratification
In the ordinal logistic regression analysis assessing the impact of various biochemical and clinical parameters on risk stratification, prothrombin activity (p = 0.0042) demonstrated statistically significant associations (Table 6).
3.7 Prognostic Analysis in the PAH-CHD Population
3.7.1 Simplified Risk Stratification Survival Analysis in PAH-CHD Patients
Patients were categorized into low-, intermediate-, and high-risk categories, following the simplified stratification strategy. Over a three-year period, within the follow-up time frame, 14 (11%) patients died. The survival rates were 96.03%, 93.65%, and 88.89% at one, two, and three years, respectively. The low-risk group achieved a 100% survival rate, while the intermediate-risk and the high-risk groups recorded declining survival rates of 90.36% and 62.5% by the third year, respectively. Notably, the median survival for the high-risk group was 26.6 months. A significant difference in survival across the groups (p < 0.001) confirmed the effectiveness of the simplified risk stratification method for PAH-CHD patients (Fig. 1).
Figure 1: The Kaplan-Meier survival curve illustrates the survival probabilities over time for PAH patients stratified into three risk categories: low, intermediate, and high. The significant difference in survival across groups (p = 0.0004) affirmed the applicability of the simplified risk stratification method for PAH-CHD patients
3.7.2 Prothrombin Activity and Mortality Risk in PAH-CHD Patients
Our analysis of 126 PAH-CHD patients, with 14 deaths, showed prothrombin activity significantly reduced mortality risk by 4.7% per unit increase (Hazard ratio = 0.95, p < 0.001). This highlights its importance as a prognostic factor, supported by robust statistical tests and good model predictivity (Harrell’s C-index = 0.70) (Table 7).
3.7.3 Stratified Prothrombin Activity Survival Analysis in PAH-CHD Patients
PAH-CHD patients were stratified into low (>100%), intermediate (70%–100%), and high (<70%) risk groups based on prothrombin activity. Kaplan-Meier analysis revealed nearly 100% survival for the low-risk group over three years, a slight decline in survival for the intermediate-risk group, and significantly lower survival for the high-risk group, especially after 20 months (p < 0.001). This highlights prothrombin activity as a valuable prognostic marker for PAH patient survival and supports its use in risk stratification (Fig. 2).
Figure 2: The Kaplan-Meier survival curve presented illustrates the survival probabilities for three groups of patients categorized by prothrombin activity levels, with the groups defined as high risk, intermediate risk, and low risk
3.7.4 Prognostic Model Assessment in PAH-CHD
A comprehensive risk stratification approach, incorporating NT-proBNP, prothrombin activity, albumin, and RAA, enhanced accessibility and provided predictive efficacy comparable to established models for PAH-CHD patients (Fig. 3).
Figure 3: The figure illustrates ROC curves for PAH-CHD predictive models. The combination of all simple risk stratification variables is represented by the black curve (AUC 0.84, C-index 0.84). The inclusion of new markers is shown by the red curve (AUC 0.89, C-index 0.85)
Current guidelines for PAH recommend a three-strata risk assessment model with a multiparameter to categorize patients into low-, intermediate-, or high-risk groups. Such stratification not only facilitates clinical risk assessments but also informs the development of targeted treatment strategies, ultimately improving the precision and efficacy of management [1].
This stratification strategy has been simplified and validated in several studies, confirming its efficacy. The SPAHR study, involving seven Swiss Pulmonary Arterial Hypertension centers, conducted a comprehensive risk stratification of 530 PAH patients using multidimensional criteria including WHO-FC, 6MWD, RAP, and CI, demonstrating significant long-term survival differences among risk groups at both diagnosis and during follow-up. However, only 13% of the study population was PAH-CHD patients [6]. Similarly, the French PH Registry, which analyzed 1591 PAH patients, found a positive correlation between achieving low-risk criteria within the first year of treatment and higher survival rates. It advocated for a non-invasive risk assessment tool to identify patients with a low risk of death or lung transplantation, avoiding routine invasive hemodynamic follow-up assessments for certain patients [8]. The COMPERA study across seven European countries, involving 1588 PAH patients, confirmed the efficacy of guideline-based risk stratification. It introduced a simplified risk stratification that used six selected variables to accurately distinguish between risk groups. Among the variables used for risk stratification, non-invasive variables such as WHO-FC and NT-proBNP provided independent prognostic information. Nevertheless, PAH-CHD representation was minimal at only 4% [7]. In contrast, a multicenter Chinese study of 551 PAH patients reported that the largest subgroup was PAH-CHD, with 273 (49.5%) patients [5]. Another study conducted a 12-month follow-up on Chinese PAH-CHD patients, identifying 6MWD and NT-proBNP as independent predictors of adverse events [11].
In our study, we implemented the simplified risk stratification approach from the COMPERA study over a three-year follow-up of a PAH-CHD patient cohort [7]. Our findings corroborated the precision of risk assessments within this subgroup and further validated the applicability and efficacy of the stratification method for PAH-CHD patients.
PAH-CHD presents a spectrum of symptoms and complications that vary with the severity of PAH and the nature of the cardiac defect. Recent investigations have consistently underscored the profound impact of biochemical markers and therapeutic interventions on the prognosis of PAH-CHD patients. Studies have shown that individuals with PAH-CHD exhibit elevated hemoglobin and hematocrit levels, along with higher creatinine and bilirubin abnormalities, leading to organ dysfunction [12–15]. A study by Scognamiglio G et al. involving 225 adults with PAH-CHD followed them over 4.8 years, with C-reactive protein identified as a robust predictor of mortality [16]. Additionally, an analysis of 2886 adult CHD patients demonstrated a clear link between hypoalbuminemia and increased mortality [17]. Data from the COMPERA registry further revealed that idiopathic PAH patients on anticoagulation therapy had significantly better three-year survival rates than those without such treatment [18].
Currently, there is no evidence to suggest that involving routine RHC is associated with better therapeutic outcomes compared to a non-invasive follow-up strategy [4]. In response, we developed a model that primarily utilizes non-invasive variables to optimize follow-up protocols for this subgroup. This approach is comparable to the established model and offers additional, easily accessible benefits. It may present a preferable alternative for risk stratification when RHC is unavailable. Our study confirmed that incorporating NT-proBNP, prothrombin activity, albumin, and RAA into the risk stratification model provided an effective and straightforward prognostic prediction for PAH-CHD patients. Specifically, higher NT-proBNP levels and enlarged RAA are associated with a poorer prognosis, consistent with previous research findings [6–8]. Regarding new biomarkers, lower prothrombin activity (<70%) and albumin levels (<40 g/L) indicate a high-risk status, predicting worse disease outcomes. Conversely, patients with prothrombin activity above 100% and albumin levels above 45 g/L are categorized as low-risk, exhibiting better prognoses.
Variations in prothrombin activity across different risk strata may be attributable to a secondary hypercoagulable state, frequently observed in patients with persistent PAH due to chronic endothelial damage. Increased shear stress and endothelial dysfunction can also lead to aberrant thrombin generation, further complicating the coagulation profile [18,19]. In this study, D-dimer levels were compared across different risk groups. Despite not being statistically significant, an upward trend in D-dimer levels was observed from low-risk to high-risk groups, particularly in the high-risk group. The lack of significant findings could be due to the small sample size and potential confounding factors such as comorbidities and therapeutic interventions. Future studies are needed to expand the sample size and improve control of confounding variables, ensuring a more precise elucidation of the correlation between D-dimer levels and risk stratification. NT-proBNP remains an important biomarker widely used in routine practice and clinical trials at PH centers, with previous studies confirming its effectiveness in risk stratification and predictive capabilities. Our findings are consistent with these results. In French studies, normal levels of NT-proBNP showed a sensitivity of 98% for excluding RAP > 8 mmHg and CI < 2.5 L/min/m2, or both conditions [8].
In this study, WHO-FC and 6MWD were ultimately not included in our model because their inclusion did not significantly enhance the predictive efficiency compared to the model we developed. This outcome may be influenced by a lower proportion of WHO-FC IV patients in our cohort and a higher rate of missing data for 6MWD. Nonetheless, the importance of WHO-FC in assessing quality of life remains critical. Moreover, 6MWD continues to be the most widely used exercise test in PH centers [4]. Echocardiography remains an essential diagnostic and follow-up tool in the management of PAH [20]. Right artial enlargement has been recognized in the pathophysiological process of PAH as a risk factor for adverse outcomes. Right atrial enlargement typically indicates elevated RAP, often resulting from functional tricuspid regurgitation or increased right ventricular diastolic pressure—both consequences of right ventricular failure. This enlargement can be quantitatively assessed through echocardiography, providing a non-invasive method to evaluate the severity of right heart failure. In our research, and similar to findings in the SPAHR study, the RAA was identified as a major indicator for risk stratification [21,22]. In our study, liver enzymes, commonly used as indicators of hepatic function, did not exhibit significant variations across different risk stratifications in patients with PAH-CHD. In contrast, albumin, a liver-synthesized protein and marker of nutritional status, showed a decrease, particularly in the high-risk group. The decreased albumin may reflect malnutrition or malabsorption caused by right heart failure inducing systemic congestion, both of which can impair liver function and reduce protein synthesis. Given the role of albumin in reflecting systemic health and its prognostic significance in PAH-CHD, further longitudinal studies are warranted to explore how changes in albumin levels correlate with disease progression and outcomes.
Our study was conducted at a single center, which may limit the generalizability of our findings across populations with different demographic or clinical characteristics. The retrospective design also introduced a risk of selection bias within the follow-up cohort. Moreover, comorbidities such as coronary artery disease and chronic obstructive pulmonary disease in older patients with CHD considerably influence the prognosis and management of PAH-CHD. Future research should aim for a more comprehensive evaluation of comorbidities to enhance our understanding of their impact on disease progression and patient outcomes.
The simplified risk stratification method is applicable to PAH-CHD patients. Prothrombin activity is a strong independent predictor of adverse outcomes. A comprehensive risk stratification approach, incorporating both established and novel biomarkers, enhances accessibility and provides predictive efficacy comparable to established models for PAH-CHD patients during follow-up.
Acknowledgement: None.
Funding Statement: This work was supported by the National Natural Science Foundation of China (82070052) and the Joint Funds of the Natural Science Foundation of Gansu Province (23JRRA1544) granted to Yunshan Cao.
Author Contributions: Yunshan Cao, Hongling Su designed the study, Wenjie Dong collected the data and wrote the draft of the manuscript. Zhibin Hong coordinated the study and participated in the study design; Yunshan Cao, Wenjie Dong performed the statistical analysis and revised the draft manuscript. Aqian Wang and Kaiyu Jiang collected the data and coordinated drafting of the manuscript. Hai Zhu, Fu Zhang, Zhaoxia Guo participated in this study. All authors reviewed the results and approved the final version of the manuscript.
Availability of Data and Materials: The datasets are not publicly available due to ethical considerations, though can be made available upon reasonable request.
Ethics Approval: The study was approved by the Ethics Committee of Gansu Provincial Hospital (Approval No. 2022-333) and the Ethics Committee of the First People’s Hospital of Tianshui (Approval No. 2024-07). Informed consent was not required due to the retrospective nature of the study.
Conflicts of Interest: The authors declare that they have no conflicts of interest to report regarding the present study.
Supplementary Materials: The supplementary material is available online at https://doi.org/10.32604/chd.2024.052267.
References
1. Humbert M, Kovacs G, Hoeper MM, Badagliacca R, Berger RMF, Brida M, et al. ESC/ERS guidelines for the diagnosis and treatment of pulmonary hypertension. Eur Heart J. 2022;43(38):3618–731, 2022. doi:10.1093/eurheartj/ehac237. [Google Scholar] [PubMed] [CrossRef]
2. Jone PN, Ivy DD, Hauck A, Karamlou T, Truong U, Coleman RD, et al. Pulmonary hypertension in congenital heart disease: a scientific statement from the american heart association. Circ: Heart Fail. 2023;16:e00080. [Google Scholar] [PubMed]
3. Humbert M, Sitbon O, Guignabert C, Savale L, Boucly A, Gallant-Dewavrin M, et al. Treatment of pulmonary arterial hypertension: recent progress and a look to the future. Lancet Respir Med. 2023;11(9):804–19. doi:10.1016/S2213-2600(23)00264-3. [Google Scholar] [PubMed] [CrossRef]
4. Leuchte HH, Ten Freyhaus H, Gall H, Halank M, Hoeper MM, Kaemmerer H, et al. Risk stratification strategy and assessment of disease progression in patients with pulmonary arterial hypertension: updated recommendations from the cologne consensus conference 2018. Int J Cardiol. 2018;272s:20–9. [Google Scholar] [PubMed]
5. Chen G, He J, Liu Z, Gu Q, Ni X, Xiong C. A comparative analysis of basic clinical features in patients with different types of pulmonary hypertension. Chin Circ J. 2013;28(4):300–3 (In Chinese). [Google Scholar]
6. Kylhammar D, Kjellström B, Hjalmarsson C, Jansson K, Nisell M, Söderberg S, et al. A comprehensive risk stratification at early follow-up determines prognosis in pulmonary arterial hypertension. Eur Heart J. 2018;39(47):4175–81. doi:10.1093/eurheartj/ehx257. [Google Scholar] [PubMed] [CrossRef]
7. Hoeper MM, Kramer T, Pan Z, Eichstaedt CA, Spiesshoefer J, Benjamin N, et al. Mortality in pulmonary arterial hypertension: prediction by the 2015 European pulmonary hypertension guidelines risk stratification model. Eur Respir J. 2017;50(2):1700740. doi:10.1183/13993003.00740-2017. [Google Scholar] [PubMed] [CrossRef]
8. Boucly A, Weatherald J, Savale L, Jaïs X, Cottin V, Prevot G, et al. Risk assessment, prognosis and guideline implementation in pulmonary arterial hypertension. Eur Respir J. 2017;50(2):1700889. doi:10.1183/13993003.00889-2017. [Google Scholar] [PubMed] [CrossRef]
9. Ferrero P, Constantine A, Chessa M, Dimopoulos K. Pulmonary arterial hypertension related to congenital heart disease with a left-to-right shunt: phenotypic spectrum and approach to management. Front Cardiovasc Med. 2024;11:1360555. doi:10.3389/fcvm.2024.1360555. [Google Scholar] [PubMed] [CrossRef]
10. Chemla D, Castelain V, Hervé P, Lecarpentier Y, Brimioulle S. Haemodynamic evaluation of pulmonary hypertension. Eur Respir J. 2002;20(5):1314–31. doi:10.1183/09031936.02.00068002. [Google Scholar] [PubMed] [CrossRef]
11. Deng X, Jin B, Li S, Li Y, Zhou H, Wu Y, et al. Guideline implementation and early risk assessment in pulmonary arterial hypertension associated with congenital heart disease: a retrospective cohort study. Clin Respir J. 2019;13(11):693–9. doi:10.1111/crj.v13.11. [Google Scholar] [CrossRef]
12. Jansen K, Constantine A, Condliffe R, Tulloh R, Clift P, Moledina S, et al. Pulmonary arterial hypertension in adults with congenital heart disease: markers of disease severity, management of advanced heart failure and transplantation. Expert Rev Cardiovasc Ther. 2021;19(9):837–55. doi:10.1080/14779072.2021.1977124. [Google Scholar] [PubMed] [CrossRef]
13. Shiina Y, Toyoda T, Kawasoe Y, Tateno S, Shirai T, Matsuo K, et al. The prevalence and risk factors for cholelithiasis and asymptomatic gallstones in adults with congenital heart disease. Int J Cardiol. 2011;152(2):171–6. doi:10.1016/j.ijcard.2010.07.011. [Google Scholar] [PubMed] [CrossRef]
14. Weaver H, Tulloh R. Thrombocytopenia in pulmonary hypertension, does it predict survival?. Eur Heart J. 2013;34(suppl_1):P318. [Google Scholar]
15. Ramjug S, Hussain N, Hurdman J, Billings C, Charalampopoulos A, Elliot CA, et al. Pulmonary arterial hypertension associated with congenital heart disease: comparison of clinical and anatomic-pathophysiologic classification. J Heart Lung Transpl Official Publ Int Soc Heart Transpl. 2016;35(5):610–8. doi:10.1016/j.healun.2015.12.016. [Google Scholar] [PubMed] [CrossRef]
16. Scognamiglio G, Kempny A, Price LC, Alonso-Gonzalez R, Marino P, Swan L, et al. C-reactive protein in adults with pulmonary arterial hypertension associated with congenital heart disease and its prognostic value. Heart. 2014;100(17):1335–41. doi:10.1136/heartjnl-2014-305494. [Google Scholar] [PubMed] [CrossRef]
17. Kempny A, Diller GP, Alonso-Gonzalez R, Uebing A, Rafiq I, Li W, et al. Hypoalbuminaemia predicts outcome in adult patients with congenital heart disease. Heart. 2015;101(9):699–705. doi:10.1136/heartjnl-2014-306970. [Google Scholar] [PubMed] [CrossRef]
18. Olsson KM, Delcroix M, Ghofrani HA, Tiede H, Huscher D, Speich R, et al. Anticoagulation and survival in pulmonary arterial hypertension: results from the comparative, prospective registry of newly initiated therapies for pulmonary hypertension (COMPERA). Circulation. 2014;129(1):57–65. doi:10.1161/CIRCULATIONAHA.113.004526. [Google Scholar] [PubMed] [CrossRef]
19. Cullivan S, Murphy CA, Weiss L, Comer SP, Kevane B, McCullagh B, et al. Platelets, extracellular vesicles and coagulation in pulmonary arterial hypertension. Pulm Circ. 2021;11(3):20458940211021036. [Google Scholar] [PubMed]
20. Hahn RT, Lerakis S, Delgado V, Addetia K, Burkhoff D, Muraru D, et al. Multimodality imaging of right heart function: JACC scientific statement. J Am Coll Cardiol. 2023;81(19):1954–73. [Google Scholar] [PubMed]
21. Guazzi M, Naeije R. Right heart phenotype in heart failure with preserved ejection fraction. Circ: Heart Fail. 2021;14:e007840. [Google Scholar] [PubMed]
22. Raymond RJ, Hinderliter AL, Willis PW, Ralph D, Caldwell EJ, Williams W, et al. Echocardiographic predictors of adverse outcomes in primary pulmonary hypertension. J Am Coll Cardiol. 2002;39(7):1214–9. doi:10.1016/S0735-1097(02)01744-8. [Google Scholar] [PubMed] [CrossRef]
Cite This Article
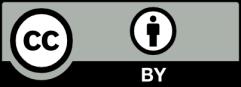
This work is licensed under a Creative Commons Attribution 4.0 International License , which permits unrestricted use, distribution, and reproduction in any medium, provided the original work is properly cited.