Open Access
CASE REPORT
Stubborn Hypoxia in Neonates with D-Transposition of the Great Arteries after Arterial Switch Operation: Central Sleep Apnea as the Cause and Potential Indicator of Brain Immaturity
1 Department of Pediatrics, Division of Pediatric Cardiology, University of Alabama Birmingham, Birmingham, 35233, USA
2 Department of Pediatrics, Division of Pediatric Pulmonology, University of Alabama Birmingham, Birmingham, 35233, USA
3 Department of Pediatrics, University of Alabama Birmingham, Birmingham, 35233, USA
4 Department of Surgery, Division of Cardiothoracic Surgery, University of Alabama Birmingham, Birmingham, 35233, USA
* Corresponding Author: Camden L. Hebson. Email:
Congenital Heart Disease 2024, 19(2), 185-195. https://doi.org/10.32604/chd.2024.048871
Received 20 December 2023; Accepted 21 February 2024; Issue published 16 May 2024
Abstract
D-transposition of the great arteries (d-TGA) is surgically repaired with the arterial switch operation (ASO) with excellent results, however short and long-term morbidities still develop including neurocognitive delay. Clinically significant central sleep apnea is uncommon in non-premature infants, but when present indicates immature autonomic control of respiration likely due to a neurologic disorder. We report the unanticipated finding of central sleep apnea in four-term neonates with d-TGA after uncomplicated ASO, with the short-term complication of delayed hospital discharge and long-term concerns regarding this early marker of brain immaturity and its hindrance to normal development. Within this report, we will review each patient’s clinical course and then examine the literature on pediatric central sleep apnea, neurodevelopmental outcomes after ASO, and the important overlap of these entities in the care of patients going forward.Keywords
D-transposition of the great arteries (d-TGA) is a cyanotic congenital heart lesion, accounting for ~3% of all congenital heart disease with an incidence of ~1:5000 live births [1,2]. Long-term results have become excellent over time due to the success of the arterial switch operation (ASO) [3,4]. As survival has improved, attention has shifted to identifying associated morbidities, in particular neurocognitive outcomes, as demonstrated by reporting of developmental delay, abnormal brain MRI findings, and social and behavioral deficits in these patients [5–8]. While evidence of long-term impairment accumulates, short-term examples of neurodevelopmental morbidity are important to describe, with the potential benefit of directing early intervention.
Over an 8-month period, four separate patients with d-TGA required prolongation of hospitalization after an uncomplicated arterial switch procedure due to the inability to wean from supplemental oxygen. These patients reflect the authors’ experience of this unexpected but not uncommon scenario after a seemingly successful surgery. Each patient was eventually found to have central sleep apnea, an unanticipated finding given their gestational age but perhaps instead evidence of brain immaturity (specifically of the medullary respiratory control centers) manifesting in the short-term. In this case series, we will describe these patients as well as the inferences that can be made from the presentations. This retrospective study was performed with the approval of the institutional review board at the University of Alabama Birmingham with waiver of informed consent.
2 A Primer on Pediatric Polysomnography
Overnight polysomnography (PSG, i.e., sleep study) is a diagnostic tool utilized to screen for sleep-related pathology and is the gold standard in identifying sleep-disordered breathing (SDB) including sleep apnea. A full pediatric PSG in an American Academy of Sleep Medicine-accredited laboratory includes monitoring of several physiologic parameters, including electroencephalogram, pulse-oximetry, end-tidal or transcutaneous CO2, electrocardiogram, electromyograms, airflow cannula, respiratory effort, and video/audio recording, in order to help characterize a patient’s sleep architecture and respiratory patterns. Respiratory events are reported as a total apnea-hypopnea index (AHI), which provides a general reflection on all types of apnea present as an average number of events per hour. The degree of airflow limitation determines whether a respiratory event is defined as a hypopnea (decrease in flow by 30% or more of the baseline) vs. an apnea (decrease by at least 90%). Events are further categorized as central apnea if there is the absence of respiratory effort, the event lasts at least the duration of 2 breaths or ≥20 s, and finally, there is an accompanying ≥3% arterial oxygen desaturation and/or an arousal from sleep [9]. While studies investigating normative data demonstrate some baseline degree of central sleep apnea in pediatric patients [10,11], there is no consensus to define a pathologic central apneic index. Oxygen supplementation via nasal cannula is utilized as first-line therapy in affected infants, with consideration for additional positive airway pressure in the most severe cases.
Patient 1 was born at 41 weeks gestation in January 2023 and had a post-natal diagnosis of d-TGA with intact ventricular septum. After birth, he had severe cyanosis and required urgent balloon atrial septostomy due to hypoxia and acidosis (pre-procedure pH 7.28, PaO2 37 mmHg, lactate 3.5 mmol/L). He underwent surgery on the day of life (DOL) 6 with an ASO including the LeCompte maneuver. He had no major complications and was transferred to the cardiac step-down unit (CSU) on DOL 10. He did well thereafter, tolerating full feedings by mouth and weaning to typical medications for home including a daily dose of furosemide. Post-operative echocardiograms showed no concerning findings after the ASO, specifically no significant branch pulmonary artery stenosis, residual atrial level shunting, neoaortic valve insufficiency, or evidence of elevated right ventricular systolic pressure. He continued to require nasal cannula (NC) oxygen to prevent hypoxia, however. Oxygen saturations off supplemental oxygen were 80%–85%. Chest radiography was reassuring and clinically there were no signs of respiratory insufficiency. Fig. 1 shows the post-operative electrocardiogram and chest radiography.
Figure 1: Post-operative electrocardiogram and chest radiograph of patient 1. Findings include normal sinus rhythm with normal intervals and repolarization, as well as no radiographic signs of pleural effusion or significant intrapulmonary process to explain hypoxia
No clinical signs of aspiration were present. Instead, lower oxygen saturations were often noted by bedside nursing during naps and overnight. Eventually, a sleep study was performed on DOL 14. Findings included:
The total sleep time was 283 min, with 41 hypopneas, 1 obstructive apnea, and 28 central apneas. The total Apnea-Hypopnea Index (AHI) for the study was 14.8 events/h with a central apnea index of 5.9 apneas/h. Prior to the initiation of supplemental oxygen, O2 saturation nadir was 85% with a total of 1.9 min spent with saturations less than 88%. Supplemental oxygen was initiated and uptitrated to a final level of 1 L nasal cannula (NC). Sleep architecture was fragmented as a consequence of respiratory events.
Due to the extent of central apnea present, a brain MRI was completed, which showed no obvious causes for apnea or significantly abnormal findings. He was discharged home on 1 L NC. Unfortunately, subsequent cardiac evaluations are not well known as his follow-up has been with an unaffiliated cardiologist. He has followed up once in a pediatric sleep medicine clinic, where the parents informed the sleep physician that they had weaned off his oxygen on their own while monitoring his oxygen saturation on home pulse oximetry. The family did not desire a repeat sleep study. The risks of untreated sleep apnea and fragmented sleep were discussed with the family who still elected to forgo further sleep testing and instead monitor for developmental delay with their pediatrician.
Patient 2 was born at 40 weeks gestation in February 2023 and had a post-natal diagnosis of d-TGA. During feedings at his birth hospital, he was noted to become cyanotic with pulse oximetry confirming oxygen saturations as low as 60%. He was transferred to our hospital and an echocardiogram confirmed the diagnosis of d-TGA with intact ventricular septum. He underwent an ASO with LeCompte maneuver on DOL 4. He did well post-operatively with no major complications and was transferred to the CSU on DOL 7. While he had no concerning findings on his subsequent echocardiograms and successfully transitioned to all feedings by mouth, he remained hypoxic when weaned from the nasal cannula. Fig. 2 demonstrates the pre- and post-operative echocardiographic imaging.
Figure 2: Pre- and post-operative echocardiographic imaging of patient 2. Panel 2A shows parasternal long-axis imaging demonstrating the parallel orientation of the great vessels with the posterior branching vessel being the pulmonary artery. Panel 2B shows the anterior aorta and posterior pulmonary artery, with the right coronary artery arising from and identifying the aorta. Panel 2C shows the characteristic relationship of the great vessels after the LeCompte maneuver with the branch pulmonary arteries straddling the ascending aorta. Panel 2D shows the atrial septum with no residual right to left shunt after patent foramen ovale suture closure
Oxygen saturations were typically in the 80%–85% range on room air but without associated signs of respiratory insufficiency or significant abnormalities on chest radiography. A sleep study was performed on DOL 12, which showed:
Total sleep time was 317 min with fragmented sleep present. There were 4 central apneas, 1 obstructive apnea, and 9 hypopneas. The overall AHI was 2.6, however during the diagnostic portion of the study, prior to initiation of oxygen, AHI was 10.6 and nadir SpO2 was 85%. Oxygen was initiated at 0.125 L NC and AHI was 1.7 and nadir SpO2 93%.
The patient was therefore discharged on 0.125 L NC, which kept his oxygen saturation >94%. No brain MRI was done, although the head ultrasound did not show significant bleeding or gross structural abnormalities. Subsequent follow-up in the cardiology clinic was reassuring-the family reported that even with unsanctioned times of leaving him off of supplemental oxygen, his saturations persistently were >92%. Surprisingly, however, a repeat sleep study done at 3 months of age continued to show abnormal findings, with 27 central apneas and 10 hypopneas during 449 min of sleep time, generating an AHI of 4.9 and a central apnea index of 3.6 apneas/h. The study was done on room air and then 0.125 L NC. The oxygen saturation nadir was 86% and it was decided to keep the same oxygen supplementation post-study. A repeat sleep study is scheduled for December 2023.
Patient 3 was born at 38 weeks gestation in May 2023. He was prenatally diagnosed with d-TGA as well as heterotaxy syndrome, left atrial isomerism. He was born via C-section due to placenta previa. Initial oxygen saturations after birth were 50%–55% and an echocardiogram confirmed the diagnoses of left atrial isomerism, d-TGA with an intact ventricular septum, and additionally a restrictive patent foramen ovale/atrial level shunt. He underwent a non-urgent balloon atrial septostomy for hypoxia but no acidosis soon after birth with improvement in oxygen saturation. He eventually underwent the ASO procedure with LeCompte maneuver on DOL 7. Given his heterotaxy and interrupted IVC, standard bicaval cannulation was not adequate for cardiac decompression and thus a single RA cannula was used. Therefore, the decision was made to cool to 22 degrees Celsius to use low flow cardiopulmonary bypass to close the ASD. Circulatory arrest was not utilized, however. Post-operatively, he has some atrial ectopy that was treated with propranolol. He otherwise did well and was transferred to the CSU on DOL 13. He did well thereafter with reassuring echocardiographic findings and achievement of full feedings by mouth. An upper GI and small bowel radiography series confirmed a diagnosis of malrotation and pediatric surgery follow-up was arranged. He had recalcitrant hypoxia with weaning of NC (oxygen saturation 80%–85%). A sleep study was done on DOL 19, which showed:
The total sleep time on room air was 117 min and sleep architecture was fragmented. There were 3 central apneas, 1 obstructive apnea, and 3 hypopneas, with an AHI of 3.6 and a central apnea index of 1.5 events/h. On 0.125 L NC, AHI improved to 0.3. The lowest oxygen saturation during testing was 87%.
Therefore, 0.125 L NC was recommended for discharge home. No brain MRI was done, although the head ultrasound was negative for significant bleeding or gross anatomic abnormalities. He was discharged home on DOL 21. He continued to require propranolol at the time of discharge as well as amoxicillin prophylaxis and a daily dose of furosemide. In follow-up, he underwent a Ladd’s procedure for malrotation and did well without complications or prolonged hospital stay. Cardiac evaluations in the clinic have been reassuring with no concerning findings on echocardiography or Holter monitoring. In follow-up with pediatric pulmonology at 2 months of age, oxygen was discontinued after parent reports of normal saturations with self-weaning of oxygen at home. No repeat sleep study was done due to family and provider preferences.
Patient 4 was born at 38 weeks gestation in August 2023. He was postnatally diagnosed with d-TGA with an intact ventricular septum. His pre-operative course was uncomplicated and he underwent ASO with LeCompte maneuver on DOL 5. Post-operatively, he had brief runs of supraventricular tachycardia that were treated with esmolol and then propranolol at the time of discharge. He was transferred to the CSU on DOL 9 and progressed to full feedings by mouth, and both echocardiogram and chest radiography showed excellent post-operative results. He remained hypoxic, with saturations in the low 80% range of oxygen, however, he was treated with 0.125 L NC oxygen. He was eventually discharged on DOL 16 and a sleep study was scheduled to be done as an outpatient due to the unavailability inpatient. This was eventually done on DOL 32 and showed:
During 369 min of sleep, the patient’s sleep architecture was considered “highly” fragmented. On room air, the patient was found to have 67 central apneas, 0 obstructive apneas, and 25 hypopneas. The AHI was 15 events/h and the central apnea index was 11.6 events/h. On 0.125 L NC, AHI improved to 0.3. The lowest oxygen saturation during testing was 85%. Fig. 3 shows a sample of the polysomnography data.
Figure 3: Snapshot from the diagnostic polysomnogram for patient 4. The patient demonstrates central apnea, characterized by cessation of airflow and respiratory effort with accompanying oxygen desaturation
Therefore, 0.125 L NC was recommended for discharge home. A brain MRI was performed, which showed no intracranial or brainstem abnormalities. He will undergo a repeat sleep study at 3 months of age. Cardiac evaluations in the clinic have been reassuring with no concerning findings on echocardiography specifically.
A summary of the clinical and sleep study results is presented in Table 1.
Neurocognitive outcomes in patients with d-TGA have become a particular focus in the field of pediatric cardiology [12,13]. Our case series highlights an early potential manifestation of brain immaturity (specifically central respiratory control) in these patients and is important both in short-term management and long-term monitoring. This is the first study to describe sleep-disordered breathing specifically in post-operative d-TGA as a clinically significant comorbidity.
Identifying central sleep apnea in the post-operative patient is important for several reasons. First, this diagnosis directs treatment of resulting hypoxia with supplemental oxygen, thus allowing earlier discharge from the hospital on proper management rather than inexpedient attempts at oxygen weaning. Secondly (and practically), the diagnosis helps justify the need for home oxygen over a period of time to insurance or other payers. Third, and as discussed below, patients with sleep apnea are at risk for developmental delay, in particular, if fragmented sleep patterns are identified, and thus providers can use these findings to prompt referral to early interventional services as well as repeat sleep studies over time to gauge progress. Finally, there is a paucity of medical literature on sleep-disordered breathing (SDB) in infants after congenital heart surgery. This said the rare report provides insight. In a retrospective database study of hospitalized infants with congenital heart disease, authors found an association between central sleep apnea and mortality, hospital length of stay, and total charges [14]. Further study of this phenomenon helps not only the care of the individual d-TGA patient but also knowledge development in our field.
4.1 Neurocognitive Outcomes in d-TGA Patients
During the fetal period, patients with d-TGA are exposed to significant cerebral hypoxia as a result of the cardiac anatomy. Normal fetal cerebral arterial oxygen saturations are >80%, whereas authors have shown fetal d-TGA cerebral saturations are more typically 40%–50% [15]. Furthermore, the early postnatal weeks of life mark a period of rapid brain growth and development, which makes this a vulnerable period for any consequences of cerebral hypoxia. While the anatomy is corrected with the ASO, authors have noted long-term neurocognitive delays in d-TGA patients likely resulting from the fetal and perioperative pathophysiology that was present.
When compared with their healthy gestational-age equivalents (utilizing amplitude-integrated electroencephalography and comparisons of sleep-wake cycles), pre-operative neonates with d-TGA have significant delays in their functional brain age [16]. Anatomically, cyanotic congenital heart patients have been shown to have restrictions in fetal brain growth as well [17]. Inadequate active sleep in d-TGA neonates might be a partial explanation for these findings, as active sleep is vital for brain growth in neonates and the higher metabolic oxygen demand of active sleep might not be adequately met in patients with d-TGA physiology [16,18]. In addition to short-term findings, authors have shown the delay in functional brain age in d-TGA neonates significantly correlates with longer-term motor delay, as measured by the Bayley Scales of Infants Development Version III (BSID-III) at 24 months of age [16]. As an explanation for the motor delay in particular, patients with d-TGA have been shown to have a predilection for white matter injury, similar to the periventricular leukomalacia seen in premature infants. This is believed to be a result of hypoxic injury to immature premyelinating glial cells. Brain growth and total brain volume have also been shown to be decreased in patients with d-TGA, in close relation to the extent of white matter injury present [8]. Authors have therefore sought to mitigate these results, with emphasis on prenatal diagnosis to facilitate expedited care and timing of ASO being points of emphasis [7,19]. Three of our patients were post-natally diagnosed (receiving only standard obstetric ultrasounds and not a fetal echocardiogram) and one of these required urgent atrial septostomy–this acute presentation could have contributed to the immediate neurologic presentation. As for the timing of ASO, Lim et al. found that neonates with d-TGA who underwent ASO at less than 2 weeks of age had significantly better perioperative brain growth when compared with infants repaired after 2 weeks [7]. When comparing these infants at 18 months of age, age at repair and days of open chest had a statistically significant association with a lower composite language score. Furthermore, hospital length of stay was associated with a lower composite cognitive score [7]. This is a particularly interesting finding when considering our case series–how many of these children had prolonged length of stay due to recalcitrant hypoxia, and how many of these might have had central sleep apnea as the underlying cause?
4.2 Pediatric Sleep Disordered Breathing (SDB) and Central Sleep Apnea
Primary control of breathing in humans is housed within the medullary respiratory centers, which are influenced by central and peripheral chemoreceptors. Medullary central chemoreceptors sense changes in the partial pressure of arterial CO2 (PaCO2) and pH. Peripheral chemoreceptors are located in the aortic and carotid bodies where they additionally sense changes in partial pressure of arterial O2 (PaO2). Detection of PaCO2 above baseline or a decrease in the PaO2 and pH will result in an increased respiratory rate; likewise, respiratory rate will diminish in response to a fall in PaCO2 or elevation of arterial pH. Immaturity of this feedback pathway in infancy results in an exaggerated respiratory response to changes in PaCO2 and pH, leading to hypocapnea and resultant central apneic events, a concept referred to as a high loop gain [20]. This is the physiology behind periodic breathing, which improves with the growth and development of a child as the feedback pathways mature. Additionally, patients with severe heart failure may develop Cheyne-Stokes respirations, characterized by central apneic events interspersed with a crescendo/decrescendo breathing pattern. This is a consequence of disruption of the natural loop gain arising from a timing delay between the pulmonary artery and systemic arterial chemoreceptors (due to poor output from the systemic ventricle). Although our patients did not have severe heart failure to explain the central apnea found as Cheyne-Stokes respirations, it is noteworthy instead that d-TGA patients uniquely have more elevated pulmonary arterial saturations compared to carotid saturations prior to their repair. What if any implications this has on the pathophysiology of central apnea is unclear, but the fact that differences in these saturations play a role in other cardiac conditions is intriguing. The specific differential diagnosis of central sleep apneas in children includes apnea of prematurity, periodic breathing, genetic conditions such as congenital central hypoventilation syndrome, Chiari malformations and brainstem tumors, and CNS depressant medications. Apnea of prematurity is a consequence of decreased responsiveness to central and peripheral chemoreceptors, which improves as the feedback loop and oxygen saturations improve [21,22]. We speculate that immature and delayed responsiveness in d-TGA patients might also arise as a consequence of exposure to hypoxia prior to and during repair. This point is particularly important when considering patients 1 and 3. Patient 1 was not a prenatal diagnosis and had a more unstable prenatal course with an urgent Rashkind procedure required. This additional insult could have contributed to his presentation and highlighted the need for excellent prenatal evaluation [19]. As for patient 3, his venous anatomy necessitated RA cannulation and lower core temperature during the operation, which does increase the risk of neurologic injury. This must be considered when evaluating him over time and considering all the causes of developmental delay detected during follow-up.
Though there is a dearth of literature on cognitive outcomes in children with central sleep apnea, numerous studies have explored the impact of obstructive sleep apnea (OSA) [23–25]. Li et al. demonstrated that affected children had lower cognitive testing scores and impaired short-term recall [23]. When comparing children with sleep-disordered breathing (SDB) to peers, those with SDB scored significantly lower on executive functioning, memory, and general intellectual ability [24]. Lower IQ scores, math performance, and short-term recall have also been reported in those with AHI > 5 compared to non-affected children [25]. Each patient in our study was also noted to have sleep fragmentation. Recurrent sleep arousal in patients with sleep apnea is the cause of fragmentation of normal sleep architecture. Sleep fragmentation then leads to decreased deep and rapid eye movement (REM) sleep, with important implications–as examples, growth hormone release and memory consolidation occur in particular during these stages. Although the mechanisms underlying the association between SDB and cognitive impairment remain unclear, authors have postulated that sleep fragmentation and intermittent nocturnal hypoxemia might lead to neurovascular inflammation [26].
The limitations of our report include those inherent to any retrospective analysis. We describe the association of central apnea in post-operative d-TGA patients, but can only speculate as to the cause(s), and even then, we cannot be certain of causality. Further studies with more robust designs will be needed to bridge this knowledge gap. Additionally, our case series does not contain a control cohort of infants without congenital heart disease or even other types of congenital heart disease in order to determine how specific our findings are for d-TGA. We hope to address this as a future direction. We also plan to follow these infants over time to observe if developmental delay does indeed occur–this will help with validating the observations made in this study and of course, provide the patients with the care they need. Another concern is that while hypoxia in our post-operative d-TGA patients is ascribed to hypoventilation due to central sleep apnea, other factors could have contributed. We do believe this is unlikely based on the clinical teams’ evaluations at the time including reassuring chest radiography and echocardiography. Finally, in terms of completeness of evaluation of the patients, two patients have not received a brain MRI to date and thus we are unable to rule out anatomic central neurologic causes of SDB in these patients.
We recommend close observation of d-TGA patients post-operatively after ASO for hypoxia disproportionate to the patient’s clinical status. In addition to typical measures to address post-operative hypoxia, consideration for a sleep study to identify central apnea facilitates both earlier discharge and ongoing treatment at home. In these patients at risk for developmental delay, identification of central apnea, SDB, and fragmented sleep also alert physicians and families to their particular risk for delay, with treatment including physical, occupational, and speech therapy being possible. As physicians caring for these patients, our mandate is not just to correct the cardiac lesion but instead to care for the complete individual by identifying and then treating all the issues that can arise as a result of their cardiac status. Central apnea should be added to the list of potential co-morbidities in patients with d-TGA.
Acknowledgement: None.
Funding Statement: The authors received no specific funding for this study.
Author Contributions: The authors confirm their contribution to the paper as follows: Camden Hebson: study conception and design. Kyle Bliton: sleep data collection, manuscript review and editing. Amr Hammouda, Kaitlyn Barr: literature review and manuscript preparation. W. Hampton Gray, Mohini Gunnett: manuscript review and editing. Waldemar F. Carlo: senior author, manuscript review and editing. All authors reviewed the results and approved the final version of the manuscript.
Availability of Data and Materials: The data used and/or analysed during the current study are available from the corresponding author on reasonable request.
Ethics Approval: No Ethics Committee approval was obtained for this study. University of Alabama Birmingham Institutional Review Board approval was obtained, IRB Project Number 300012208.
Conflicts of Interest: The authors declare that they have no conflicts of interest to report regarding the present study.
References
1. Reller MD, Strickland MJ, Riehle-Colarusso T, Mahle WT, Correa A. Prevalence of congenital heart defects in metropolitan Atlanta, 1998–2005. J Pediatr. 2008;153(6):807–13. doi:10.1016/j.jpeds.2008.05.059. [Google Scholar] [PubMed] [CrossRef]
2. Samánek M. Congenital heart malformations: prevalence, severity, survival, and quality of life. Cardiol Young. 2000;10(3):179–85. doi:10.1017/S1047951100009082. [Google Scholar] [PubMed] [CrossRef]
3. Broberg CS, van Dissel A, Minnier J, Aboulhosn J, Kauling RM, Ginde S, et al. Long-term outcomes after atrial switch operation for transposition of the great arteries. J Am Coll Cardiol. 2022;80(10):951–63. doi:10.1016/j.jacc.2022.06.020. [Google Scholar] [PubMed] [CrossRef]
4. Ortinau CM, Smyser CD, Arthur L, Gordon EE, Heydarian HC, Wolovits J, et al. Optimizing neurodevelopmental outcomes in neonates with congenital heart disease. Pediatr. 2022;150(Suppl 2):e2022056415L. [Google Scholar]
5. Gaynor JW, Stopp C, Wypij D, Andropoulos DB, Atallah J, Atz AM, et al. Impact of operative and postoperative factors on neurodevelopmental outcomes after cardiac operations. Ann Thorac Surg. 2016;102(3):843–9. doi:10.1016/j.athoracsur.2016.05.081. [Google Scholar] [PubMed] [CrossRef]
6. Kordopati-Zilou K, Sergentanis T, Pervanidou P, Sofianou-Petraki D, Panoulis K, Vlahos N, et al. Dextro-transposition of great arteries and neurodevelopmental outcomes: a review of the literature. Children. 2022;9(4):502. doi:10.3390/children9040502. [Google Scholar] [PubMed] [CrossRef]
7. Lim JM, Porayette P, Marini D, Chau V, Au-Young SH, Saini A, et al. Associations between age at arterial switch operation, brain growth, and development in infants with transposition of the great arteries. Circ. 2019;139(24):2728–38. doi:10.1161/CIRCULATIONAHA.118.037495. [Google Scholar] [PubMed] [CrossRef]
8. Peyvandi S, Lim JM, Marini D, Xu D, Reddy VM, Barkovich AJ, et al. Fetal brain growth and risk of postnatal white matter injury in critical congenital heart disease. J Thorac Cardiovasc Surg. 2021;162(3):1007–14.e1. doi:10.1016/j.jtcvs.2020.09.096. [Google Scholar] [PubMed] [CrossRef]
9. Troester MM, Quan SF, Berry RB. The American Academy of Sleep Medicine manual for the scoring of sleep and associated events: rules, terminology and technical specifications. 3rd edDarien, IL: American Academy of Sleep Medicine; 2023. [Google Scholar]
10. Brockmann PE, Poets A, Poets CF. Reference values for respiratory events in overnight polygraphy from infants aged 1 and 3 months. Sleep Med. 2013;14(12):1323–7. doi:10.1016/j.sleep.2013.07.016. [Google Scholar] [PubMed] [CrossRef]
11. Daftary AS, Jalou HE, Shively L, Slaven JE, Davis SD. Polysomnography reference values in healthy newborns. J Clin Sleep Med. 2019;15(3):437–43. doi:10.5664/jcsm.7670. [Google Scholar] [PubMed] [CrossRef]
12. Bellinger DC, Wypij D, duPlessis AJ, Rappaport LA, Jonas RA, Wernovsky G, et al. Neurodevelopmental status at eight years in children with dextro-transposition of the great arteries: the Boston Circulatory Arrest Trial. J Thorac Cardiovasc Surg. 2003;126(5):1385–96. doi:10.1016/S0022-5223(03)00711-6. [Google Scholar] [PubMed] [CrossRef]
13. Rivkin MJ, Watson CG, Scoppettuolo LA, Wypij D, Vajapeyam S, Bellinger DC, et al. Adolescents with D-transposition of the great arteries repaired in early infancy demonstrate reduced white matter microstructure associated with clinical risk factors. J Thorac Cardiovasc Surg. 2013;146(3):543–9.e1. doi:10.1016/j.jtcvs.2012.12.006. [Google Scholar] [PubMed] [CrossRef]
14. Combs D, Skrepnek G, Seckeler MD, Barber BJ, Morgan WJ, Parthasarathy S. Sleep-disordered breathing is associated with increased mortality in hospitalized infants with congenital heart disease. J Clin Sleep Med. 2018;14(9):1551–8. doi:10.5664/jcsm.7334. [Google Scholar] [PubMed] [CrossRef]
15. Sakazaki S, Masutani S, Sugimoto M, Tamura M, Kuwata S, Kurishima C, et al. Oxygen supply to the fetal cerebral circulation in hypoplastic left heart syndrome: a simulation study based on the theoretical models of fetal circulation. Pediatr Cardiol. 2015;36(3):677–84. doi:10.1007/s00246-014-1064-6. [Google Scholar] [PubMed] [CrossRef]
16. Hermans T, Thewissen L, Gewillig M, Cools B, Jansen K, Pillay K, et al. Functional brain maturation and sleep organisation in neonates with congenital heart disease. Eur J Paediatr Neurol. 2022;36:115–22. doi:10.1016/j.ejpn.2021.12.008. [Google Scholar] [PubMed] [CrossRef]
17. Inversetti A, Fesslova V, Deprest J, Candiani M, Giorgione V, Cavoretto P. Prenatal growth in fetuses with isolated cyanotic and non-cyanotic congenital heart defects. Fetal Diagn Ther. 2020;47(5):411–9. doi:10.1159/000493938. [Google Scholar] [PubMed] [CrossRef]
18. Sun L, Macgowan CK, Sled JG, Yoo SJ, Manlhiot C, Porayette P, et al. Reduced fetal cerebral oxygen consumption is associated with smaller brain size in fetuses with congenital heart disease. Circ. 2015;131(15):1313–23. doi:10.1161/CIRCULATIONAHA.114.013051. [Google Scholar] [PubMed] [CrossRef]
19. Quaresima P, Fesslova V, Farina A, Kagan KO, Candiani M, Morelli M, et al. How to do a fetal cardiac scan. Arch Gynecol Obstet. 2023;307(4):1269–76. doi:10.1007/s00404-023-06951-8. [Google Scholar] [PubMed] [CrossRef]
20. Deacon-Diaz N, Malhotra A. Inherent vs. induced loop gain abnormalities in obstructive sleep apnea. Front Neurol. 2018;9:896. doi:10.3389/fneur.2018.00896. [Google Scholar] [PubMed] [CrossRef]
21. Erickson G, Dobson NR, Hunt CE. Immature control of breathing and apnea of prematurity: the known and unknown. J Perinatol. 2021;41(9):2111–23. doi:10.1038/s41372-021-01010-z. [Google Scholar] [PubMed] [CrossRef]
22. Darnall RA. The role of CO2 and central chemoreception in the control of breathing in the fetus and the neonate. Respir Physiol Neurobiol. 2010;173(3):201–12. doi:10.1016/j.resp.2010.04.009. [Google Scholar] [PubMed] [CrossRef]
23. Li H, Chen L, Wu X, Zhu F, Bing X, Shi L, et al. The effects of obstructive sleep apnea-hypopnea syndrome (OSAHS) on learn and memory function of 6–12 years old children. Int J Pediatr Otorhinolaryngol. 2022;159:111194. doi:10.1016/j.ijporl.2022.111194. [Google Scholar] [PubMed] [CrossRef]
24. Gottlieb DJ, Chase C, Vezina RM, Heeren TC, Corwin MJ, Auerbach SH, et al. Sleep-disordered breathing symptoms are associated with poorer cognitive function in 5-year-old children. J Pediatr. 2004;145(4):458–64. doi:10.1016/j.jpeds.2004.05.039. [Google Scholar] [PubMed] [CrossRef]
25. Kaemingk KL, Pasvogel AE, Goodwin JL, Mulvaney SA, Martinez F, Enright PL, et al. Learning in children and sleep disordered breathing: findings of the tucson children’s assessment of sleep apnea (tuCASA) prospective cohort study. J Int Neuropsychol Soc. 2003;9(7):1016–26. doi:10.1017/S1355617703970056. [Google Scholar] [PubMed] [CrossRef]
26. Trosman I, Trosman SJ. Cognitive and behavioral consequences of sleep disordered breathing in children. Med Sci. 2017;5(4):30. [Google Scholar]
Cite This Article
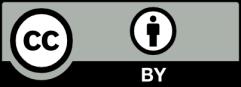