Open Access
ARTICLE
Maternal Vascular Dysfunction in Congenital Heart Defects
1 Department of Obstetrics and Gynecology, Nanfang Hospital, Southern Medical University, Guangzhou, 510515, China
2 Department of Obstetrics and Gynecology, Guangdong Provincial People’s Hospital (Guangdong Academy of Medical Sciences), Southern Medical University, Guangzhou, 510080, China
3 Prenatal Diagnosis Centre, Guangdong Provincial People’s Hospital (Guangdong Academy of Medical Sciences), Southern Medical University, Guangzhou, 510080, China
4 Department of Cardiovascular Surgery, Guangdong Cardiovascular Institute, Guangdong Provincial People’s Hospital (Guangdong Academy of Medical Sciences), Southern Medical University, Guangzhou, 510080, China
5 Global Health Research Center, Guangdong Cardiovascular Institute, Guangdong Provincial People’s Hospital (Guangdong Academy of Medical Sciences), Southern Medical University, Guangzhou, 510080, China
* Corresponding Authors: Haiping Wang. Email: ; Liping Huang. Email:
Congenital Heart Disease 2023, 18(5), 561-570. https://doi.org/10.32604/chd.2023.030511
Received 10 April 2023; Accepted 07 August 2023; Issue published 10 November 2023
Abstract
Background: Research on fetal congenital heart defect (CHD) mostly focuses on etiology and mechanisms. However, studies on maternal complications or pathophysiology are limited. Our objective was to determine whether vascular dysfunction exists in pregnant women carrying a fetus with congenital heart defects. Methods: We conducted a case-control study. 27 cases of pregnant women carrying a fetus with major CHD admitted to our hospital for delivery between April 2021 and August 2022 were selected. Every case was matched with about 2 pregnant complication-free controls without fetal abnormalities. The proangiogenic and anti-angiogenic factors and pregnancy outcomes were compared. Results: The proangiogenic factors include vascular endothelial growth factor (VEGF) and placental growth factor (PlGF). The anti-angiogenic factors involve soluble fms-like tyrosine kinase 1 (sFlt-1) and soluble endoglin (sEng). No differences were found in maternal plasma concentrations of PlGF, VEGF, and sFlt-1 between case-control groups when analyzed at 36 weeks ≤ gestational age (GA) < 39 weeks and 39 weeks ≤ GA ≤ 41 weeks. The concentrations of sEng in maternal plasma in the fetal CHD group were significantly higher than those in the control group: 0.60 (0.77) vs. 0.32 (0.26) ng/ml at 36 weeks ≤ GA < 39 weeks, p = 0.001 and 0.75 (0.55) vs. 0.28 (0.27) ng/ml at 39 weeks ≤ GA ≤ 41 weeks, p < 0.001. Conclusion: Vascular dysfunction exists in pregnant women with fetal congenital heart defects, manifesting significantly elevated sEng concentration at delivery.Graphical Abstract
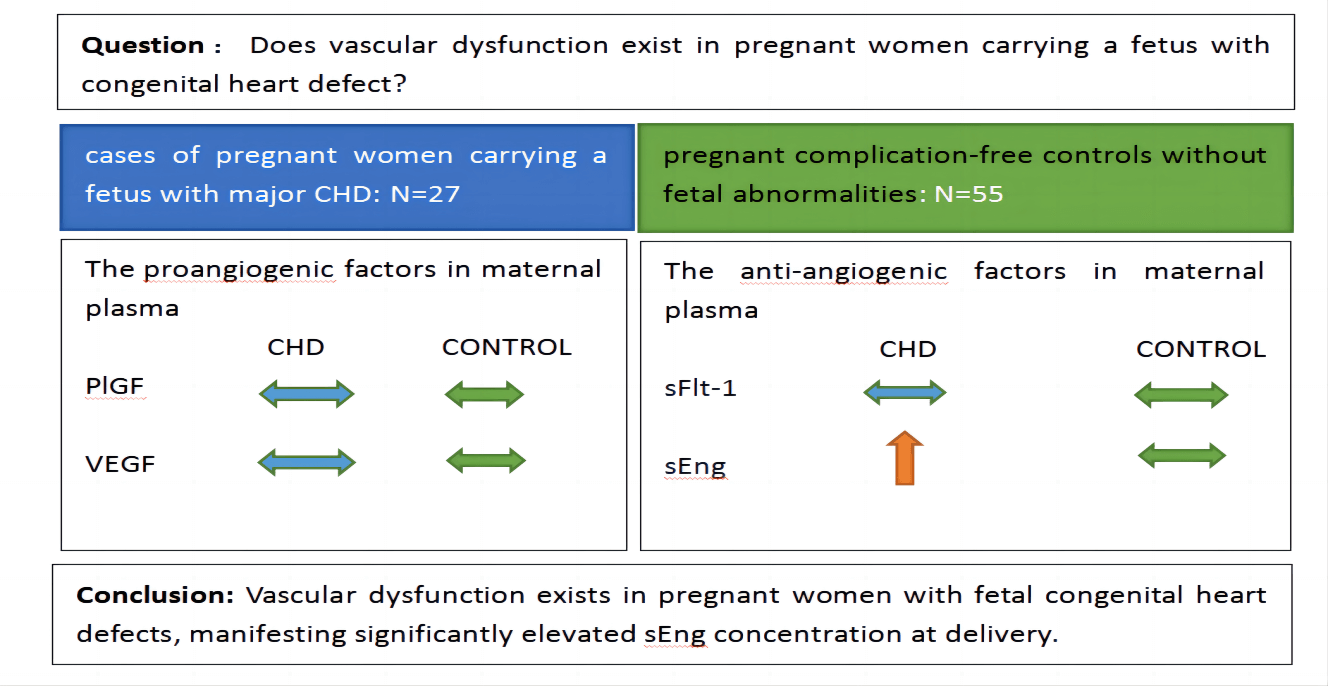
Keywords
Congenital heart defects (CHDs) are the most common birth defects, with a prevalence of 0.8% in the general population [1]. The heart and great vessels are the major abnormalities, accounting for about 20% of all stillbirths and 30% of neonatal deaths [2]. Approximately half of the cases usually require one or more surgical procedures in the neonatal period or during childhood, causing a significant burden not only to CHD families but also to society [3]. Although significant advances have been achieved in the mechanisms of determining heart formation, the causes and risk factors for CHD in humans still remain unknown [4].
Research on fetal CHD mostly focuses on etiology and mechanisms. However, studies on maternal complications or pathophysiology are limited. Llurba and his colleagues first reported that maternal proangiogenic imbalance was associated with fetal congenital heart defects. These proangiogenic factors include vascular endothelial growth factor (VEGF) and placental growth factor (PlGF) [5]. The anti-angiogenic factors consist of soluble fms-like tyrosine kinase 1 (sFlt-1) and endoglin (sEng). They revealed that, in isolated critical fetal heart defects, maternal serum PlGF declined and sFlt-1 elevated at 18–37 weeks of gestation, indicating damaged placental angiogenesis. But no differences were found in sEng. These same factors are abnormally altered in preeclampsia, a pregnancy-induced disorder characterized by hypertension and proteinuria developing after 20 weeks of gestation in pregnant women who were previously normotensive. Excess soluble endoglin and sFlt-1 relative to PlGF and VEGF were noted in preeclampsia [6]. Two years later, Nathalie Auger and his colleagues conducted a population-based investigation involving 1,942,072 neonates and proved that preeclampsia was significantly associated with heart defects in offspring [7]. These studies indicated that there is a correlation between maternal preeclampsia and fetal CHD and they may have a shared pathway.
Since preeclampsia is a systemic vascular disease characterized by endothelial dysfunction [8], we hypothesize that there is also a vascular dysfunction in pregnant women carrying a fetus with CHD. Although Llurba et al. have described the maternal imbalanced angiogenic factors in pregnant women with fetal CHD, the range of the study gestational ages was broad (18–37 weeks of gestation) and they did not illustrate whether the maternal vascular dysfunction extended to the delivery time. To solve the above problem, we designed this case-control study. Firstly, we tested the proangiogenic and anti-angiogenic factors in maternal plasma in case-control groups at delivery and then analyzed the correlations between these factors and maternal or fatal clinical characteristics.
This was a case-control study. We enrolled all pregnant women admitted to the Obstetrical Department of Guangdong Provincial People’s Hospital between April 2021 and August 2022 for fetus cardiac defect. The inclusion criteria for the case group were: (1) singleton pregnancy with gestational age between 36 and 41 weeks; (2) fetus was examined by a fetal cardiologist and confirmed to have a major congenital defect after birth. The exclusion criteria contained: (1) fetus with other structural defects determined by prenatal ultrasound screening; (2) fetal growth restriction, defined by low birth weight (<2500 g) (3) aberrant Doppler result of umbilical artery before delivery; (4) fetuses with genetic abnormalities; (5) with maternal disease, such as preeclampsia, diabetes, intrahepatic cholestasis of pregnancy, heart disease, renal disease, liver disease and hemopathy. Every case was matched with two healthy pregnant controls whose neonates have normal phenotypes. At last, 27 cases of pregnant women carrying a fetus with critical CHD and 55 controls were selected. The flowchart is shown in Fig. 1.
Figure 1: Flowchart of subjects selection
Maternal venous blood was drawn one day before delivery or at delivery and processed within 1 h. Plasma was separated by centrifugation at 3000 rpm for 15 min at 4°C, and samples were quickly stored at −80°C.
VEGF (R&D Systems), PlGF (Biosharp), sFlt-1 (Arigo), and sEng (Cusabio) concentrations in the maternal plasma were measured by ELISA kits.
The continuous variables with normal distribution were denoted as the mean ± standard deviation (SD), whereas the variables with non-normal distribution were denoted as the median (interquartile range). The Kolmogorov–Smirnov test checked if the distribution of the continuous variables meet a criterion of the normality. If the hypothesis of normality was satisfied, data were analyzed by the independent-sample Student’s t-test; otherwise, the non-parametric Mann–Whitney U-test was undertaken. The categorical variables were presented as number (percentage) and analyzed by Pearson Chi-Square test.
Spearman’s correlation analysis was adopted to relate maternal sEng concentration to placental weight, neonate birth weight, length, and head circumference in the study population.
Pearson’s correlation analysis was used to relate placental weight to neonate birth weight in the study groups.
The p threshold applied for significant differences was <0.05 (two-sided). Statistical analysis was performed using Statistical Product and Service Solutions software (IBM SPSS 25.0, Armonk, NY, USA).
3.1 Demographic and Clinical Characteristics
Eighty-two subjects were invovled in the study, including 27 cases and 55 controls. In case group, major fetal cardiac defects were shown in Table 1. Tetralogy of Fallot and transposition of the great arteries were the main type of CHD, accounting for 29.6%, respectively.
Maternal characteristics and pregnancy outcomes were presented in Table 2. Compared with control group, pregnant women carrying a CHD fetus manifested higher rates of altering to Cesarean section (55.56% vs. 21.82%, p = 0.002) and fetal heart rate deceleration (25.93% vs. 7.27%, p = 0.020) at delivery. Although the median value of Apgar scores at 1 min of newborns in two groups were both 10, significant differences were found in two groups (p = 0.003). This result indicated that the Apgar scores at 1 min of newborns with CHD were lower than that of infants with normal phenotype.
3.2 Comparisons of Proangiogenic/Anti-Angiogenic Factors in Maternal Plasma
The proangiogenic factors include PlGF and VEGF. The anti-angiogenic factors consist of sFlt-1 and sEng. The gestational age (GA) at sampling was stratified into two layers: 36 weeks ≤ GA < 39 weeks group and 39 weeks ≤ GA ≤ 41 weeks group. No significant differences were detected in demographic characteristics between the case-control populations after stratification. No differences were found in maternal plasma concentrations of PlGF, VEGF, and sFlt-1 between the case-control groups when analyzed at 36 weeks ≤ GA < 39 weeks and 39 weeks ≤ GA ≤ 41 weeks (Tables 3 and 4). The concentrations of sEng in maternal plasma in fetal CHD group were significantly higher than those in control group: 0.60 (0.77) vs. 0.32 (0.26) ng/ml at 36 weeks ≤ GA < 39 weeks, p = 0.001 and 0.75 (0.55) vs. 0.28 (0.27) ng/ml at 39 weeks ≤ GA ≤ 41 weeks, p < 0.001 (Tables 3 and 4).
3.3 Correlations between Maternal Plasma sEng Concentration and Placental Weight
A negative and significant correlation between maternal plasma sEng concentration and placental weight was detected in the study population (Spearman r: −0.221, p = 0.046) (Fig. 2). We also found that placental weight was positively correlated to neonate birth weight, showing a significant difference (Pearson r: 0.662, p < 0.001) (Fig. 3). There were no correlations between maternal sEng concentration and neonate birth weight, length, and head circumference in the study population.
Figure 2: Correlation between maternal plasma sEng concentration and placental weight. Spearman r: −0.221, p = 0.046
Figure 3: Correlation between placenta weight and neonate birth weight. Pearson r: 0.662, p < 0.001
Our experiments found that, in major fetal congenital heart defects, maternal plasma soluble endoglin concentrations were significantly elevated at delivery, suggesting maternal vascular dysfunction. However, no differences were found in maternal plasma levels of PlGF, VEGF, and sFlt-1 between case-control groups. This study provides the first evidence that excess sEng exists in pregnant women carrying a fetus with CHD.
Vascular endothelial growth factors (VEGF) are secreted dimeric glycoproteins, participating in vasculogenesis and angiogenesis [9]. This family consists of VEGF-A and placental growth factor (PlGF) in humans. VEGF-A (often called VEGF) is the first discovered and prototypical protein in this family. VEGF is also a proangiogenic factor, responsible for the proliferation and survival of endothelial cells. In addition, It can induce vascular permeability [10]. PlGF is a VEGF homolog derived from the placenta, possessing proangiogenic activity [11]. The anti-angiogenic factor, soluble fms-like tyrosine kinase (sFlt-1, the other name is sVEGFR-1) is a soluble form of the VEGF/PlGF receptor Flt-1 generated by alternative splicing [12]. Experiments in vitro have proved that sFlt-1 acts as an inhibitor of VEGF and PlGF activity. It can restrain endothelial tube formation and hinder the vasodilatory effect of VEGF and PlGF [12].
A pregnancy requires fetal vasculogenesis, angiogenesis, and maternal angiogenesis [13]. Placenta-derived proangiogenic factors (VEGF and PlGF) are also secreted into the maternal circulation where they help normal maternal cardiovascular adaptation to pregnancy in processes that primarily do not refer to angiogenesis. Increased secretion of anti-angiogenic factors could result in an anti-angiogenic state in the mother and induce the development of pregnancy complications such as preeclampsia and fetal growth restriction (FGR) [14]. Studies have already illustrated that an unbalance of proangiogenic and anti-angiogenic proteins plays a crucial part in the pathophysiology of preeclampsia [15]. On account of the fact that the vascular endothelium depends on proangiogenic factors, the excess secretion of anti-angiogenic factors derived from the placenta contributes to endothelial dysfunction which has been already detected in preeclampsia [16].
However, most studies on these factors were performed in preeclampsia. Is there any other pathology showing a similar unbalance of proangiogenic and anti-angiogenic factors or endothelial dysfunction in maternal circulation? Llurba and his colleagues conducted a case-control study and firstly demonstrated that, in critical fetal heart defects, maternal serum PlGF declined and sFlt-1 elevated at 18–37 weeks’ gestation, indicating the unbalance of proangiogenic and anti-angiogenic factors in maternal plasma [5]. This damaged condition was discovered in the conotruncal and septal-valve heart defects but not in congenital defects of the left side of the heart [12]. In order to further verify this conclusion, we designed similar research and extended the study GA to the delivery time, mostly >37 weeks. It could be regarded as a supplement for to Llurba’s study. Surprisingly, we did not find differences in the concentrations of PlGF, VEGF, and sFlt-1 in maternal plasma between case-control groups. We speculate that there may be two reasons that could well explain the difference: firstly, in Llurba’s study, PlGF was not decreased when analyzed at 32 weeks ≤ GA< 37 weeks. This may indicate that the PlGF level would keep stable from 32 weeks of gestation, which is consistent with our results; Secondly, the catch-up level of PlGF and balanced sFlt-1 concentration in maternal plasma at delivery may be a compensation for previously declined condition.
Another anti-angiogenic factor termed soluble endoglin (sEng) was determined by the gene expression profile of placentas from women who suffered from preeclampsia. An increasing amount of evidence has proven that VEGF and TGF-β1 are essential for maintaining endothelial health in some human organs including the placenta. In normal pregnancy, moderate levels of VEGF and TGF-β1 signaling in the vasculature keep the vascular homeostasis. In preeclampsia, overmuch placental release of sFlt-1 and sEng blocks VEGF and TGF-β1 signaling, respectively, in the vasculature. This leads to endothelial cell dysfunction, containing declined prostacyclin, nitric oxide production, and excretion of procoagulant factors [17,18].
Our another result differing from Llurba’s study is that maternal sEng level was profoundly increased in pregnant women with critical fetal CHD at delivery, suggesting that maternal endothelial dysfunction is present in congenital heart defects. Since many studies have indicated that excess sEng is a crucial element in the development of preeclampsia [8,16], our result again revealed that maternal preeclampsia and fetal CHD may share a common pathway, maybe not sFlt-1/PlGF pathway but sEng pathway. This result could further support the conclusion that preeclampsia was significantly associated with heart defects in offspring, made by Nathalie Auger and his colleagues who conducted a population-based investigation involving 1,942,072 neonates [7].
On the other hand, Caroline Signore found that plasma concentrations of the anti-angiogenic factor sEng are increased before the progress of hypertension and placental abruption. The increases are not obvious until the late second trimester (26–27 weeks, on average), but they keep stable from this time with the gestation onward [19]. They believe that sEng may be helpful in recognizing pregnant women at risk of abruption and hypertension. Therefore, combined with our study, it is alert for us to notice the maternal blood pressure and abdominal signs for early diagnosis of hypertension and placental abruption when handling the delivery of pregnant women with fetal CHD.
In addition, we found that the maternal plasma sEng level negatively correlated with placenta weight. Furthermore, placenta weight was positively correlated to the birth weight of the newborn, with a correlation coefficient of 0.662. As a result, it could be speculated that the elevated sEng level released from the placenta may suggest an impaired placenta function and manifest a low placenta weight, leading to low birth weight of newborns in pregnant women. Low birth weight would increase the rate of fetal heart rate deceleration and altering to Cesarean Section at delivery in the CHD group, which is consistent with our study and the guideline in 2014 [20]. Unfortunately, we could not find a correlation between maternal plasma sEng concentration and neonate birth weight statistically. It can be explained that maternal plasma sEng level may not be the direct impact factor that causes a low birth weight of newborns in pregnant women with fetal CHD.
Indeed, the small number of subjects was the limitation of our study. The future study should enlarge the number of specimens. We only verified the association between the maternal increased sEng level and fetal congenital heart defects. Further research should focus on the origination of elevated sEng, such as placental histology and so on.
In conclusion, vascular dysfunction exists in pregnant women with fetal congenital heart defects, manifesting significantly elevated sEng concentration at delivery.
Acknowledgement: The authors acknowledge all of the study staff and participants who contributed to this project.
Funding Statement: The work was supported by grants from the Guangzhou Municipal Science and Technology Bureau (Nos. 202102080466, 202201011423, 202206010049, 2023B03J0596, 2023B03J1254, 2023B03J1255), Department of Science and Technology of Guangdong Province (Nos. 2020B1111170011, 2023A1515012501), the Natural Science Foundation of Guangdong Province (Nos. 2023A1515010801, 2021A1515011445) and the National Natural Science Foundation of China (Nos. 82100371, 81903287).
Author Contributions: The authors confirm contribution to the paper as follows: study conception and design: Yanli Liu, Haiping Wang; data collection: Yanyan Lin, Weina Zhang, Yanji Qu; experimental operation: Yanli Liu, Haiping Wang; analysis and interpretation of results: Yanli Liu, Haiping Wang; draft manuscript preparation: Yanli Liu, Haiping Wang; financial support: Jian Zhuang, Yanqiu Ou, Haiping Wang; professional support: Fengzhen Han, Liping Huang. All authors reviewed the results and approved the final version of the manuscript.
Availability of Data and Materials: The datasets generated during the current study are available from the corresponding author on reasonable request.
Ethics Approval: This study was conducted in accordance with the Declaration of Helsinki and approved by the Ethics Committee of Guangdong Provincial People’s Hospital (No. KY-Z-2021-428-02). Informed written consent was acquired from all patients prior to their enrollment in this study.
Conflicts of Interest: The authors declare that they have no conflicts of interest to report regarding the present study.
References
1. van der Linde, D., Konings, E. E. M., Slager, M. A., Witsenburg, M., Helbing, W. A. et al. (2011). Birth prevalence of congenital heart disease worldwide: A systematic review and meta-analysis. Journal of the American College of Cardiology, 58(21), 2241–2247. https://doi.org/10.1016/j.jacc.2011.08.025 [Google Scholar] [CrossRef]
2. van der Bom, T., Zomer, A. C., Zwinderman, A. H., Meijboom, F. J., Bouma, B. J. et al. (2011). The changing epidemiology of congenital heart disease. Nature Reviews Cardiology, 8(1), 50–60. https://doi.org/10.1038/nrcardio.2010.166 [Google Scholar] [PubMed] [CrossRef]
3. Dearani, J. A., Mavroudis, C., Quintessenza, J., Deal, B. J., Backer, C. L. et al. (2009). Surgical advances in the treatment of adults with congenital heart disease. Current Opinion in Pediatrics, 21(5). [Google Scholar]
4. Srivastava, D. (2006). Making or breaking the heart: From lineage determination to morphogenesis. Cell, 126(6), 1037–1048. https://doi.org/10.1016/j.cell.2006.09.003 [Google Scholar] [CrossRef]
5. Llurba, E., Sanchez, O., Ferrer, Q., Nicolaides, K. H., Ruiz, A. et al. (2014). Maternal and foetal angiogenic imbalance in congenital heart defects. European Heart Journal, 35(11), 701–707. https://doi.org/10.1093/eurheartj/eht389 [Google Scholar] [PubMed] [CrossRef]
6. von Dadelszen, P., Magee, L. A. (2014). Pre-eclampsia: An update. Current Hypertension Reports, 16(8), 454. https://doi.org/10.1007/s11906-014-0454-8 [Google Scholar] [PubMed] [CrossRef]
7. Auger, N., Fraser, W. D., Healy-Profitos, J., Arbour, L. (2015). Association between preeclampsia and congenital heart defects. JAMA, 314(15), 1588–1598. https://doi.org/10.1001/jama.2015.12505 [Google Scholar] [CrossRef]
8. Nikuei, P., Rajaei, M., Malekzadeh, K., Nejatizadeh, A., Mohseni, F. et al. (2017). Accuracy of soluble endoglin for diagnosis of preeclampsia and its severity. Iranian Biomedical Journal, 21(5), 312–320. https://doi.org/10.18869/acadpub.ibj.21.5.312 [Google Scholar] [PubMed] [CrossRef]
9. Leung, D. W., C, G., Kuang, W. J., Goeddel, D. V., Ferrara, N. (1989). Vascular endothelial growth factor is a secreted angiogenic mitogen. Science, 246(4935), 1306–1309. https://doi.org/10.1126/science.2479986 [Google Scholar] [PubMed] [CrossRef]
10. Keck, P. J., Hauser, S. D., Krivi, G., Sanzo, K., Warren, T. et al. (1989). Vascular permeability factor, an endothelial cell mitogen related to PDGF. Science, 246(4935), 1309–1312. https://doi.org/10.1126/science.2479987 [Google Scholar] [PubMed] [CrossRef]
11. Maglione, D., Guerriero, V., Viglietto, G., Delli-Bovi, P., Persico, M. G. (1991). Isolation of a human placenta cDNA coding for a protein related to the vascular permeability factor. Proceedings of the National Academy of Sciences, 88(20), 9267–9271. https://doi.org/10.1073/pnas.88.20.9267 [Google Scholar] [PubMed] [CrossRef]
12. Kendall, R. L., T, K. (1993). Inhibition of vascular endothelial cell growth factor activity by an endogenously encoded soluble receptor. Proceedings of the National Academy of Sciences, 90(22), 10705–10709. https://doi.org/10.1073/pnas.90.22.10705 [Google Scholar] [PubMed] [CrossRef]
13. Sliwa, K., Mebazaa, A. (2014). Possible joint pathways of early pre-eclampsia and congenital heart defects via angiogenic imbalance and potential evidence for cardio-placental syndrome. European Heart Journal, 35(11), 680–682. https://doi.org/10.1093/eurheartj/eht485 [Google Scholar] [PubMed] [CrossRef]
14. Cuffe, J. S. M., Holland, O., Salomon, C., Rice, G. E., Perkins, A. V. (2017). Review: Placental derived biomarkers of pregnancy disorders. Placenta, 54, 104–110. https://doi.org/10.1016/j.placenta.2017.01.119 [Google Scholar] [PubMed] [CrossRef]
15. Noori, M., Donald, A. E., Angelakopoulou, A., Hingorani, A. D., Williams, D. J. (2010). Prospective study of placental angiogenic factors and maternal vascular function before and after preeclampsia and gestational hypertension. Circulation, 122(5), 478–487. https://doi.org/10.1161/CIRCULATIONAHA.109.895458 [Google Scholar] [PubMed] [CrossRef]
16. Powe, C. E., Levine, R. J., Karumanchi, S. A. (2011). Preeclampsia, a disease of the maternal endothelium: The role of antiangiogenic factors and implications for later cardiovascular disease. Circulation, 123(24), 2856–2869. https://doi.org/10.1161/CIRCULATIONAHA.109.853127 [Google Scholar] [PubMed] [CrossRef]
17. Venkatesha, S., Toporsian, M., Lam, C., Hanai, J. I., Mammoto, T. et al. (2006). Soluble endoglin contributes to the pathogenesis of preeclampsia. Nature Medicine, 12(6), 642–649. https://doi.org/10.1038/nm1429 [Google Scholar] [PubMed] [CrossRef]
18. Maharaj, A. S., Walshe, T. E., Saint-Geniez, M., Venkatesha, S., Maldonado, A. E. et al. (2008). VEGF and TGF-beta are required for the maintenance of the choroid plexus and ependyma. Journal of Experimental Medicine, 205(2), 491–501. https://doi.org/10.1084/jem.20072041 [Google Scholar] [PubMed] [CrossRef]
19. Signore, C., Mills, J. L., Qian, C., Yu, K. F., Rana, S. et al. (2008). Circulating soluble endoglin and placental abruption. Prenat Diagn, 28(9), 852–858. https://doi.org/10.1002/pd.2065 [Google Scholar] [PubMed] [CrossRef]
20. Donofrio, M. T., Moon-Grady, A. J., Hornberger, L. K., Copel, J. A., Sklansky, M. S. et al. (2014). Diagnosis and treatment of fetal cardiac disease: A scientific statement from the American Heart Association. Circulation, 129(21), 2183–2242. https://doi.org/10.1161/01.cir.0000437597.44550.5d [Google Scholar] [PubMed] [CrossRef]
Cite This Article
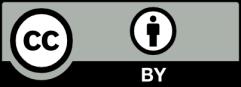