Open Access
ARTICLE
Intraoperative Blood Glucose Levels and Postoperative Acute Kidney Injury in Pediatric Patients Having Congenital Heart Surgery under Cardiopulmonary Bypass
1 Department of Anesthesiology, Fuwai Hospital, National Centre for Cardiovascular Diseases, Chinese Academy of Medical Sciences and Peking Union Medical College, Beijing, China
2 Department of Anesthesiology, Fuwai Hospital, Chinese Academy of Medical Sciences, Shenzhen, China
3 Department of Anesthesiology, Peking Union Medical College Hospital, Beijing, China
* Corresponding Authors: Jianhui Wang. Email: ; Fuxia Yan. Email:
# Contributed equally as co-first authors
Congenital Heart Disease 2023, 18(4), 475-488. https://doi.org/10.32604/chd.2023.028017
Received 22 November 2022; Accepted 21 May 2023; Issue published 15 September 2023
Abstract
Purpose: This study sought to explore the effect of intraoperative mean blood glucose levels and variability on postoperative acute kidney injury (AKI) in children undergoing congenital cardiac surgery. Methods: We conducted a prospective nested case-control study in children (age < 18 years) undergoing congenital heart surgery with cardiopulmonary bypass (CPB) at the Fuwai Hospital between April 01, 2022 and July 30, 2022. Cases were individuals who developed AKI within the first postoperative 7 days (AKI group) and controls were those without AKI (Non-AKI group) according to KDIGO criteria. AKI and Non-AKI groups unmatched and 1:1 matched by age, sex, and baseline serum creatinine were separately analyzed. Multivariate logistic and conditional logistic regressions were used to assess the associations between blood glucose variables and AKI. Results: 688 consecutively approached patients were included in the final analysis. On multivariate analysis, intra-CPB (adjusted odds ratio [OR] 0.802; 95% confidence interval [CI], 0.706 to 0.912; p = 0.001) and post-CPB (adjusted OR 0.830; 95% CI, 0.744 to 0.925; p = 0.001) blood glucose levels were associated with postoperative AKI. There were no significant differences in pre-CPB blood glucose (adjusted OR 0.926; 95% CI, 0.759 to 1.129; p = 0.446) or intraoperative glycemic fluctuations (adjusted OR 0.905; 95% CI, 0.723 to 1.132; p = 0.382) between AKI and Non-AKI groups. Results based on matched cases and controls were consistent with those from the unmatched analyses. Conclusion: Higher intraoperative blood glucose levels during and after CPB were protective factors against postoperative AKI in pediatric patients after congenital heart surgery.Graphical Abstract
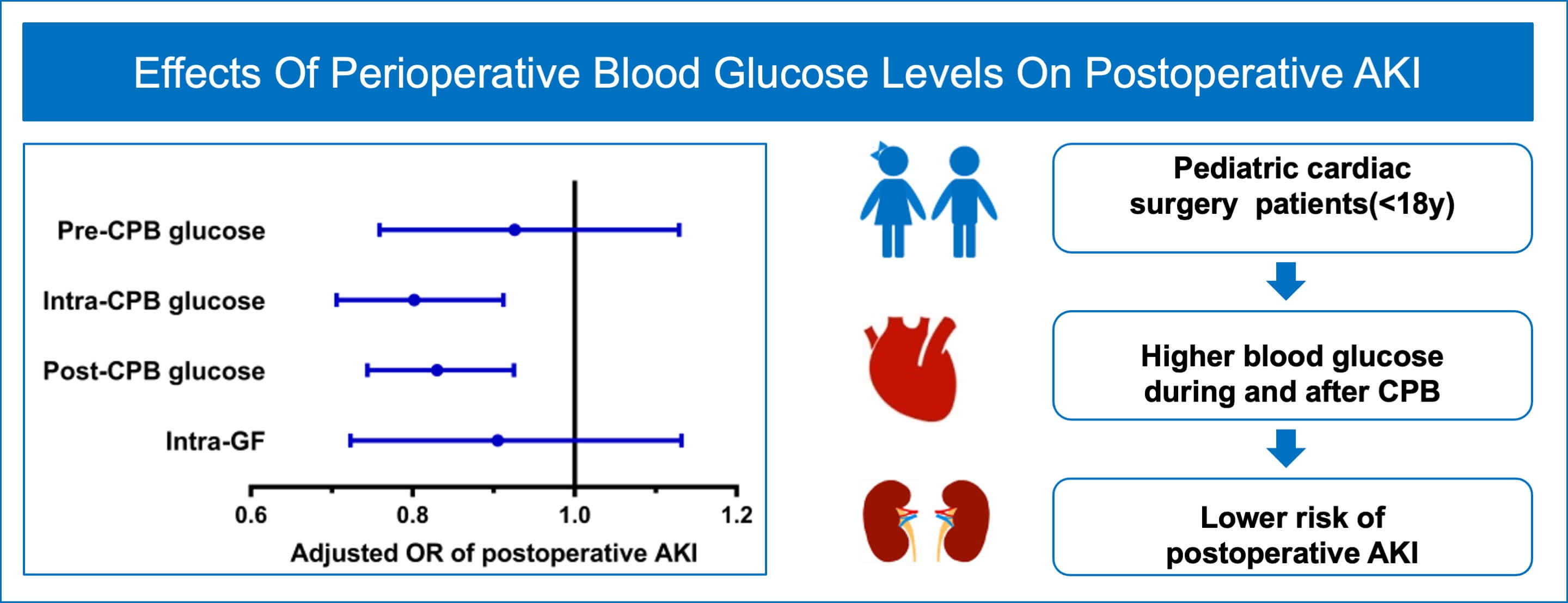
Keywords
Abbreviations
AKI | acute kidney injury |
CPB | cardiopulmonary bypass |
CICU | cardiac intensive care units |
IntraGF | intraoperative glycemic fluctuation |
KDIGO | Kidney Disease Improving Global Outcomes |
SCr | serum creatinine |
LVEF | left ventricular ejection fraction |
CI | confidence interval |
OR | odds ratio |
IQR | interquartile range |
Acute kidney injury (AKI) is a common postoperative complication for children undergoing congenital heart surgery with cardiopulmonary bypass (CPB), accounting for 30% to 50% of them [1,2]. AKI is independently associated with elevated mortality and morbidity in pediatric patients following cardiac surgery, including prolonged duration of mechanical ventilation and longer ICU stay [3,4]. Unfortunately, the specific mechanism of AKI currently remains unclear, and its occurrence may be a result of a combination of multiple risk factors.
Fluctuation of blood glucose levels occurs frequently in cardiac surgery, especially with CPB [5]. Some studies demonstrated that AKI occurrence was associated with intra-, postoperative hyperglycemia and dramatic blood glucose variability in adults undergoing cardiac surgery [6,7]. Additionally, strict perioperative blood glucose control could significantly reduce the incidence of postoperative renal impairment and failure in adult cardiac surgery patients [8]. Concerning pediatric patients having cardiac surgery, especially with CPB, they are prone to varieties of blood glucose levels intraoperatively. Unexpectedly, studies as to the harm from intraoperative blood glucose levels or glucose variability are inconclusive [9–12]. Meanwhile, tight blood glucose control might not be beneficial to a decreased incidence of AKI [13]. So we conducted this study to further explore the effect of intraoperative mean blood glucose levels and variability on AKI in children following their congenital cardiac surgery.
This prospective nested case-control study was performed in accordance with the Declaration of Helsinki and was approved by the Ethics Committee of Fuwai Hospital, chaired by Professor Shoujun Li and Professor Wei Li, on 03 March 2022 (Ethical approval number: 2021–1607), and written informed consent was obtained from all participants and/or their legal guardians before enrollment. This trial was registered at https://clinicaltrials.gov/ (Registered 11 March 2022, NCT05489263) and adhered to the Strengthening the Reporting of Observational Studies in Epidemiology (STROBE) reporting guideline for observational studies.
We consecutively selected pediatric patients aged less than 18 years who were scheduled to undergo congenital heart surgery under CPB between April 01, 2022, and July 30, 2022, at Fuwai Hospital, Chinese Academy of Medical Sciences, Beijing, China. The exclusion criteria were any of the following: 1) patients refusing to participate; 2) patients with preoperative kidney dysfunction; 3) patients with a history of a diabetic disease; 4) patients undergoing off-pump surgeries; 5) patients suffering surgical procedures during the first 7 postoperative days.
Cases were patients who developed AKI within the first 7 postoperative days as defined in the original cohort. Controls were sampled among patients without AKI diagnosis on day 7. Propensity-score matching was used to select the control for each case. Cases and controls were 1:1 matched by age, sex, and baseline serum creatinine (SCr) to generate a paired sample set. The description of the source population and case-control selection was presented in Fig. 1.
Figure 1: Flow chart of the study
Note: AKI = acute kidney injury; SCr = serum creatinine.
Blood glucose values were prospectively collected from the arterial blood gas analysis. Considering the use of CPB was significantly associated with perioperative hyperglycemia [14]. We divided intraoperative blood glucose into three parts according to the process of CPB: 1) pre-CPB: the mean blood glucose levels after 5 min following heparinization; 2) intra-CPB: average of all glucose levels during the CPB (testing at an interval of 30 min); 3) post-CPB: mean blood glucose values from protamine infusion to the end of surgery (testing at an interval of 30 min). The magnitude of intraoperative glycemic fluctuation (intra-GF) was defined as the standard difference (SD) of each patient’s intraoperative blood glucose values, reflecting the variability of intraoperative glucose levels [9].
The general characteristics of eligible patients included age, sex, weight, gestation weeks, baseline SCr, preoperative left ventricular ejection fraction (LVEF), and cardiac surgery history [15]. Surgical complexity was assessed using the Risk Adjustment in Congenital Heart Surgery (RACHS)-1 score [16]. The history of diabetes and chronic kidney disease were validated from clinician-entered data in the preoperative evaluation form. We also recorded intraoperative medical data, such as CPB time, aortic cross-clamping time, the lowest core temperature during CPB, modified ultrafiltration, blood products transfusion, intraoperative steroids administration, and blood glucose values. Postoperative SCr was tested daily during the first 7 postoperative days. Besides, we also recorded the length of cardiac intensive care unit (CICU) and hospital stay.
During the first 7 days after surgery, we assessed AKI based on SCr with the kidney disease: Improving Global Outcome (KDIGO) Group definition: 1) Stage 1: an increase ≥ 26.5 μmol/L within 48 h or a 1.5–1.99-fold increase, 2) Stage 2: a 2.0–2.99-fold increase, and 3) Stage 3: a ≥ 3.0-fold increase or SCr level > 354 μmol/L or the initiation of dialysis, or estimated glomerular filtration rate < 35 ml/min/1.73 m2 [17].
Continuous data were presented as medians with interquartile range (IQR) and categorical variables as numbers with proportions. Before matching, patient characteristics between cases and controls were compared using Wilcoxon rank-sum tests for continuous variables and Chi-Squared tests (or Fisher exact tests) for categorical variables. All clinically sensible variables with values of p < 0.2 in univariate analysis were entered into a multivariate logistic regression model to analyze the independent relationships between intraoperative glycemic metrics and postoperative AKI. The effect size was quantified by the adjusted odds ratio (adjusted OR) and 95% confidence interval (CI).
In the matched analysis, patients were 1:1 propensity-score matched by age, sex, and baseline SCr using the nearest neighbor methodology with a maximum caliper of 0.02 of the propensity scores. Variables were compared by paired Wilcoxon rank-sum tests and McNemar’s test in this matched sample set. Conditional logistic regression was used to assess associations between intraoperative blood glucose metrics and AKI by adjusting for potential confounders. In our study, we required a minimum of 10 events per predictor to avoid overfit models and included only one factor if two factors were correlated (i.e., Pearson’s correlation coefficient r > 0.5) to avoid collinearity. A two-sided p-value < 0.05 was considered a significant difference. Statistical analyses were performed using SPSS V.25 (IBM Co., Armonk, NY, USA), and Graph Pad Prism version 7.0 for Windows was used (Graph Pad, La Jolla, CA, USA) for drawing figures.
As shown in Fig. 1, from April 01, 2022, and July 30, 2022, we screened 688 eligible patients from 791 patients, including 196 cases and 492 controls. After propensity score matching by age, sex, and baseline SCr, 140 cases and 140 controls were paired.
Table 1 demonstrated the univariate analyses of demographic data and potential risk factors by unmatched and 1:1 matched method. In the entire study population, the median age was 25.7 months and 49.6% were male patients. We selected the patients undergoing all kinds of congenital heart surgeries, and 48.3% (332/688) of the patients experienced high-risk surgery (RACHS-1 ≥ 3) [18]. The overall incidence of AKI was 28.5% (196/688), and patients who were categorized into AKI stages 1, 2, and 3 were 19.9% (137/688), 6.1% (42/688), and 2.5% (17/688) separately (data are given in Appendix A).
Before matching, there were no significant differences between patients with and without AKI in sex, gestation weeks, or preoperative LVEF (all p > 0.05), but those who developed AKI were more likely to be younger and have cyanotic heart disease, undergo more complex surgeries (RACHS-1 ≥ 4), experience lower core temperature during CPB, more frequent use of modified ultrafiltration and intraoperative platelet transfusions (all p < 0.05). Fig. 2A showed the distributions of intraoperative glycemic metrics between AKI and Non-AKI groups. The median [IQR] pre-CPB blood glucose levels of AKI and Non-AKI patients were 4.8 (4.3 to 5.6) and 5.1 (4.5 to 5.6) mmol/L respectively, the intra-CPB blood glucose levels were 6.3 (5.2 to 7.4) and 6.6 (5.6 to 7.7) mmol/L, and the post-CPB blood glucose levels were 7.7 (6.2 to 9.2) and 7.9 (6.7 to 9.4) mmol/L. Intra-GFs between the two groups were similar at 1.6 (0.9 to 2.3) and 1.6 (1.0 to 2.2) mmol/L. By univariate analysis, higher intra-CPB (OR 0.896; 95% CI, 0.812 to 0.989; p = 0.029) and post-CPB blood glucose levels (OR 0.903; 95% CI, 0.831 to 0.982; p = 0.017) during the perioperative time were significantly associated with postoperative AKI, while pre-CPB and intra-GF were not. After accounting for known and potential confounding factors (p < 0.2), the multivariate regression analysis showed a consistent result, that higher intra-CPB (adjusted OR 0.802; 95% CI, 0.706 to 0.912; p = 0.001) and post-CPB blood glucose levels (adjusted OR 0.830; 95% CI, 0.744 to 0.925; p = 0.001) both significantly associated with lower risk of postoperative AKI. However, the pre-CPB and intra-GF were not associated with AKI in this study. Results are shown in Table 2.
Figure 2: The distributions of intraoperative mean blood glucose levels and glycemic fluctuations between AKI and Non-AKI groups. Panel A shows the data before 1:1 matched by sex, age, and baseline SCr. Panel B shows the corresponding data after matching
Note: AKI = acute kidney injury; CPB = cardiopulmonary bypass; GF = glycemic fluctuation.
After matching, the differences between age, sex, and baseline SCr were well-balanced (p < 0.05). The median [IQR] age of the cases and controls were 11.2 (3.9 to 30.4) and 10.6 (6.0 to 31.8) months, respectively. Male patients made up about 50% of both two groups. The distributions of intraoperative glycemic metrics were presented in Fig. 2B. Multivariate conditional logistic regression showed that intra-CPB (adjusted OR 0.784; 95% CI, 0.649 to 0.948; p = 0.012) and post-CPB glucose levels (adjusted OR 0.803; 95% CI, 0.691 to 0.933; p = 0.004) were significantly associated with AKI after adjusting for confounders (p < 0.2), including preoperative LVEF, CPB time, the lowest core temperature during CPB and intraoperative platelet transfusion, RACHS-1 score ≥4, cyanosis, neonate. Results were consistent in the study population before and after matching. The univariate and multivariate analyses of the associations between intraoperative glycemic metrics and postoperative AKI after matching are presented in Table 3, and the results of multivariate analyses are shown in Fig. 3.
Figure 3: The relationships of intraoperative glucose metrics and postoperative AKI
Note: AKI = acute kidney injury; CPB = cardiopulmonary bypass; GF = glycemic fluctuation
This prospective nested-case control study showed that higher intraoperative blood glucose levels during and after CPB were independent protective factors against AKI in pediatric patients following cardiac surgery. However, there was no significant difference in blood glucose levels before CPB and variability of intraoperative blood glucose levels between AKI and control groups.
Our study assessed the potential effects of intraoperative blood glucose and glycemic fluctuations on postoperative AKI in pediatric patients undergoing congenital heart surgery. Based on previous studies, hyperglycemia, hypoglycemia, and glucose variability were significantly associated with poor clinical outcomes [19]. Some experiments reported that hyperglycemia-induced reactive oxygen species could inhibit Na+/glucose cotransporter activity in renal proximal tubule cells and result in renal oxidative stress and kidney injury [20,21]. Certainly, hypoglycemia could decrease glucose intake and compromise cellular biosynthetic abilities, and also result in acute organ impairment [19,22]. Patients with a minimum glucose level less than 75 mg/dL both intraoperatively and postoperatively had a 3-fold greater adjusted odds ratio of developing the composite morbidity-mortality endpoints including renal failure requiring dialysis [18]. Besides, acute glycemic fluctuations were independently associated with postoperative AKI induced by endothelial apoptosis, greater endothelial dysfunction, and activation of oxidative stress in non-cardiac surgery patients and children who underwent congenital heart surgery [11,23–25]. Overall, these findings above display a potential biological association between intraoperative blood glucose metrics and postoperative AKI in children undergoing congenital heart surgery though the underlying mechanism is unclear.
In this study, we selected peri-CPB as the points of investigation mainly because CPB was strikingly associated with intraoperative elevated blood glucose levels and variability. The process of CPB induces stress response and systemic inflammatory reaction which are associated with hyperinsulinemia and insulin resistance, posing a dominating contribution to perioperative hyperglycemia [14,26]. During stress, increased secretion of hormones like glucagon, catecholamines, growth hormone and cortisol promotes gluconeogenesis in the liver and impairs insulin action in peripheral tissues, resulting in elevated serum glucose [27,28]. At the same time, prophylactic glucocorticoids have been routinely administered to infants undergoing heart surgery with CPB in the perioperative period to attenuate the systemic inflammatory response. Recent studies have demonstrated a significant association between glucocorticoids administration and hyperglycemia in pediatric patients undergoing cardiac surgery [29,30].
Several studies have examined the effect of hyperglycemia and adverse outcomes in the pediatric cardiac population [31,32]. Average postoperative glucose >143 mg/dL and peak glucose level ≥250 mg/dL were associated with greater adjusted odds of reaching a composite morbidity-mortality end point after complex congenital heart surgery [18]. In O’Brien’s study, intraoperative hyperglycemia which was defined more than 175 mg/dL had a significant association with postoperative bacteremia in a pediatric cardiac population aged less than 18 years old [33]. In contrast to previous studies involving postoperative hyperglycemia in the intensive care unit, our study showed no harmful effects of higher mean blood glucose on postoperative AKI during surgery. The underlying factors affecting intraoperative glucose levels, including surgical insults and the process of CPB, differ from the causes of postoperative hyperglycemia. Duncan and colleagues suggested that intraoperative mild hyperglycemia (141 to 170 mg/dL/7.8 to 9.4 mmol/L) was well tolerated in cardiac surgical patients and may be associated with benefits on postoperative outcomes [12]. In Lou’s study, perioperative hyperglycemia (>150 mg/dL) was not detrimental to infants undergoing cardiac surgery with CPB [9]. What’s more, a blood glucose level of no more than 8.1 mmol/L (146 mg/dL) during CPB is associated with a greatly lower risk probability of severe systemic inflammatory response syndrome in pediatric patients [34].
Our data showed that the median intra-CPB blood glucose levels were 6.3 and 6.6 mmol/L, and the post-CPB blood glucose were 7.7 and 7.9 mmol/L respectively in AKI and Non-AKI groups before matching, which suggested that most of our pediatric patients were at a relatively normal and safe glycemic threshold. Higher intraoperative glucose levels may ensure increased glucose uptake and confer protection from hypoxic-ischemic injury by promoting anti-apoptotic pathways and favoring angiogenesis [35], yielding the roles of organ protection in infants undergoing deep hypothermic circulatory arrest [36,37]. In our study, the average serum blood glucose in the Non-AKI group was higher than that in the AKI group and the difference was statistically significant. Besides, there was no significant difference in intraoperative steroids administration between AKI and Non-AKI groups, which meant that the difference of serum glucose between AKI and Non-AKI groups may be not associated with steroids administration in this study. So we reported that higher blood glucose levels during and after CPB may be nephroprotective. Since no optimal perioperative serum glucose range has been made in pediatric patients with a large age range undergoing cardiac surgery, we did not define a cutoff value of hyperglycemia which would be associated with increased incidence of adverse outcomes. But we believe that there is a certain upper limit which the intraoperative serum glucose exceeds would cause a harmful effect.
This study did not show an association between pre-CPB blood glucose and postoperative AKI. Our findings are somehow consistent with another observational study in pediatric patients undergoing congenital heart surgery, which found that blood glucose before CPB was not a predictor of mortality, infection, or increased duration of ventilation time [38]. Unlike adults who are commonly associated with obesity, dyslipidemia, insulin resistance, and hypertension, the children in our study have no diabetic complications or other metabolic syndromes. In addition, the pre-CPB blood glucose defined in our study did not represent a long-term but acute glycometabolic status. Pre-CPB blood glucose may be not an independent predictor of postoperative AKI in pediatric patients following cardiac surgery, and the subsequently elevated CPB blood glucose levels may pose a more important role in AKI.
Previous observational studies in adults and children have demonstrated that intraoperative glycemic fluctuation is an independent predictor of postoperative AKI [11,39]. However, this association was not observed in this study. Similarly high levels of intraoperative glycemic fluctuations were seen in AKI and Non-AKI patients in our study. This could be partly explained by the different study design that we included all kinds of congenital heart surgeries in the final analysis and almost half of them (51.7%, 356/688) are not complex congenital heart surgeries (RACHS-1 < 3) [18]. The whole blood glucose variability during surgery is not so great. In addition, we included patients aged less than 18 years which represents a wide pediatric age range. Though uniform normoglycemia thresholds and definitions of diabetes have been adopted in children, there are still age-related differences in response to dysglycemia [40,41]. For example, cortisoland growth hormone responses to spontaneous hypoglycemia are highly age-dependent, and impaired fasting glucose risk is positively correlated with increasing age [42,43]. Previous studies also showed a age influence on glucose levels or insulin requirement to achieve target glycemic control [44,45]. Considering the age-related effects on glucose metabolism, we did not provide specific cutoff limits in this study. Furthermore, it remains unknown whether age-related differences affect the biological sequelae of intraoperative hyperglycemia in children undergoing cardiac surgery.
Our study has some limitations that merit the next explorations. Firstly, we collected glucose levels intermittently intraoperatively and might miss the peak values of blood glucose. However, we acquired the blood glucose data at the same time points for all enrolled patients, thereby generating relatively good comparability between the two groups. Secondly, although we obtained that higher blood glucose levels may be a protective factor against AKI, we did not provide the specific cutoff value of optimal blood glucose level. We will enlarge our sample size to further explore the optimal blood glucose thresholds for postoperative renal function according to different age gaps. Thirdly, though the incidence of AKI in our study is consistent with that in a previous study diagnosing AKI solely according to serum creatinine [46], it is probable that some additional AKI would have been identified when combined with the urine output KDIGO criteria. Lastly, this was a single-center observational study, some confounding factors were unavoidable, which might affect the final results. However, we prospectively collect the data, thereby guaranteeing the integrity and reliability of the data as far as possible. Besides, we performed strictly univariate and multivariate analyses under both unmatched and matched methods and obtained consistent results.
This nested-case control study demonstrated that higher intraoperative glucose levels during and after CPB may be protective factors against AKI in the pediatric population having congenital heart surgery. The intraoperative glucose levels before CPB and the glycemic fluctuations during the whole surgery were not significantly associated with postoperative AKI. These results suggested that intraoperative blood glucose levels after CPB initiation could produce a great influence on AKI occurrence in pediatric cardiac surgery patients. Unluckily, we did not identify the specific cutoff value of optimal blood glucose level due to the large age range of enrolled patients. Another prospective study is in progress in our center to investigate the optimal cutoff values of intraoperative blood glucose associated with reduced AKI incidence in children following cardiac surgery based on different age gaps.
Acknowledgement: Not applicable.
Funding Statement: This study was supported by the CAMS Innovation Fund for Medical Sciences (CIFMS) (2021-I2M-C&T-B-036).
Author Contributions: Dongyun Bie, Hongbai Wang, Jianhui Wang, Fuxia Yan conceptualized and designed the study, carried out the initial analyses, drafted the initial manuscript, and critically reviewed and revised the manuscript; Chaobin Zhang, Chunrong Wang, Sheng Shi, Jiangshan Huang collected data, carried out the initial analyses, and critically reviewed and revised the manuscript; Yuan Jia and Su Yuan conceptualized and designed the study, coordinated and supervised data collection, and critically reviewed and revised the manuscript for important intellectual content. All authors approved the final manuscript as submitted and agree to be accountable for all aspects of the work.
Availability of Data and Materials: The datasets used and/or analysed during the current study are available from the corresponding author on reasonable request.
Ethics Approval: Research involving human subjects complied with all relevant national regulations, institutional policies and is in accordance with the tenets of the Helsinki Declaration and has been approved by the Ethics Committee of Fuwai Hospital (Ethical approval number: 2021-1607).
Conflicts of Interest: The authors declare that they have no conflicts of interest to report regarding the present study.
References
1. Madsen, N. L., Goldstein, S. L., Frøslev, T., Christiansen, C. F., Olsen, M. (2017). Cardiac surgery in patients with congenital heart disease is associated with acute kidney injury and the risk of chronic kidney disease. Kidney International, 92(3), 751–756. [Google Scholar] [PubMed]
2. Peng, K., McIlroy, D. R., Bollen, B. A., Billings, F. T., Zarbock, A. et al. (2022). Society of cardiovascular anesthesiologists clinical practice update for management of acute kidney injury associated with cardiac surgery. Anesthesia and Analgesia, 135(4), 744–756. [Google Scholar] [PubMed]
3. Li, S., Krawczeski, C. D., Zappitelli, M., Devarajan, P., Thiessen-Philbrook, H. et al. (2011). Incidence, risk factors, and outcomes of acute kidney injury after pediatric cardiac surgery: A prospective multicenter study. Critical Care Medicine, 39(6), 1493–1499. [Google Scholar] [PubMed]
4. Blinder, J. J., Goldstein, S. L., Lee, V. V., Baycroft, A., Fraser, C. D. et al. (2012). Congenital heart surgery in infants: Effects of acute kidney injury on outcomes. The Journal of Thoracic and Cardiovascular Surgery, 143(2), 368–374. [Google Scholar] [PubMed]
5. Anderson, R. E., Brismar, K., Barr, G., Ivert, T. (2005). Effects of cardiopulmonary bypass on glucose homeostasis after coronary artery bypass surgery. European Journal of Cardio-Thoracic Surgery, 28(3), 425–430. [Google Scholar] [PubMed]
6. Nam, K., Jeon, Y., Kim, W. H., Jung, D. E., Kwon, S. M. et al. (2019). Intraoperative glucose variability, but not average glucose concentration, may be a risk factor for acute kidney injury after cardiac surgery: A retrospective study. Journal Canadien D’anesthesie, 66(8), 921–933. [Google Scholar] [PubMed]
7. Mendez, C. E., Der Mesropian, P. J., Mathew, R. O., Slawski, B. (2016). Hyperglycemia and acute kidney injury during the perioperative period. Current Diabetes Reports, 16(1), 10. [Google Scholar] [PubMed]
8. Lecomte, P., van Vlem, B., Coddens, J., Cammu, G., Nollet, G. et al. (2008). Tight perioperative glucose control is associated with a reduction in renal impairment and renal failure in non-diabetic cardiac surgical patients. Critical Care, 12(6), R154. [Google Scholar] [PubMed]
9. Lou, S., Ding, F., Long, C., Liu, J., Zhao, J. et al. (2011). Effects of peri-operative glucose levels on adverse outcomes in infants receiving open-heart surgery for congenital heart disease with cardiopulmonary bypass. Perfusion, 26(2), 133–139. [Google Scholar]
10. Huang, X. Z., Wang, H., Xu, H. Z., Ye, M., Jiang, P. et al. (2012). Association between perioperative glucose levels and adverse outcomes in infants receiving open-heart surgery with cardiopulmonary bypass for congenital heart disease. Anaesthesia and Intensive Care, 40(5), 789–794. [Google Scholar] [PubMed]
11. Hu, G. H., Duan, L., Jiang, M., Zhang, C. L., Duan, Y. Y. (2018). Wider intraoperative glycemic fluctuation increases risk of acute kidney injury after pediatric cardiac surgery. Renal Failure, 40(1), 611–617. [Google Scholar] [PubMed]
12. Duncan, A. E., Abd-Elsayed, A., Maheshwari, A., Xu, M., Soltesz, E. et al. (2010). Role of intraoperative and postoperative blood glucose concentrations in predicting outcomes after cardiac surgery. Anesthesiology, 112(4), 860–871. [Google Scholar] [PubMed]
13. Blinder, J. J., Asaro, L. A., Wypij, D., Selewski, D. T., Agus, M. S. D. et al. (2017). Acute kidney injury after pediatric cardiac surgery: A secondary analysis of the safe pediatric Euglycemia after cardiac surgery trial. Pediatric Critical Care Medicine, 18(7), 638–646. [Google Scholar] [PubMed]
14. Floh, A. A., Manlhiot, C., Redington, A. N., McCrindle, B. W., Clarizia, N. A. et al. (2015). Insulin resistance and inflammation are a cause of hyperglycemia after pediatric cardiopulmonary bypass surgery. The Journal of Thoracic and Cardiovascular Surgery, 150(3), 498–504.e1. [Google Scholar] [PubMed]
15. Garg, R., Grover, A., McGurk, S., Rawn, J. D. (2013). Predictors of hyperglycemia after cardiac surgery in nondiabetic patients. The Journal of Thoracic and Cardiovascular Surgery, 145(4), 1083–1087. [Google Scholar] [PubMed]
16. Jenkins, K. J., Gauvreau, K. (2002). Center-specific differences in mortality: Preliminary analyses using the risk adjustment in congenital heart surgery (RACHS-1) method. The Journal of Thoracic and Cardiovascular Surgery, 124(1), 97–104. [Google Scholar] [PubMed]
17. Kellum, J. A., Lameire, N., (2013). KDIGO AKI guideline work group. diagnosis, evaluation, and management of acute kidney injury: A KDIGO summary (Part 1). Critical Care, 17(1), 204. [Google Scholar] [PubMed]
18. Polito, A., Thiagarajan, R. R., Laussen, P. C., Gauvreau, K., Agus, M. S. et al. (2008). Association between intraoperative and early postoperative glucose levels and adverse outcomes after complex congenital heart surgery. Circulation, 118(22), 2235–2242. [Google Scholar] [PubMed]
19. Lheureux, O., Prevedello, D., Preiser, J. C. (2019). Update on glucose in critical care. Nutrition, 59, 14–20. [Google Scholar] [PubMed]
20. Sedeek, M., Nasrallah, R., Touyz, R. M., Hébert, R. L. (2013). NADPH oxidases, reactive oxygen species, and the kidney: Friend and foe. Journal of the American Society of Nephrology, 24(10), 1512–1518. [Google Scholar] [PubMed]
21. Han, H. J., Lee, Y. J., Park, S. H., Lee, J. H., Taub, M. (2005). High glucose-induced oxidative stress inhibits Na+/glucose cotransporter activity in renal proximal tubule cells. American Journal of Physiology. Renal Physiology, 288(5), F988–F996. [Google Scholar] [PubMed]
22. van Niekerk, G., Davis, T., Engelbrecht, A. M. (2017). Hyperglycaemia in critically ill patients: The immune system’s sweet tooth. Critical Care, 21(1), 202. [Google Scholar] [PubMed]
23. Monnier, L., Mas, E., Ginet, C., Michel, F., Villon, L. et al. (2006). Activation of oxidative stress by acute glucose fluctuations compared with sustained chronic hyperglycemia in patients with type 2 diabetes. JAMA, 295(14), 1681–1687. [Google Scholar] [PubMed]
24. Dungan, K. M., Braithwaite, S. S., Preiser, J. C. (2009). Stress hyperglycaemia. Lancet, 373(9677), 1798–1807. [Google Scholar] [PubMed]
25. Yoo, S., Lee, H. J., Lee, H., Ryu, H. G. (2017). Association between perioperative hyperglycemia or glucose variability and postoperative acute kidney injury after liver transplantation: A retrospective observational study. Anesthesia and Analgesia, 124(1), 35–41. [Google Scholar] [PubMed]
26. Najmaii, S., Redford, D., Larson, D. F. (2006). Hyperglycemia as an effect of cardiopulmonary bypass: Intra-operative glucose management. The Journal of Extra-Corporeal Technology, 38(2), 168–173. [Google Scholar] [PubMed]
27. Galindo, R. J., Fayfman, M., Umpierrez, G. E. (2018). Perioperative management of hyperglycemia and diabetes in cardiac surgery patients. Endocrinology and Metabolism Clinics of North America, 47(1), 203–222. [Google Scholar] [PubMed]
28. Riad, M., Mogos, M., Thangathurai, D., Lumb, P. D. (2002). Steroids. Current Opinion in Critical Care, 8(4), 281–284. [Google Scholar] [PubMed]
29. Hill, K. D., Kannankeril, P. J., Jacobs, J. P., Baldwin, H. S., Jacobs, M. L. et al. (2022). Methylprednisolone for heart surgery in infants–A randomized, controlled trial. The New England Journal of Medicine, 387(23), 2138–2149. [Google Scholar] [PubMed]
30. Lomivorotov, V., Kornilov, I., Boboshko, V., Shmyrev, V., Bondarenko, I. et al. (2020). Effect of intraoperative dexamethasone on major complications and mortality among infants undergoing cardiac surgery: The DECISION randomized clinical trial. JAMA, 323(24), 2485–2492. [Google Scholar] [PubMed]
31. Falcao, G., Ulate, K., Kouzekanani, K., Bielefeld, M. R., Morales, J. M. et al. (2008). Impact of postoperative hyperglycemia following surgical repair of congenital cardiac defects. Pediatric Cardiology, 29(3), 628–636. [Google Scholar] [PubMed]
32. Yates, A. R., Dyke2nd, P. C., Taeed, R., Hoffman, T. M., Hayes, J. et al. (2006). Hyperglycemia is a marker for poor outcome in the postoperative pediatric cardiac patient. Pediatric Critical Care Medicine, 7(4), 351–355. [Google Scholar] [PubMed]
33. O’BrienJr, J. E., Marshall, J. A., Tarrants, M. L., Stroup, R. E., Lofland, G. K. (2010). Intraoperative hyperglycemia and postoperative bacteremia in the pediatric cardiac surgery patient. The Annals of Thoracic Surgery, 89(2), 578–584. [Google Scholar] [PubMed]
34. Zeng, Z. H., Yu, X. Y., Liu, X. C., Liu, Z. G. (2022). Effect of CPB glucose levels on inflammatory response after pediatric cardiac surgery. BMC Cardiovascular Disorders, 22(1), 222. [Google Scholar] [PubMed]
35. Marik, P. E., Bellomo, R. (2013). Stress hyperglycemia: An essential survival response! Critical Care, 17(2), 305. [Google Scholar] [PubMed]
36. Depre, C., Vanoverschelde, J. L., Taegtmeyer, H. (1999). Glucose for the heart. Circulation, 99(4), 578–588. [Google Scholar] [PubMed]
37. de Ferranti, S., Gauvreau, K., Hickey, P. R., Jonas, R. A., Wypij, D. et al. (2004). Intraoperative hyperglycemia during infant cardiac surgery is not associated with adverse neurodevelopmental outcomes at 1, 4, and 8 years. Anesthesiology, 100(6), 1345–1352. [Google Scholar] [PubMed]
38. DeCampli, W. M., Olsen, M. C., Munro, H. M., Felix, D. E. (2010). Perioperative hyperglycemia: Effect on outcome after infant congenital heart surgery. The Annals of Thoracic Surgery, 89(1), 181–185. [Google Scholar] [PubMed]
39. Sim, M. A., Liu, W., Ng, R. R., Ti, L. K., Chew, S. T. (2015). Wider perioperative glycemic fluctuations increase risk of postoperative acute kidney injury: A prospective cohort study. Medicine, 94(44), e1953. [Google Scholar] [PubMed]
40. Mayer-Davis, E. J., Kahkoska, A. R., Jefferies, C., Dabelea, D., Balde, N. et al. (2018). ISPAD clinical practice consensus guidelines 2018: Definition, epidemiology, and classification of diabetes in children and adolescents. Pediatric Diabetes, 19(Suppl 27), 7–19. [Google Scholar] [PubMed]
41. Güemes, M., Rahman, S. A., Hussain, K. (2016). What is a normal blood glucose? Archives of Disease in Childhood, 101(6), 569–574. [Google Scholar]
42. Crofton, P. M., Midgley, P. C. (2004). Cortisol and growth hormone responses to spontaneous hypoglycaemia in infants and children. Archives of Disease in Childhood, 89(5), 472–478. [Google Scholar] [PubMed]
43. Hagman, E., Reinehr, T., Kowalski, J., Ekbom, A., Marcus, C. et al. (2014). Impaired fasting glucose prevalence in two nationwide cohorts of obese children and adolescents. International Journal of Obesity, 38(1), 40–45. [Google Scholar] [PubMed]
44. Kanthimathinathan, H. K., Sundararajan, S. B., Laker, S., Scholefield, B. R., Morris, K. P. (2015). Targeting glycemic control after pediatric cardiac surgery: The influence of Age on insulin requirement. Pediatric Critical Care Medicine, 16(9), 853–858. [Google Scholar] [PubMed]
45. Moga, M. A., Manlhiot, C., Marwali, E. M., McCrindle, B. W., van Arsdell, G. S. et al. (2011). Hyperglycemia after pediatric cardiac surgery: Impact of age and residual lesions. Critical Care Medicine, 39(2), 266–272. [Google Scholar] [PubMed]
46. Ricci, Z., Raggi, V., Marinari, E., Vallesi, L., di Chiara, L. et al. (2022). Acute kidney injury in pediatric cardiac intensive care children: Not all admissions are equal: A retrospective study. Journal of Cardiothoracic and Vascular Anesthesia, 36(3), 699–706. [Google Scholar] [PubMed]
Supplementary Materials
Cite This Article
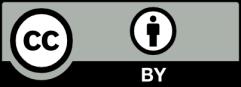