Open Access
ARTICLE
Anatomic Correlates of Mitral Systolic Anterior Motion in Transposition of the Great Arteries Following Atrial Switch Operation
1 Cardiovascular Division, Leon H. Charney Division of Cardiology, NYU Langone Health, School of Medicine, New York University Grossman, New York, USA
2 Department of Cardiovascular Disease, Medical School, The University of Minnesota, Minneapolis, USA
3 The Lillehei Heart Institute, Minneapolis, MN, USA
4 Radiology Department, NYU Langone Health, School of Medicine, New York University Grossman, New York, USA
5 Cardiothoracic Surgery Department, NYU Langone Health, School of Medicine, New York University Grossman, New York, USA
6 Pediatric Cardiology Division, NYU Langone Health, School of Medicine, New York University Grossman, New York, USA
7 Department of Internal Medicine, NYU Langone Health, School of Medicine, New York University Grossman, New York, USA
* Corresponding Author: Dan G. Halpern. Email:
Congenital Heart Disease 2023, 18(3), 267-277. https://doi.org/10.32604/chd.2023.025853
Received 02 August 2022; Accepted 16 November 2022; Issue published 09 June 2023
Abstract
Introduction: We sought to investigate whether the development of sub-pulmonic systolic anterior motion (SAM) may be inherent to the anatomy of the the mitral valve (MV) or affected by external factors, such as a dilated right ventricle or chest abnormalities in d-looped transposition of the great arteries post atrial switch operation (d-TGA/AtS). Methods: Analysis was performed of clinical and cardiac imaging studies acquired on 19 adult patients with d-TGA/AtS (age 42 ± 6 years old, 56% male) between 2015–2019. Echocardiography data included mitral apparatus anatomy, and CT/MRI data included biventricular dimensions, function, and Haller index (HI) for pectus deformity. Results: Patients with leaflet SAM (n = 6) compared to patients without SAM (n = 13) had higher MV protrusion height (2.3 ± 0.5 vs. 1.5 ± 0.4 cm, p ≤ 0.01) and longer anterior MV leaflet length (3.1 ± 0.4 cm vs. 2.6 ± 0.3 cm p ≤ 0.05), when compared to those without. CT/MRI showed higher sub-pulmonic left ventricular ejection fraction (LVEF) in the SAM group (71% ± 8% vs. 54% ± 7%, respectively). RV size and function, significant chest deformity (HI > 3.5), presence of a ventricular lead pacemaker, and septal thickness did not play a role in development of SAM. Conclusions: An elongated mitral apparatus is associated with the development of SAM, and the development of left ventricular outflow tract obstruction (LVOTO), in d-TGA/AtS. LV hyperkinesia is associated with SAM. Systemic RV dimensions, septal thickness, and degree of chest deformity did not differ significantly between subjects with SAM and those without.Keywords
The development of systolic anterior motion (SAM) of the mitral valve has been linked to abnormalities of the mitral apparatus, such as leaflet elongation and chordal slack, anterior displacement of the mitral leaflets or papillary muscles, and abnormal ventricular septal geometry in which the ventricular inflow overlaps with the outflow tract [1,2]. The severity and clinical consequence of SAM is related to the duration of mitral-septal contact, and can range from life-threatening, due to significant left ventricular outflow tract obstruction (LVOTO), to an incidental finding. These mechanisms have been extensively studied in hypertrophic cardiomyopathy (HCM); they provide the pathophysiological rationale for the resect-plicate-release operation, in which septal myectomy and ancillary anterior mitral leaflet shortening by plication are performed together with papillary muscle release in selected patients [3].
SAM in conditions other than HCM is less well studied. In congenital heart diseases, SAM of the subpulmonic mitral valve has been reported in cases of both d-TGA post atrial switch (i.e., Mustard or Senning) and congenitally corrected transposition of the great arteries (cc-TGA or L-TGA). In both conditions, SAM develops when the large, hypertrophied systemic subaortic right ventricle (RV) displaces the interventricular septum towards the subpulmonic LV, causing inflow/outflow region overlap [4]. In contrast to systemic SAM, subpulmonic SAM may produce subpulmonic obstruction and mitral regurgitation. Overall, there is evidence to suggest that subpulmonic LVOTO may be beneficial for systemic RV and left-sided tricuspid valve function by limiting RV dilation and allowing for better coaption of the tricuspid valve. In this investigation, we sought to identify anatomical factors that affect SAM in patients with d-looped transposition of the great arteries post atrial switch (d-TGA/AtS). While the atrial switch techniques are rarely used in the current era due to advent of the arterial switch operation (ASO)—there are a large number of adults with atrial switch palliation who have reached adulthood. We sought to investigate whether the development of SAM may be inherent to the anatomy of the MV or affected by external factors, such as a dilated right ventricle or pectus deformity.
2.1 Mitral Apparatus Evaluation from Cardiac Imaging
Anatomic measurements were performed on Syngo and PACS for echocardiographic and cardiac MRI data, respectively. Measurements were done twice by blinded cardiac imagers. Two-dimensional echocardiography measurements were performed according to guidelines from the American Society of Echocardiography (ASE) guidelines [5]. Echocardiography data consisted of mitral apparatus anatomy, including anterior and posterior mitral leaflet length (mm), mitral coaptation point to septal distance (mm), mitral coaptation point to posterior wall distance (mm), mitral regurgitation grade (0–4, ranges from 0—no regurgitation to 4—severe regurgitation), tricuspid regurgitation grade (0–4) (Figs. 1 and 2) [6]. Distances were measured between the mitral valve coaptation point and the anteroseptum and the posterior wall on the parasternal long-axis view. Also in this view, anterior mitral leaflet (AML) length was measured in diastole from the tip of the valve to the aortic annulus, and protrusion height was measured at coaptation from the most protruding mitral leaflet tip to the mitral annular plane. SAM was defined as displacement of the distal AML into the LVOT during systole. LVOT velocity measured by Doppler ultrasound according to guidelines from the ASE guidelines [5]. CT data included biventricular dimensions, ventricular function, and Haller index (HI), which is calculated by dividing the transverse diameter of the chest by the anterior-posterior distance in CT of the chest [7]. MRI was used to verify biventricular function as ejection fraction. The study was approved by the institutional review board of New York University School of Medicine.
Figure 1: Transthoracic echocardiography mitral valve measurements in a d-looped transposition of the great arteries, in the parasternal long axis view
Note: AML = anterior mitral leaflet, PML = posterior mitral leaflet, PH = protrusion height (measured in early systole from the coapted leaflet tip to the mitral annular plane). LV = left ventricle, RV = right ventricle, AV = aortic valve, PV = pulmonary valve, S-CP = Septal to Mitral coapt distance, CP-PW = Mitral Coapt to Posterior wall.
Figure 2: Representative echocardiogram in the parasternal long axis view, of a patient with d-TGA/AtS and SAM with LVOTO
In this frame, both the PML and AML are elongated and show bileaflet SAM with complete obstruction of blood flow through the PV during mid to late ventricular systole; pressure gradient measured at 110 mmHg.
Data were expressed as mean ± standard deviation (SD), unless stated otherwise. Student’s t-test was used to detect differences in AML, mitral valve protrusion height and EF. Subgroup analysis was performed within a one-way ANOVA with post hoc testing (i.e., Bonferroni correction for homogenous variables or Dunnett T3 for non-homogenous variables), which was used to detect differences between groups. Data was analyzed using GraphPad Prism 8 for Windows (San Diego, USA). A p value < 0.05 was considered statistically significant.
In this retrospective cohort study, we included 19 adult patients with d-looped transposition of the great arteries, a single discordant ventriculo-arterial connection, who are post-surgical atrial switch. For inclusion in the study, patients were 18 years or older, and were excluded if the d-TGA was repaired with surgery other than atrial switch (i.e., arterial switch operation or Rastelli) or if they had a hypoplastic ventricle. Clinical factors, including age in years, gender assigned at birth, height (cm), weight (kg), body surface area (BSA), medications, presence of pacemaker, baseline heart rhythm, and comorbidities, were obtained from chart review and are included in (Table 1). For analysis, subjects were separated into three groups: no SAM, the presence of SAM, and SAM with LVOTO. Furthermore, cases with other causes of LVOTO (e.g., pulmonary stenosis) were excluded.
Study participants were aged 42 [6] years, predominantly male (58%), and Caucasian (84%), as reported in (Table 1). Thirteen patients had a Mustard procedure, four had a Senning procedure, one had a Senning procedure with Shumaker Modification, and one patient had an uncharacterized procedure (Supplemental Table 1). Three patients had ventricular septal defects (Supplemental Table 1). Participants were assessed by non-invasive mean systolic blood pressure (116 [15], mmHg) and diastolic pressure (67 [10], mmHg) at time of imaging. Participants were generally free of traditional cardiovascular risk factors (Table 2). Half of the population had pre-existing pacemaker implantation (50%), of which 26% had a lead traversing into the LV. Baseline rhythm was predominantly sinus (53%), followed by paced rhythm (37%) (Table 2). The most used cardiac medications were ACE-inhibitors (47%) and beta-blockers (63%) (Table 2). Of note, two of the three patients with SAM and LVOTO were on beta-blockade. None of the subjects with non-obstructive leaflet SAM were on beta-blockers.
3.3 Qualitative and Quantitative Assessment of SAM in the Left Ventricle
Mitral valve protrusion height (Fig. 1) differed between patients with SAM (n = 6), SAM with LVOTO (n = 3), and without SAM (n = 13) (2.2 [0.5]cm vs. 2.3 [0.4]cm vs. 1.5 [0.4]cm, respectively, p-interaction < 0.01, Table 3). This difference was driven by the presence of SAM (p < 0.05). AML length differed between patients with SAM, SAM with LVOTO, and without SAM (3.1 [0.4]cm vs. 3.4 [0.4]cm vs. 2.6 [0.3]cm, respectively, p-interaction < 0.05). AML was driven by differences between patients with SAM with LVOTO and those without SAM (p < 0.05). No difference was seen when those with pulmonic stenosis (n = 2) were separated from the analysis, relative to when they were included in the no-SAM group. Left ventricular ejection fraction (LVEF) differed between patients with SAM, SAM with LVOTO, and without SAM (71 [8]% vs. 73 [9]% vs. 54 [7]%, respectively, p < 0.0005) (Table 4). Patients with SAM and LVOTO had longer AML, and this was driven by differences between patients with SAM and those without (p < 0.05). There were no observed differences in posterior mitral leaflet lengths (PML), mitral coapt to septal distance, indexed left ventricular end diastolic volume (LVEDVi), or mitral coapt to wall distance. There was too little MR to qualitatively obtain pulmonary pressure; however, qualitatively there was no evidence of pulmonary hypertension by septal position. Notably, both leaflet and chordal SAM could occur in the absence of significant LVOT velocity as measured by Doppler ultrasound (Table 3).
3.4 Qualitative Assessment of the Right Ventricle and Chest Anatomy on SAM
Among subjects with SAM, SAM with LVOTO, and no SAM, RV Stroke volume (86 [24]mL vs. 77 [29]mL vs. 78 [25]mL, p = 0.6, respectively), RVEF (41 [16]% vs. 43 [22]% vs. 40 [14]%, p = 0.99, respectively), RVEDVi measured (120 [26]mL vs. 110 [24]mL vs. 118 [37]mL, respectively, p = 0.85), and Haller Index (3.3 [0.9] vs. 3.0 [0.6] vs. 2.9 [0.7], p = 0.7, respectively) did not differ significantly between groups (Table 4). In order to illustrate the biventricular interaction, the RV/LV ratio was also evaluated and found to have no statistical significance.
3.5 Effect of Pacemaker on SAM
Subgroup analysis was performed on patients with pacemakers relative to those without to evaluate if those with pacemakers had a higher rate of SAM. Of those with SAM, four had pacemakers (one ventricular paced, two atrial paced, and one dual chamber rate modulated) and five did not. Of those without SAM, seven had pacemakers and three did not. There was no association between pacemakers and SAM (p = 0.37). The presence of pacemakers did not have a significant effect on limiting the interpretation of imaging measurements and there was no tethering of the mitral valve.
Although there have been case reports which describe the presence of SAM in patients with a systemic RV, this retrospective review of clinical and cardiac imaging data is the first study to our knowledge to examine anatomical correlations of SAM in adult patients with d-TGA/AtS, in a systematic fashion [8,9]. The primary findings of this investigation are: (1) SAM is prevalent in the d-TGA/AtS population (6/19 32%); (2) SAM is associated with more elongated AML and a higher protrusion height; (3) LVOTO is observed in subjects with a longer AML leaflet and MV protrusion height; (4) SAM is associated with hyperdynamic LV function; (5) systemic right heart global ventricle factors, such as volume or function, did not correlate with SAM in this cohort; (6) chest deformity producing left lateral displacement of the heart into the left hemithorax did not affect the presence of SAM and (7) the presence of a pacemaker was not associated with the presence of SAM. Taken together, these observations suggest a primary intrinsic mitral valve apparatus mechanism to explain SAM in d-TGA/AtS.
The primary association with SAM observed in this cohort involved mitral anatomy, specifically a longer AML and higher MV protrusion height. On average, in patients with HCM and obstruction from SAM, the average MV protrusion height is 2.6 cm, compared to 1.9 cm in non-obstructive HCM and 1.3 cm in normal hearts [10]. This data shows that, in normal hearts, the mitral valve coapts in the mitral annular plane while in obstructive HCM it protrudes into the LV where it is subject to abnormal flow vectors early in systole. In our cohort, protrusion height was 2.2 cm in patients with SAM and 1.5 cm in patients without SAM. Analogously, the AML was 3.4 ± 0.2 cm in patients with SAM and LVOTO, compared to 2.6 ± 0.3 in those without SAM. The constellation of a reduced septo-mitral distance with anteriorly displaced papillary muscles predisposes to SAM in d-TGA/AtS patients but does not explain why it occurs in particular patients though a higher MV protrusion height appears to be the cause. Of the three patients in our study with leaflet SAM and LVOTO, all had mitral regurgitation. Of those patients in our study with leaflet SAM but no LVOTO, all had mitral regurgitation. All patients without SAM had mitral regurgitation with the exception of one who had no mitral regurgitation. It does not seem that in our cohort mitral regurgitation made a difference on the occurrence of SAM.
Most of the current understanding of SAM and its mechanism is derived from observations of patient populations with HCM, in which SAM is best characterized, and which was originally believed to be pathognomonic for the disease. Drag forces, the pushing force of flow on the valve which can occur at low velocity (unlike the Venturi effect), are thought to play a dominant role in the development of SAM [10–13]. SAM has previously been found to occur in patients with excessive MV leaflet tissue, as this tissue provides a large surface area on which drag and vortex forces can act to cause SAM, with or without LVOTO. Some studies have shown that elongation of the AML can be associated with SAM in patients with normal LV anatomy without hypertrophy, particularly during stress testing [14,15]. Changes in posterior leaflet height have also been proposed to cause SAM, by shifting the mitral apparatus anteriorly. Subsequent studies showed that malpositioning of the posterior leaflet of the mitral valve can result in SAM, even in low flow, due to drag forces as described above [13]. In this cohort, both leaflet and chordal SAM occurred in the absence of a significant increase in Doppler LVOT velocity. Recently, positioning of the anterior and posterior mitral leaflets has been shown to be connected to flow vortices occurring in late diastole, which help to pre-position the leaflets, subsequently causing SAM in patients with hypertrophic cardiomyopathy (HCM) [16]. SAM has also been associated with anterior and basilar displacement of the base of the anterolateral papillary muscle and muscular connections between the head of the anterolateral papillary muscle and the anterolateral wall in patients with obstructive HCM [14].
LV hyperkinesia was observed in patients with obstruction. SAM occurs very early in systole in patients with severe obstruction and is caused by early LV systolic ejection flow acceleration that catches the valve and sweeps it into the septum in early systole [17]. LV hyperkinesia increases the hydrodynamic drag force on the mitral valve by the square of the instantaneous velocity, predisposing to SAM. This phenomenon has been observed after administration of positively inotropic drugs. Of note, in this cohort, there was too little MR to qualitatively obtain pulmonary pressure; however, there was no evidence of pulmonary hypertension by septal position.
Like SAM in HCM, sub-pulmonic SAM in d-TGA/AtS patients has also been observed in the setting of ventricular abnormalities. Such anomalies include asymmetric septal hypertrophy, chordal abnormalities, and an abnormally shaped LV in d-TGA [18]. An important ventricular geometrical consideration in the presented cohort includes the pressurization of the systemic subaortic RV relative to the subpulmonic low-pressure LV, which results in. bulging of the IVS into the LV. This was observed in all subjects. Displacement of the IVS serves to bring the anterior mitral leaflet closer into the LVOT during systole and may serve to trigger LVOTO [9,19]. Previous studies have shown the relative frequency of mitral valve anomalies in patients with d-TGA/AtS [20]. One study demonstrated that in infants who had undergone AtS, 4% were found to have MV anomalies, including anterior displacement of the MV toward the LVOT and rotation of the MV leaflets so that the commissure is oriented towards the anterior ventricular septum [20]. Our study, while limited in size, shows a higher prevalence of SAM (32%, 16% with obstruction), which is associated primarily with mitral valve abnormalities, as described above.
In this cohort, the LV faces a lower pressure than the RV; mechanistically, this study builds upon previous research showing that SAM does not require the presence of high flow velocity, as previously postulated. The pressurized RV, with the interventricular septum bulging into the lower pressure LV, reduces LV cavity size further and reduces the distance between the mitral apparatus and the interventricular septum, promoting SAM. Prior observations showed a clinical advantage of pressurizing the sub-pulmonic LV in patients with systemic subaortic RVs (i.e., d-TGA/AtS or cc-TGAs) by shifting the septum towards the systemic RV, decreasing RV sphericity, thus increasing its efficiency and improving tricuspid valve coaptation. Moreover, reports show that patients with cc-TGA, a double discordant lesion (both atrioventricular and ventriculo-arterial), who underwent relief of pulmonic obstruction had paradoxical increase in systemic tricuspid regurgitation (TR) and decrease in systemic RV function [21]. However, a critical difference between the pressurization of the LV that occurs due to pulmonic stenosis or subpulmonic stenosis and the pressurization which occurs from SAM, is that the latter only causes this pressurization during late systole and not during the entire cardiac cycle [22]. Notably, the exclusion of two patients with pulmonic stenosis did not significantly alter the analysis presented, and these patients were included in the no-SAM groups. Theoretically, increasing the afterload to the sub-pulmonic LV by the creation of pulmonary arterial stenosis (i.e., placement pulmonary artery band) may reduce SAM. Thus, the effect of septal deviation towards the systemic RV has not been elucidated in this cohort. In addition, there were no associated differences in RV size, function, atrial and/or ventricular pacing or degree of tricuspid regurgitation in patients with SAM, relative to those without SAM. However, the presence of SAM was associated with a hyperdynamic subpulmonic LV.
Finally, although few studies exist in regard to pharmacological treatment of patients with sub-pulmonic SAM, use of beta-blockade was shown to mildly reduce LVOT gradients and mitral regurgitation in patients with d-TGA/AtS. Of note, however, d-TGA/AtS patients commonly have sinus node dysfunction and thus beta-blockade usage carries higher risk in this population [23].
Given the small number of patients in the study, generalization to the whole population of d-TGA/AtS should be made with caution.
6 Conclusions and Future Directions
Patients with a history of d-TGA/AtS have a higher prevalence of SAM than previously reported. This study demonstrates that those with a higher mitral protrusion height, longer AML and higher LVEF are more likely to have associated SAM. The pressurized systemic RV causes a septal shift that, in the right constellation of elongated MV apparatus findings, creates SAM. The clincical significance of SAM in this patient population remains unclear and larger studies with longer follow-up may demonstrate the potential risks of SAM in d-TGA/AtS patients.
Funding Statement: The authors received no specific funding for this study.
Author Contributions: The authors confirm contribution to the paper as follows: study conception and design: Aiad and Halpern; data collection: Aiad, Elnabawi, and Halpern; analysis and interpretation of results: all authors; draft manuscript preparation: all authors. All authors reviewed the results and approved the final version of the manuscript.
Availability of Data and Materials: Data available on request from Dr. Dan Halpern, MD at Dan.Halpern@nyulangone.org.
Ethics Approval:: The study was approved by the institutional review board of New York University School of Medicine.
Conflicts of Interest: The authors declare that they have no conflicts of interest to report regarding the present study.
References
1. Raut, M., Maheshwari, A., Swain, B. (2018). Awareness of ‘Systolic Anterior Motion’ in different conditions. Clinical Medicine Insights: Cardiology, 12, 117954681775192. [Google Scholar]
2. Halpern, D. G., Swistel, D. G., Ricardo Po, J., Joshi, R., Winson, G. et al. (2015). Echocardiography before and after resect-plicate-release surgical myectomy for obstructive hypertrophic cardiomyopathy. Journal of the American Society of Echocardiography, 28(11), 1318–1328. [Google Scholar] [PubMed]
3. Silverman, N. H., Payot, M., Stanger, P., Rudolph, A. M. (1978). The echocardiographic profile of patients after Mustard’s operation. Circulation, 58(6), 1083–1093. [Google Scholar] [PubMed]
4. Sherrid, M. V., Männer, J., Swistel, D. G., Olivotto, I., Halpern, D. G. (2020). On the cardiac loop and its failing: Left ventricular outflow tract obstruction. Journal of the American Heart Association, 9(3), e014857. [Google Scholar] [PubMed]
5. Cohen, M. S., Eidem, B. W., Cetta, F., Fogel, M. A., Frommelt, P. C. et al. (2016). Multimodality imaging guidelines of patients with transposition of the great arteries: A report from the American society of echocardiography developed in collaboration with the society for cardiovascular magnetic resonance and the society of cardiovascular computed tomography. Journal of the American Society of Echocardiography, 29(7), 571–621. [Google Scholar] [PubMed]
6. Halpern, D. G., Swistel, D. G., Po, J. R., Joshi, R., Winson, G. et al. (2015). Echocardiography before and after resect-plicate-release surgical myectomy for obstructive hypertrophic cardiomyopathy. Journal of the American Society of Echocardiography, 28(11), 1318–1328. [Google Scholar] [PubMed]
7. Daunt, S. W., Cohen, J. H., Miller, S. F. (2004). Age-related normal ranges for the Haller index in children. Pediatric Radiology, 34(4), 326–330. [Google Scholar] [PubMed]
8. Vitarelli, A., D’Addio, P., Gentile, R., Burattini, M. (1984). Echocardiographic evaluation of left ventricular outflow tract obstruction in complete transposition of the great arteries. American Heart Journal, 108, 531–538. [Google Scholar] [PubMed]
9. Kirmani, B. H., Moideen, I., Fernandez-Jimenez, P., Bewsher, M., Dua, J. et al. (2016). Systolic anterior motion obstructing the pulmonary outflow tract after tricuspid valve replacement. The Annals of Thoracic Surgery, 101(4), 1580–1581. [Google Scholar] [PubMed]
10. Ro, R., Halpern, D., Sahn, D. J., Homel, P., Arabadjian, M. et al. (2014). Vector flow mapping in obstructive hypertrophic cardiomyopathy to assess the relationship of early systolic left ventricular flow and the mitral valve. Journal of the American College of Cardiology, 64(19), 1984–1995. [Google Scholar] [PubMed]
11. Ibrahim, M., Rao, C., Ashrafian, H., Chaudhry, U., Darzi, A. et al. (2012). Modern management of systolic anterior motion of the mitral valve. European Journal of Cardio-Thoracic Surgery, 41(6), 1260–1270. [Google Scholar] [PubMed]
12. Swistel, D. G., Sherrid, M. V. (2017). The surgical management of obstructive hypertrophic cardiomyopathy: The RPR procedure-resection, plication, release. Annals of Cardiothoracic Surgery, 6(4), 423–425. [Google Scholar] [PubMed]
13. Sherrid, M. V., Gunsburg, D. Z., Moldenhauer, S., Pearle, G. (2000). Systolic anterior motion begins at low left ventricular outflow tract velocity in obstructive hypertrophic cardiomyopathy. Journal of the American College of Cardiology, 36(4), 1344–1354. [Google Scholar] [PubMed]
14. Sherrid, M. V., Balaram, S., Kim, B., Axel, L., Swistel, D. G. (2016). The mitral valve in obstructive hypertrophic cardiomyopathy: A test in context. Journal of the American College of Cardiology, 67(15), 1846–1858. [Google Scholar] [PubMed]
15. He, S., Hopmeyer, J., Lefebvre, X. P., Schwammenthal, E., Yoganathan, A. P. et al. (1997). Importance of leaflet elongation in causing systolic anterior motion of the mitral valve. The Journal of Heart Valve Disease, 6(2), 149–159. [Google Scholar] [PubMed]
16. Levine, R. A., Schwammenthal, E., Song, J. K. (2014). Diastolic leading to systolic anterior motion: New technology reveals physiology. Journal of the American College of Cardiology, 64(19), 1996–1999. [Google Scholar] [PubMed]
17. Sherrid, M. V., Pearle, G., Gunsburg, D. Z. (1998). Mechanism of benefit of negative inotropes in obstructive hypertrophic cardiomyopathy. Circulation, 97(1), 41–47. [Google Scholar] [PubMed]
18. Lintermans, J. P. (1981). Differential diagnosis in pediatric echocardiography. Berlin, Heidelberg: Springer. [Google Scholar]
19. Hornung, T. S., Calder, L. (2010). Congenitally corrected transposition of the great arteries. Heart, 96(14), 1154–1161. [Google Scholar] [PubMed]
20. Camarda, J., Harris, S., Hambrook, J., Tweddell, J., Frommelt, P. (2012). Anatomic mitral valve anomalies in D-transposition of the great arteries: ECHO findings and impact on arterial switch repair technique and outcome. Journal of the American College of Cardiology, 59(13), E804. [Google Scholar]
21. Halpern, D. G., Nathan, M., Yaacov, O., Marx, G. (2016). Tricuspid valve reconfiguration following pulmonary artery banding in patients with congenitally corrected transposition of the great arteries as seen on echocardiography. Journal of the American College of Cardiology, 67(13), 977. [Google Scholar]
22. Buber, J., Mcelhinney, D. B., Valente, A. M., Marshall, A. C., Landzberg, M. J. (2015). Tricuspid valve regurgitation in congenitally corrected transposition of the great arteries and a left ventricle to pulmonary artery conduit. The Annals of Thoracic Surgery, 99, 1348–1356. [Google Scholar] [PubMed]
23. Stachel, M., Halpern, D., Saric, M. (2019). Management of dyanmic subpulmonuc left ventrcular outflow tract obstruction in a patient with dextro-transposition of the great arteries following atrial switch repair. Journal of the American College of Cardiology, 73(9), 2294. [Google Scholar]
Cite This Article
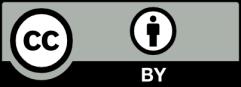
This work is licensed under a Creative Commons Attribution 4.0 International License , which permits unrestricted use, distribution, and reproduction in any medium, provided the original work is properly cited.