Open Access
ARTICLE
Preoperative Fasting of More Than 14 Hours Increases the Risk of Time-to-Death after Cardiothoracic Surgery in Children: A Retrospective Cohort Study
Department of Anesthesiology, Faculty of Medicine, Prince of Songkla University, Hat Yai, Songkhla, 90110, Thailand
* Corresponding Author: Maliwan Oofuvong. Email:
Congenital Heart Disease 2023, 18(1), 23-39. https://doi.org/10.32604/chd.2023.026026
Received 11 August 2022; Accepted 20 September 2022; Issue published 09 January 2023
Abstract
Background: Prolonged preoperative fasting can cause hypoglycemia, hyperglycemia, and intravascular volume depletion in children. We aimed to examine whether prolonged preoperative fasting is associated with in-hospital mortality and other morbidities in pediatric cardiothoracic surgery. Methods: This retrospective cohort study included children aged 0–3 years who underwent cardiac surgery between July 2014 and October 2020. The patient demographic data, surgery-related and anesthesia-related factors, and postoperative outcomes, including hypoglycemia, hyperglycemia, sepsis, length of intensive care unit stay, and in-hospital mortality, were recorded. The main exposure and outcome variables were prolonged fasting and time-to-death after surgery, respectively. The associations between prolonged fasting and perioperative death were analyzed using multivariate Cox regression analysis. Results: In total, 402 patients were recruited. The incidence of perioperative mortality was 21% (85/402). The proportion of perioperative deaths was significantly higher in the prolonged fasting group than that in the normal fasting group. The proportion of postoperative bacteremia and hypoglycemia was significantly higher in the very prolonged fasting group than that in the prolonged fasting group. After adjusting for preoperative conditions and anesthesia- and surgery-related factors, preoperative prolonged fasting >14.4 h was significantly associated with time-to-death (HR [95% CI]: 2.2 [1.2, 3.9], p = 0.036). The 30-day survival rates of fasting time >14.4 h, 9.25–14.4 h, and <9.25 h were 0.67 (0.55, 0.81), 0.79 (0.72, 0.87), and 0.85 (0.79, 0.91), respectively. Conclusions: Preoperative fasting of more than 14.4 h was associated with a two-fold increase in the hazard rate of time-to-death in children who underwent cardiac surgery.Graphic Abstract
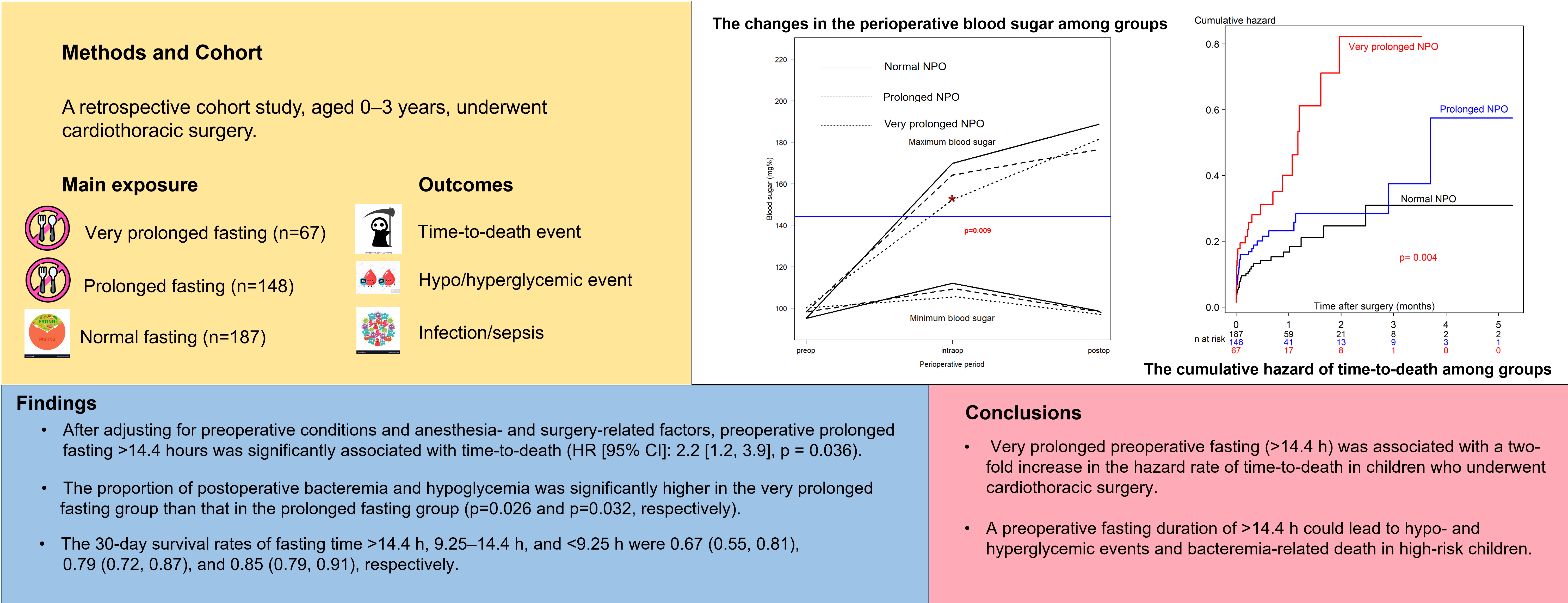
Keywords
The preoperative fasting guidelines before general anesthesia (8 h for solid foods, 6 h for infant formula, 4 h for breast milk, and 2 h for clear liquids), which were last updated in 2017, are considered by the American Society of Anesthesiologists (ASA) to reduce the risk of pulmonary aspiration [1]. Reports of children fasting for more than 12 h due to changes in surgery schedules or delays in operative time can cause hypercatabolic states, such as gluconeogenesis and ketogenesis [2–4], resulting in hypoglycemia, hyperglycemia, intravascular volume depletion, and difficult intravenous access [5]. Moreover, prolonged fasting can cause metabolic stress leading to postoperative hyperglycemia [6,7] and enhance the inflammatory markers of acute response after elective abdominal surgery [8,9]. However, studies reporting the effect of prolonged preoperative fasting on mortality in pediatric cardiac surgery are scarce. Therefore, we examined the association between prolonged preoperative fasting and in-hospital mortality in pediatric cardiac surgery patients.
This retrospective cohort study (Approval No. 6246383) was approved by the Human Research Ethics Committee, Faculty of Medicine, Prince of Songkla University, on May 19, 2020, and was in full compliance with the international guidelines of the Declaration of Helsinki. The requirement for written informed consent was waived by the ethics committee as this was a retrospective study. Medical and anesthetic records were obtained from the hospital information system of the Songklanagarind Hospital. All data were fully anonymized before being accessed by the investigators. Children aged 0–3 years who underwent cardiovascular and thoracic anesthesia between January 2014 and October 2020 at a super-tertiary care hospital were eligible to participate in this study. This manuscript adheres to the STROBE guidelines.
2.1 Standard Operating Procedure
Routine preoperative evaluation by anesthetic residents was performed on all delayed patients/patients’ caregivers on the day before the surgery regarding solid/infant formula fasting time and intravenous fluid. Preoperative laboratory tests related to surgery, such as complete blood count, electrolytes, blood sugar, and chest radiography (CXR), were performed. General anesthesia with endotracheal intubation was performed in all patients. Patients routinely received propofol, midazolam, or ketamine at the induction of anesthesia, and anesthesia was maintained with volatile anesthetic agents, such as fentanyl and cisatracurium. Standard monitoring, including non-invasive blood pressure, pulse oximetry, electrocardiography, and end-tidal carbon dioxide, were used for all patients. Additional arterial line or central venous catheterization was performed for those undergoing open heart surgery. The pediatricians routinely check the blood sugar every 4–6 h and perform complete blood count, electrolyte tests, and CXR daily in the pediatric intensive care unit (PICU). The patients were ventilated using the control ventilator mode until they regained consciousness. Endotracheal tube extubation was performed by a pediatric intensivist.
The primary exposure variable was preoperative fasting. Preoperative fasting time was calculated from the last solid/infant formula to the time of anesthesia induction. Since there is no definite definition of prolonged fasting among children, we used the two cut-off points that gave the highest sensitivity and specificity from the receiver operating characteristic (ROC) curve for perioperative mortality [10]. Subsequently, a prolonged fasting variable was constructed.
The primary outcome of this study was time-to-death, defined as the time from postoperative cardiac surgery to death during admission. Censored survival time was defined as the time from postoperative cardiac surgery to discharge. The secondary outcomes of the study were postoperative hypoglycemia, hyperglycemia, and pneumonia. Hyperglycemia was defined as a blood sugar level >180 mg% [11], and hypoglycemia was defined as a blood sugar level <50 mg% if the patients were aged at least 1 month [12] or <40 mg% if the patients were aged less than 1 month [13]. Postoperative pneumonia was defined as a new pulmonary infection and infiltration after the surgery. Other secondary outcomes included postoperative sepsis, duration of intensive care unit stay, and duration of mechanical ventilation.
Potential confounders included patient characteristics, such as age, weight, sex, and history of prematurity; preoperative conditions, such as pneumonia, sepsis, inotropic drug use, and intravenous fluid; surgery-related factors, such as elective or emergency cases, risk adjustment for congenital heart surgery (RACHS-1) category [14], use of cardiopulmonary bypass, duration of surgery, and intraoperative blood loss; and anesthesia-related factors such as ASA classification.
2.5 Definition of Explanatory Variables
Prematurity was defined as a gestational age less than 37 weeks at birth. Pneumonia was defined as an elevated white blood cell count, new infiltration on chest radiography, and positive endotracheal tube culture. Sepsis was defined as a systemic inflammatory response syndrome in the presence of, or because of, suspected or proven infection. The RACHS-1 category was based on the following risk adjustment for surgery for congenital heart disease: Category 1 included patients requiring surgery for patent ductus arteriosus (aged >30 days), coarctation of the aorta (aged >30 days), or atrial septal defect; Category 2 included patients requiring surgery for coarctation of the aorta (age <30 days), ventricular septal defect (VSD), tetralogy of Fallot, vascular ring, or aortopulmonary window; Category 3 included patients requiring arterial switch operation, surgery for tetralogy of Fallot/pulmonary atresia, Ross, double-outlet right ventricle repair, surgery of coarctation/VSD, Blalock–Taussig shunt, common atrioventricular canal repair, or pulmonary artery banding; Category 4 included patients requiring truncus arteriosus repair, double switch, hypoplastic arch repair, unifocalization, Rastelli procedure, arterial switch operation/VSD, or the Ross–Konno procedure; Category 5 included patients requiring surgery for Ebstein anomaly (aged <30 days) or truncus/interrupted aortic arch repair; and Category 6 included patients requiring the Norwood or Damus–Kaye–Stansel procedure.
For the primary outcome, we estimated the overall mortality rate of children undergoing cardiac surgery to be 20%. For survival analysis, a hazard ratio (r) for time-to-death of 1.5, which represents a clinically significant increase in hazard, was used to calculate the required sample size based on a significance level of 0.05 and power of 80% using the following formula [15,16]:
where p = 1 − pa (exp(−ln(2)F/m)), pa = {1 − exp(−ln(2)A/m)}/ln(2)A/m, F is the additional follow-up time after the end of recruitment = 150 days, A is the accrual time during which children were recruited into the study = 60 days, and m is the ratio of the number of unexposed participants to certain risk factors per exposed participant = 1. Using these parameters, the required sample size was calculated as 96 children for exposed cases and 96 children for unexposed cases. The total sample size was increased to 240 to account for a dropout rate of 20%.
For the secondary outcome, based on our pilot study, we estimated the incidence of postoperative hyperglycemia after cardiac surgery to be 20%. We assumed that the prevalence of hyperglycemia in the normal fasting group was 10%. Using the formula for comparing two independent proportions with a normal fasting group to a prolonged fasting group ratio of 1:1 to detect an odds ratio of at least 2.5, a significance level of 0.05, and a power of 80%, a minimum sample size of 168 prolonged and 168 normal fasting cases was required. Therefore, almost 6 years of data collection was adequate to obtain the complete required sample size.
Data record forms were created, and information was abstracted from electronic medical records. The R software (R version 4.1.1, R Core Team, Vienna, Austria) was used to analyze the data. Descriptive statistics are presented as frequencies with percentages and medians with interquartile ranges (IQR). The chi-square test or Fisher’s exact test was used to compare categorical variables. Continuous variables were compared using the Kruskal–Wallis test if more than two groups had to be compared. A post-hoc analysis was performed for multiple comparisons between groups when the overall differences were significant. The changes in the perioperative blood sugar among the fasting groups were compared using the generalized estimating equations method. To create an aggregate plot of the changes in the perioperative blood sugar, the missing data of perioperative blood sugar had to be replaced with the median values of each blood sugar period to accomplish the same duration between the periods and fasting groups.
A directed acyclic graph (DAG) was used to represent the potential causal relationships between the covariates (including prolonged fasting variables) and perioperative death using DAGitty software version 3.0 [17]. Potential confounding variables, including age, ASA classification, emergency surgery, prematurity, preoperative sepsis, preoperative inotropic drug use, and the RACHS-1 category suggested by DAG were then selected for a multivariate time-dependent Cox regression model, and each was retained irrespective of their statistical significance [18]. The association between prolonged fasting and time-to-death after surgery was presented as adjusted hazard ratios (HR) and 95% confidence intervals (CI) and was considered statistically significant if the p-value was <0.05. The effect of the modification between the potential predictors and prolonged fasting variables on the outcomes was evaluated for the final model.
A total of 402 children underwent cardiothoracic surgery during the study period. The majority of the missing data was observed in the preoperative blood sugar variable, which was not selected as a confounder by DAG; therefore, it was excluded from the initial multivariate Cox regression model. The majority of the participants (>80%) did not receive clear water after fasting. The median (IQR) fasting time was 580 (430–795) min. The median (IQR) age of patients was 2.1 (0.6 to 13.4) months. After plotting the ROC curve of fasting time for death (Appendix A), a cut-off point between 555 (9.25 h) and 865 (14.4 h) and a cut-off point >865 were considered as prolonged fasting time and very prolonged fasting time, respectively. Therefore, the prolonged fasting group comprised 148 patients, and the very prolonged fasting group comprised 67 patients (Fig. 1). The distribution of patients, anesthesia, and surgery-related factors is shown in Table 1. ASA classification, preoperative sepsis, receiving emergency surgery, preoperative inotropic drug use, and RACHS-1 category were significantly different between the prolonged, very prolonged, and normal fasting groups, for which a minimally sufficient adjusted set was observed in the DAG model.
Figure 1: Flow diagram of the study
The outcomes of the prolonged fasting and very prolonged fasting groups compared with those of the normal fasting group are shown in Table 2. The proportion of perioperative deaths was significantly higher in the prolonged fasting group than that in the normal fasting group (36% vs. 16%). The proportions of postoperative bacteremia, hypoglycemia, and reoperation were significantly higher in the very prolonged fasting group than those in the prolonged fasting group (42% vs. 25%, 13% vs. 4%, and 13% vs. 4%, respectively). Fig. 2 compares the changes in the perioperative minimum and maximum blood sugar among the prolonged fasting and very prolonged fasting groups compared with those of the normal fasting group. Regardless of the fasting condition, the overall minimum and maximum blood sugar were significantly higher during the intraoperative period (17 mg%, p < 0.001 and 74 mg%, p < 0.001, respectively). The maximum blood sugar was significantly higher during the postoperative period compared with that in the preoperative period (94 mg%, p < 0.001). Among the fasting subgroups, there was an interaction between the periods and the fasting condition group; on comparing the intraoperative period with the preoperative period, the maximum blood sugar was significantly lower in the very prolonged fasting group than that in the normal fasting group (23 mg%, p = 0.009), whereas there was no interaction between the postoperative periods and the fasting condition group (p > 0.05).
Figure 2: The changes in the perioperative minimum and maximum blood sugar among the prolonged fasting and very prolonged fasting groups compared with those in the normal fasting group. *p = 0.009 between the normal and very prolonged fasting groups during the intraoperative period compared with those in the preoperative period. preop, preoperative; intraop, intraoperative; postop, postoperative; NF, normal fasting; PF, prolonged fasting; VPF, very prolonged fasting
3.1 Prolonged Fasting and Perioperative Death and Time-to-Death Event
The related causes of perioperative death are shown in Appendix B, where the prolonged and very prolonged fasting groups were combined. The two most common postoperative deaths were cardiovascular-related (29%; low cardiac output syndrome, myocardial dysfunction, and cardiogenic shock) and infection-related (23%; sepsis, ventilator-related pneumonia, and necrotizing enterocolitis). The proportion of infection-related deaths was not significantly higher in the prolonged and very prolonged fasting than that in the normal fasting group (28% vs. 12%). The potential risk factors associated with perioperative death and the time-to-death are shown in Table 3.
3.2 DAG to Reduce Potential Bias in the Relationship between Prolonged Fasting and Perioperative Death
Fifteen variables (age, body weight at surgery, preoperative pneumonia and sepsis, history of prematurity, emergency surgery, ASA classification, preoperative inotropic drug use, preoperative electrolytes and blood sugar, intravenous fluid, risk adjustment for congenital heart surgery, duration of surgery, required cardiopulmonary bypass, and intraoperative blood loss) which may relate to prolonged fasting and perioperative death, were introduced in the network relationship of DAG. According to DAG, the potential confounders (the ancestors of the exposure and outcome) and the mediators between the exposure and the outcome were shown. We used the total effect of the DAG method to identify biasing pathways (Fig. 3A) that needed to be blocked by including the potential confounders in the final model for perioperative death. Seven potential biasing variables (age, ASA classification, emergency surgery, prematurity, preoperative sepsis, preoperative inotropic drug use, and risk adjustment for congenital heart surgery) indicated by DAG (Fig. 3B) were included as the minimally sufficient adjustment set with prolonged fasting variables (Table 4). There was no evidence of effect modification between prolonged fasting and any other variables in the time-to-death model. After adjusting for preoperative condition and type and risk of surgery, preoperative very prolonged fasting (fasting time >865 min), but not prolonged fasting (fasting time >555 min), was significantly associated with time-to-death after surgery (HR [95% CI]: 2.18 [1.22, 3.92]). Fig. 4 compares the cumulative hazard of time-to-death between very prolonged fasting, prolonged fasting, and normal fasting (p = 0.004). The 30-day survival rate (95% CI) of very prolonged, prolonged fasting, and normal fasting were 0.67 (0.55 to 0.81), 0.79 (0.72 to 0.87), and 0.85 (0.79 to 0.91), 0.79 (0.72 to 0.87), and 0.85 (0.79 to 0.91), respectively.
Figure 3: Hypothesized causal relationship between prolonged fasting and perioperative death. Before adjusting for the minimally sufficient adjusted set (A) and after adjusting for the minimally sufficient adjusted set (B) using directed acyclic graph. ASA, American Society of Anesthesiologists; BW, body weight; BS, blood sugar; CPB, cardiopulmonary bypass; IV, intravenous; NPO, nothing by month; periop, perioperative; preop, preoperative; RACHS-1, risk adjustment for congenital heart surgery; Sx, surgery
Figure 4: The cumulative hazard Cox regression model of time-to-death between prolonged fasting and normal fasting. NPO, nothing by month
Since there was no definitive definition of prolonged fasting that might be associated with perioperative death among children after they undergo cardiothoracic surgery, we examined the two best cut-off points of fasting time including fasting time >9.25 h (prolonged fasting) and >14.4 h (very prolonged fasting), which had the highest and second highest ROC curves of perioperative death, as the main exposure variables in this study. Consequently, the incidence of prolonged fasting from solids prior to pediatric cardiothoracic surgery at our hospital between July 2014 and October 2020 was 53.5% (215/402). Our average prolonged fasting duration of 10 h for solids among children was similar to that reported in another study [19], which revealed a mean preoperative fasting duration in children aged <15 years (11.3 h for solids in non-cardiac surgery). We found that a cut-off fasting time of more than 14.4 h showed a two-fold increase in the hazard rate of time-to-death event after cardiothoracic surgery, after adjusting for preoperative condition and anesthesia-related and surgery-related factors suggested by DAG (p = 0.036).
4.1 Preoperative Prolonged Fasting and Perioperative Hyper/Hypoglycemia
From our results (Fig. 2), regardless of the fasting condition, the intraoperative and postoperative blood sugar levels were significantly higher than the preoperative blood sugar level. Higher blood sugar was significantly associated with perioperative death in the univariate Cox analysis (Table 3, p = 0.042). Perioperative hyperglycemia could arise due to the increase in metabolic stress and inflammatory markers during cardiothoracic surgery [6–9]. Zeng et al. [20] reported that a turning point of blood sugar >8.1 mmol/L (144 mg%) during or after cardiopulmonary bypass increased the risk of severe systemic inflammatory response syndrome after pediatric cardiac surgery. According to Fig. 2, most children had a maximum blood sugar level of >144 mg% during and after cardiothoracic surgery, and some children also experienced hypoglycemia events (<50 mg%), which was most frequent in the very prolonged fasting group (13%). Johnston et al. [21] reported that patients aged >18 years who experienced both postoperative hypo- and hyperglycemic events were at an 8-fold increased risk of death, whereas patients who experienced only postoperative hypoglycemia were at a 5-fold increased risk of death after cardiac surgery. We found that the proportion of children in the very prolonged fasting groups experiencing both postoperative hypo- and hyperglycemic events was almost 3.6-fold higher than that in the normal fasting group (7.5% vs. 2.1%), which could increase morbidity and mortality after cardiothoracic surgery in our study.
Thomas [22] reported that preoperative prolonged fasting of 8–10 h in children aged <4 years who underwent eye surgery increased the risk of postoperative hypoglycemia. Polito et al. [23] reported that children with blood sugar <75 mg% were at an increased risk of composite morbidity (nosocomial infection, cardiovascular failure, and hepatorenal failure) and an increase in mortality of 2.6-fold after complex cardiac surgery. Our criteria of preoperative fasting of >14.4 h showed a significantly lower intraoperative maximum blood sugar level in the normal fasting group when compared with the maximum blood sugar level in the preoperative period (176 vs. 153 mg%, Fig. 2). A higher incidence of postoperative hypoglycemia (13.4%) was observed when the fasting time was >14.4 h than that when the fasting time was <14.4 h (4.1%) (Table 2). We observed that children in the very prolonged fasting group were the younger (1.2 months) than those in the prolonged fasting (1.9 months) and normal fasting (2.9 months) groups. Moreover, this group had the highest proportion of premature children (25%). We also found that the body weight of the patients in the very prolonged fasting group at the time of surgery was significantly lower than that of the patients in the normal fasting group (3.4 vs. 3.7 kg). Premature infants with low glycogen and fat stores and restricted ability to produce sugar via the gluconeogenesis pathway could easily develop hypoglycemia [24]. According to our findings, a longer fasting duration and a higher incidence of postoperative hypoglycemia occurred despite the preoperative administration of intravenous fluid containing dextrose and surgical stress response, supporting our hypothesis that the proposed mechanism of very prolonged fasting leading to death may be related to metabolic derangement and postoperative infection/sepsis, especially among premature infants with low weight.
4.2 Preoperative Prolonged Fasting and Time-to-Death Event
Postoperative infection, including pneumonia and sepsis, was the second most common cause of perioperative death (23%). Its incidence was non-significantly higher in the prolonged and very prolonged fasting groups (28%) than that in the normal fasting group (12%). Our postoperative outcome regarding the incidence of postoperative bacteremia, which showed a significantly higher proportion of bacteremia in the very prolonged fasting group (42%) than that in the prolonged fasting group (25%), supported evidence that postoperative sepsis-related death might be the consequence of very prolonged fasting (>14.4 h). Prolonged fasting, especially in low-birth-weight infants, could promote hypercatabolism [25] and an inflammatory response (cytokines) [8], leading to insulin resistance [26] and an increased risk of infection [27]. Moreover, other studies support our finding that longer preoperative fasting can result in decreased human leukocyte antigen–DR expression on monocytes after surgery, which may promote postoperative infection [28,29]. We also found that the very prolonged fasting group had a higher proportion of reoperations than that in the prolonged fasting group (13% vs. 4%, p < 0.05). Prolonged fasting in critically ill patients is associated with a longer duration of mechanical ventilation, a higher proportion of reoperation, and postoperative myocardial injury [30]. Insulin resistance and perioperative infection following prolonged fasting can also lead to prolonged hospital stay [31,32]. However, we found that the duration of mechanical ventilation and length of hospital stay were not different among the fasting group. Postoperative sepsis, especially pneumonia, is a serious non-cardiac complication after cardiac surgery, as it increases mortality, morbidity, and cost [33].
According to Fig. 3, the cumulative hazard of time-to-death in the very prolonged fasting group increases two-fold compared with that in the normal fasting group after adjusting with the minimally sufficient adjusted set (p = 0.036). The 30-day survival rate (95% CI) of the very prolonged fasting group was 0.67 (0.55 to 0.81), whereas it was 0.85 (0.79 to 0.91) in the normal fasting group. We observed that no children in the very prolonged fasting group survived 3 months after the surgery. Therefore, very prolonged fasting associated with hypo- and hyperglycemic events, metabolic derangement, and composite morbidity, including nosocomial infection/sepsis, could aggravate higher mortality which may require a longer period of postoperative care until death after cardiothoracic surgery; in contrast, the children in the other fasting groups continued to survive after 5 months.
4.3 Overall Mortality Compared to Other Studies
The mortality rate of cardiothoracic surgery between July 2014 and October 2020 in our study was 21% which was high compared with that of other studies [34,35]. We included children aged ≤3 years old in the study as this age group with cyanotic heart disease was associated with a high mortality rate (89%) in a previous study [34]. More than 85% of the patients who did not survive in our study had cyanotic heart disease with a high RACHS-1 category, and 60% had high morbidity and mortality (ASA classification 4–5). Berger et al. [35] reported that higher postoperative morbidity was associated with a higher RACHS category (from 2% to 13%). Moreover, 50% of the patients who did not survive in our study had low weight (≤3 kg) at the time of surgery, Chittithavorn et al. [36] reported a mortality rate of 22% among children weighing <3 kg who received a modified Blalock–Taussig shunt, which supports our finding. In addition, around 54% of the children fasted >9.25 h, and 17% fasted >14.4 h, which may have resulted in death after cardiothoracic surgery among these high-risk children.
4.4 Implication for Clinical Practice
Surgery schedules or operative time delays should be minimized to avoid serious harmful events such as perioperative death, especially in vulnerable children undergoing cardiothoracic surgery. However, according to our findings, if the operative time cannot be reduced due to delayed schedules or PICU availability, the fasting time should be limited to not more than 14 h. Although a fasting duration of more than 9.25 h did not increase the risk of time-to-death events in our study, the longer fasting time associated with hypo- and hyperglycemic events, metabolic derangement, and postoperative infection remains a major concern and should be reduced. Therefore, morning surgery schedules should be restricted, as should PICU preparedness, in high-risk children scheduled for cardiothoracic surgery.
The strengths of this study are as follows. First, we used a time-dependent Cox regression analysis to examine the effect of time on death after cardiac surgery. Second, we used multivariate analysis with DAG to adjust for the effects of confounders. DAG showed a clearer cause-effect relationship among the main exposure and outcome (direct effect), mediators (indirect effect), and confounders. Third, this study included only children aged 0–3 years, which promoted the homogeneity of the population. However, this study has some limitations. First, the retrospective design may have resulted in information bias, such as preoperative blood sugar levels, which may be susceptible to the secondary outcomes of postoperative hyper- or hypoglycemia. Second, we did not perform a multivariate analysis of the other outcomes regarding the morbidity of prolonged fasting, and univariate analysis of postoperative outcomes was performed to determine the possible outcomes related to perioperative death. In addition, generalizability could be limited as the study was confined to a single hospital.
Very prolonged preoperative fasting (>14.4 h) was associated with a two-fold increase in the hazard rate of time-to-death in children who underwent cardiothoracic surgery. A preoperative fasting duration of >14.4 h could lead to hypo- and hyperglycemic events and bacteremia-related death in high-risk children.
Authorship: All the authors contributed to the preparation of this manuscript. Each author participated in the work to defend its contents. LR designed the study, collected the data, and wrote the draft of the manuscript. MO coordinated the study, participated in the study design, performed the statistical analysis, and revised the draft manuscript. JT participated in the study design and coordinated drafting of the manuscript. TC participated in this study. All the authors have read and approved the final version of the manuscript.
Availability of Data and Materials: De-identified participant data (including data dictionaries) are shared in the appendix file.
Acknowledgement: We would like to thank Dr. Alan Frederick Geater for his advice on statistical analysis and editage and Assistant Professor Edward McNeil for editing the manuscript and for their valuable suggestions.
Funding Statement: This study was funded by the Faculty of Medicine, Prince of Songkla University, Hat Yai, Songkhla, Thailand. L.R. received a grant amount of ฿0 in May 2020 (Grant No. 62-463-8-3; http://medinfo.psu.ac.th/).
Conflicts of Interest: The authors declare that they have no conflicts of interest to report regarding the present study.
References
1. Anonymous (2017). Practice guidelines for preoperative fasting and the use of pharmacologic agents to reduce the risk of pulmonary aspiration: Application to healthy patients undergoing elective procedures an updated report by the American Society of Anesthesiologists task force on preoperative fasting and the use of pharmacologic agents to reduce the risk of pulmonary aspiration. Anesthesiology, 126(3), 376–393. [Google Scholar]
2. Maclean, A. R., Renwick, C. (1993). Audit of pre-operative starvation. Anaesthesia, 48(2), 164–166. [Google Scholar]
3. Buller, Y., Sims, C. (2016). Prolonged fasting of children before anaesthesia is common in private practice. Anaesthesia and Intensive Care, 44(1), 107–110. [Google Scholar]
4. Engelhardt, T., Wilson, G., Horne, L., Weiss, M., Schmitz, A. (2011). Are you hungry? Are you thirsty?--Fasting times in elective outpatient pediatric patients. Paediatric Anaesthesia, 21(9), 964–968. [Google Scholar]
5. Andersson, H., Zarén, B., Frykholm, P. (2015). Low incidence of pulmonary aspiration in children allowed intake of clear fluids until called to the operating suite. Paediatric Anaesthesia, 25(8), 770–777. [Google Scholar]
6. Thorell, A., Nygren, J., Ljungqvist, O. (1999). Insulin resistance: A marker of surgical stress. Current Opinion in Clinical Nutrition and Metabolic Care, 2(1), 69–78. [Google Scholar]
7. Faria, M. S., de Aguilar-Nascimento, J. E., Pimenta, O. S., Alvarenga Jr, L. C., Dock-Nascimento, D. B. et al. (2009). Preoperative fasting of 2 h minimizes insulin resistance and organic response to trauma after video-cholecystectomy: A randomized, controlled, clinical trial. World Journal of Surgery, 33(6), 1158–1164. [Google Scholar]
8. Pimenta, G. P., de Aguilar-Nascimento, J. E. (2014). Prolonged preoperative fasting in elective surgical patients: Why should we reduce it? Nutrition in Clinical Practice, 29(1), 22–28. [Google Scholar]
9. Xu, D., Zhu, X., Xu, Y., Zhang, L. (2017). Shortened preoperative fasting for prevention of complications associated with laparoscopic cholecystectomy: A meta-analysis. The Journal of International Medical Research, 45(1), 22–37. [Google Scholar]
10. Unal, I. (2017). Defining an optimal cut-point value in ROC analysis: An alternative approach. Computational and Mathematical Methods in Medicine, 2017, 3762651. [Google Scholar]
11. El-Sherbini, S. A., Marzouk, H., El-Sayed, R., Hosam-ElDin, S. (2018). Etiology of hyperglycemia in critically ill children and the impact of organ dysfunction. Revista Brasileira de Terapia Intensiva, 30(3), 286–293. DOI 10.5935/0103-507X.20180051. [Google Scholar] [CrossRef]
12. Thornton, P. S., Stanley, C. A., de Leon, D. D., Harris, D., Haymond, M. W. et al. (2015). Recommendations from the pediatric endocrine society for evaluation and management of persistent hypoglycemia in neonates, infants, and children. The Journal of Pediatrics, 167(2), 238–245. DOI 10.1016/j.jpeds.2015.03.057. [Google Scholar] [CrossRef]
13. Jain, A., Aggarwal, R., Jeevasanker, M., Agarwal, R., Deorari, A. K. et al. (2008). Hypoglycemia in the newborn. Indian Journal of Pediatrics, 75(1), 63–67. DOI 10.1007/s12098-008-0009-6. [Google Scholar] [CrossRef]
14. Jenkins, K. J., Gauvreau, K., Newburger, J. W., Spray, T. L., Moller, J. H. et al. (2002). Consensus-based method for risk adjustment for surgery for congenital heart disease. The Journal of Thoracic and Cardiovascular Surgery, 123(1), 110–118. DOI 10.1067/mtc.2002.119064. [Google Scholar] [CrossRef]
15. Dupont, W. D., Plummer Jr, W. D. (1990). Power and sample size calculations: A review and computer program. Controlled Clinical Trials, 11(2), 116–128. DOI 10.1016/0197-2456(90)90005-M. [Google Scholar] [CrossRef]
16. Schoenfeld, D. A., Richter, J. R. (1982). Nomograms for calculating the number of patients needed for a clinical trial with survival as an endpoint. Biometrics, 38(1), 163–170. DOI 10.2307/2530299. [Google Scholar] [CrossRef]
17. Textor, J., van der Zander, B., Gilthorpe, M. S., Liskiewicz, M., Ellison, G. T. (2016). Robust causal inference using directed acyclic graphs: The R package ‘dagitty’. International Journal of Epidemiology, 45(6), 1887–1894. [Google Scholar]
18. Oofuvong, M., Geater, A. F., Chongsuvivatwong, V., Chanchayanon, T., Sriyanaluk, B. et al. (2021). Does perioperative respiratory event increase length of hospital stay and hospital cost in pediatric ambulatory surgery? PLoS One, 16(5), e0251433. DOI 10.1371/journal.pone.0251433. [Google Scholar] [CrossRef]
19. Arun, B. G., Korula, G. (2013). Preoperative fasting in children: An audit and its implications in a tertiary care hospital. Journal of Anaesthesiology, Clinical Pharmacology, 29(1), 88–91. DOI 10.4103/0970-9185.105810. [Google Scholar] [CrossRef]
20. Zeng, Z. H., Yu, X. Y., Liu, X. C., Liu, Z. G. (2022). Effect of CPB sugar levels on inflammatory response after pediatric cardiac surgery. BMC Cardiovascular Disorders, 22(1), 222. DOI 10.1186/s12872-022-02667-w. [Google Scholar] [CrossRef]
21. Johnston, L. E., Kirby, J. L., Downs, E. A., LaPar, D. J., Ghanta, R. K. et al. (2017). Postoperative hypoglycemia is associated with worse outcomes after cardiac operations. The Annals of Thoracic Surgery, 103(2), 526–532. DOI 10.1016/j.athoracsur.2016.05.121. [Google Scholar] [CrossRef]
22. Thomas, D. K. (1974). Hypoglycaemia in children before operation: Its incidence and prevention. British Journal of Anaesthesia, 46(1), 66–68. DOI 10.1093/bja/46.1.66. [Google Scholar] [CrossRef]
23. Polito, A., Thiagarajan, R. R., Laussen, P. C., Gauvreau, K., Agus, M. S. et al. (2008). Association between intraoperative and early postoperative sugar levels and adverse outcomes after complex congenital heart surgery. Circulation, 118(22), 2235–2242. DOI 10.1161/CIRCULATIONAHA.108.804286. [Google Scholar] [CrossRef]
24. Sharma, A., Davis, A., Shekhawat, P. S. (2017). Hypoglycemia in the preterm neonate: Etiopathogenesis, diagnosis, management and long-term outcomes. Translational Pediatrics, 6(4), 335–348. DOI 10.21037/tp. [Google Scholar] [CrossRef]
25. Dennhardt, N., Beck, C., Huber, D., Sander, B., Boehne, M. et al. (2016). Optimized preoperative fasting times decrease ketone body concentration and stabilize mean arterial blood pressure during induction of anesthesia in children younger than 36 months: A prospective observational cohort study. Paediatric Anaesthesia, 26(8), 838–843. DOI 10.1111/pan.12943. [Google Scholar] [CrossRef]
26. Sato, H., Carvalho, G., Sato, T., Lattermann, R., Matsukawa, T. et al. (2010). The association of preoperative glycemic control, intraoperative insulin sensitivity, and outcomes after cardiac surgery. The Journal of Clinical Endocrinology and Metabolism, 95(9), 4338–4344. DOI 10.1210/jc.2010-0135. [Google Scholar] [CrossRef]
27. Aguilar-Nascimento, J. E., Marra, J. G., Slhessarenko, N., Fontes, C. J. (2007). Efficacy of national nosocomial infection surveillance score, acute-phase proteins, and interleukin-6 for predicting postoperative infections following major gastrointestinal surgery. Sao Paulo Medical Journal, 125(1), 34–41. DOI 10.1590/S1516-31802007000100007. [Google Scholar] [CrossRef]
28. Melis, G. C., van Leeuwen, P. A., von Blomberg-van der Flier, B. M., Goedhart-Hiddinga, A. C., Uitdehaag, B. M. et al. (2006). A carbohydrate-rich beverage prior to surgery prevents surgery-induced immunodepression: A randomized, controlled, clinical trial. Journal of Parenteral and Enteral Nutrition, 30(1), 21–26. DOI 10.1177/014860710603000121. [Google Scholar] [CrossRef]
29. Hershman, M. J., Cheadle, W. G., Wellhausen, S. R., Davidson, P. F., Polk Jr, H. C. (1990). Monocyte HLA-DR antigen expression characterizes clinical outcome in the trauma patient. The British Journal of Surgery, 77(2), 204–207. DOI 10.1002/bjs.1800770225. [Google Scholar] [CrossRef]
30. Zhou, G., Zhu, F., An, Y., Qin, L., Lv, J. et al. (2020). Prolonged preoperative fasting and prognosis in critically ill gastrointestinal surgery patients. Asia Pacific Journal of Clinical Nutrition, 29(1), 41–47. [Google Scholar]
31. Thieme, R. D., Cutchma, G., Chieferdecker, M. E., Campos, A. C. (2013). Nutritional risk index is predictor of postoperative complications in operations of digestive system or abdominal wall? ABCD. Arquivos Brasileiros de Cirurgia Digestiva, 26(4), 286–292. DOI 10.1590/S0102-67202013000400007. [Google Scholar] [CrossRef]
32. Francisco, S. C., Batista, S. T., Pena, G. D. (2015). Fasting in elective surgical patients: Comparison among the time prescribed, performed and recommended on perioperative care protocols. Arquivos Brasileiros de Cirurgia Digestive, 28(4), 250–254. DOI 10.1590/s0102-6720201500040008. [Google Scholar] [CrossRef]
33. Bicer, Y., Simsek, S., Yapici, N., Aydin, O., Sogut, F. et al. (2005). Risk factor analysis of pneumonias developing after open heart surgery. Critical Care, 9(Suppl 1), 1–2. DOI 10.1186/cc3064. [Google Scholar] [CrossRef]
34. Zheng, G., Wu, J., Chen, P., Hu, Y., Zhang, H. et al. (2021). Characteristics of in-hospital mortality of congenital heart disease (CHD) after surgical treatment in children from 2005 to 2017: A single-center experience. BMC Pediatrics, 21(1), 521. DOI 10.1186/s12887-021-02935-2. [Google Scholar] [CrossRef]
35. Berger, J. T., Holubkov, R., Reeder, R., Wessel, D. L., Meert, K. et al. (2017). Morbidity and mortality prediction in pediatric heart surgery: Physiological profiles and surgical complexity. The Journal of Thoracic and Cardiovascular Surgery, 154(2), 620–628.e6. DOI 10.1016/j.jtcvs.2017.01.050. [Google Scholar] [CrossRef]
36. Chittithavorn, V., Duangpakdee, P., Rergkliang, C., Pruekprasert, N. (2017). Risk factors for in-hospital shunt thrombosis and mortality in patients weighing less than 3 kg with functionally univentricular heart undergoing a modified blalock-taussig shunt. Interactive Cardiovascular and Thoracic Surgery, 25(3), 407–413. DOI 10.1093/icvts/ivx147. [Google Scholar] [CrossRef]
Appendix A: The receiver operating characteristic curve of fasting time for death
Cite This Article
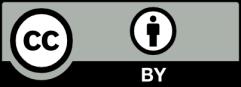
This work is licensed under a Creative Commons Attribution 4.0 International License , which permits unrestricted use, distribution, and reproduction in any medium, provided the original work is properly cited.