Open Access
REVIEW
Associations between Bodyweight and Clinical Outcome in Patients Post-Fontan Procedure: A Systematic Review
1 The University of Sydney, Sydney, Australia
2 The University of Melbourne, Melbourne, Australia
3 The Royal Children’s Hospital, Melbourne, Australia
4 The Royal Prince Alfred Hospital, Sydney, Australia
5 The Children’s National Hospital, Washington, USA
* Corresponding Author: Julian Ayer. Email:
Congenital Heart Disease 2022, 17(6), 617-639. https://doi.org/10.32604/chd.2022.024775
Received 07 June 2022; Accepted 26 July 2022; Issue published 11 October 2022
Abstract
Background: Patients born with a single ventricle circulation commonly experience growth failure in early life, which is associated with adverse outcomes in infancy. However, associations between bodyweight or weight trajectory and clinical outcome post-Fontan procedure are yet to be determined. Methods: On the 1st of July 2021, a systematic review was performed in MEDLINE, EMBASE, the Cochrane Library, and Scopus of studies of patients with clinical outcome data post-Fontan procedure and association with bodyweight. Quality of studies was evaluated by Newcastle–Ottawa scale for cohort studies and Joanna Briggs Institute tool for cross-sectional studies. Results: Of 527 studies that underwent title and abstract screening, 15 were selected for final review. An increased risk of adverse post-Fontan outcomes was found for low weight patients, consistent with findings in infants. Whilst there is some evidence to suggest increased mortality in overweight adult patients, studies are conflicting as to whether overweight status is associated with increased heart failure. Increased BMI is associated with diminished exercise capacity and deceased physiological functioning. Negative weight trajectory is associated with adverse outcomes in the peri-Fontan period, whereas a positive weight trajectory is associated with increased Fontan failure in adulthood. Abnormal BMI (high or low) is associated with increased heart failure and poorer performance in quality-of-life scores. Conclusions: Bodyweight is a modifiable risk factor for poor clinical outcome in patients with a single ventricle circulation. Recognizing associations between bodyweight and Fontan pathophysiology may help to define patient-centered exercise and diet interventions that minimize patient morbidity and mortality.Keywords
Patients born with single ventricle (SV) cardiac defects may survive into adulthood after undergoing a series of palliative surgical procedures in early life [1–3]. This process often begins with a procedure in early infancy that stabilizes pulmonary blood flow and normalizes output from the SV (for example, pulmonary artery banding, systemic to pulmonary artery shunt, or a Norwood procedure). Subsequent procedures during childhood include superior cavopulmonary connection and Fontan completion, which result in all systemic venous blood being passively diverted to the pulmonary arteries [3–5]. Although this staged palliation has dramatically improved survival, patients are still subject to significant early and late morbidity and mortality [1,4,6].
Infants born with SV defects are at risk of growth failure in early life [7–13]. Possible factors contributing to growth failure include increased baseline energy demands, reduced nutritional input, malabsorption, and sequelae of prematurity or genetic syndromes [8,11–17]. Although early life growth failure has been consistently linked with increased morbidity and mortality during infancy [18–23], its relationship with long-term Fontan outcomes remains unclear.
By contrast, a rising prevalence of obesity has been observed in children and adults with complex congenital heart disease (CHD) [24–30]. Factors contributing to this may include disease severity-based activity restriction, as well as overcorrection of early life growth failure through standardized feeding interventions [31–33]. In CHD, as in the general population, obesity is associated with modifiable risk factors for coronary artery disease including hyperlipidemia and hypertension [34–37]. Features of the SV circulation, such as reduced lung compliance, impaired diastolic function, and increased pulmonary vascular resistance and ventricular mass, may compound any adverse consequences of obesity on cardiovascular function [38–40]. However, some studies in patients with CHD have demonstrated lower incidence of morbidity in those with high body mass index (BMI) compared to those with low or normal BMI [35,41,42]. It is as yet unclear whether this association is also present in patients with SV defects. Due to the potential risks of abnormal BMI on Fontan physiology, determination of ideal weight patterns for SV patients is important for optimizing their care. In this way, clinicians can instigate appropriate weight monitoring and interventions to minimize morbidity and mortality.
Therefore, we undertook a systematic review of existing literature to better understand the relationship between relative bodyweight and clinical outcomes in patients with an SV circulation post-Fontan procedure.
The protocol was registered with the international prospective register of systematic reviews (PROSPERO), in accordance with PRISMA-P guidelines (PROSPERO CRD42021272315) [43].
We included studies on human participants with all cardiac defects defined as ‘single ventricle’ that are managed surgically with the Fontan procedure. These conditions included, but were not limited to, tricuspid atresia, double outlet right ventricle, double inlet left ventricle, pulmonary atresia with VSD, congenitally corrected transposition of the great arteries (ccTGA) (VA discordance and AV discordance), Ebstein’s anomaly, atrioventricular septal defect (unbalanced AVSD or common AV valve), pulmonary atresia with intact ventricular septum, and hypoplastic left heart syndrome. Studies on patients with congenital heart disease of non-single ventricle type were excluded.
Only studies with outcome data post-Fontan completion were included. No age limitation was imposed.
We included studies that compared outcomes based on weight relative to height (BMI, weight-for-length), weight relative to age (weight-for-age), and weight change over time (change in either body mass, weight-for-age, weight-for-length, or BMI). Studies were excluded if they only addressed alternative measures of adiposity (for example body composition, adipose tissue mass, triglyceride levels) without the relative weight measures, as detailed above.
Studies that included patient weight prior to Fontan procedure were included if they examined clinical outcome data post-Fontan completion.
Studies were excluded if the outcomes examined were a measure or surrogate measure of body composition (for example, percentage body fat, waist circumference, blood lipid levels). All other outcome measures were examined, including, but not limited to, mortality, heart transplant, Fontan takedown, and physiological parameters such as maximum aerobic capacity, echocardiographic findings, and measures of morbidity such as NYHA class, infection, and length of hospital stay.
No settings were excluded. No date restriction was imposed.
Only studies published in English were included.
We searched MEDLINE (OVID interface), EMBASE (OVID interface), the Cochrane Library (Wiley Interface), and Scopus. Literature search strategies were developed using medical subject headings (MeSH) and terms. The specific search strategies were developed in consultation with a Health Sciences librarian experienced in the conduct of systematic reviews who was not otherwise associated with either the project or the project team. Search strategies for MEDLINE and EMBASE are included in Appendixes A and B. These were adapted for the syntax and subject headings of other bibliographic databases (see Appendixes C and D).
To ensure literature saturation, we also reviewed reference lists of included studies.
Screening of titles and abstracts was performed independently by two reviewers (EP and TW) in duplicate for eligibility, as per the inclusion criteria outlined above. Full text reports for the identified studies were assessed for eligibility against the inclusion and exclusion criteria by two reviewers independently, any disputes were resolved by consensus with a third reviewer (JA), and authors were contacted for missing data whenever possible.
Literature search results were uploaded to Covidence (Veritas Health Innovation), an Internet-based software program that aids reviewers in collaborating during study selection [44]. Citation titles and abstracts were uploaded to Covidence.
Data extraction was performed by the primary reviewer. Data collected included study features (setting, study type, funding sources, information for risk of bias) and data features (patient demographic information and baseline characteristics, methodology, weight comparators, all reported clinical outcomes, and suggested mechanism of association between weight and outcome).
Data from the included publications were organized by bodyweight category of focus, and tabulated. Pooling of results through meta-analysis was not performed due to the limited study number and high inter-study heterogeneity [45]. Thus, a narrative synthesis of results was performed.
2.7 Critical Appraisal Assessment
The quality of cohort studies was assessed by the Newcastle–Ottawa Quality Assessment Scale (NOS) for non-randomized cohort studies. This scale is applicable to the type of study relevant to this review and is supported by use in previous systematic reviews [46–48]. In addition, for cross-sectional studies, the quality was assessed by the Joanna Briggs Institute (JBI) Critical Appraisal tool for analytical cross-sectional studies [49].
Studies were not excluded from the review on the grounds of critical appraisal assessment.
The online database search on the 1st of July 2021 identified 1,029 references, 496 of which were removed as duplicates. No additional relevant publications were found in searching the reference lists of included studies. The remaining 533 publications underwent title and abstract screening to select relevant peer-reviewed articles according to the criteria above (Fig. 1). In total, 42 publications were sought for full-text review. Of these, 14 were only available as an abstract, or could not be retrieved as a full text. Of 28 full-text reports assessed for eligibility, 6 only examined BMI as a correlate of other measures of adiposity/body composition, 6 publications had patient populations that did not meet inclusion criteria and 1 did not report an outcome (Appendixes A–D). Thus, a total of 15 studies were included in the final review, with 8 cross-sectional studies (Table 1) and 7 cohort studies (Table 2).
Figure 1: PRISMA flowchart for inclusion of articles in systematic review
All studies were published from the year 2000 onwards, with 10 of the 15 total studies performed in the last five years. When examining association with clinical outcomes, studies primarily examined high weight class as a binary exposure. In line with the relative paucity of data, we elected not to undertake a meta-analysis [45].
The quality of the 7 cohort studies included was evaluated using the Newcastle–Ottawa scale. All studies achieved a score of 6/9 or above (Table S1). The 8 cross-sectional studies were evaluated using the JBI critical appraisal tool, with all studies achieving a score of 6/8 or above (Table S2).
3.3 BMI-Associated Patient Factors
The studies in this review identified several factors positively associated with BMI [21,27,28,39,50–60]. As is supported in the broader population, increasing age in the adolescent and young adult populations was generally strongly associated with increasing BMI, and age was thus often adjusted for in the analyses. BMI z-scores (BMIz) were also found to increase with age for Fontan patients into adolescence and adulthood, reflecting the trend towards correction of poor growth over time [56–58,60]. Other factors associated with increased BMI included higher oxygen saturations [61], younger age at Fontan procedure [57], and left ventricular dominance [60].
In contrast to studies of patients with a SV circulation in infancy, studies post-Fontan procedure largely did not address clinical outcome in lower weight classes (Table 3) [7,28,39,51,52]. The studies that chose to address low weight categories demonstrated an increased morbidity and mortality in low-weight patients. A large, multicenter study conducted by Wallace et al. [28] found that, in 2747 patients, low weight-for-age z-score (WAZ ≤ –2) at the time of Fontan completion was a significant predictor of in-hospital mortality (P = 0.001) and Fontan failure—defined as combined in-hospital mortality and Fontan takedown/revision (P < 0.001). In addition, in a study of 232 adult patients, Callegari et al. [51] found an association between low BMI and reduced FEV1 on multiple regression analysis with adjustment for the presence of chronic respiratory conditions (effect size not provided, P = 0.01). The clinical implications of this finding were potentially significant given the association found between FEV1 and VO2max (r = 0.43, P < 0.0001), physical functioning independent of exercise capacity (physical functioning (r = 0.25, P = 0.008), and bodily pain (r = 0.22, P = 0.02) [51].
Protein-losing enteropathy (PLE) has been associated with low weight class in infants with a SV circulation prior to Fontan completion [17,59,61,62]. However, there was insufficient evidence to support an association in this review of patients post-Fontan completion. Of the three studies that addressed PLE, none found a significant association between PLE and weight class [27,39,52]. In the study by Mancilla et al. [52] (n = 210, mean age 11.1 years), those with and without PLE did not have a statistically significant difference in BMIz (–0.45 vs. –0.1, P = 0.58).
3.5.1 BMI, Mortality, and Heart Transplant
Studies revealed limited evidence of an association between obesity and transplant-free survival in post-Fontan patients (Table 4) [39,60,61]. In the study of Martinez et al. (2016) (n = 79, mean age 29.52 ± 8.85 years) [61], there was an age-adjusted hazard ratio of 3.206 (95% CI, 1.096–9.379) per 1 kg/m2 unit increase in BMI, where outcome was defined as mortality, heart transplant, or hospice care. However, Lambert et al. (n = 546, mean age 12 ± 3.4 years at first visit) did not find a significant difference in BMIz between survivors vs. patients who experienced mortality over the 10-year follow-up [60]. In addition, in the study by Freud et al. [39], (n = 139, mean age 23.2 years), transplant-free survival was not associated with overweight status (log-rank P = 0.20). The differences between these studies may arise from the differing definitions of exposure to increasing BMI—continuous in the former and categorical in the latter.
The literature is divided regarding the impact of higher BMI on heart failure in adults with a SV circulation. In a study of 129 adults (>18 years, mean adult age not specified) with a Fontan circulation, Chung et al. [57], found that overweight/obese (BMI ≥ 25) patients had lower rates of heart failure than normal or underweight patients (8% vs. 19%; P = 0.03) [57]. They hypothesized that this finding might relate to increased weight as a marker of positive nutritional balance, a sign of improved cardiac health, or less severe disease. Freud et al. [39] found no association between BMI class and heart failure at the time of Fontan conversion (no data provided).
By contrast, Martinez et al. [61] found that higher BMI was associated with a higher prevalence of symptomatic heart failure. In 79 adult patients post-Fontan, they found that symptomatic (NYHA class II–IV) patients had significantly higher BMI than asymptomatic patients (23.61 ± 4.77 kg/m2 vs. 28.11 ± 7.98 kg/m2, P = 0.004). Furthermore, patients with a higher diuretic requirement had a higher BMI than those without (32.15 ± 9.12 kg/m2 vs. 30.91 ± 8.67 kg/m2, P = 0.004).
In examining potential factors explaining these divergent findings, the diagnosis of heart failure was much less prevalent in the cohort reported by Chung et al. (18%) [57] compared with the older Fontan cohort in Martinez et al. (50%) [61]. The higher prevalence of heart failure in the later study may imply reverse causality in older Fontan cohorts with more severe disease; namely, advanced heart failure being associated with weight gain.
3.5.3 BMI and Exercise Capacity
The literature consistently demonstrates reduced exercise capacity in overweight and obese Fontan patients compared to those of normal weight. In a study of 544 adolescent Fontan patients, Cohen et al. [27] found a significant difference in percentage of predicted peak aerobic capacity (% predicted VO2max) in overweight/obese vs. normal weight and underweight groups (BMIz ≤ –1.5: 68.2 ± 12.6; −1.5 < BMIz < 1.5: 67.9 ± 14.4; BMIz ≥ 1.5: 51.9 ± 15.3, P = 0.002). Similarly, Weinreb et al. [55], in a study of 265 Fontan patients (median age 12.8 (9.5–16.4) years), found that overweight/obese status was significantly associated with lower VO2max compared to normal weight status (–3.12; P = 0.002, age-adjusted). Further, in a study by Goldberg et al. [59] (n = 373, mean age 21.1 ± 3.3 years), a disproportionate number of obese subjects were in the lowest performing tertile for VO2max and VO2 at anaerobic threshold.
3.5.4 BMI and Other Physiological Parameters
Certain aspects of the SV circulation may make it specifically vulnerable to the pathophysiological impacts of obesity, such as the high pulmonary artery pressure and volume overload [27,39,57,63–65]. Chung et al. [57] studied changes in increased afterload, or systolic blood pressure in Fontan patients across the pediatric (n = 129) and adult (n = 395) age groups. In children, they noted significantly raised systolic blood pressure in overweight/obese (BMI >85th percentile) compared with normal weight or underweight patients (effect size not provided; P < 0.01 in 2–5 and 12–19 age groups). In a study of paediatric SV by Cohen et al. [27], systolic blood pressure was higher in those with a BMIz > 1.5 compared to those whose BMIz was <1.5, although this difference was not statistically significant (115.6 ± 12.5 mmHg vs. 102.93 ± 17.8 mmHg; P = 0.06). Cohen et al. [27] also found evidence of ventricular hypertrophy in obese patients, measured as mass-to-volume ratio on echocardiography (P = 0.027).
The implications of higher BMI on Fontan vascular physiology were also explored by Nanayakkara et al. [54] in a study of Fontan patients with obstructive sleep apnea (OSA). The study compared seven symptomatic patients (as defined by Epworth Sleepiness Score > 10) to seven asymptomatic patients regarding prevalence of OSA by Apnea Hypopnea Index (AHI). In this study, BMI was closely associated with AHI (R = 0.7, P = 0.005), with those who were symptomatic (daytime sleepiness) having a BMI closer to the obese range (29.2 ± 6.5 kg/m2) whilst asymptomatic patients had a BMI in the high normal range (24.9 ± 2.6 kg/m2). This has important clinical implications, as Fontan patients may be more susceptible to long-term consequences of OSA including pulmonary hypertension and tachyarrhythmias, although investigation of the physiological impacts of OSA in the SV population is very limited to date [54]. The effects of OSA may be compounded by the inability of some Fontan patients to safely tolerate positive pressure treatment of OSA due to the respiratory dependence on pulmonary blood flow [66,67]. The small participant number in the study by Nanayakkara somewhat limits broader interpretation of its findings, but highlights an important subset of patients, those with OSA, to target for weight loss interventions.
3.6 Weight Trajectory and Outcomes
Comparison of studies examining association between trajectory of weight or BMI and outcomes is difficult because of the underlying heterogeneity in the study populations, particularly in terms of average age and BMI (Table 5). An increasing BMI post-Fontan in a patient who is already overweight could have vastly different implications compared to increasing BMI that brings a patient into a ‘normal’ weight category.
In a study of 65 Fontan patients (age range 2–13 years), Day et al. [58] found that heart transplant and mortality were increased in patients who experienced negative change in WAZ > –1 over 2–13 years post-Fontan completion (P < 0.01). This is in keeping with other research revealing increased morbidity and mortality in poorly growing single ventricle patients in early life [18–21,23,28]. The average WAZ in this population was –1.4, and therefore a relative slowing of weight gain in an already ‘underweight’ population may have stronger associations with poor clinical outcome compared to slower growth in the general SV population.
Lambert et al. [60] studied serial BMIs of 362 post-Fontan patients over a 10-year period (mean age 21 ± 3.5 years at last follow-up). Under 20 years of age, the average BMI of this cohort was not significantly different to CDC norms. In this age group, an increase in BMIz over the 10-year follow-up was associated with decreased maximal exercise capacity (VO2max), though this finding was not statistically significant (B = –2.02, P = 0.27). Contrastingly, for those who were over 20 years of age over the 10-year study period, there was a significantly lower prevalence of overweight and obese patients compared to CDC norms (effect size and P-value were not shown). In this age group, an increase in BMI from the post-Fontan period to 10-year follow-up was also associated with decreased maximal exercise capacity (VO2max) (B = –1.25, P < 0.001). In addition, these authors found no significant difference in change in BMIz over a 10-year follow-up (mean age 12–21 years) between survivors and those who died (P = 0.11). In this analysis, survivors had BMI approaching that of the general population, whilst those who died maintained BMIz below population norms (z-score mean approximately –0.4). This perhaps implies that adverse clinical outcomes have a greater association with underweight status.
Conversely, Byrne et al. [56], in a study of 104 adult patients (mean age not provided), focused on the clinical implications of high weight gain post-Fontan. They found that increasing weight during adulthood was independently associated with a combined endpoint of mortality, heart transplant, PLE, VO2 <50%, and new use of a loop diuretic (HR 1.36, CI 1.07–1.73; P = 0.011).
Menon et al. [53], in a study of 299 adolescent Fontan patients (mean age 13.9 years), defined ‘abnormal BMI’ as either <5th or >95th percentile (CDC Growth Charts [67]). Approximately 16% of their study population was found to have abnormal BMI—as opposed to roughly 10% of the US population (P < 0.0001)—with approximately half of these patients in each extreme of BMI class. These authors found that abnormal BMI was associated with an increased risk of heart failure compared to patients with a normal BMI (OR 10.9 (1.8, 124.3); P = 0.046).
3.8 Correlates with Measures of Quality of Life
In the study of Menon et al. [53], abnormal BMI was associated with lower quality of life scores (Pediatric Quality of Life Inventory) (62.3 ± 17 vs. 72.5 ± 16.6; P < 0.001) and lower parent-reported perceived physical appearance scores (72.2 ± 27.4 vs. 79.8 ± 21.5; P < 0.01). Other studies, however, found no evidence of an association between BMI and quality of life [27,60]. In adolescents, Cohen et al. [27] examined the association between higher BMI and functional health status through the Parent Report form of the Child Health Questionnaire (CHQ) for physical and psychosocial wellbeing. The authors found no difference in CHQ scores between high, normal, and low BMI classes (defined by BMI CDC z-scores ≤–1.5 and >1.5, results not provided). Similarly, Lambert et al. [60] found no significant association between BMIz (age ≤ 20) or BMI (age > 20) and Pediatric Quality of Life Inventory 4.0 (PedsQL) Generic Core Scales or physical function subdomain score (slope = –0.39, –0.32, respectively; P = 0.58 and 0.15).
4.1 Heterogeneity in Definitions of Relative Bodyweight and BMI Class
Comparison of the 15 studies—6 paediatric and 9 adults—was difficult due to heterogeneity of the study populations (particularly mean age and BMI) and outcomes. In addition, there was heterogeneity in the definition of weight classes or relative weight metrics. Many researchers used BMI for examining weight relative to height. However, others used ‘weight-for-age’ or ‘weight-for-height’ z-scores (WAZ and WHZ, respectively) [68–70]. Normative data for BMI also varied across studies with z-score or percentiles generated from Center for Disease Control (CDC), World Health Organization (WHO) or the United States National Center for Health Statistics reference populations.
4.2 Impact of Underweight on Adverse Outcome
A number of studies demonstrate adverse clinical outcomes faced by poorly growing infants born with an SV circulation [27,28,39,52]. In this review, underweight status in SV children was associated with poor outcome post-Fontan procedure [28,51]. However, no statistically significant association was found between underweight and adverse outcomes in adulthood [27,39]. Therefore, the specific risks faced by underweight adult Fontan patients are still unclear.
4.3 Impact of Overweight on Mortality and Heart Failure
The limited literature examining transplant-survival of overweight Fontan patients suggests a possible increased risk of mortality faced by those with a high BMI [61], yet this is contradicted by studies demonstrating no association [39,51]. Evidence is also unclear regarding the association between higher bodyweight class and heart failure. Contrasting to findings of Martinez et al. [61], Chung et al. [57] reported a decreased risk of heart failure in overweight compared to normal weight patients. Potential reasons for this include higher weight status as a sign of positive nutritional balance and less severe disease. Another suggestion is that this effect mirrors the “obesity paradox” in non-congenital heart disease, by which abnormally high bodyweight is a risk factor for development of heart failure yet is protective once a diagnosis of heart failure is established [71–73]. Improved prognosis for patients with a high BMI may be due to a relatively higher metabolic reserve, greater skeletal muscle mass, and beneficial alterations in cytokines or adipokines [72–74].
4.4 Impact of Overweight on Physiological Parameters
The weight of evidence supports an association between overweight/obesity and reduced exercise capacity in Fontan patients. These findings are potentially significant given the evidence of VO2 as a predictor of mortality and heart transplant [61]. There is also evidence of higher systolic blood pressure and ventricular hypertrophy in young overweight Fontan patients [28,56]. The effects of obesity mediated by hypertension on the functional single ventricle require further evaluation. However, the described associations are of potential concern given the high rates of systemic right ventricular dysfunction.
4.5 Completeness and Applicability of Evidence
Our systematic review indicates an association between BMI and a number of clinical outcomes including mortality, exercise capacity, and physiological parameters. In particular, patients at the extremes of BMI—both low and high—experience poor clinical outcome. The ease of performing BMI assessments makes BMI an appealing prognostic tool.
However, BMI may misclassify adiposity in patients with a Fontan circulation. Several studies have observed moderate to severe skeletal muscle mass deficit in Fontan patients [75–79], with a recent study by Tran et al. [75] finding sarcopenia in 39%. This ‘Fontan-associated myopenia’, can mask high adiposity when assessed by BMI, as BMI cannot distinguish between fat and lean mass. Reduced muscle mass is associated with reduced exercise capacity in Fontan patients [75,80,81]. In addition, a higher percentage body fat (%BF) is associated with negative trend in exercise capacity [82]. A recent study found that a 1% increase in %BF was associated with an increased risk of reaching the composite clinical endpoint of death, heart transplantation, NYHA III/IV heart failure, protein-losing enteropathy, and/or plastic bronchitis (HR 1.10, 95% CI 1.01–1.19; P = 0.03) [83]. Associations have also been found between low HDL and increased mortality [84], and higher intra-abdominal visceral fat and increased pulmonary vascular resistance [84].
In examining the association between measures of relative weight and clinical outcomes, a number of potential confounding factors exist. Firstly, both overweight/obesity and the development of adverse outcomes are associated with increasing age. Secondly, low height or linear growth stunting is associated with adverse outcomes and may impact the assessment of relative weight (BMI for example). Thirdly, raw BMI values do not account for sex. Some evidence exists that male single ventricle patients grow better than females in early childhood [30,74]. However, in some studies, Fontan failure appears to occur more often in male patients compared to females [54,62,75].
4.6 Potential Biases in Review
We were unable to contact several authors regarding inclusion and assessment of their data, and other publications were still in process, so data were not yet available. There were difficulties in aggregate reporting due to heterogeneity of the studies, which may present biases in the narrative reporting style.
To the best of our knowledge, this is the only systematic review assessing the association between weight class and outcome following Fontan procedure. Bodyweight (high or low) is a modifiable potential risk factor for poor clinical outcome in patients born with a single ventricle circulation. Whilst there is only limited evidence, with only 15 relevant studies identified, data indicates that extremes of bodyweight, both low and high, are associated with increased morbidity and mortality post-Fontan procedure. Bodyweight—relative to height and age—is a commonly used, widely available, and rapid screening tool that can be used to aid prognosis and direct therapeutic interventions in vulnerable patients. However, many studies in this review comment on the lack of readily available institutional height and weight data. Increased knowledge of the associations between bodyweight and Fontan pathophysiology may help to define an optimal relative weight target for Fontan patients with their unique circulation.
Regarding achievement of weight targets in patients with single ventricle cardiac defects, some studies have suggested the efficacy of home monitoring and interventions in aiding infant growth in the interstage period [85–89]. However, there remains substantial variation in weight targets and management strategies. Suggested strategies include monitoring for weight loss “red flags”, regular dietician and speech pathology phone consultation, postoperative feeding algorithms, and protocols for transition to oral feed [85–89].
Given the propensity for underweight in the CHD population, research is less clear regarding weight loss strategies for overweight patients with single ventricle conditions. A systematic review of weight reduction strategies for patients with chronic healthcare needs found that most interventions to date involve dietary counselling or an energy deficit, and many are family-focused, multicomponent, and are delivered by a multidisciplinary team [90]. Remote lifestyle counselling in a small 12-month pilot study of adolescents with CHD who were overweight and obese resulted in reductions in BMI z-score and waist circumference at 6 and 12 months [91]. However, data remain limited in this area. Further research is required to determine optimum weight targets, as well as the most effective means of reaching these targets in patients with a single ventricle circulation.
Given the paucity of data, further research is required to investigate the association between weight status and clinical outcomes post-Fontan completion. This is important, in part, because of the rising prevalence of obesity in patients with congenital heart disease, and the general population [24–30]. Data from national and international registries incorporating longitudinal growth parameters and long-term outcomes are vital in gathering further information about the impact of excess weight and obesity on SV patients.
Authorship: The authors confirm contribution to the paper as follows: study conception and design: Ayer J, d’Udekem Y, Payne E; data collection: Payne E, Wilson T; analysis and interpretation of results: Haghighi M; draft manuscript preparation: Payne E, McCallum Z. All authors reviewed the results and approved the final version of the manuscript.
Acknowledgement: We thank Tess Aitken, a health sciences librarian at the University of Sydney, NSW for consultation in developing a search strategy.
Funding Statement: The ANZFR gratefully receives funding from the Heart Foundation, Heart Kids, ANZ Trustees, the Murdoch Children’s Research Institute, and the Australian Government National Health and Medical Research Council. Thomas G Wilson is the recipient of a National Health and Medical Research Council (NHMRC) Postgraduate Scholarship (GNT1168270).
Conflicts of Interest: Yves d’Udekem is a consultant for Actelion.
References
- D’udekem, Y., Iyengar, A. J., Galati, J. C., Forsdick, V., & Weintraub, R. G. (2014). Redefining expectations of long-term survival after the fontan procedure twenty-five years of follow-up from the entire population of Australia and New Zealand. Circulation, 130(11), S32-S38. [Google Scholar]
- Bartmus, D. A., Driscoll, D. J., Offord, K. P., Humes, R. A., & Mair, D. D. (1990). The modified Fontan operation for children less than 4 years old. Journal of the American College of Cardiology, 15(2), 429-435. [Google Scholar] [CrossRef]
- Kay, W. A., Moe, T., Suter, B., Tennancour, A., & Chan, A. (2018). Long term consequences of the fontan procedure and how to manage them. Progress in Cardiovascular Disease, 61(3–4), 365-376. [Google Scholar] [CrossRef]
- Pundi, K., Johnson, J., Dearani, J., Pundi, K., & Li, Z. (2015). 40-year follow-up after the fontan operation long-term outcomes of 1,052 patients. Journal of the American College of Cardiology, 66(15), 1700-1710. [Google Scholar] [CrossRef]
- Sadeghpour, A., Rezaei, M. E., Alizadeasl, A. (2014). Comprehensive approach to adult congenital heart disease. In: Fontan Operation, pp. 271–274. London: Springer.
- Downing, T. E., Allen, K. Y., Glatz, A. C., Rogers, L. S., & Ravishankar, C. (2017). Long-term survival after the Fontan operation: Twenty years of experience at a single center. Journal of Thoracic Cardiovascular Surgery, 154(1), 243-253. [Google Scholar] [CrossRef]
- Cohen, M. I., Bush, D. M., Ferry, R. J., Spray, T. L., & Moshang, T. (2000). Somatic growth failure after the Fontan operation. Cardiology in the Young, 10(5), 447-457. [Google Scholar] [CrossRef]
- Kelleher, D., Laussen, P., Teixeira-Pinto, A., & Duggan, C. (2006). Growth and correlates of nutritional status among infants with hypoplastic left heart syndrome (HLHS) after stage 1 Norwood procedure. Nutrition, 22(3), 237-244. [Google Scholar] [CrossRef]
- Newburger, J. W., Sleeper, L. A., Frommelt, P. C., Pearson, G. D., & Mahle, W. T. (2014). Transplantation-free survival and interventions at 3 years in the single ventricle reconstruction trial. Circulation, 129(20), 2013-2020. [Google Scholar] [CrossRef]
- Newburger, J. W., Sleeper, L. A., Gaynor, J. W., Hollenbeck-Pringle, D., & Frommelt, P. C. (2018). Transplant-free survival and interventions at 6 years in the SVR trial. Circulation, 137(21), 2246-2253. [Google Scholar] [CrossRef]
- Schwarz, S., Gewitz, M., See, C., Berezin, S., & Glassman, M. (1990). Enteral nutrition in infants with congenital heart disease and growth failure. Pediatrics, 86(3), 368-373. [Google Scholar] [CrossRef]
- Ades, A., Johnson, B., & Berger, S. (2005). Management of low birth weight infants with congenital heart disease. Clinical Perinatology, 32(4), 999-1015. [Google Scholar] [CrossRef]
- Davis, D., Davis, S., Cotman, K., Worley, S., & Londrico, D. (2008). Feeding difficulties and growth delay in children with hypoplastic left heart syndrome versus d-transposition of the great arteries. Pediatric Cardiology, 29(2), 328-333. [Google Scholar] [CrossRef]
- Rosenthal, G., Wilson, P., Permutt, T., Boughman, J., & Ferencz, C. (1991). Birth weight and cardiovascular malformations: A population-based study: The baltimore-washington infant study. American Journal of Epidemiology, 133(12), 1273-1281. [Google Scholar] [CrossRef]
- Williams, R., Ravishankar, C., Zak, V., Evans, F., & Atz, A. (2010). Birth weight and prematurity in infants with single ventricle physiology: Pediatric heart network infant single ventricle trial screened population. Congenital Heart Disease, 5(2), 96-103. [Google Scholar] [CrossRef]
- Medoff-Cooper, B., & Ravishankar, C. (2013). Nutrition and growth in congenital heart disease: A challenge in children. Current Opinion in Cardiology, 28(2), 122-129. [Google Scholar] [CrossRef]
- Schumacher, K. R., Yu, S., Butts, R., Castleberry, C., & Chen, S. (2019). Fontan-associated protein-losing enteropathy and post–heart transplant outcomes: A multicenter study. The Journal of Heart and Lung Transplant, 38(1), 17-25. [Google Scholar] [CrossRef]
- Carlo, W. F., Carberry, K. E., Heinle, J. S., Morales, D. L., & McKenzie, E. D. (2011). Interstage attrition between bidirectional Glenn and Fontan palliation in children with hypoplastic left heart syndrome. Journal of Thoracic Cardiovascular Surgery, 142(3), 511-516. [Google Scholar] [CrossRef]
- Anderson, J. B., Beekman, R. H., Border, W. L., Kalkwarf, H. J., & Khoury, P. R. (2009). Lower weight-for-age z score adversely affects hospital length of stay after the bidirectional Glenn procedure in 100 infants with a single ventricle. Journal of Thoracic Cardiovascular Surgery, 138(2), 397-404. [Google Scholar] [CrossRef]
- Anderson, J. B., Kalkwarf, H. J., Kehl, J. E., Eghtesady, P., & Marino, B. S. (2011). Low weight-for-age z-score and infection risk after the fontan procedure. The Annals of Thoracic Surgery, 91(5), 1460-1466. [Google Scholar] [CrossRef]
- Menon, S. C., McCandless, R. T., Mack, G. K., Lambert, L. M., & McFadden, M. (2013). Clinical outcomes and resource use for infants with hypoplastic left heart syndrome during bidirectional glenn: Summary from the joint council for congenital heart disease national pediatric cardiology quality improvement collaborative registry. Pediatric Cardiology, 34(1), 143-148. [Google Scholar] [CrossRef]
- Williams, R. V., Zak, V., Ravishankar, C., Altmann, K., & Anderson, J. (2010). Factors affecting growth in infants with single ventricle physiology: A report from the pediatric heart network infant single ventricle trial. Journal of Pediatrics, 159(6), 1017-1022.e2. [Google Scholar] [CrossRef]
- Srinivasan, C., Jaquiss, R. D. B., Morrow, W. R., Frazier, E. A., & Martin, D. A. (2010). Impact of staged palliation on somatic growth in patients with hypoplastic left heart syndrome. Congenital Heart Disease, 5(6), 546-551. [Google Scholar] [CrossRef]
- Ono, M., Boethig, D., Goerler, H., Lange, M., & Westhoff-Bleck, M. (2007). Somatic development long after the Fontan operation: Factors influencing catch-up growth. Journal of Thoracic Cardiovascular Surgery, 134(5), 1199-1206. [Google Scholar] [CrossRef]
- Vogt, K. N., Manlhiot, C., van Arsdell, G., Russell, J. L., & Mital, S. (2007). Somatic growth in children with single ventricle physiology impact of physiologic state. Journal of the American College of Cardiology, 50(19), 1876-1883. [Google Scholar] [CrossRef]
- Newell, A. C., Davis, K., Wang, L., Bichell, D., & Clay, M. A. (2018). Obesity: Risk factor for increased resource utilization at bidirectional glenn. Journal of Parenteral and Enteral Nutrition, 42(1), 49-55. [Google Scholar]
- Cohen, M. S., Zak, V., Atz, A. M., Printz, B. F., & Pinto, N. (2010). Anthropometric measures after Fontan procedure: Implications for suboptimal functional outcome. American Heart Journal, 160(6), 1092-1098. [Google Scholar] [CrossRef]
- Wallace, M. C., Jaggers, J., Li, J. S., Marshall, L., & Jacobs, J. P. (2011). Center variation in patient age and weight at fontan operation and impact on postoperative outcomes. Annals of Thoracic Surgery, 91(5), 1445-1452. [Google Scholar] [CrossRef]
- Power, A., Schultz, L., Dennis, K., Rizzuto, S., & Hollander, A. M. (2020). Growth stunting in single ventricle patients after heart transplantation. Pediatric Transplantation, 24(1), e13634. [Google Scholar] [CrossRef]
- Burch, P. T., Gerstenberger, E., Ravishankar, C., Hehir, D. A., & Davies, R. R. (2014). Longitudinal assessment of growth in hypoplastic left heart syndrome: Results from the single ventricle reconstruction trial. Journal of the American Heart Association, 3(3), 1-12. [Google Scholar] [CrossRef]
- François, K., Bové, T., Panzer, J., de Groote, K., & Vandekerckhove, K. (2012). Univentricular heart and fontan staging: Analysis of factors impacting on body growth. European Journal of Cardio-thoracic Surgery, 41(6), 139-145. [Google Scholar] [CrossRef]
- Majnemer, A., Limperopoulos, C., Shevell, M., Rohlicek, C., & Rosenblatt, B. (2006). Health and well-being of children with congenital cardiac malformations, and their families, following open-heart surgery. Cardiology in the Young, 16(2), 157-164. [Google Scholar] [CrossRef]
- Sandberg, C., Rinnström, D., Dellborg, M., Thilén, U., & Sörensson, P. et al. (2015). Height, weight and body mass index in adults with congenital heart disease. International Journal of Cardiology, 187(1), 219-226. [Google Scholar] [CrossRef]
- Pinto, N., Marino, B., Wernovsky, G., de Ferranti, S., & Walsh, A. (2007). Obesity is a common comorbidity in children with congenital and acquired heart disease. Pediatrics, 120(5), e1157-1164. [Google Scholar] [CrossRef]
- Zaidi, A., Bauer, J., Michalsky, M., Olshove, V., & Boettner, B. (2011). The impact of obesity on early postoperative outcomes in adults with congenital heart disease. Congenital Heart Disease, 6(3), 241-246. [Google Scholar] [CrossRef]
- Maskatia, S., Spinner, J., Nutting, A., Slesnick, T., & Krishnamurthy, R. (2013). Impact of obesity on ventricular size and function in children, adolescents and adults with tetralogy of fallot after initial repair. American Journal of Cardiology, 112(4), 594-598. [Google Scholar] [CrossRef]
- O’Byrne, M. L., McBride, M. G., Paridon, S., & Goldmuntz, E. (2018). Association of habitual activity and body mass index in survivors of congenital heart surgery: A study of children and adolescents with tetralogy of fallot, transposition of the great arteries, and fontan palliation. World Journal for Pediatric and Congenital Heart Surgery, 9(2), 177-184. [Google Scholar] [CrossRef]
- Senzaki, H., Masutani, S., Ishido, H., Taketazu, M., & Kobayashi, T. (2006). Cardiac rest and reserve function in patients with fontan circulation. Journal of the American College of Cardiology, 47(12), 2528-2535. [Google Scholar] [CrossRef]
- Freud, L. R., Webster, G., Costello, J. M., Tsao, S., & Rychlik, K. (2015). Growth and obesity among older single ventricle patients presenting for fontan conversion. World Journal for Pediatric and Congenital Heart Surgery, 6(4), 514-520. [Google Scholar] [CrossRef]
- Anderson, P. A. W., Sleeper, L. A., Mahony, L., Colan, S. D., & Atz, A. M. (2008). Contemporary outcomes after the Fontan procedure: A Pediatric Heart Network multicenter study. Journal of the American College of Cardiology, 52(2), 85-98. [Google Scholar] [CrossRef]
- Brida, M., Dimopoulos, K., Kempny, A., Liodakis, E., & Alonso-Gonzalez, R. (2017). Body mass index in adult congenital heart disease. Heart, 103(16), 1250-1257. [Google Scholar] [CrossRef]
- Zaqout, M., Vandekerckhove, K., Michels, N., Demulier, L., & Bove, T. (2019). Body mass index in adults with congenital heart disease. Congenital Heart Disease, 14(3), 479-486. [Google Scholar] [CrossRef]
- Payne, E., Wilson, T., Haghighi, M., McCallum, Z., d’Udekem, Y. et al. (2021). Weight status, morbidity, and mortality in patients with single ventricle cardiac defects post-Fontan procedure: Protocol for a systematic review. PROSPERO: International Prospective Register of Systematic Reviews. https://www.crd.york.ac.uk/prospero/display_record.php?ID=CRD42021272315.
- Covidence Systematic Review Software, Veritas Health Innovation, Melbourne, Australia. www.covidence.org.
- Udholm, S., Aldweib, N., Hjortdal, V. E., & Veldtman, G. R. (2018). Prognostic power of cardiopulmonary exercise testing in Fontan patients: A systematic review. Open Heart Journal, 5(1), e000812. [Google Scholar] [CrossRef]
- Wang, T., Chen, L., Yang, T., Huang, P., & Wang, L. (2019). Congenital heart disease and risk of cardiovascular disease: A meta-analysis of cohort studies. Journal of the American Heart Association, 8(10), e012030. [Google Scholar] [CrossRef]
- Cortese, S., Moreira-Maia, C. R., St Fleur, D., Morcillo-Peñalver, C., & Rohde, L. (2016). Association between ADHD and obesity: A systematic review and meta-analysis. American Journal of Psychiatry, 173(1), 34-43. [Google Scholar] [CrossRef]
- Eikelboom, R., Sanjanwala, R., Le, M. L., Yamashita, M., & Rakesh, A. (2021). Postoperative atrial fibrillation after cardiac surgery: A systematic review and meta-analysis. Annals of Thoracic Surgery, 111(2), 544-554. [Google Scholar] [CrossRef]
- Liberali, R., Kupek, E., & de Assis, M. (2020). Dietary patterns and childhood obesity risk: A systematic review. Childhood Obesity, 16(2), 70-85. [Google Scholar] [CrossRef]
- Banks, L., McCrindle, B. W., Russell, J. L., & Longmuir, P. E. (2013). Enhanced physiology for submaximal exercise in children after the Fontan procedure. Medicine and Science in Sports and Exercise, 45(4), 615-621. [Google Scholar] [CrossRef]
- Callegari, A., Neidenbach, R., Milanesi, O., Castaldi, B., & Christmann, M. (2019). A restrictive ventilatory pattern is common in patients with univentricular heart after Fontan palliation and associated with a reduced exercise capacity and quality of life. Congenital Heart Disease, 14(2), 147-155. [Google Scholar] [CrossRef]
- Mancilla, E. E., Zielonka, B., Roizen, J. D., Dodds, K. M., & Rand, E. B. (2021). Growth in children with a Fontan circulation. The Journal of Pediatrics, 235, 149-155.e2. [Google Scholar] [CrossRef]
- Menon, S. C., Al-Dulaimi, R., McCrindle, B. W., Goldberg, D. J., & Sachdeva, R. (2018). Delayed puberty and abnormal anthropometry and its associations with quality of life in young Fontan survivors: A multicenter cross-sectional study. Congenital Heart Disease, 13(3), 463-469. [Google Scholar] [CrossRef]
- Nanayakkara, B., Lau, E., Yee, B., Gauthier, G., & Melehan, K. (2020). Sleep disordered breathing in adults living with a Fontan circulation and CPAP titration protocol. International Journal of Cardiology, 317(1), 70-74. [Google Scholar] [CrossRef]
- Weinreb, S. J., Dodds, K. M., Burstein, D. S., Huang, J., & Rand, E. B. (2020). End-organ function and exercise performance in patients with fontan circulation: What characterizes the high performers?. Journal of the American Heart Association, 9(24), 1-10. [Google Scholar] [CrossRef]
- Byrne, R. D., Weingarten, A. J., Clark, D. E., Healan, S. J., & Richardson, T. L. (2021). Sizing up Fontan failure: Association with increasing weight in adulthood. Pediatric Cardiology, 42(6), 1425-1432. [Google Scholar] [CrossRef]
- Chung, S. T., Hong, B., Patterson, L., Petit, C. J., & Ham, J. N. (2016). High overweight and obesity in fontan patients: A 20-year history. Pediatric Cardiology, 37(1), 192-200. [Google Scholar] [CrossRef]
- Day, R. W., Denton, D. M., & Jackson, W. D. (2000). Growth of children with a functionally single ventricle following palliation at moderately increased altitude. Cardiology in the Young, 10(3), 193-200. [Google Scholar] [CrossRef]
- Goldberg, D. J., Zak, V., McCrindle, B. W., Ni, H., & Gongwer, R. (2021). Exercise capacity and predictors of performance after Fontan: Results from the Pediatric Heart Network Fontan 3 study. Pediatric Cardiology, 42(1), 158-168. [Google Scholar] [CrossRef]
- Lambert, L. M., McCrindle, B. W., Pemberton, V. L., Hollenbeck-Pringle, D., & Atz, A. M. (2020). Longitudinal study of anthropometry in Fontan survivors: Pediatric Heart Network Fontan study. American Heart Journal, 224(6), 192-200. [Google Scholar] [CrossRef]
- Martinez, S. C., Byku, M., Novak, E. L., Cedars, A. M., & Eghtesady, P. (2016). Increased body mass index is associated with congestive heart failure and mortality in adult fontan patients. Congenital Heart Disease, 11(1), 71-79. [Google Scholar] [CrossRef]
- Rychik, J. (2007). Protein-losing enteropathy after Fontan operation. Congenital Heart Disease, 2(5), 288-300. [Google Scholar] [CrossRef]
- Yoon, T. G., Jang, K., Oh, C. S., Kim, S. H., & Kang, W. S. (2018). The correlation between the change in thoracic fluid content and the change in patient body weight in Fontan procedure. Biomed Research International, 2018, 3635708. [Google Scholar] [CrossRef]
- Wellnitz, K., Harris, I. S., Sapru, A., Fineman, J. R., & Radman, M. (2015). Longitudinal development of obesity in the post-Fontan population. European Journal of Clinical Nutrition, 69(10), 1105-1108. [Google Scholar] [CrossRef]
- Rychik, J. (2016). The relentless effects of the Fontan paradox. Seminars in Thoracic and Cardiovascular Surgery: Pediatric Cardiac Surgery Annual, 19(1), 37-43. [Google Scholar]
- Hsia, T. Y., Khambadkone, S., Redington, A. N., Migliavacca, F., & Deanfield, J. E. (2000). Effects of respiration and gravity on infradiaphragmatic venous flow in normal and Fontan patients. Circulation, 102, III148-53. [Google Scholar]
- Shekerdemian, L. S., Bush, A., Shore, D. F., Lincoln, C., & Redington, A. N. (1997). Cardiopulmonary interactions after Fontan operations: Augmentation of cardiac output using negative pressure ventilation. Circulation, 96(11), 3934-3942. [Google Scholar] [CrossRef]
- Centers for Disease Control and Prevention (CDC) (2000). CDC growth charts. http://www.cdc.gov/growthcharts/.
- Butto, A., Mercer-Rosa, L., Teng, C., Daymont, C., & Edelson, J. (2019). Longitudinal growth in patients with single ventricle cardiac disease receiving tube-assisted feeds. Congenital Heart Disease, 14(6), 1058-1065. [Google Scholar] [CrossRef]
- Hill, G. D., Tanem, J., Ghanayem, N., Rudd, N., & Ollberding, N. J. (2020). Selective use of inpatient interstage management after norwood procedure. Annals of Thoracic Surgery, 109(1), 139-147. [Google Scholar] [CrossRef]
- Lavie, C. J., Sharma, A., Alpert, M. A., de Schutter, A., & Lopez-Jimenez, F. (2016). Update on obesity and obesity paradox in heart failure. Progress in Cardiovascular Disease, 58(4), 393-400. [Google Scholar] [CrossRef]
- Vitanova, K., Shiraishi, S., Mayr, B., Beran, E., & Cleuziou, J. (2019). Impact of characteristics at stage-2-palliation on outcome following fontan completion. Pediatric Cardiology, 40(7), 1476-1487. [Google Scholar] [CrossRef]
- Elagizi, A., Kachur, S., Lavie, C. J., Carbone, S., & Pandey, A. (2018). An overview and update on obesity and the obesity paradox in cardiovascular diseases. Progress in Cardiovascular Disease, 61(2), 142-150. [Google Scholar] [CrossRef]
- Sharma, A., Lavie, C. J., Borer, J. S., Vallakati, A., & Goel, S. (2015). Meta-analysis of the relation of body mass index to all-cause and cardiovascular mortality and hospitalization in patients with chronic heart failure. American Journal of Cardiology, 115(10), 1428-1434. [Google Scholar] [CrossRef]
- Tran, D., D’Ambrosio, P., Verrall, C. E., Attard, C., & Briody, J. (2020). Body composition in young adults living with a Fontan circulation: The myopenic profile. Journal of the American Heart Association, 9(8), e015639. [Google Scholar] [CrossRef]
- Cordina, R., O’Meagher, S., Gould, H., Rae, C., & Kemp, G. (2013). Skeletal muscle abnormalities and exercise capacity in adults with a Fontan circulation. Heart, 99(23), 1530-1534. [Google Scholar] [CrossRef]
- Sandberg, C., Johansson, K., Christersson, C., Hlebowicz, J., & Thilén, U. (2019). Sarcopenia is common in adults with complex congenital heart disease. International Journal of Cardiology, 296(2), 57-62. [Google Scholar] [CrossRef]
- Avitabile, C. M., Leonard, M. B., Zemel, B. S., Brodsky, J. L., & Lee, D. (2014). Lean mass deficits, vitamin D status and exercise capacity in children and young adults after Fontan palliation. Heart, 100(21), 1702-1707. [Google Scholar] [CrossRef]
- Possner, M., Alsaied, T., Siddiqui, S., Morales, D., & Trout, A. T. (2020). Abdominal skeletal muscle index as a potential novel biomarker in adult Fontan patients. Canadian Journal of Cardiology Open, 2(2), 55-61. [Google Scholar] [CrossRef]
- Avitabile, C. M., Leonard, M. B., Zernei, B. S., Brodksy, J. L., & Lee, L. (2014). Lean mass deficits vitamin D status and exercise capacity in children and young adults after Fontan palliation. Heart, 100(20), 1702-1707. [Google Scholar] [CrossRef]
- Avitabile, C. M., Goldberg, D. J., Leonard, M. B., Wei, Z. A., & Tang, E. (2018). Leg lean mass correlates with exercise systemic output in young Fontan patients. Heart, 104(8), 680-684. [Google Scholar] [CrossRef]
- Ohuchi, H., Negishi, J., Miike, H., Toyoshima, Y., & Morimoto, H. (2019). Positive pediatric exercise capacity trajectory predicts better adult Fontan physiology rationale for early establishment of exercise habits. International Journal of Cardiology, 274, 80-87. [Google Scholar] [CrossRef]
- Cao, J. Y., Tran, D., Briody, J., Attard, C., & Hassan, E. B. (2021). Impact of adiposity on clinical outcomes in people living with a Fontan circulation. International Journal of Cardiology, 329, 82-88. [Google Scholar] [CrossRef]
- Lubert, A. M., Alsaied, T., Palermo, J. J., Anwar, N., & Urbina, E. M. (2021). Fontan-associated dyslipidemia. Journal of the American Heart Association, 10(7), e019578. [Google Scholar] [CrossRef]
- Anderson, J. B., Iyer, S. B., Schidlow, D. N., Williams, R., & Varadarajan, K. (2012). Variation in growth of infants with a single ventricle. The Journal of Pediatrics, 161(1), 16-21. [Google Scholar] [CrossRef]
- Ghanayem, N. S., Cava, J. R., Jaquiss, R. D. B., & Tweddell, J. S. (2004). Home monitoring of infants after stage one palliation for hypoplastic left heart syndrome. Seminars in Thoracic and Cardiovascular Surgery: Pediatric Cardiac Surgery Annual, 2004(7), 32-38. [Google Scholar]
- Weston, C., Husain, S., Curzon, C., Neish, S., & Kennedy, G. (2016). Improving outcomes for infants with single ventricle physiology through standardized feeding during the interstage. Nursing Research and Practice, 2016(10), 9505629. [Google Scholar] [CrossRef]
- Oster, M. E., Ehrlich, A., King, E., Petit, C. J., & Clabby, M. (2015). Association of interstage home monitoring with mortality, readmissions, and weight gain: A multicenter study from the National Pediatric Cardiology Quality Improvement Collaborative. Circulation, 2015(132), 502-508. [Google Scholar] [CrossRef]
- Hehir, D. A., Rudd, N., Slicker, J., Mussatto, K. A., & Simpson, P. (2012). Normal interstage growth after the Norwood operation associated with interstage home monitoring. Pediatric Cardiology, 33(8), 1315-1322. [Google Scholar] [CrossRef]
- Billich, N., Maugri, I., Calligaro, L., Truby, H., & Davidson, Z. E. (2022). Weight management interventions that include dietary components for young people with chronic health care needs: A systematic review. Nutrition and Dietetics, 71(1), 94-109. [Google Scholar] [CrossRef]
- Atamirano-Diaz, L., Rombeek, M., de Jesus, S., Welish, E., & Prapavessis, H. (2017). Remote lifestyle counseling influences cardiovascular health outcomes in youth with overweight or obesity and congenital heart disease. Frontiers in Pediatrics, 5, 269. [Google Scholar]
Search Terms: Medline (OVID)
1. exp Fontan procedure/
2. exp univentricular heart/su [Surgery]
3. exp norwood procedures/
4. (single-ventric* or bcpc or univentric* or uni-ventric* or monoventric*).tw, kf.
5. ((bidirectional-cavopulmonary or bi-directional-cavopulmonary or total-cavopulmoary or cavopulmonary or glenn) adj2 (shunt? or procedure? or connection?)).tw, kf.
6. 1 or 2 or 3 or 4 or 5
7. adiposity/
8. exp body weight changes/
9. obesity, abdominal/
10. obesity, morbid/
11. pediatric obesity/
12. thinness/
13. body mass index/
14. (bmi or body-mass-index or weight-for-age or weight-for-height or weight-for-length or overweight* or underweight* or failure-to-thrive or ftt or (z-score adj3 weight*) or (z-score adj3 bmi)).tw, kf.
15. (p?ediatr* adj2 obes*).tw, kf.
16. 7 or 8 or 9 or 10 or 11 or 12 or 13 or 14
17. 6 and 15
18. Limit 16 to English language
Search Terms: EMBASE (OVID)
1. exp Fontan procedure/
2. exp norwood procedure/
3. exp cavopulmonary connection/
4. Glenn shunt/
5. exp heart single ventricle/
6. (single-ventric* or bcpc or univentric* or uni-ventric* or monoventric*).tw, kf, dq.
7. ((bidirectional-cavopulmonary or bi-directional-cavopulmonary or total-cavopulmoary or cavopulmonary or glenn) adj2 (shunt? or procedure? or connection?)).tw, kf, dq.
8. 1 or 2 or 3 or 4 or 5 or 6 or 7
9. exp weight change/
10. exp obesity/
11. stunting/
12. failure to thrive/
13. exp underweight/
14. neonatal weight loss/
15. wasting syndrome/
16. (bmi or body-mass-index or weight-for-age or weight-for-height or weight-for-length or overweight* or underweight* or failure-to-thrive or ftt or (z-score adj3 weight*) or (z-score adj3 bmi)).tw, kf, dq.
17. (p?ediatr* adj2 obes*).tw, kf, dq.
18. 10 or 11 or 12 or 13 or 14 or 15 or 16 or 17
19. 8 and 17
20. Limit 18 to english language
SCOPUS
((fontan OR norwood OR cavopulmonary OR glenn OR bcpc) w/2 (shunt* OR procedur* OR connection* OR operation*)) OR “single ventric*” OR univentric* OR monoventric*
AND
(bmi OR “body mass index” OR weight-for-age OR weight-for-height OR weight-for-length OR overweight OR underweight OR failure-to-thrive OR ftt OR waz OR whz OR obes* OR stunt* OR “weight loss”)
Cochrane
1. MeSH descriptor: [Fontan Procedure] explode all trees
2. MeSH descriptor: [Univentricular Heart] explode all trees
3. MeSH descriptor: [Norwood Procedures] explode all trees
4. (single ventric* or bcpc or single-ventricle or univentric* or uni-ventric* or monoventric*):ti, ab, kw
5. ((bidirectional-cavopulmonary or bi-directional-cavopulmonary or cavopulmonary or “total near/2 cavopulmonary” or glenn) next (shunt? or procedure? or connection?)):ti, ab, kw
6. MeSH descriptor: [Obesity, Abdominal] explode all trees
7. MeSH descriptor: [Pediatric Obesity] explode all trees
8. MeSH descriptor: [Obesity, Morbid] explode all trees
9. MeSH descriptor: [Overweight] this term only
10. MeSH descriptor: [Body Weight Changes] explode all trees
11. MeSH descriptor: [Body Mass Index] explode all trees
12. MeSH descriptor: [Thinness] explode all trees
13. MeSH descriptor: [Adiposity] explode all trees
14. (bmi or “body mass index” or weight-for-age or weight-for-height or weight-for-length or overweight* or underweight* or “failure to thrive” or ftt or (z-score near/3 weight*) or (z-score near/3 bmi)):ti, ab, kw
15. (p?ediatr* near/2 obes*):ti, ab, kw
16. #1 or #2 or #3 or #4 or #5
17. #6 or #7 or #8 or #9 or #10 or #11 or #12 or #13 or #14 or #15
18. #16 and #17
Supplementary Tables
Cite This Article
Payne, E., Wilson, T., Haghighi, M., McCallum, Z., D’Udekem, Y. et al. (2022). Associations between Bodyweight and Clinical Outcome in Patients Post-Fontan Procedure: A Systematic Review. Congenital Heart Disease, 17(6), 617–639. https://doi.org/10.32604/chd.2022.024775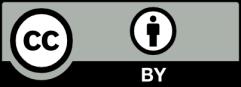