Open Access
COMMENTARY
Retinal Focus on Relationships between Diet-Induced, Advanced Glycation End Products and Supplemental Estradiol
1 School of Environmental and Biological Sciences, Department of Animal Sciences, Rutgers, The State University of New Jersey, New Brunswick, NJ 08901, USA
2 School of Arts and Sciences, Department of Cell Biology and Neuroscience, Rutgers, The State University of New Jersey, Piscataway, NJ 08854, USA
3 School of Engineering, Department of Biomedical Engineering, Rutgers, The State University of New Jersey, Piscataway, NJ 08854, USA
* Corresponding Author: Maribel Vazquez. Email:
(This article belongs to the Special Issue: Advances in Cellular and Molecular Mechanisms and Therapeutic Strategies for Neurodegenerative Diseases)
BIOCELL 2025, 49(3), 349-354. https://doi.org/10.32604/biocell.2025.061810
Received 03 December 2024; Accepted 04 March 2025; Issue published 31 March 2025
Abstract
Neurodegeneration of retinal tissue leads to progressive vision loss in millions of working-age adults each year. Metabolic alterations caused by modern diets that are high in fats and sugars contribute to the development of diabetic retinopathy. Chronic, diet-induced metabolic changes are linked to high glucose and harmful, pro-inflammatory compounds in the blood, called advanced glycation end products (AGEs), that can alter the integrity of neurovascular barriers. AGEs-induced changes to the permeability of the inner blood-retinal barrier can lead to progressive vision loss with disparate impacts in patients with low estrogen, such as via natural aging processes, chronic illness, or aggressive medical interventions. Application of supplemental estradiol has been shown to ameliorate degeneration across the cardiovascular, intestinal, and nervous systems. This commentary describes the potential benefits of estradiol-based therapies on the visual health of older women with progressive diabetic retinopathy.Keywords
1 Diet-Induced Neuroinflammation
Degenerative vision loss is an escalating global challenge as millions of adults will lose their sight with aging due to retinal diseases, such as age-related macular degeneration, glaucoma, and diabetic retinopathy [1,2]. A dramatic rise in progressive vision loss from diabetic retinopathy is driven by the rising incidence of Type II diabetes mellitus (T2DM), associated with metabolic alterations from the excess intake of calories [3,4]. Modern-day ultra-processed foods, high in fats and sugars, can also stimulate neuroinflammation via an altered balance of intestinal microbiota leading to elevated neuronal metabolism [5], and persistently activated inflammatory signaling pathways [6]. The contributing mechanisms to these chronic, diet-induced metabolic changes are linked to the accumulation of harmful, pro-inflammatory compounds in the blood, called advanced glycation end products (AGEs). Many AGEs are produced from the Maillard reaction, which is the browning process during cooking or baking to enhance the color and taste of food. Alarmingly, AGEs can alter protein structure and function and work in concert with high blood glucose to promote inflammatory signaling and neurodegeneration [7]. Moreover, non-nutritive additives used extensively in the global food supply chain, such as anti-microbial, synthetic preservatives, and artificial sweeteners [8], bind to ingested proteins, adhere to the extracellular matrix, and interact with surface receptors to create inclusion complexes that impact ocular outcomes [9]. As a result, research into the metabolic and neural impact of diet compositions and non-nutritive additives has been at the forefront of nutritional neuroscience, as shown in Fig. 1.
Figure 1: Schematic description of the impacts of diet-based, advanced glycation end products (AGEs) on inflammatory pathways, metabolic alterations, and the inner blood-retinal barrier (iBRB). Image created with BioRender
Inflammation-induced changes can be critical for influencing the permeability of neurovascular barriers, such as the blood-brain barrier (BBB) and inner blood-retinal barrier (iBRB), which regulates transport from circulating blood. Indeed, inflammatory-induced vascular changes can impede the clearance of metabolic waste allowing harmful, blood-borne molecules and cells to enter the brain. In vivo studies of high-fat diets have implicated excess consumption with cognitive decline, which is due, in part, to insulin resistance (Reviewed in [10]). In line with these findings, our group has reported high-fat diet-induced changes in rodent sensorimotor gating of the acoustic startle response [11] that reflects similar data gathered from non-human primates and clinical patients with neurovascular disorders of the prefrontal and visual cortex [12,13]. Analogous alterations can be involved in the diet-induced, metabolic changes associated with other vascular barriers. The iBRB of the eye is a vision-critical, semi-permeable membrane that regulates the transport of molecules and ions between the retinal capillaries and the neuroretina. The iBRB is comprised of endothelial cells and pericytes, alongside Muller glia and astrocytes that communicate via junctional, gap, and adhesion proteins, among others (Fig. 2). Molecules, such as zonula occludens, facilitate the formation of monolayer barriers of endothelial cells to provide resistance to the transport of harmful compounds from circulating blood to neural tissue. Endothelial cells respond collectively with neuroglia in barrier tissues, as these retinal cells are key regulators of oxygen, toxins, and nutrients needed to support neuronal synapses for vision [14,15]. Neuroglia, thereby, exhibits bi-directional communication with endothelial cells via gap junctions as well as with one another through gliotransmitters, such as glutamate [16]. AGEs are well-known to cause pericyte death and stimulate astrogliosis in patients with chronic, diabetic retinopathy [17], indicating that collective responses from barrier cells are significant but understudied components of emerging therapies.
Figure 2: Structure of the inner blood-retinal barrier (iBRB) and communication between endothelial cells (ECs) and neuroglia (NG). The inner blood-retinal barrier is comprised of endothelial cells that line the inner lumen of retinal capillaries, pericytes that wrap around the capillaries, and neuroglia of the retina that communicate via synapse with retinal neurons to facilitate vision with permission from [15]. These cell types form a dynamic tissue barrier and collectively communicate with one another across a basement membrane comprised largely of collagen IV and laminin. Neuroglia cells primarily communicate with each other, and with endothelial cells, via gap junctions formed by connexin 43 (CX-43) proteins, to enable the passage of small molecules via juxtracrine communication. In complement, endothelial cells form tight junctions, most notably zonula occludens-1 (ZO-1), that selectively regulate transcellular transport across the cell barrier. When the integrity of the iBRB is compromised, higher permeability across the vascular wall leads to unregulated molecular transport of water, toxins, and cytokines that disrupt cell signaling and lead to the dysfunction and eventual death of retinal neurons
3 Estrogen as a Retinal Neuroprotectant
A growing body of evidence demonstrates that estrogen decline during natural aging processes or medically treated conditions drives a systemic inflammatory state [18]. This low-grade chronic inflammation can compromise the iBRB to make the retina more susceptible to neurodegenerative disease [19]. Clinical data illustrate that women are less disposed to the effects of neurodegeneration before menopause transition [20], suggesting that rising, worldwide incidence in women as their age may be related to decreasing levels of circulating estrogen. The neuroprotective effects of beta-estradiol in the nervous system are well-established, including major anti-inflammatory effects on microglia. Estrogen also facilitates higher cognitive functions by inducing spinogenesis and synaptogenesis in the visual cortex and hippocampus [21], which play important roles in vision and learning. Moreover, supplemental estrogen improves memory and reduces the accumulation of amyloid-beta prodromal to Alzheimer’s disease in female mice after ovariectomy [20].
Estrogen receptors are present on endothelial, pericyte, and neuroglial cells of the iBRB, where recent studies suggest that hormonal imbalance may serve as global markers for inflammation and metabolic syndromes that impact neurovascular outcomes [22]. Moreover, activated receptors are shown to prevent oxidative stress and stimulate the production of neurotrophic factors that attenuate retinal degeneration. Recent work from our group illustrates that supplemental estradiol was able to increase the resistivity of cell barriers formed by, both, endothelial and neuroglial cells of the iBRB [23] by impacting gap and tight junctional communication [15].
Significant biological sex-based, health disparities have been recorded in vision loss [24], where women experience disparate impacts and poorer outcomes of retinal disease as they grow into mature adulthood. Globally, women over the age of 55 endure progressive vision loss from diabetic retinopathy at higher rates than their male counterparts. The combination of lifelong age consumption in the form of ultra-processed foods and lowered estrogen may produce low-grade chronic inflammation in aging women that fuels retinal degeneration. Our group has developed micro-physiological systems that model molecular transport across retinal tissue and demonstrate that increased concentrations of AGEs elevate the production of cell reactive oxidation species, alter cell morphology, and increase the formation of blood clots in retinal vascular barriers [25]. Our recent studies have used endothelial-glial bilayers to illustrate that increased AGEs reduce glia-to-glia and glia-to-endothelial cell communication via decreased expression of the critical proteins connexin 43 (Cx43) and a cluster of differentiation 40 (CD40) [15]. Moreover, as patients with diabetic retinopathy are frequently treated with anti-VEGF pharmacotherapies to reduce ocular angiogenesis (e.g., Avastin and Eylea), we tested the effects of anti-VEGF on the resistivity of bilayers of iBRB cells. Our results showed that bilayers of endothelial and glial cells cultured long-term in high glucose and AGEs exhibited greater barrier resistivity (measured via Trans-Endothelial Electrical Resistance, or TEER) after treatment with anti-VEGF than did respective bilayers cultured in normoglycemic medium [15]; suggesting an underexplored method of action for anti-VEGF. Surprisingly, the effects of AGEs in all studies were attenuated with supplemental estradiol [23], highlighting understudied molecular relationships between hormones and cells of barrier tissue that are significant to aging as well as to women’s health.
In summary, accruing evidence illustrates that supplemental estradiol may have neuroprotective effects throughout aging, such as during menopause transition and chronic illness or treatment. Multiple recent studies suggest direct positive benefits of estradiol in combination with accumulating levels of AGEs consumed in modern high-fat diets. These understudied relationships are critical to emerging therapies for women’s health as AGEs impact the collective cell behavior of neurovascular barriers and could adversely influence the transport of non-nutritive additives and pharmaceutical agents across the BBB and iBRB.
Acknowledgement: Not applicable.
Funding Statement: This research was funded by a Life Sciences Alliance Pilot Seed Grant from the Rutgers–New Brunswick Office of the Vice Provost for Research, Grant Number OVPR-100 (MV), https://newbrunswick.rutgers.edu/provost/research/life-sciences-alliance (accessed on 3 March 2025).
Author Contributions: The authors confirm contribution to the paper as follows: Conceptualization, Nicholas T. Bello, Bonnie L. Firestein, and Maribel Vazquez; investigation, Nicholas T. Bello, Bonnie L. Firestein, and Maribel Vazquez; resources, Nicholas T. Bello, Bonnie Firestein, and Maribel Vazquez; writing—original draft preparation, Maribel Vazquez; writing—review and editing, Nicholas T. Bello, Bonnie L. Firestein, and Maribel Vazquez; visualization, Nicholas T. Bello, Bonnie L. Firestein, and Maribel Vazquez; funding acquisition, Nicholas T. Bello, Bonnie L. Firestein, and Maribel Vazquez. All authors reviewed the results and approved the final version of the manuscript.
Availability of Data and Materials: This article does not involve data availability.
Ethics Approval: Not applicable.
Conflicts of Interest: The authors declare no conflicts of interest to report regarding the present study.
References
1. Rein DB, Wittenborn JS, Zhang P, Sublett F, Lamuda PA, Lundeen EA, et al. The economic burden of vision loss and blindness in the United States. Ophthalmology. 2022;129(4):369–78. doi:10.1016/j.ophtha.2021.09.010. [Google Scholar] [PubMed] [CrossRef]
2. GBD 2019 Blindness and Vision Impairment Collaborators, Vision Loss Expert Group of the Global Burden of Disease Study. Trends in prevalence of blindness and distance and near vision impairment over 30 years: an analysis for the global burden of disease study. Lancet Glob Health. 2021;9(2):e130–43. doi:10.1016/S2214-109X(20)30425-3. [Google Scholar] [PubMed] [CrossRef]
3. Milluzzo A, Maugeri A, Barchitta M, Sciacca L, Agodi A. Epigenetic mechanisms in type 2 diabetes retinopathy: a systematic review. Int J Mol Sci. 2021;22(19):10502. doi:10.3390/ijms221910502. [Google Scholar] [PubMed] [CrossRef]
4. Iatcu CO, Steen A, Covasa M. Gut microbiota and complications of type-2 diabetes. Nutrients. 2021;14(1):166. doi:10.3390/nu14010166. [Google Scholar] [PubMed] [CrossRef]
5. Cavaliere G, Trinchese G, Penna E, Cimmino F, Pirozzi C, Lama A, et al. High-fat diet induces neuroinflammation and mitochondrial impairment in mice cerebral cortex and synaptic fraction. Front Cell Neurosci. 2019;13:509. doi:10.3389/fncel.2019.00509. [Google Scholar] [PubMed] [CrossRef]
6. Duan Y, Zeng L, Zheng C, Song B, Li F, Kong X, et al. Inflammatory links between high fat diets and diseases. Front Immunol. 2018;9:2649. doi:10.3389/fimmu.2018.02649. [Google Scholar] [PubMed] [CrossRef]
7. Shen CY, Lu CH, Wu CH, Li KJ, Kuo YM, Hsieh SC, et al. The development of maillard reaction, and advanced glycation end product (AGE)-receptor for AGE (RAGE) signaling inhibitors as novel therapeutic strategies for patients with AGE-related diseases. Molecules. 2020;25(23):5591. doi:10.3390/molecules25235591. [Google Scholar] [PubMed] [CrossRef]
8. Iizuka K. Is the use of artificial sweeteners beneficial for patients with diabetes mellitus? the advantages and disadvantages of artificial sweeteners. Nutrients. 2022;14(21):4446. doi:10.3390/nu14214446. [Google Scholar] [PubMed] [CrossRef]
9. Clarkson-Townsend DA, Douglass AJ, Singh A, Allen RS, Uwaifo IN, Pardue MT. Impacts of high fat diet on ocular outcomes in rodent models of visual disease. Exp Eye Res. 2021;204(12):108440. doi:10.1016/j.exer.2021.108440. [Google Scholar] [PubMed] [CrossRef]
10. Freeman LR, Haley-Zitlin V, Rosenberger DS, Granholm AC. Damaging effects of a high-fat diet to the brain and cognition: a review of proposed mechanisms. Nutr Neurosci. 2014;17(6):241–51. doi:10.1179/1476830513Y.0000000092. [Google Scholar] [PubMed] [CrossRef]
11. Scarpa LL, Bello NT. Dietary-induced binge-like eating impairs acoustic startle responses to acute nisoxetine in male mice. Behav Pharmacol. 2023;34(7):411–23. doi:10.1097/FBP.0000000000000748. [Google Scholar] [PubMed] [CrossRef]
12. Rolls ET. Sensory processing in the brain related to the control of food intake. Proc Nutr Soc. 2007;66(1):96–112. doi:10.1017/S0029665107005332. [Google Scholar] [PubMed] [CrossRef]
13. Adams MS, Andrew D, Staines WR. The contribution of the prefrontal cortex to relevancy-based gating of visual and tactile stimuli. Exp Brain Res. 2019;237(10):2747–59. doi:10.1007/s00221-019-05633-9. [Google Scholar] [PubMed] [CrossRef]
14. Pena JS, Vazquez M. Harnessing the neuroprotective behaviors of muller glia for retinal repair. Front Biosci. 2022;27(6):169. doi:10.31083/j.fbl2706169. [Google Scholar] [PubMed] [CrossRef]
15. Pena JS, Berthiaume F, Vazquez M. Muller glia co-regulate barrier permeability with endothelial cells in an vitro model of hyperglycemia. Int J Mol Sci. 2024;25(22):12271. doi:10.3390/ijms252212271. [Google Scholar] [PubMed] [CrossRef]
16. Kugler EC, Greenwood J, MacDonald RB. The “Neuro-Glial-Vascular” unit: the role of glia in neurovascular unit formation and dysfunction. Front Cell Dev Biol. 2021;9:732820. doi:10.3389/fcell.2021.732820. [Google Scholar] [PubMed] [CrossRef]
17. Yamagishi S, Nakamura K, Imaizumi T. Advanced glycation end products (AGEs) and diabetic vascular complications. Curr Diabetes Rev. 2005;1(1):93–106. doi:10.2174/1573399052952631. [Google Scholar] [PubMed] [CrossRef]
18. McCarthy M, Raval AP. The peri-menopause in a woman’s life: a systemic inflammatory phase that enables later neurodegenerative disease. J Neuroinflammation. 2020;17(1):317. doi:10.1186/s12974-020-01998-9. [Google Scholar] [PubMed] [CrossRef]
19. Kutsyr O, Noailles A, Martinez-Gil N, Maestre-Carballa L, Martinez-Garcia M, Maneu V, et al. Short-term high-fat feeding exacerbates degeneration in retinitis pigmentosa by promoting retinal oxidative stress and inflammation. Proc Natl Acad Sci U S A. 2021;118(43):e2100566118. doi:10.1073/pnas.2100566118. [Google Scholar] [PubMed] [CrossRef]
20. Saha P, Weigle IQ, Slimmon N, Poli PB, Patel P, Zhang X, et al. Early modulation of the gut microbiome by female sex hormones alters amyloid pathology and microglial function. Sci Rep. 2024;14(1):1827. doi:10.1038/s41598-024-52246-6. [Google Scholar] [PubMed] [CrossRef]
21. Sengupta DC, Lantz CL, Rumi MAK, Quinlan EM. 17α Estradiol promotes plasticity of spared inputs in the adult amblyopic visual cortex. Sci Rep. 2019;9(1):19040. doi:10.1038/s41598-019-55158-y. [Google Scholar] [PubMed] [CrossRef]
22. Dubey P, Singh V, Venishetty N, Trivedi M, Reddy SY, Lakshmanaswamy R, et al. Associations of sex hormone ratios with metabolic syndrome and inflammation in US adult men and women. Front Endocrinol. 2024;15:1384603. doi:10.3389/fendo.2024.1384603. [Google Scholar] [PubMed] [CrossRef]
23. Castro N, Pena JS, Cliver R, Berthiaume F, Vazquez M. Estradiol impacts muller glia and endothelial cell responses in hyperglycemic microenvironments with advanced glycation end products. Exp Eye Res. 2024;251:110185. doi:10.1016/j.exer.2024.110185. [Google Scholar] [PubMed] [CrossRef]
24. Xu Y, Wang A, Lin X, Xu J, Shan Y, Pan X, et al. Global burden and gender disparity of vision loss associated with diabetes retinopathy. Acta Ophthalmol. 2021;99(4):431–40. doi:10.1111/aos.14644. [Google Scholar] [PubMed] [CrossRef]
25. Pena JS, Ramanujam RK, Risman RA, Tutwiler V, Berthiaume F, Vazquez M. Neurovascular relationships in AGEs-based models of proliferative diabetic retinopathy. Bioengineering. 2024;11(1):63. doi:10.3390/bioengineering11010063. [Google Scholar] [PubMed] [CrossRef]
Cite This Article
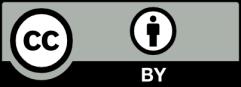
This work is licensed under a Creative Commons Attribution 4.0 International License , which permits unrestricted use, distribution, and reproduction in any medium, provided the original work is properly cited.