Open Access
ARTICLE
20-Hydroxyecdysone Partially Alleviates Ischemia/Reperfusion-Induced Damage of Mouse Hind Limb Skeletal Muscle
1 Department of Biochemistry, Cell Biology, and Microbiology, Mari State University, Yoshkar-Ola, 424001, Russia
2 Institute of Petrochemistry and Catalysis, Russian Academy of Sciences, Ufa, 450075, Russia
* Corresponding Author: Alena A. Semenova. Email:
(This article belongs to the Special Issue: Mitochondrial Dysfunction in Metabolic and Neuromuscular Diseases: Mechanisms and Therapeutic Strategies)
BIOCELL 2025, 49(3), 437-450. https://doi.org/10.32604/biocell.2025.061798
Received 03 December 2024; Accepted 20 February 2025; Issue published 31 March 2025
Abstract
Objectives: Skeletal muscle ischemia/reperfusion injury (IRI) occurs as a result of a marked reduction in arterial perfusion to a limb and can lead to tissue death and threaten limb viability. This work assessed the effects of 20-hydroxyecdysone (20E) on hindlimb skeletal tissue following tourniquet-induced ischemia/reperfusion injury. Methods: Animals were divided into 4 groups—control group (Control), Control + 20E (C + 20E), mice with IRI (IRI), and mice with IRI + 20E (IRI + 20E). IRI was modeled by applying a tourniquet to the hind limb for 2 h with reperfusion for 1 h. 5 mg/kg of 20E was administered intraperitoneally for 14 days. Afterward, the physical activity of mice, the histological structure of the quadriceps femoris, the expression of genes encoding proteins induced by hypoxia and involved in tissue adaptation to ischemia, and the functional parameters of skeletal muscle mitochondria were assessed. Results: It was shown that IRI of the limbs leads to functional disorders, depression of muscle function, accumulation of malondialdehyde (MDA) in mitochondria, and a decrease in their Ca2+ buffering capacity, as well as an increase in the expression of HIF-1α, VGEF-A, PGC1α and PDGF-BB genes associated with adaptation to ischemia. 20E reduced the intensity of degenerative processes in skeletal muscles, which was expressed in a decrease in the number of centrally nucleated fibers. Analysis of gene expression levels indicated a high degree of adaptation of animals to IRI. 20E reduced the level of MDA in mitochondria, but did not affect the rate of respiration and calcium retention capacity of organelles both in normal conditions and during IRI. Conclusion: 20E partially alleviates the skeletal muscle damage caused by IRI and can be used as part of combination therapy.Keywords
Acute limb ischemia continues to pose a significant challenge in clinical settings. After traumatic injuries or during vascular and orthopedic surgeries, blood circulation to the limbs is often disrupted or temporarily stopped, resulting in conditions like hypoperfusion or ischemia. This interruption can lead to ischemia/reperfusion injury (IRI), which may cause severe damage and even result in fatal outcomes [1,2].
Limb injury caused by ischemia/reperfusion may be related to both local and systemic proinflammatory reactions, which may adversely affect the outcome of the body [3–5]. Various medical conditions, such as the consequences of atherosclerosis, thrombosis, injury to large vessels of the limbs, arterial embolism, and surgical interventions (including vascular surgeries), can lead to ischemia/reperfusion injury of the limb [1,5]. The ischemic-reperfusion process of the limb can cause skeletal muscle dysfunction, edema, and necrosis, and it can also progress to structural damage and dysfunction of other organs like the brain, heart, lung, and small intestine [6]. Clinical research has become increasingly focused on protecting against limb ischemia/reperfusion injury. Various models of unilateral hindlimb ischemia are utilized, employing vascular clamps to occlude the femoral artery, a tourniquet, or an inflatable cuff wrapped around the thigh [5,7,8].
The tourniquet is also used in many surgical fields, such as traumatology, orthopedics, and vascular surgery. Several strategies have been developed in experimental studies to reduce ischemia/reperfusion injury, but only a few of them have been implemented in clinical practice. Considerable efforts have been made to develop various therapeutic strategies to prevent or reduce ischemia/reperfusion injury, including pharmacological and surgical approaches.
The disruption of mitochondrial respiration and the decreased expression of mitochondrial enzymes in the muscles of limbs affected by ischemia have been shown to play essential roles in the mechanisms that lead to the deterioration of skeletal muscle viability after ischemia/reperfusion injury [9,10]. As a result, energy production decreases and muscle function is limited, even after blood flow is restored. In this case, the issue of selecting a drug that promotes muscle tissue recovery after injury becomes relevant. Our study focused on 20-hydroxyecdysone (20E), an ecdysteroid hormone that regulates molting and reproduction in arthropods [11,12]. Ecdysteroids have a diverse array of physiological effects on mammals, including anti-inflammatory, hepatoprotective, immunomodulatory, and hypoglycemic properties [12–14]. In particular, 20E plays a role in regulating key metabolic pathways like protein synthesis, lipid metabolism, and carbohydrate metabolism. Ecdysteroids are particularly known in mammals for their anabolic properties, which resemble those of anabolic steroids but lack the androgenic side effects [15–17]. Anabolic effects of 20E, observed at a dosage of 5 mg/kg, include an enlargement of muscle fibers and a rise in myonuclei count within both normal and regenerating muscle tissue [15]. This makes it compelling to examine whether 20E administration has a therapeutic effect on muscle tissue after ischemia/reperfusion injury.
Male C57BL/10 mice were sourced from the Shemyakin and Ovchinnikov Institute of Bioorganic Chemistry, Russian Academy of Sciences (“Bio-model,” Pushchino, Russia). The animals were maintained under controlled conditions (22 ± 1°C, 12-h light-dark cycle) and provided with ad libitum access to food and water. The study with mice was in line with the European Convention for the Protection of Vertebrates used for experimental and other purposes (Strasbourg, 1986). The research protocol was approved by the Mari State University Ethics Committee (protocol No. 03/2023 of 15.12.2023). Eight-week-old wild-type C57BL/10 mice were randomly assigned to one of four groups for the in vivo study: Control (n = 9), Control + 20E (C + 20E, n = 9), hindlimb ischemia/reperfusion injury (IRI, n = 9), and IRI + 20E (n = 9). Mice received daily injections of 20E in sterile saline (5 mg/kg body weight) or sterile saline alone (control groups) for 14 days, consistent with prior research [15,18]. The 20E was obtained from Serratula coronata L. juice, using the extraction method described in [19].
To simulate ischemia, a tourniquet was applied to both hind limbs of the mouse in the proximal part of the femur for 2 h [8]. After this time, the tourniquet was removed. One hour after the commencement of reperfusion, the animals were administered appropriate doses of 20E, varying in volume from 100 to 150 μL, depending on the animal’s weight.
2.2 Grip Strength Test and Running Wheel Test
The grip strength test (IITC Life Science, Woodland Hills, CA, USA) was used to evaluate the muscular strength of the animals. Mice grasped a specialized mesh linked to a digital force transducer with all four paws, and the force exerted was measured in grams per unit of body weight. Three trials were conducted per mouse, and the average grip strength was calculated. In addition, a running wheel test was employed to assess endurance and motor activity. One week prior to testing, animals (n = 3 per group) were acclimated to the testing environment. During the testing phase, animals (n = 3 per group) were housed in a cage equipped with a running wheel (Ugo Basile, Varese, Italy) for 24 h. Each group underwent testing every two days throughout the study, and the average running distance over this 24-h period was recorded and analyzed.
The quadriceps muscle, obtained from six animals, was prepared for histological analysis. The muscle tissue was initially fixed in neutral buffered 10% formalin and subsequently embedded in paraffin. For examination, sections were prepared using a Minux S710 rotary microtome (RWD, Shenzhen, China) with a thickness of 5 μm. The sections underwent staining with hematoxylin and eosin following standard protocols. To visualize and analyze the stained sections, an EVOS M5000 imaging system (Thermo Fisher Scientific, Waltham, MA, USA) was utilized alongside ImageJ software version 1.53 (National Institutes of Health, Bethesda, MD, USA). The sections were observed under a microscope, and centrally nucleated fibers (CNF) were counted across 8 to 10 distinct fields of view at a magnification of 20 . The results were expressed as a percentage of the total myofibers counted. Additionally, the extent of fibrosis in the muscle tissues of the mice was evaluated by calculating the area stained with Sirius Red (GC307014, Servicebio, Wuhan, China), which indicates connective tissue components (including collagen), relative to the total tissue area on the histological slides. This assessment involved analyzing at least 10 sections for each sample.
2.4 RNA Extraction, Reverse Transcription, and Quantitative RT-PCR
Quadriceps muscle samples (100 mg, deep-frozen, n = 6 per group, from separate animals) were used for total RNA extraction with an ExtractRNA kit (#BC032, Eurogen, Moscow, Russia). Real-time PCR was then carried out using the qPCRmix-HS SYBR reaction mixture (Eurogen, PK147L, Moscow, Russia) and a DTLite5 amplifier (DNA-Technologies LLC, Moscow, Russia). Primer-BLAST was employed for the selection and in silico analysis of gene-specific primers [20]. Relative gene expression was quantified using the comparative CT method [21], with Rplp2 as the reference gene for normalization. The following types of DNA primers were used: HIF-1α forward (fw), 5′-GGCAGCGATGACACAGAAAC-3′; HIF-1α reverse (rv), 3′-GCAGGATCAGCACTACTTCG-5′; VEGF-A fw, 5′-CCTGGAGCGTTCACTGTGAG-3′; VEGF-A rv, 3′-CCTTGGCTTGTCACATCTGC-5′; Ppargc1a fw, 5′-CTGCCATTGTTAAGACCGAG-3′; Ppargc1a rv, 3′-GTGTGAGGAGGAGGTCCGTT-5′; PDGF-BB fw, 5′-CCGGTCCAGGTGAGAAAGA-3′; PDGF-BB rv, 3′-CCGAGGGGTCACTACTGTCT-5′; Rplp2 fw, 5′-CGGCTCAACAAGGTCATCAGTGA-3′; Rplp2 rv, 3′-AGCAGAAACAGCCACACCCCCAC-5′.
2.5 Evaluation of the Integrity of Cell Membranes and the Intensity of the Inflammation
The extent of the inflammatory response and the structural integrity of muscle tissue was evaluated by measuring the levels of creatine kinase (CK) and lactate dehydrogenase (LDH) in the mice’s blood serum using Olvex Diagnosticum kits (Olvex Diagnosticum, 028.003, 023.002, Saint Petersburg, Russia).
2.6 Isolation of Mouse Skeletal Muscle Mitochondria and Functional Studies
The extraction of mitochondria from skeletal muscle was performed following a standardized protocol [22]. The Bradford assay was used to determine the concentration of mitochondrial protein. Mitochondrial respiration was assessed via polarography using an Oxygraph+ apparatus (Hansatech Instruments, King’s Lynn, UK), maintaining a temperature of 25°C and continuous stirring, as previously reported [22,23]. Analysis of mitochondrial Ca2+ transport followed the methods described in [23]. The extent of lipid peroxidation in mitochondria was determined using a commercial kit (Agat-Med, 411212, Moscow, Russia) that quantifies TBA-reactive products, primarily malondialdehyde (MDA).
2.7 Statistical Processing of Results
The findings are reported as mean values accompanied by the standard error of the mean (m ± SEM). A one-way ANOVA followed by Tukey’s post-hoc test was executed with GraphPad Prism software version 8.0.1 for Windows (GraphPad Software Inc., La Jolla, CA, USA). Differences among groups were deemed to be significant at p < 0.05.
3.1 Effect of 20E on the Muscle Strength and Motor Activity of Mice
Limb compression ischemia leads to skeletal muscle dysfunction and impaired limb function [9]. Data [18] indicate the ability of 20E to exert multiple physiological effects in mammals, including anabolic effects and increased physical performance. In our work, it was shown that mice with IRI demonstrated a reliable decrease in the magnitude of muscle strength in the grip strength test compared to control animals by 1.5 times (Fig. 1A), as well as a significant decrease in motor activity (Fig. 1B). Administration of 20E to mice did not affect the strength of the mice, but a tendency towards improved endurance can be noted in the IRI + 20E group.
Figure 1: Impact of 20E on muscle strength and motor activity in mice. (A) Grip strength test results were recorded using a mesh that required the animal to use all four paws. Data are displayed as means ± SEM (n = 9). (B) The average distance traveled (in km) over a 24-h period by three mice is presented. Data are displayed as means ± SEM (n = 3, where n represents the number of cages containing animals)
The disruption of tissue blood supply and muscle damage that occurs during ischemia-reperfusion results in partial atrophy and a reduction in muscle mass, which also contributes to the observed decrease in muscle strength in animals [3,24]. Animals were weighed once every 3 days and average values in the group were compared. Weight loss in the IRI group was shown only for day 4 of the experiment, after which no differences were observed. (Fig. 2). It is noteworthy that 20-hydroxyecdysone has been demonstrated to possess anabolic effects on muscle tissues [15,16]. However, the weight of animals did not increase in the groups receiving 20E. Furthermore, the anabolic effect of 20E is not significant in healthy animals, as evidenced by several studies [15,16,25,26]. However, it has been observed to have a notable impact on certain pathological conditions.
Figure 2: Body weight fluctuations in the different experimental animal groups. Data are displayed as means ± SEM (n = 9). *p < 0.05
3.2 Effect of 20Е on the Histological Structure of the Quadriceps
We assessed the level of fibrosis and the occurrence of degeneration/regeneration cycles in the quadriceps muscle. Quadriceps samples from IRI mice revealed a marked increase in muscle fibers with centrally located nuclei compared to control animals (Fig. 3A–D,I). Centrally positioned nuclei in muscle fibers signify recovery, and a higher count of these nuclei correlates with intensified degeneration and regeneration cycles. In the IRI + 20E group, treatment with 20-hydroxyecdysone during ischemia led to a statistically significant decrease in the number of muscle fibers containing centrally located nuclei, indicating a reduction in the intensity of degenerative processes within skeletal muscles.
Figure 3: The histological characteristics of quadriceps skeletal muscle tissue from each mouse group. Myofibers with centrally located nuclei (A–D) and fibrotic areas (E–H, indicated by black arrows) are visible in the Control (A, E), C + 20E (B, F), IRI (C, G), and IRI + 20E (D, H) groups. The percentage of centrally nucleated fibers and the extent of interstitial fibrosis in the quadriceps of the experimental animals are quantified in the diagrams (I, J). Data are displayed as means ± SEM (n = 6)
We conducted a comparison of the progression of fibrosis in skeletal muscles in all experimental groups (Fig. 3E–H). The IRI group showed a tendency towards an increase in the area of connective tissue (Fig. 3J). This may indicate replacement of damaged tissue with fibrous connective tissue. 20E did not affect the level of fibrosis in the tissue.
3.3 Effect of 20E on Gene Expression of Hypoxia-Inducible Proteins Associated with Tissue Adaptation to Ischemia
Ischemia/reperfusion injury is associated with changes in gene expression, particularly those encoding proteins responsible for cell adaptation to hypoxia (HIF-1α) [27–29]. We examined the gene expression levels of proteins whose expression is induced by increased HIF-1α. These are genes for proteins responsible for angiogenesis (VGEF-A), mitochondrial biogenesis (PGC1α) [4,26], and platelet-derived growth factor (PDGF-BB). IRI led to elevated expression of HIF-1α (Fig. 4A). Ischemia also resulted in increased expression of VGEF-A and PDGF-BB genes (Fig. 4B,C). Additionally, the expression level of PGC1α, a gene involved in mitochondrial biogenesis, was increased (Fig. 4D). In IRI mice, 20E administration tended to gradually decrease the expression of these genes (except for HIF-1α), whereas in healthy mice, 20E treatment conversely increased their expression.
Figure 4: The effect of 20E on the relative levels of mRNA for HIF1α (A), VEGF-A (B), PDGF-BB (C), and PGC-1α (D) in the quadriceps muscle tissue of experimental animals. Data are displayed as means ± SEM (n = 9)
3.4 Assessment of the Integrity of Cell Membranes and the Intensity of the Inflammation in Skeletal Muscles
Ischemia/reperfusion injury is accompanied by an inflammatory process in the damaged tissue, which may be reflected by biochemical parameters. In this work, the intensity of the inflammatory process was assessed by the level of enzyme markers: creatine kinase (CK) and lactate dehydrogenase (LDH) in the blood serum of experimental groups of animals. It is known that CK and LDH are intracellular enzymes, therefore, an increase in their activity in the blood serum indicates damage to the cell membranes of muscle tissues [30]. In the IRI + 20E group, creatine kinase activity increased compared to control mice, indicating the presence of inflammatory processes in muscle tissue, but these differences were not statistically significant (Fig. 5A). At the same time, administration of 20E to mice showed a trend toward decreased CK activity. LDH activity was not statistically altered during ischemic injury (Fig. 5B).
Figure 5: Quantifying inflammatory enzyme activity in the serum of experimental animals (U/L) (A) Creatine kinase activity in the blood serum of the experimental group of animals. Data are displayed as means ± SEM (n = 9). (B) Measurement of lactate dehydrogenase activity in the blood serum of the experimental animals. Data are displayed as means ± SEM (n = 9)
3.5 The Effect of 20E on the Function of the Mitochondria in Skeletal Muscles of Mice
IRI is known to suppress mitochondrial oxidative phosphorylation and disrupt proper mitochondrial function [9]. Ischemia interferes with the mitochondrial electron transport chain, leading to a decline in ATP synthesis efficiency [9,31]. Respiration and oxidative phosphorylation parameters in quadriceps mitochondria are summarized in Table 1. Our experiment demonstrated a significant reduction in the rate of uncoupled respiration at state 3UDNP (maximum respiratory rate) in the IRI and IRI + 20E groups. In addition, we found a lower respiration rate at state 4 in the IRI + 20E group. However, respiration rates in states 2 and 3, the respiratory control (RC) ratio, and the adenosine diphosphate/oxygen (ADP/O) ratio, which reflect oxidative phosphorylation efficiency, were similar to the control group. Furthermore, 20E did not affect any of these parameters.
Ischemia and ischemia/reperfusion are characterized by an increase in free radical oxidation processes within cells and mitochondria, resulting in elevated reactive oxygen species (ROS) production, increased cytosolic Ca2+ levels, and induction of the opening of the mitochondrial permeability transition pore (MPT pore), which ultimately leads to cell death [31,32]. Reduced resistance of mitochondria to MPT pore opening correlates with a decrease in their calcium retention capacity. This capacity is defined as the maximum amount of Ca2+ that the mitochondrial matrix can accumulate before the MPT pore opens. We also assessed the ability of skeletal muscle mitochondria to take up and retain calcium ions in the matrix. In the IRI group, the calcium retention capacity of mitochondria decreased, and this was especially pronounced in mitochondria fueled by glutamate and malate (Fig. 6A,C). Succinate-energized mitochondria exhibited significantly higher calcium retention capacity, which is well-known and related to the rate of electron flow through complex I [33]. At the same time, we did not note any changes in the level of calcium retention capacity of the mitochondria of the studied groups of animals energized with succinate (Fig. 6B,D).
Figure 6: The impact of 20E on the ability of mitochondria to maintain Ca2+ homeostasis. Ca2+ uptake (using 20 μM pulses) by skeletal muscle mitochondria energized with glutamate/malate (A) or succinate (B). The capacity of isolated quadriceps mitochondria to retain calcium when fueled by glutamate/malate (C) or succinate (D). Data are displayed as means ± SEM (n = 5). Panels A and B depict representative data from a single mitochondrial preparation. Comparable results were observed in 7 additional independent experiments
IRI is associated with elevated levels of reactive oxygen species and the development of oxidative stress [32,34], which can be assessed by measuring malondialdehyde (MDA), an end product of lipid peroxidation, in skeletal muscle mitochondria. We observed increased MDA levels in the mitochondria of IRI group mice compared to controls (Fig. 7). Treatment with 20E significantly reduced the concentration of malondialdehyde in skeletal muscle mitochondria of the IRI + 20E group to levels comparable to the control group. This finding aligns with existing literature suggesting that 20E has antioxidant properties [12,13,35].
Figure 7: The concentration of MDA products in the isolated mitochondria. Data are displayed as means ± SEM (n = 5) (TBARS—thiobarbituric acid reactive substances, mainly MDA)
Ischemia/reperfusion injury is a subject of clinical and basic research [3–5]. IRI is a condition that occurs when tissue regains blood supply after a period of ischemia. It is a consequence of acute inflammation, which is triggered by a variety of processes. IRI can manifest in a spectrum of scenarios, from minor wounds to severe trauma. Cellular IRI can result in the loss of organ or limb function. Numerous factors impact the outcomes of these injuries, highlighting the importance for clinicians to understand IRI to reduce morbidity and its sequelae [6]. While various strategies have been explored in experimental settings to mitigate IRI, few have translated into clinical applications. Among pharmacological options, 20-hydroxyecdysone is a promising research candidate. Literature suggests it possesses anti-inflammatory and anabolic effects comparable to anabolic steroids but without the associated androgenic effects [15,16,18]. Furthermore, it has been reported to enhance physical performance and overall endurance in laboratory animals [18]. In our study, we also examined the effect of 20E on muscle strength in mice using a grip strength test. Although we didn’t observe a statistically significant increase in strength, there was a trend toward improvement. We also did not observe any anabolic effect on the muscles and weight of animals in our work. While numerous studies have confirmed the anabolic effects of 20E, its impact on body weight and muscle mass has shown variability [15,18,25]. These inconsistencies are likely attributable to variations in the animal strains utilized in the research, in addition to differences in the routes and methods employed for the administration of 20E. 20E is rapidly eliminated by various pathways, and the half-life of this compound in rodents is short [16]. This may explain the differences in the effects of 20E on muscle mass in animals in different experiments. In addition, the literature indicates [25] that when 20E is administered intraperitoneally, the drug is metabolized differently than transformation through the gastrointestinal tract following oral administration. At the same time, the physiological effects of the metabolites of 20E on skeletal muscles have not been fully understood. However, large doses of poststerone, a major metabolite of 20E, have been shown to increase the cross-sectional area of muscle fibers following subcutaneous injection adjacent to the muscle [36]. Given these data, further research is required to determine the optimal dose and duration of 20E administration, as well as to determine the plasma concentration of 20E throughout the experiment. Histological changes in ischemia/reperfusion injury indicate an increase in the number of muscle fibers containing centrally located nuclei compared to control animals, and the introduction of 20-hydroxyecdysone in the experiment had a positive effect and reduced this parameter. As is known, the presence of centrally located nuclei in muscle fibers is a marker of their recovery, and an increase in the content of such fibers in muscle tissue is associated with an increase in the intensity of the cycles of degeneration and regeneration of muscle fibers [37]. Thus, 20E helps to reduce the intensity of degenerative processes in skeletal muscles, which may be associated with the anti-inflammatory and anabolic effects of 20E described in the literature [12,15,18]. The level of fibrosis in skeletal tissues showed virtually no differences between the samples of the different groups, however, small areas of connective tissue were observed in the IRI group. Some literature sources indicate that despite the progressive nature of histological changes in IRI, it may be reversible since ultrastructural signs of recovery (regeneration phenomenon) were observed after 72 h of reperfusion [32,37]. This recovery may be partly due to the low levels of oxidative stress, as well as the two-week duration of the experiment, which was likely sufficient for recovery.
It is known that the ischemic stimulus implements its action through genomic and non-genomic pathways [6]. However, such a division is very arbitrary and indicates a deep relationship between these two pathways. In our work, we investigated the levels of gene expression that encode proteins involved in cell adaptation to hypoxia (HIF-1α), angiogenesis (VGEF-A), mitochondrial biogenesis (PGC1α), and platelet-derived growth factor (PDGF-BB). We found an increase in HIF-1α gene expression during skeletal muscle ischemia-reperfusion, which was expected since HIF-1α is known to promote long-term adaptive responses to hypoxia [4]. The expression levels of VGEF-A and PDGF-BB genes also increase during ischemia, and this is associated with increased expression of HIF-1α and the property of this factor to trigger compensatory angiogenesis [4,27]. A rise in the level of expression of the PGC1α gene, which is responsible for organelle biogenesis, is associated with an increased need for energy in cells and can also be considered as a mechanism for cell adaptation to damage [31]. Administration of 20-hydroxyecdysone to mice did not affect the expression levels of the indicated genes, however, a tendency towards a gradual decrease, and in healthy mice, on the contrary, towards an increase in the expression of the indicated genes (with the exception of HIF-1α) can be noted. This effect may be due to the adaptogenic effect of 20-hydroxyecdysone, which also promotes better tissue recovery after damage.
The physiological, biochemical, and immunological shifts occurring during ischemia and reperfusion cause an intense inflammatory response in IRI, resulting in local and systemic consequences. We assessed the intensity of inflammatory processes based on the level of enzyme markers and did not find significant changes in the activity of CK and LDH. On the one hand, this may indicate a mild inflammatory process, characteristic of this model. On the other hand, the adaptive mechanisms that develop over two weeks could lead to normalization of the enzyme activity level.
Mitochondrial damage plays a critical role in the decline of skeletal muscle function following IRI. This damage leads to compromised mitochondrial respiration and a decrease in mitochondrial enzyme expression in the muscles of ischemic limbs in IRI patients, ultimately resulting in diminished energy production and restricted muscle function, as demonstrated in various studies [9,30,31]. In our work, we did not observe significant mitochondrial dysfunction as a result of IRI. In particular, the respiratory and oxidative phosphorylation parameters of mitochondria in IRI group mice were largely unchanged compared to the control group. The only exception was a decreased rate of uncoupled respiration, potentially indicating a slight reduction in the maximum electron transport rate along the mitochondrial respiratory chain. However, the respiratory control ratio of skeletal muscle mitochondria remained unaffected by IRI. It can be assumed that this is due to the duration of the experiment. Since IRI is not a chronic pathology, therefore, within 14 days, defective mitochondria (damaged during ischemia-reperfusion) could be eliminated from the body through mitophagy processes. At the same time, 20E had no impact on the examined characteristics of organelle respiration. Furthermore, it is evident that the mitochondria of animals in the IRI group exhibit elevated levels of MDA and diminished capacity for calcium retention. This may be attributed to the impact of general oxidative stress on mitochondrial function in damaged muscle tissue, even after a period of 14 days following muscle damage caused by IRI. In this case, we did not observe the effect of 20-hydroxyecdysone on mitochondrial respiration and calcium retention capacity. However, 20E therapy was effective in reducing malondialdehyde levels in the mitochondria of mice with ischemia/reperfusion injury to control group values. This may indicate that the administration of 20-hydroxyecdysone triggers antioxidant defense mechanisms in the skeletal muscles, but they are apparently not associated with a direct effect of this agent on mitochondrial function. However, additional experiments are needed to evaluate mitochondrial function and the effect of 20E on it immediately after IRI, which induces mitochondrial dysfunction in the first days. This will allow us to clarify whether the therapeutic effects of ecdysterone are related to its direct effect on mitochondria, as shown in other studies [38,39].
The findings suggest that 20-hydroxyecdysone assists in mitigating the adverse consequences of ischemia. However, further research is also required to determine the dose-response relationship of 20E in various IRI models. In particular, Y. Kuroda showed [30] that the degree of damage is highly dependent on time, and with 1-h reperfusion, changes are observed to a lesser extent than with 3-h reperfusion.
It has been shown that ischemia/reperfusion injuries of the limbs lead to functional impairments, suppressed muscle function, the accumulation of MDA in the mitochondria, and a decrease in the calcium retention capacity of the organelles, and also increased the expression of genes encoding hypoxia-induced proteins associated with tissue adaptation to ischemia (HIF-1α, VGEF-A, PGC1α, and PDGF-BB). The use of 20E resulted in a decrease in the intensity of degenerative changes in skeletal muscles, and a decrease in the level of MDA in mitochondria and was accompanied by a tendency to improve muscle strength in animals. It should be noted that the results of the analysis of the level of protein gene expression indicate a high degree of adaptation of animals to ischemia. This probably caused the absence of pronounced inflammatory processes in mice with ischemia/reperfusion injury of skeletal muscle tissue.
Acknowledgement: None.
Funding Statement: This work was supported by a grant from the Russian Science Foundation (23-75-01061, https://rscf.ru/project/23-75-01061/) (accessed on 19 February 2025).
Author Contributions: The authors confirm their contribution to the article as follows: study concept and design: Alena A. Semenova; data collection: Alena A. Semenova, Anastasia D. Igoshkina, Alena A. Cherepanova, Natalia V. Mikina, Anastasia E. Stepanova, Olga E. Krasnoshchekova, Vyacheslav A. Sharapov, Mikhail V. Dubinin, Rimma G. Savchenko, Lyudmila V. Parfenova; analysis and interpretation of results: Alena A. Semenova, Mikhail V. Dubinin; manuscript drafting: Alena A. Semenova. All authors reviewed the results and approved the final version of the manuscript.
Availability of Data and Materials: The datasets generated and analyzed during the current study are available from the corresponding author upon reasonable request.
Ethics Approval: The study with mice was in line with the European Convention for the Protection of Vertebrates used for experimental and other purposes (Strasbourg, 1986). The research protocol was approved by the Mari State University Ethics Committee (protocol No. 03/2023 of 15.12.2023).
Conflicts of Interest: The authors declare no conflicts of interest to report regarding the present study.
References
1. Olinic DM, Stanek A, Tataru DA, Homorodean C, Olinic M. Acute limb ischemia: an update on diagnosis and management. J Clin Med. 2019;8(8):1215. doi:10.3390/jcm8081215. [Google Scholar] [PubMed] [CrossRef]
2. Naito H, Nojima T, Fujisaki N, Tsukahara K, Yamamoto H, Yamada T, et al. Therapeutic strategies for ischemia reperfusion injury in emergency medicine. Acute Med Surg. 2020;7(1):e501. doi:10.1002/ams2.501. [Google Scholar] [PubMed] [CrossRef]
3. Gillani S, Cao J, Suzuki T, Hak DJ. The effect of ischemia reperfusion injury on skeletal muscle. Injury. 2012;43(6):670–5. doi:10.1016/j.injury.2011.03.008. [Google Scholar] [PubMed] [CrossRef]
4. Wang T, Zhou YT, Chen XN, Zhu AX. Putative role of ischemic postconditioning in a rat model of limb ischemia and reperfusion: involvement of hypoxia-inducible factor-1α expression. Braz J Med Biol Res. 2014;47(9):738–45. doi:10.1590/1414-431X20142910. [Google Scholar] [PubMed] [CrossRef]
5. Korei C, Szabo B, Varga A, Barath B, Deak A, Vanyolos E, et al. Hematological, micro-rheological, and metabolic changes modulated by local ischemic pre-and post-conditioning in rat limb ischemia-reperfusion. Metabolites. 2021;11(11):776. doi:10.3390/metabo11110776. [Google Scholar] [PubMed] [CrossRef]
6. Apichartpiyakul P, Shinlapawittayatorn K, Rerkasem K, Chattipakorn SC, Chattipakorn N. Mechanisms and interventions on acute lower limb ischemia/reperfusion injury: a review and insights from cell to clinical investigations. Ann Vasc Surg. 2022;86:452–81. doi:10.1016/j.avsg.2022.04.040. [Google Scholar] [PubMed] [CrossRef]
7. Rosero O, Nemeth K, Turoczi Z, Fulop A, Garbaisz D, Gyorffy A, et al. Collateral circulation of the rat lower limb and its significance in ischemia/reperfusion studies. Surg Today. 2014;44(12):2345–53. doi:10.1007/s00595-013-0822-z. [Google Scholar] [PubMed] [CrossRef]
8. Orfany A, Arriola CG, Doulamis IP, Guariento A, Ramirez-Barbieri G, Moskowitzova K, et al. Mitochondrial transplantation ameliorates acute limb ischemia. J Vasc Surg. 2020;71(3):1014–26. doi:10.1016/j.jvs.2019.03.079. [Google Scholar] [PubMed] [CrossRef]
9. Lejay A, Meyer A, Schlagowski AI, Charles AL, Singh F, Bouitbir J, et al. Mitochondria: mitochondrial participation in ischemia/reperfusion injury in skeletal muscle. Int J Biochem Cell Biol. 2014;50:101–5. doi:10.1016/j.biocel.2014.02.013. [Google Scholar] [PubMed] [CrossRef]
10. Chen TH, Koh KY, Lin KM, Chou CK. Mitochondrial dysfunction as an underlying cause of skeletal muscle disorders. Int J Mol Sci. 2022;23(21):12926. doi:10.3390/ijms232112926. [Google Scholar] [PubMed] [CrossRef]
11. Savchenko RG, Veskina NA, Odinokov VN, Benkovskaya GV, Parfenova LV. Ecdysteroids: isolation, chemical transformations, and biological activity. Phytochem Rev. 2022;21(5):1445–86. doi:10.1007/s11101-021-09792-y. [Google Scholar] [CrossRef]
12. Dinan L, Dioh W, Veillet S, Lafont R. 20-hydroxyecdysone, from plant extracts to clinical use: therapeutic potential for the treatment of neuromuscular, cardio-metabolic and respiratory diseases. Biomedicines. 2021;9(5):492. doi:10.3390/biomedicines9050492. [Google Scholar] [PubMed] [CrossRef]
13. Mallek A, Movassat J, Ameddah S, Liu J, Semiane N, Khalkhal A, et al. Experimental diabetes induced by streptozotocin in the desert gerbil, Gerbillus gerbillus, and the effects of short-term 20-hydroxyecdysone administration. Biomed Pharmacother. 2018;102:354–61. doi:10.1016/j.biopha.2018.03.070. [Google Scholar] [PubMed] [CrossRef]
14. Jin Z, Wang B, Ren L, Yang J, Zheng Z, Yao F, et al. 20-hydroxyecdysone inhibits inflammation via SIRT6-mediated NF-κB signaling in endothelial cells. Biochim Biophys Acta Mol Cell Res. 2023;1870(5):119460. doi:10.1016/j.bbamcr.2023.119460. [Google Scholar] [PubMed] [CrossRef]
15. Tóth N, Szabó A, Kacsala P, Héger J, Zádor E. 20-hydroxyecdysone increases fiber size in a muscle-specific fashion in rat. Phytomedicine. 2008;15(9):691–8. doi:10.1016/j.phymed.2008.04.015. [Google Scholar] [PubMed] [CrossRef]
16. Zwetsloot KA, Shanely RA, Godwin JS, Hodgman CF. Phytoecdysteroids accelerate recovery of skeletal muscle function following in vivo eccentric contraction-induced injury in adult and old mice. Front Rehabil Sci. 2021;2:757789. doi:10.3389/fresc.2021. [Google Scholar] [CrossRef]
17. Serova M, Didry-Barca B, Deloux R, Foucault AS, Veillet S, Lafont R, et al. BIO101 stimulates myoblast differentiation and improves muscle function in adult and old mice. J Cachexia Sarcopenia Muscle. 2024;15(1):55–66. doi:10.1002/jcsm.13326. [Google Scholar] [PubMed] [CrossRef]
18. Hirunsai M, Yimlamai T, Suksamrarn A. Effect of 20-hydroxyecdysone on proteolytic regulation in skeletal muscle atrophy. In Vivo. 2016;30(6):869–77. doi:10.21873/invivo.11007. [Google Scholar] [PubMed] [CrossRef]
19. Odinokov VN, Galyautdinov IV, Nedopekin DV, Khalilov LM, Shashkov AS, Kachala VV et al. Phytoecdysteroids from the juice of Serratula coronata L. (Asteraceae). Insect Biochem Mol Biol. 2002;32(2):161–5. doi:10.1016/s0965-1748(01)00106-0. [Google Scholar] [PubMed] [CrossRef]
20. Ye J, Coulouris G, Zaretskaya I, Cutcutache I, Rozen S, Madden TL. Primer-BLAST: a tool to design target-specific primers for polymerase chain reaction. BMC Bioinform. 2012;13:134. [Google Scholar]
21. Schmittgen TD, Livak KJ. Analyzing real-time PCR data by the comparative CT method. Nat Protoc. 2008;3:1101–8. [Google Scholar] [PubMed]
22. Dubinin MV, Starinets VS, Belosludtseva NV, Mikheeva IB, Chelyadnikova YA, Igoshkina AD, et al. BKCa activator NS1619 improves the structure and function of skeletal muscle mitochondria in duchenne dystrophy. Pharmaceutics. 2022;14(11):2336. doi:10.3390/pharmaceutics14112336. [Google Scholar] [PubMed] [CrossRef]
23. Dubinin MV, Talanov EY, Tenkov KS, Starinets VS, Mikheeva IB, Sharapov MG, et al. Duchenne muscular dystrophy is associated with the inhibition of calcium uniport in mitochondria and an increased sensitivity of the organelles to the calcium-induced permeability transition. Biochim Biophys Acta Mol Basis Dis. 2020;1866(5):165674. doi:10.1016/j.bbadis.2020.165674. [Google Scholar] [PubMed] [CrossRef]
24. Ferreira J, Carneiro A, Vila I, Silva C, Cunha C, Longatto-Filho A, et al. Inflammation and loss of skeletal muscle mass in chronic limb threatening ischemia. Ann Vasc Surg. 2023;88:164–73. doi:10.1016/j.avsg.2022.07.009. [Google Scholar] [PubMed] [CrossRef]
25. Lawrence MM, Zwetsloot KA, Arthur ST, Sherman CA, Huot JR, Badmaev V, et al. Phytoecdysteroids do not have anabolic effects in skeletal muscle in sedentary aging mice. Int J Environ Res Public Health. 2021;18(2):370. doi:10.3390/ijerph18020370. [Google Scholar] [PubMed] [CrossRef]
26. Das N, Mishra SK, Bishayee A, Ali ES, Bishayee A. The phytochemical, biological, and medicinal attributes of phytoecdysteroids: an updated review. Acta Pharm Sin B. 2021;11(7):1740–66. doi:10.1016/j.apsb.2020.10.012. [Google Scholar] [PubMed] [CrossRef]
27. Tarantul VZ, Gavrilenko AV. Gene therapy for critical limb ischemia: per aspera ad astra. Curr Gene Ther. 2022;22(3):214–27. doi:10.2174/1566523221666210712185742. [Google Scholar] [PubMed] [CrossRef]
28. Loor G, Schumacker PT. Role of hypoxia-inducible factor in cell survival during myocardial ischemia-reperfusion. Cell Death Differ. 2008;15(4):686–90. doi:10.1038/cdd.2008.13. [Google Scholar] [PubMed] [CrossRef]
29. Kalakech H, Tamareille S, Pons S, Godin-Ribuot D, Carmeliet P, Furber A, et al. Role of hypoxia inducible factor-1α in remote limb ischemic preconditioning. J Mol Cell Cardiol. 2013;65:98–104. doi:10.1016/j.yjmcc.2013.10.001. [Google Scholar] [PubMed] [CrossRef]
30. Kuroda Y, Togashi H, Uchida T, Haga K, Yamashita A, Sadahiro M. Oxidative stress evaluation of skeletal muscle in ischemia/reperfusion injury using enhanced magnetic resonance imaging. Sci Rep. 2020;10(1):10863. doi:10.1038/s41598-020-67336-4. [Google Scholar] [PubMed] [CrossRef]
31. Anzell AR, Maizy R, Przyklenk K, Sanderson TH. Mitochondrial quality control and disease: insights into ischemia-reperfusion injury. Mol Neurobiol. 2018;55(3):2547–64. doi:10.1007/s12035-017-0503-9. [Google Scholar] [PubMed] [CrossRef]
32. Paradis S, Charles AL, Meyer A, Lejay A, Scholey JW, Chakfé N, et al. Chronology of mitochondrial and cellular events during skeletal muscle ischemia-reperfusion. Am J Physiol Cell Physiol. 2016;310(11):C968–82. doi:10.1152/ajpcell.00356.2015. [Google Scholar] [PubMed] [CrossRef]
33. Fontaine E, Eriksson O, Ichas F, Bernardi P. Regulation of the permeability transition pore in skeletal muscle mitochondria: modulation by electron flow through the respiratory chain complex I. J Biol Chem. 1998;273(20):12662–8. doi:10.1074/jbc.273.20.12662. [Google Scholar] [PubMed] [CrossRef]
34. Zeng J, Liu J, Ni H, Zhang L, Wang J, Li Y, et al. Mitochondrial transplantation reduces lower limb ischemia/reperfusion injury by increasing skeletal muscle energy and adipocyte browning. Mol Ther Methods Clin Dev. 2023;31:101152. doi:10.1016/j.omtm.2023.101152. [Google Scholar] [PubMed] [CrossRef]
35. Sun J, Zhao K, Zhang W, Guo C, Liu H. Ecdysterone improves oxidative damage induced by acute ischemic stroke via inhibiting ferroptosis in neurons through ACSL4. J Ethnopharmacol. 2024;331:118204. doi:10.1016/j.jep.2024.118204. [Google Scholar] [PubMed] [CrossRef]
36. Csábi J, Rafai T, Hunyadi A, Zádor E. Poststerone increases muscle fibre size partly similar to its metabolically parent compound, 20-hydroxyecdysone. Fitoterapia. 2019;134(1):459–64. doi:10.1016/j.fitote.2019.03.017. [Google Scholar] [PubMed] [CrossRef]
37. Carmo-Araújo EM, Dal-Pai-Silva M, Dal-Pai V, Cecchini R, Anjos Ferreira AL. Ischaemia and reperfusion effects on skeletal muscle tissue: morphological and histochemical studies. Int J Exp Pathol. 2007;88(3):147–54. doi:10.1111/j.1365-2613.2007.00526.x. [Google Scholar] [PubMed] [CrossRef]
38. Todorova V, Ivanova S, Chakarov D, Kraev K, Ivanov K. Ecdysterone and turkesterone-compounds with prominent potential in sport and healthy nutrition. Nutrients. 2024;16(9):1382. doi:10.3390/nu16091382. [Google Scholar] [PubMed] [CrossRef]
39. Baev AY, Charishnikova OS, Khasanov FA, Nebesnaya KS, Makhmudov AR, Rakhmedova MT, et al. Ecdysterone prevents negative effect of acute immobilization stress on energy metabolism of rat liver mitochondria. J Steroid Biochem Mol Biol. 2022;219:106066. doi:10.1016/j.jsbmb.2022.106066. [Google Scholar] [PubMed] [CrossRef]
Cite This Article
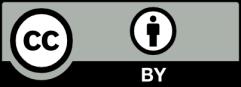
This work is licensed under a Creative Commons Attribution 4.0 International License , which permits unrestricted use, distribution, and reproduction in any medium, provided the original work is properly cited.