Open Access
REVIEW
The Impact of Exercise during Pregnancy on Maternal and Offspring Outcomes in Gestational Diabetes Mellitus
1 Department of Obstetrics and Gynecology, Wuxi Maternal and Child Health Hospital, Wuxi School of Medicine, Jiangnan University, Wuxi, 214002, China
2 Department of Endocrinology, Affiliated Children’s Hospital of Jiangnan University Wuxi Children’s Hospital, Wuxi, 214023, China
* Corresponding Authors: Kerong Liu. Email: ; Ying Gu. Email:
# These authors contributed equally to this work
BIOCELL 2025, 49(2), 181-198. https://doi.org/10.32604/biocell.2025.058745
Received 19 September 2024; Accepted 11 December 2024; Issue published 28 February 2025
Abstract
The increasing prevalence of gestational diabetes mellitus (GDM) is associated with an array of pregnancy complications and enduring health challenges in both mothers and their offspring. Studies have indicated that exposure to the intrauterine environment can prompt adaptations in the offspring, thereby programming transgenerational inheritance. Physical activity during pregnancy, as a non-pharmacological intervention, mitigates metabolic risks through epigenetic modifications, mediating placental adaptations, the action of exercise factors, and gut microbiota. Here, we provide a review summarizing how regular exercise can reduce the risk of GDM and positively influence pregnancy outcomes. It also discusses the exercise-induced reduction in obesity, insulin resistance, and fatty liver disease in the offspring of GDM, outlining the mechanisms that underpin these health benefits. This review underscores the importance of exercise in safeguarding the health of GDM mothers and their offspring.Keywords
Chronic metabolic diseases such as obesity, hypertension, and diabetes are rampant worldwide [1]. Gestational diabetes mellitus (GDM) is one of the most common metabolic disorders during pregnancy and is defined as abnormal glucose tolerance that occurs or is first diagnosed during pregnancy, with a global prevalence ranging from 1% to 14% [2]. Recognized risk factors for GDM include overweight or obesity, excessive weight gain during pregnancy, family history of GDM or type 2 diabetes, multiparity, and advanced maternal age [3]. Although the exact etiology remains unclear, inflammation, insulin resistance, and oxidative stress are considered key factors in the occurrence and progression of GDM [4].
GDM is associated not only with an increased risk of adverse pregnancy outcomes during the perinatal period, including large-for-gestational-age (LGA) infants, macrosomia, neonatal hypoglycemia, and elevated perinatal morbidity and mortality but also with heightened long-term metabolic risk for both mothers and their offspring. In pregnancies affected by GDM, excessive fetal growth primarily occurs in the abdominal and shoulder regions. This results in macrosomia in 15% to 45% of cases, which elevates the likelihood of shoulder dystocia and injuries during birth [5]. A recent systematic review based on the diagnostic criteria of the Oral Glucose Tolerance Test (OGTT) indicates that the incidence of large LGA infants among pregnant women with GDM is 13%, compared to the overall prevalence of LGA (18%) [6]. Research suggests that a significant proportion of women, up to 70%, who experience GDM are likely to develop type 2 diabetes mellitus (T2DM) within a period of 22 to 28 years. However, this risk may be reduced through modifications in diet and lifestyle [7]. Both mothers with GDM and their offspring have a higher risk of metabolic diseases later in life [8]. Pregnant women with GDM have a twofold increased risk of developing cardiovascular diseases in the future, compared to healthy ones [9]. Additionally, maternal GDM, gestational hypertensive disorders, or a combination of both, increase the risk of obesity in offspring [10]. Furthermore, An International and epidemiological study: Hyperglycemia and Adverse Pregnancy Outcome (HAPO) significantly found a positive correlation between maternal blood glucose levels and in obesity children aged 10–14 years [11]. It is currently believed that hyperglycemia during pregnancy is a risk factor for elevated blood pressure in the offspring, and prenatal interventions targeting gestational hyperglycemia may help control blood pressure in children [12]. Therefore, there is an urgent need for an effective intervention that can improve glycemic control in mothers with GDM and enhance maternal and fetal clinical outcomes, while also reducing health risks for the offspring.
Nutritional therapy along with physical activity is currently the first-line treatment for GDM. Studies show that for 70%–85% of women diagnosed with GDM, changes in lifestyle alone are enough to manage blood sugar levels. However, the formulation of dietary plans for women with GDM remains a complex issue that has not been fully resolved [13]. Observational studies of women engaging in exercise during pregnancy suggest that physical activity offers benefits such as reduced risks of gestational diabetes, cesarean delivery, and longer postpartum recovery times. Physical activity and exercise during pregnancy carry minimal risks and have been shown to be beneficial for most women [14]. Self-care is crucial in the management of women with GDM, especially in the aspects of physical activity, and blood glucose control [15]. A systematic review conducted by Karavasileiadou et al. demonstrated that a certain level of self-management and self-efficacy enables women to exert specific control over their diet and weight, which not only optimizes the management of GDM but also prevents postpartum diabetes [16]. A study conducted in China identified a positive correlation between self-efficacy and self-management behaviors in pregnant women with GDM [17]. A cross-sectional study conducted in Jordan found that mothers with GDM possessing higher levels of self-efficacy acquired higher standards of self-care [18]. This review discusses the effects of exercise during pregnancy on glycemic control in mothers with GDM under insulin-resistant conditions as well as its impact on offspring health and the underlying mechanisms. The aim was to enhance the self-care and compliance of women with GDM in exercise interventions, thereby providing optimal benefits for both mothers and their offspring.
2 Forms of Exercise during Pregnancy
Physical activity (PA) during pregnancy is a crucial component of prenatal care that promotes both maternal and fetal health. Exercise can be categorized into aerobic and resistance training, with aerobic exercise being particularly beneficial for maintaining fitness and overall well-being [19]. The aerobic exercises recommended for pregnant women include walking, stationary cycling, aerobic dance, and aquatic exercise [14]. The study found reduced maternal cesarean section rates and fetal shoulder injuries in patients who regularly walked for 30–60 min at least 1 day per week throughout pregnancy [20]. Similarly, a randomized controlled trial by Adamczak et al. demonstrated significantly less macrosomia in patients with GDM and obese pregnancies after pedometer supervision [21]. Pregnant women can benefit from stationary cycling as an alternative form of aerobic exercise that engages both the cardiovascular system and skeletal muscles [22]. A randomized crossover controlled study by Andersen et al. revealed that pregnant women at risk of GDM experienced reduced glycemic fluctuations after glucose intake when they participated in moderate-intensity cycling for 20 min [23]. Additionally, a study by Halse et al. found that compared to treadmill walking, stationary cycling resulted in notably lower postprandial blood glucose levels at 120 min following an OGTT, as well as decreased insulin levels 120 min after glucose consumption [24]. A recent meta-analysis of various exercise modalities for the prevention of prenatal depression by Ji et al. demonstrated that the preventive effects of efficacious stress interventions were, in descending order, yoga, and aerobics combined with resistance training [25]. Furthermore, a study conducted in Turkey revealed that pregnant women who participated in a 60 min prenatal yoga class once a week for 4 weeks experienced significant reductions in pregnancy-related gastrointestinal, respiratory, and mental health symptoms [26]. Dance has also been demonstrated to elicit positive feelings and emotions regarding pregnancy [27]. Aquatic exercise is a form of physical activity that exhibits a high level of participation among women during pregnancy. A multicenter randomized controlled trial conducted in Spain demonstrated that moderate-intensity aquatic exercise during pregnancy reduced postpartum anxiety and depressive symptoms in mothers [28]. Cancela-Carral et al. also indicated that aquatic exercise in pregnant women had a positive effect on the prevention of excessive maternal weight gain and on the reduction of the mean difference in neonatal birth weight [29]. Animal studies have shown that maternal swimming influences mitochondrial responses to hypoxia in the brain of the offspring enhances spatial memory, and prevents cognitive impairment, with male rats showing the most notable improvements [30]. However, there is a paucity of literature exploring the mechanisms of various types of aerobic exercise at present. The American College of Sports Medicine (ACSM) outlines the FITT (Frequency, Intensity, Type, and Time) principles for aerobic exercise prescriptions, which are also applicable to pregnant women [31,32]. International guidelines generally suggest that pregnant women engage in moderate-intensity aerobic exercise for at least 150 min per week, spread over at least three days [33]. The American College of Obstetricians and Gynecologists (ACOG) specifically advises women with GDM to exercise for at least 150 min per week [34], while the Society of Obstetricians and Gynecologists of Canada (SOGC) and The Canadian Society for Exercise Physiology (CSEP) recommend a similar regimen for all pregnant women without contraindications [35].
Exercise intensity should be moderate, corresponding to a metabolic equivalent of task (MET) of 3–4, such as brisk walking. Most guidelines recommend aiming for a heart rate reserve (HRR) of 40%–59% during exercise, with vigorous exercise (heart rates exceeding 90% of HRR) to be avoided [36]. The Rating of Perceived Exertion (RPE) scale, also known as the Borg scale, is a useful tool for self-assessment, with pregnant women advised to target an RPE of 13–14, indicating a “somewhat difficult” level of exertion [37].
The “talk test” is another simple method to gauge exercise intensity; if a woman can converse comfortably during exercise, she is likely within the appropriate intensity range. It is important to note that individual responses to exercise can vary, and pregnant women should consult their healthcare providers to determine the most suitable exercise regimen based on their specific health conditions and fitness levels [14].
3 Maternal Exercise Effects on the Health of Mothers
Exercise is beneficial for women with GDM, as it can improve blood glucose control in GDM patients and reduce key risk factors associated with GDM, such as maternal hypertension, preeclampsia, and birth injuries related to macrosomia, especially for controlling blood glucose and insulin levels [13]. Research by Davenport et al. revealed that pregnant women who engaged in exercise experienced a 39% lower risk of developing GDM and a 41% decrease in pre-eclampsia incidence [38]. The extant literature indicates that maternal exercise is associated with a reduction in the risk of macrosomia, with reported decreases ranging from 4% to 61% [39]. Exercise can improve peripheral glucose tolerance via both insulin-dependent and insulin-independent mechanisms, addressing the underlying metabolic dysregulation such as progressively reduced peripheral and hepatic insulin sensitivity and impaired insulin action at the receptor level [40]. Moreover, the distinct metabolic impacts of aerobic exercise and resistance training uniquely contribute to combating insulin resistance in GDM patients, providing a comprehensive strategy for managing this condition [41].
A recent meta-analysis indicated that exercise interventions reduced the incidence of GDM by 39%, with optimal effects observed when light-to-moderate intensity exercise is initiated during the first trimester of pregnancy [42]. Structured exercise programs have also been shown to lower the risk of GDM development by up to 49%, with 140 min of weekly exercise correlating with a 25% reduction in GDM risk [43]. Research findings [44–46] suggest that engaging in physical exercise during pregnancy may lead to decreased blood glucose levels. However, an alternative study by Brislane et al. found that exercise programs had no significant effect on postprandial glucose concentrations [47]. Nevertheless, the exercise protocol employed in this study was of low intensity, and the sample size of pregnant women participating in the exercise intervention was relatively limited. Resistance training emerges as an effective strategy for managing insulin requirements and improving glucose control, as it can decrease fasting blood glucose levels and enhance postprandial glucose management [40]. Regular exercise during pregnancy can significantly enhance skeletal muscle strength, insulin sensitivity, and glucose uptake [48].
A randomized controlled trial involving 340 pregnant women has shown that dietary and physical activity interventions, facilitated by a smartphone app, can lead to lower average blood glucose levels compared to a control group [49]. Further evidence from a meta-analysis of nine studies confirms that exercise interventions can positively influence blood glucose levels in GDM patients, including postprandial glucose levels at 2 h and Hemoglobin A1c (HbA1c) levels [50]. Another randomized controlled trial (RCT) conducted in China highlighted that healthy lifestyle interventions had significant effects on fasting insulin levels and Homeostasis Model Assessment of Insulin Resistance (HOMA-IR) changes in women with a history of GDM, with interactions observed in those with cyclin-dependent kinase 5 regulatory subunit associated protein 1-like 1 (CDKAL1) gene variants persisting for two years, although the impact diminished over time [51]. CDKAL1 is currently found as a risk gene for diabetes, associated with the resistance and impaired synthesis of insulin [52]. Although the precise molecular mechanisms underlying remain unknown, CDKAL1 plays a crucial role in predicting the potential risk of the future development of T2DM in women with GDM [53,54]. Table 1 summarizes the clinical trials and parallel exercise studies in women with GDM.
Different types of exercise have varying effects on the prognosis of patients with gestational diabetes. In patients with gestational diabetes mellitus (GDM), aerobic workouts can effectively reduce fasting and post-meal blood glucose levels, along with HbA1c values. Conversely, resistance exercises have been shown to decrease the amount of insulin required by these patients [55]. Results from a meta-analysis also showed that resistance training improves blood glucose levels, insulin use, and macrosomia rates in women with GDM [56]. However, Keating et al. indicated that exercise, while beneficial for glycemic control, had no effect on preterm birth outcomes eventually [41]. A randomized controlled trial by Zhao et al. demonstrated that moderate-intensity exercise helped enhance glycemic regulation, insulin utilization, pregnancy-related weight management, and blood pressure control in patients with GDM [57]. However, it should be noted that this study was conducted with a limited number of participants. Another randomized controlled trial conducted by Xie et al. revealed that mothers engaging in resistance training exhibited reduced postprandial glucose levels at the 2 h mark compared to those performing aerobic exercises. Furthermore, the resistance training group showed superior compliance with the exercise regimen [44].
Aerobic exercise has been a focus of pregnancy exercise research for some time, while studies on resistance exercise have emerged in recent years [58]. There is a lack of research directly comparing resistance and aerobic exercise in the management of GDM, particularly with the lack of large RCTs. Future investigations should assess the long-term impacts of resistance exercise on pregnant women with GDM, increase comparative studies between resistance and aerobic exercise, and conduct more large-sample RCTs. By adding more forms of exercise during pregnancy, exercise programs can be designed individually according to the FITT principle for diverse pregnant populations, potentially enhancing patient adherence.
4 Effects of Maternal Exercise on Offspring
Maternal exercise during pregnancy positively impacts both the mother’s metabolic health and the metabolic programming of the fetus [60]. Studies indicate that maternal PA can enhance the offspring’s metabolic profile, with higher maternal moderate-to-vigorous physical activity (MVPA) correlating inversely with neonatal fat mass in male offspring [61]. A 7-year multicenter trial revealed that children of mothers with GDM risk factors are predisposed to higher body mass index (BMI) and overweight conditions [62]. Exercise is crucial for mitigating metabolic disorders, enhancing mitochondrial function, reducing inflammation and oxidative stress associated with obesity and hyperglycemia, and improving insulin resistance [48].
Animal research underscores the protective role of prenatal exercise against the adverse effects of maternal obesity and diabetes, such as obesity, insulin resistance, and hepatic steatosis. Maternal exercise during pregnancy can regulate the in-utero environment, influencing liver metabolism and preventing early weight gain and fat accumulation in female offspring of mothers with GDM, particularly those on high-fat, high-sugar diets [63]. It also reduces hepatic triglyceride accumulation in male offspring and enhances liver mitochondrial function, with benefits persisting post-exercise cessation [64]. The liver’s response to exercise may relate to improved insulin sensitivity and lipid metabolism, although the precise mechanisms remain under investigation [65].
Offspring of exercise-trained mothers exhibited increased maximal workload performance (MWP), associated with in-utero regulation of skeletal muscle mitochondrial density and functional markers, as evidenced by elevated AMP-activated protein kinase (AMPK) levels in the soleus muscle and reduced expression of Complex IV subunits in the tibialis anterior. Maternal exercise also counteracts diet-induced changes in miRNA expression [66]. Similar studies demonstrate that gestational exercise enhances insulin sensitivity and glucose tolerance in female offspring, with benefits extending to improved glucose tolerance and reduced insulin and cholesterol levels in adult offspring, irrespective of maternal weight and offspring diet [67]. Evidence also suggests that adequate PA during pregnancy and childbirth, combined with stress reduction and appropriate nutrition, can influence the early development of the central nervous and immune systems, and help prevent neurodevelopmental and psychiatric disorders in offspring [68]. In general, research has predominantly focused on investigating the effects of maternal exercise on neonatal outcomes, primarily utilizing rodent models. Consequently, there is a need for more research, especially clinical trials involving diverse human populations, to better understand the long-term health effects of prenatal exercise on offspring.
5 Mechanisms of Maternal Exercise on Mothers with GDM and Their Offspring
Exercise increases the resting metabolic rate (RMR) in women and improves insulin sensitivity in skeletal muscle [69]. Current reports indicate that muscle contractions during exercise stimulate the translocation of glucose transporters type 4 (GLUT4) glucose transporters from inside the cells to the surfaces of muscle cells, thus primarily increasing glucose uptake by muscle cells through the movement of GLUT4 [70]. The redistribution of GLUT4 during exercise primarily occurs through two independent but additive pathways. In the first pathway, insulin binds to its receptor insulin receptor substrates (IRS), leading to the phosphorylation of intracellular tyrosine residues, activation of the phosphoinositide 3-kinase (PI3K) pathway, and subsequent catalysis of phosphatidylinositol (3,4,5)-trisphosphate (PI3P) formation. PI3P further activates protein kinase B (Akt) and atypical protein kinase C (aPKC), triggering the translocation of GLUT4 to the cell membrane. The second pathway is the insulin-independent pathway and includes: (a) the release of Ca2+ from the sarcoplasmic reticulum for muscle contraction, leading to an increase in intracellular Ca2+; concentration and activation of Ca2+/calmodulin-dependent protein kinase (CaMK); (b) a decrease in the ATP/ADP ratio and activation of AMP-activated protein kinase (AMPK); and (c) a transient increase in oxidative stress, resulting in elevated levels of reactive oxygen species (ROS) [49]. Other exercise-induced mechanisms include increased endothelial nitric oxide activity, activation of mitogen-activated protein kinases (MAPK), activation of protein kinase C (PKC), bradykinin, hypoxia, elevation of muscle temperature, and mechanical deformation during muscle contractions. Evidence also suggests that exercise may enhance the activity and gene expression of GLUT4. The combined endpoint of these pathways is the activation of intracellular proteins TBC1D1/TBC1D4, leading to increased insulin sensitivity [71]. Furthermore, prolonged exposure of the pancreas to a hyperglycemic environment can trigger inflammation, which impairs glucose-stimulated insulin secretion and exacerbates β-cell apoptosis. Exercise may have beneficial effects on β-cell proliferation, neogenesis, and death by increasing circulating growth hormone concentrations and reducing blood glucose and lipid levels [72]. According to existing literature, exercise during pregnancy mainly influences maternal and offspring health through several mechanisms (Fig. 1).
Figure 1: A brief overview of effects and mechanisms of maternal exercise on mothers with GDM and their offspring. From the articles we obtained and screened, we summarized six modes of maternal exercise that most closely influence the clinical outcomes, glucose tolerance, insulin sensitivity, central nervous system development, and psychological behaviors in women with GDM and their offspring. VEGF = vascular endothelial growth factor. VEGFR1 = VEGF receptors 1. GDM = gestational diabetes mellitus
5.1 Maternal Exercise-Mediated Placental Adaptation
Maternal exercise has a mediating effect on both the mother and the fetus, and the placenta serves as the interface between them. Currently, research on exercise-mediated placental adaptation and its relationship with uterine placental perfusion and placental weight during pregnancy has yielded inconsistent findings. Studies have shown that women who follow a regular running regimen during pregnancy exhibit an increase in absolute (total chorionic capillary volume) and relative (total chorionic capillary volume/total chorionic volume) chorionic capillary volume [73]. Recently, Bhattacharjee et al. discovered that maternal exercise increased the levels of the angiogenic factor vascular endothelial growth factor (VEGF) and its receptor VEGF receptors 1 (VEGFR1), with no observed effects on placental growth factor (PlGF) and VEGF receptors 2 (VEGFR2) [74]. Research suggests a positive correlation between the cytokine VEGF and GDM. VEGF’s varying expression in placental and trophoblast cells makes it a potential biomarker for tracking GDM progression [75]. However, more research is needed in the future to demonstrate the specific mechanisms involved in pregnant women with GDM after exercise. Another recent cohort study involving obese pregnant women found that placental expression of pro-angiogenic and anti-angiogenic factors was not associated with average MVPA or percentage of sedentary time [76]. The pro-angiogenic effects of exercise on the placenta were also evident in mice, as data indicated that exercise training rescued the decrease in vascular density in a placental injury model induced by a high-fat diet [77].
GDM may be associated with placental mitochondrial dysfunction [78]. Recent studies have also found that physical exercise during pregnancy can enhance oxidative metabolism and improve placental function, possibly mediated in part by exercise factors secreted by the placenta, referred to as placental factors, including apelin, superoxide dismutase 3 (SOD3), irisin, and adiponectin. These factors mediate fetal development and maternal metabolism [79]. Irisin is an exercise-induced myokine released into the bloodstream due to cleavage of the extracellular domain of fibronectin type III domain containing 5 (FNDC5), primarily regulating thermogenesis and biogenesis in brown adipose tissue. Exercise-induced release of irisin can modulate muscle metabolism through AMPK activation [80]. Further findings indicate that regular maternal exercise can increase irisin production, stimulate the conversion of white fat to brown fat, regulate blood sugar levels, and boost calorie burning. This may be one of the ways exercises help prevent GDM. Pregnant women who exercised exhibited notably higher irisin levels and significantly reduced insulin resistance compared to the control group. Furthermore, the exercise group showed increased expression of beige adipose tissue marker genes (CD137, TMEM26, and TBX-1) in inguinal adipose tissue, as well as enhanced CD137 gene expression in subcutaneous adipose tissue. In a mouse study, exercising animals demonstrated markedly elevated irisin levels. Additionally, the expression of beige adipose tissue marker genes CD137 and TBX-1 was substantially higher in both inguinal subcutaneous and interscapular adipose tissues of the exercise group mice compared to their control counterparts [81].
Among the hormones increased due to maternal exercise, the level of apelin was found to be higher, existing in large quantities in the circulation of mothers, fetuses, and placenta [82]. Animal experiments have demonstrated that maternal exercise leads to increased placental apelin levels. High levels of apelin activate ten-eleven translocation (Tet), which converts 5-methylcytosine in the PR-domain containing 16 (Prdm16) promoters into 5-hydroxymethylcytosine [83]. Exercise-induced release of apelin increases acetyl-CoA carboxylase (ACC) phosphorylation via AMPK activation, which stimulates fatty acid oxidation. Exercise during pregnancy activates AMPK and peroxisome proliferator-activated receptor gamma coactivator 1alpha (PGC-1α) in the muscles of offspring in mother rats to stimulate the expression of uncoupling protein 3 (UCP3) and myoglobin, thereby inhibiting sarco-endoplasmic reticulum Ca-ATPase (SERCA), increasing intracellular Ca2+ levels, activating calmodulin-dependent kinase kinase 2 (CaMKK2) and enhancing the heat produced by muscle contractions [84]. Maternal exercise activates the apelin-APJ-AMPK axis, enhancing the expression of non-shivering thermogenesis (NST) genes in the muscle of offspring affected by maternal obesity, thus providing transgenerational protection against diet-induced obesity and metabolic disorders [85].
Leptin is a fat-derived hormone controlled by the hypothalamus, critically involved in the feedback regulation of food intake metabolism. Aberrant leptin expression can lead to weight gain, irregular food intake, and chronic obesity, particularly in women with GDM who often display elevated leptin levels [86]. Recent studies have found that maternal exercise can increase lipid levels, as well as plasma leptin and insulin levels in women, thereby improving the harmful effects of a high-fat diet [87,88]. Exercising women during pregnancy had lower leptin levels. However, when these women ceased exercising, the rate of increase in leptin levels was comparable to that in the control group [89]. Studies on rats have demonstrated that when mothers exercise, it decreases leptin resistance in their offspring by enhancing the expression of leptin receptor (Leprb), agouti-related peptide (Agrp), signal transducer and activator of transcription 3 (Stat3), insulin receptor (Insr), and pro-opiomelanocortin (Pomc) genes within the fetal hypothalamus [90].
Exercise can induce upregulation of SOD3, thereby mitigating the effects of oxidative stress [91]. Recent animal experiments have demonstrated that maternal exercise leads to an increase in the expression and secretion of placental SOD3 through vitamin D receptor-mediated mechanisms. This activation triggers the AMPK/TET signaling pathway in the offspring’s livers, particularly in cases where mother rats were fed high-fat diets. Consequently, this process leads to the demethylation of promoters for genes involved in glucose metabolism, resulting in improved liver function and glucose tolerance in the offspring [92].
Adiponectin, a hormone produced by fat cells, plays a crucial role in glucose regulation during pregnancy and exhibits anti-inflammatory and insulin-sensitizing effects. When adiponectin interacts with its receptors, it triggers protein kinase signaling pathways, which enhance fatty acid oxidation and suppress gluconeogenesis. In cases of GDM, the levels of adiponectin are markedly decreased, leading to impaired insulin sensitivity and elevated blood glucose levels [93]. Studies have shown that serum levels of adiponectin and C1q/tumor necrosis factor-related protein 3 (CTRP3) in subjects with GDM are lower than those in the control group [94]. Research conducted on animals has revealed that male progeny of mothers who exercised exhibited enhanced adiponectin expression in muscle tissue, along with decreased leptin concentrations and improved insulin responsiveness, when compared to the male offspring of inactive mothers consuming high-fat diets [95].
In recent years, metabolomics research to study exercise-responsive metabolites has enhanced our understanding of the potential effects of exercise. A considerable amount of literature has explored the metabolomic changes associated with different types intensities, and durations of exercise [96,97]. Recently, Contrepois et al. identified 728 metabolites that were significantly impacted by exercise through a analysis of blood metabolites in humans before and after acute exercise [98]. Furthermore, Schranner et al. found 196 metabolites that had significant changes in humans after endurance or resistance exercise [99]. Both resistance exercise (RE) and endurance exercise (EE) activate the metabolic pathways of glycolysis, the Tricarboxylic Acid (TCA) cycle, amino acid catabolism, and fat oxidation. However, RE shows a stronger tendency to significantly enhance the production of metabolites linked to glycolysis and the TCA cycle. In contrast, EE primarily stimulates an increase in succinate levels [100]. Many metabolites derived from branched-chain amino acids (BCAAs) exhibit significant responses to RE, similar to metabolites involved in the tricarboxylic acid cycle and glycolysis, while lipid-related metabolites show significant changes following EE, particularly several acylcarnitines and the ketone metabolite β-hydroxybutyrate (BHBA) [101]. Physically active pregnant women have significantly higher levels of monounsaturated fatty acids (MUFAs) during the second trimester. The positive correlation observed between MUFAs and MVPA in the plasma of pregnant women with GDM may be attributed to elevated levels of lipogenesis and fat deposition in early pregnancy. During the second trimester, the intervention group showed significantly lower levels of two long-chain monounsaturated acylcarnitines (myristoylcarnitine and palmitoylcarnitine) at 120 min post-OGTT compared to the control group [102]. Further studies found that sedentary time from early to mid-pregnancy was associated with the expression of placental genes related to lipid transport, indicating that a longer sedentary time was correlated with lower expression of placental fatty acid transport protein 2 (FATP2) and FATP3. In contrast, MVPA was negatively correlated with GLUT1 mRNA expression [103]. A clinical cohort study reported a significant association between PA and reduced BCAA levels in late pregnancy [104]. In contrast, another RCT found no association between umbilical cord blood metabolites and lifestyle interventions during pregnancy [105].
In recent years, it has been found that sustained alterations in the gut microbiota may be a key factor in the metabolic benefits of maternal exercise. The gut microbiome is associated with glucose homeostasis in both non-pregnant and pregnant women and may play a role in the development and persistence of GDM. The gut microbiota of women with GDM differs markedly in structure, composition, and metabolic function compared to that of women without GDM [106]. Studies have indicated that differences in glycemic regulation in late pregnancy are related to relatively modest changes in the gut microbiome composition of offspring within the first week and nine months after birth [107]. A cohort study observed significant differences in gut microbiota structure and composition at six months of age between the GDM and non-GDM groups, as well as significant differences in the dynamic changes of α-diversity from one to six months of age based on GDM status. Additionally, we found that changes in the gut bacteria of offspring born to GDM mothers were associated with infant growth [108]. The potential mechanisms by which maternal GDM alters the gut microbiota of offspring and subsequently affects infant growth are unclear. Animal experiments have demonstrated that maternal exercise can induce sustained changes in the gut microbiota, significantly alleviating the adverse metabolic effects of maternal high-fat diets on both mothers and male offspring. Maternal exercise significantly improved maternal insulin sensitivity and reduced metabolic disorders in offspring from childhood to adulthood through the reduction of detrimental bacteria and sustained enrichment of short-chain fatty acid producers, particularly Odoribacter [109]. In Wistar-Kyoto rats, maternal pre-pregnancy and pregnancy exercise increased the abundance of Helicobacter, which is associated with inflammatory bowel disease, as well as gastrointestinal inflammatory markers, inhibited the development of metabolic dysfunction, enhanced pancreatic β-cell mass, and prevented changes in the gut microbiome of GDM females [110]. Maternal voluntary wheel running can comprehensively program changes in gut microbiota composition and fecal metabolite levels, subsequently regulating butyrate metabolism and mediating gut gluconeogenesis in the offspring. The offspring of high-fat-fed and exercised mothers showed an increase in the abundance of Desulfovibrio and Verrucomicrobia [111].
The etiology of GDM is complex and involves genetic and environmental factors, mechanisms, and epidemiological studies [112]. It has been demonstrated that genes are not immutable; their expression can adapt to environmental changes, and increasing attention has been paid to the effects of exercise on the genome. Epigenetics suggests that adaptation of genes to the environment can lead to reversible changes in gene function without altering the DNA sequence [113]. A substantial amount of evidence indicates intergenerational epigenetics, with metabolic dysfunction significantly influencing transgenerational effects through epigenetic mechanisms [114]. An RCT found that lifestyle interventions during pregnancy can alter the impact of the prenatal maternal glycemic environment on epigenetics. Interventions involving diet and physical activity during pregnancy seem to reduce GDM and 1-h glucose-related methylation signatures in infant umbilical cord blood, affecting 87% of GDM-related and 77% of 1-h glucose-related dmCpGs [115]. Research on female mice has investigated whether maternal exercise during pregnancy could protect offspring from the adverse effects of maternal high-fat diets. Researchers found that maternal exercise prevented high-fat diet-induced hypermethylation of Pgc-1α and enhanced the expression of Pgc-1α and its target genes, thereby improving age-related metabolic dysfunction in progeny at 9 months of age [116]. The mechanism by which a maternal high-fat diet induces glucose metabolic dysregulation in the offspring of C57BL/6 mice involves increased reactive oxygen species, carbonylation of WD repeat domain 82 (WDR82), and inactivation of histone H3 lysine 4 (H3K4) methyltransferase leading to reduced H3K4me3 at glucose metabolism gene promoters, while exercise during pregnancy can reverse this signaling pathway [117].
Regular exercise can mitigate mitochondrial impairment, allowing these cellular powerhouses to sustain equilibrium between mitochondrial processes and self-degradation. These long-term adaptations resulting from consistent exercise enhance the capacity for oxidative metabolism [118]. Mitochondria are considered the “powerhouses of the cell” as they are the primary sites for adenosine triphosphate (ATP) production. Mitochondrial ROS can alter the insulin/insulin receptor substrate signaling pathways. Furthermore, overexpression of mitochondrial antioxidant enzymes that mitigate H₂O₂ can reduce the loss of insulin sensitivity induced by high-fat diets, whereas damaged or dysfunctional mitochondria can lead to a range of complex diseases, including obesity and diabetes [119]. Maternal exercise alters the redox state and mitochondrial function of offspring skeletal muscles in a sex-dependent manner [120]. Animal studies have shown that maternal exercise can reduce the oxidative stress associated with pre-gestational diabetes and decrease ROS and oxidative damage in the fetal heart, leading to a lower incidence of congenital heart defects in the offspring of mothers with pre-gestational diabetes [121].
The intrauterine hyperglycemic environment associated with gestational diabetes not only affects maternal health but can also be passed down across generations, increasing the metabolic susceptibility of the offspring. As an important regulator in utero, appropriate exercise during pregnancy does not increase the risk of preterm birth and can control excessive weight gain in pregnant women by influencing placental blood flow, mediating placental mitochondrial oxidative metabolism through exercise factors, or regulating plasma metabolites. This, in turn, reduces insulin resistance and the risk of developing gestational diabetes. Additionally, it can reprogram the metabolic characteristics of offspring through epigenetic changes and gut microbiota, thereby minimizing the negative effects on GDM offspring and improving maternal and child outcomes, thereby ensuring the safety and health of both mothers and children. However, further exploration of the molecular mechanisms by which exercise affects the health of mothers with GDM and their offspring, as well as the long-term metabolic health of descendants, will be beneficial in identifying therapeutic targets to improve the health of both mothers and offspring.
Acknowledgement: None.
Funding Statement: This work was supported by grants from the National Natural Science Foundation of China (Grant No. 82401994), the Top Medical Expert Team of the Wuxi Taihu Talent Plan (Grant Nos. DJTD202106, GDTD202105), and the Medical Key Discipline Program of the Wuxi Health Commission (Grant Nos. ZDXK2021007, CXTD2021005); Top Talent Support Program for Young and Middle-Aged People of Wuxi Health Committee (Grant No. HB2023079).
Author Contributions: The authors confirm their contribution to the paper as follows: study conception and design: Sha Chen, Minkai Cao; draft manuscript preparation: Sha Chen; review and editing: Kerong Liu, Ying Gu; visualization: Kerong Liu; supervision: Ying Gu. All authors reviewed the results and approved the final version of the manuscript.
Availability of Data and Materials: Not applicable.
Ethics Approval: Not applicable.
Conflicts of Interest: The authors declare no conflicts of interest to report regarding the present study.
References
1. Chew NWS, Ng CH, Tan DJH, Kong G, Lin C, Chin YH, et al. The global burden of metabolic disease: data from 2000 to 2019. Cell Metab. 2023;35(3):414–28. doi:10.1016/j.cmet.2023.02.003. [Google Scholar] [PubMed] [CrossRef]
2. Rodríguez-Martínez C, Leirós-Rodríguez R. The influence of practising physical activity on the prevention and treatment of gestational diabetes: a systematic review. Curr Diabetes Rev. 2022;18(4):e200821195742. doi:10.2174/1573399817666210820110941. [Google Scholar] [PubMed] [CrossRef]
3. Motahari-Tabari NS, Nasiri-Amiri F, Faramarzi M, Shirvani MA, Bakhtiari A, Omidvar S. The effectiveness of information-motivation-behavioral skills model on self-care practices in early pregnancy to prevent gestational diabetes mellitus in iranian overweight and obese women: a randomized controlled trial. Community Health Equity Res Policy. 2023;43(3):257–64. doi:10.1177/0272684X211020300. [Google Scholar] [PubMed] [CrossRef]
4. Chen T, Liu D. The mystery of exosomes in gestational diabetes mellitus. Oxid Med Cell Longev. 2022;2022:2169259. doi:10.1155/2022/2169259. [Google Scholar] [PubMed] [CrossRef]
5. Alejandro EU, Mamerto TP, Chung G, Villavieja A, Gaus NL, Morgan E, et al. Gestational diabetes mellitus: a harbinger of the vicious cycle of diabetes. Int J Mol Sci. 2020;21(14):5003. doi:10.3390/ijms21145003. [Google Scholar] [PubMed] [CrossRef]
6. Chatzakis C, Eleftheriades A, Demertzidou E, Dinas K, Vlahos N, Sotiriadis A, et al. Pregnancy outcomes in the different phenotypes of gestational diabetes mellitus based on the oral glucose tolerance test. A systematic review and meta-analysis. Diabetes Res Clin Pract. 2023;204:110913. doi:10.1016/j.diabres.2023.110913. [Google Scholar] [PubMed] [CrossRef]
7. Zito G, Della Corte L, Giampaolino P, Terzic M, Terzic S, Di Guardo F, et al. Gestational diabetes mellitus: prevention, diagnosis and treatment. A fresh look to a busy corner. J Neonatal Perinat Med. 2020;13(4):529–41. doi:10.3233/NPM-190305. [Google Scholar] [PubMed] [CrossRef]
8. James-Allan LB, Rosario FJ, Madi L, Barner K, Nair S, Lai A, et al. A novel technique using chronic infusion of small extracellular vesicles from gestational diabetes mellitus causes glucose intolerance in pregnant mice. Clin Sci. 2022;136(21):1535–49. doi:10.1042/CS20220484. [Google Scholar] [PubMed] [CrossRef]
9. Gunderson EP, Sun B, Catov JM, Carnethon M, Lewis CE, Allen NB, et al. Gestational diabetes history and glucose tolerance after pregnancy associated with coronary artery calcium in women during mid-life: the CARDIA study. Circulation. 2021;143(10):974. doi:10.1161/CIRCULATIONAHA.120.047320. [Google Scholar] [PubMed] [CrossRef]
10. Gu Y, Lu J, Li W, Liu H, Wang L, Leng J, et al. Joint associations of maternal gestational diabetes and hypertensive disorders of pregnancy with overweight in offspring. Front Endocrinol. 2019;10:645. doi:10.3389/fendo.2019.00645. [Google Scholar] [PubMed] [CrossRef]
11. Josefson JL, Catalano PM, Lowe WL, Scholtens DM, Kuang A, Dyer AR, et al. The joint associations of maternal BMI and glycemia with childhood adiposity. J Clin Endocrinol Metab. 2020;105(7):2177–88. doi:10.1210/clinem/dgaa180. [Google Scholar] [PubMed] [CrossRef]
12. Zhang X, Wang Y, Xiao W, Jiang D, Zhou J, Ye X, et al. Hyperglycaemia in pregnancy and offspring blood pressure: a systematic review and meta-analysis. Diabetol Metab Syndr. 2023;15(1):10. doi:10.1186/s13098-023-00978-2. [Google Scholar] [PubMed] [CrossRef]
13. Rasmussen L, Poulsen CW, Kampmann U, Smedegaard SB, Ovesen PG, Fuglsang J. Diet and healthy lifestyle in the management of gestational diabetes mellitus. Nutrients. 2020;12(10):3050. doi:10.3390/nu12103050. [Google Scholar] [PubMed] [CrossRef]
14. Physical activity and exercise during pregnancy and the postpartum period: ACOG committee opinion, number 804. Obstet Gynecol. 2020;135(4):e178–88. doi:10.1097/AOG.0000000000003772. [Google Scholar] [PubMed] [CrossRef]
15. American Diabetes Association. 14. Management of diabetes in pregnancy: standards of medical care in diabetes—2021. Diabetes Care. 2021;44:S200–10. doi:10.2337/dc21-S014. [Google Scholar] [PubMed] [CrossRef]
16. Karavasileiadou S, Almegwely W, Alanazi A, Alyami H, Chatzimichailidou S. Self-management and self-efficacy of women with gestational diabetes mellitus: a systematic review. Glob Health Action. 2022;15(1):2087298. doi:10.1080/16549716.2022.2087298. [Google Scholar] [PubMed] [CrossRef]
17. Tang F, Zhong X, Liu S, Guo X, Li D. Pathway analysis of the impact of health literacy, social support and self-efficacy on self-management behaviors in pregnant women with gestational diabetes mellitus. Front Public Health. 2023;11:1188072. doi:10.3389/fpubh.2023.1188072. [Google Scholar] [PubMed] [CrossRef]
18. Assaf EA, Al Sabbah H, Momani A, Al-Amer R, AA-Sa G, Ababneh A. Factors influencing gestational diabetes self-care among pregnant women in a Syrian refugee camp in Jordan. PLoS One. 2024;19:e0297051. doi:10.1371/journal.pone.0297051. [Google Scholar] [PubMed] [CrossRef]
19. Gascoigne EL, Webster CM, Honart AW, Wang P, Smith-Ryan A, Manuck TA. Physical activity and pregnancy outcomes: an expert review. Am J Obstet Gynecol MFM. 2023;5(1):100758. doi:10.1016/j.ajogmf.2022.100758. [Google Scholar] [PubMed] [CrossRef]
20. Bulut AN, Ceyhan V, Demir MB, Ak M, Dolanbay M. Exercise and outcomes in macrosomic pregnancies. J Matern Fetal Neonat. 2021;34(23):3958–62. doi:10.1080/14767058.2021.1875447. [Google Scholar] [PubMed] [CrossRef]
21. Adamczak L, Mantaj U, Sibiak R, Gutaj P, Wender-Ozegowska E. Physical activity, gestational weight gain in obese patients with early gestational diabetes and the perinatal outcome—a randomised-controlled trial. BMC Pregnancy Childbirth. 2024;24(1):104. doi:10.1186/s12884-024-06296-3. [Google Scholar] [PubMed] [CrossRef]
22. Chavarrias M, Carlos-Vivas J, Collado-Mateo D, Pérez-Gómez J. Health benefits of indoor cycling: a systematic review. Medicina. 2019;55(8):452. doi:10.3390/medicina55080452. [Google Scholar] [PubMed] [CrossRef]
23. Andersen MB, Ovesen PG, Daugaard M, Ostenfeld EB, Fuglsang J. Cycling reduces blood glucose excursions after an oral glucose tolerance test in pregnant women: a randomized crossover trial. Appl Physiol Nutr Metab. 2020;45(11):1247–52. doi:10.1139/apnm-2020-0020. [Google Scholar] [PubMed] [CrossRef]
24. Halse RE, Wallman KE, Newnham JP, Guelfi KJ. Pregnant women exercise at a higher intensity during 30 min of self-paced cycling compared with walking during late gestation: implications for 2 h postprandial glucose levels. Metabolism. 2013;62(6):801–7. doi:10.1016/j.metabol.2012.12.009. [Google Scholar] [PubMed] [CrossRef]
25. Ji M, Li R, Xu Y. Meta-analysis of the effect of different exercise modalities in the prevention and treatment of perinatal depression. J Affect Disord. 2024;350:442–51. doi:10.1016/j.jad.2024.01.076. [Google Scholar] [PubMed] [CrossRef]
26. Sahin ES, Gürkan ÖC. The effect of prenatal yoga on pregnancy-related symptoms: a pilot quasi-experimental study. Complement Med Res. 2022;30(3):195. doi:10.1159/000528801. [Google Scholar] [PubMed] [CrossRef]
27. Gönenç İ M, Dikmen HA. Effects of dance and music on pain and fear during childbirth. J Obstet Gynecol Neonatal Nurs. 2020;49(2):144–53. doi:10.1016/j.jogn.2019.12.005. [Google Scholar] [PubMed] [CrossRef]
28. Navas A, Carrascosa MDC, Artigues C, Ortas S, Portells E, Soler A, et al. Effectiveness of moderate-intensity aerobic water exercise during pregnancy on quality of life and postpartum depression: a multi-center, randomized controlled trial. J Clin Med. 2021;10(11):2432. doi:10.3390/jcm10112432. [Google Scholar] [PubMed] [CrossRef]
29. Cancela-Carral JM, Blanco B, López-Rodríguez A. Therapeutic aquatic exercise in pregnancy: a systematic review and meta-analysis. J Clin Med. 2022;11(3):501. doi:10.3390/jcm11030501. [Google Scholar] [PubMed] [CrossRef]
30. Sanches EF, Dos Santos TM, Odorcyk F, Untertriefallner H, Rezena E, Hoeper E, et al. Pregnancy swimming prevents early brain mitochondrial dysfunction and causes sex-related long-term neuroprotection following neonatal hypoxia-ischemia in rats. Exp Neurol. 2021;339:113623. doi:10.1016/j.expneurol.2021.113623. [Google Scholar] [PubMed] [CrossRef]
31. Franklin BA. Evolution of the ACSM guidelines: historical perspectives, new insights, and practical implications. ACSM’s Health Fitness J. 2021;25(2):26. doi:10.1249/FIT.0000000000000645. [Google Scholar] [CrossRef]
32. Perales M, Artal R, Lucia A. Exercise during pregnancy. JAMA. 2017;317(11):1113–4. doi:10.1001/jama.2017.0593. [Google Scholar] [PubMed] [CrossRef]
33. Evenson KR, Barakat R, Brown WJ, Dargent-Molina P, Haruna M, Mikkelsen EM, et al. Guidelines for physical activity during pregnancy: comparisons from around the world. Am J Lifestyle Med. 2014;8(2):102–21. doi:10.1177/1559827613498204. [Google Scholar] [PubMed] [CrossRef]
34. ACOG practice bulletin no. 190: gestational diabetes mellitus. Obstet Gynecol. 2018;131(2):e49. doi:10.1097/AOG.0000000000002501. [Google Scholar] [PubMed] [CrossRef]
35. Mottola MF, Davenport MH, Ruchat SM, Davies GA, Poitras VJ, Gray CE, et al. 2019 Canadian guideline for physical activity throughout pregnancy. Br J Sports Med. 2018;52(21):1339–46. doi:10.1136/bjsports-2018-100056. [Google Scholar] [PubMed] [CrossRef]
36. Savvaki D, Taousani E, Goulis DG, Tsirou E, Voziki E, Douda H, et al. Guidelines for exercise during normal pregnancy and gestational diabetes: a review of international recommendations. Hormones. 2018;17(4):521–9. doi:10.1007/s42000-018-0085-6. [Google Scholar] [PubMed] [CrossRef]
37. Noble BJ. Clinical applications of perceived exertion. Med Sci Sports Exerc. 1982;14(5):406–11. doi:10.1249/00005768-198205000-00016. [Google Scholar] [PubMed] [CrossRef]
38. Davenport MH, Ruchat SM, Poitras VJ, Jaramillo Garcia A, Gray CE, Barrowman N, et al. Prenatal exercise for the prevention of gestational diabetes mellitus and hypertensive disorders of pregnancy: a systematic review and meta-analysis. Br J Sports Med. 2018;52(21):1367–75. doi:10.1136/bjsports-2018-099355. [Google Scholar] [PubMed] [CrossRef]
39. Ribeiro MM, Andrade A, Nunes I. Physical exercise in pregnancy: benefits, risks and prescription. J Perinat Med. 2022;50(1):4–17. doi:10.1515/jpm-2021-0315. [Google Scholar] [PubMed] [CrossRef]
40. Allman BR, McDonald S, May L, Børsheim E. Resistance training as a countermeasure in women with gestational diabetes mellitus: a review of current literature and future directions. Sports Med. 2022;52(12):2871–88. doi:10.1007/s40279-022-01724-w. [Google Scholar] [PubMed] [CrossRef]
41. Keating N, Coveney C, McAuliffe FM, Higgins MF. Aerobic or resistance exercise for improved glycaemic control and pregnancy outcomes in women with gestational diabetes mellitus: a systematic review. Int J Environ Res Public Health. 2022;19(17):10791. doi:10.3390/ijerph191710791. [Google Scholar] [PubMed] [CrossRef]
42. Martínez-Vizcaíno V, Sanabria-Martínez G, Fernández-Rodríguez R, Cavero-Redondo I, Pascual-Morena C, Álvarez-Bueno C, et al. Exercise during pregnancy for preventing gestational diabetes mellitus and hypertensive disorders: an umbrella review of randomised controlled trials and an updated meta-analysis. BJOG: Int J Obstet Gynaecol. 2023;130(3):264–75. doi:10.1111/1471-0528.17304. [Google Scholar] [PubMed] [CrossRef]
43. Herzberger V, Bäz E, Kunze M, Markfeld-Erol F, Juhasz-Böss I. Exercise during pregnancy. Dtsch Arztebl Int. 2022;119(46):793–7. doi:10.3238/arztebl.m2022.0305. [Google Scholar] [PubMed] [CrossRef]
44. Xie Y, Zhao H, Zhao M, Huang H, Liu C, Huang F, et al. Effects of resistance exercise on blood glucose level and pregnancy outcome in patients with gestational diabetes mellitus: a randomized controlled trial. BMJ Open Diabetes Res Care. 2022;10(2):e002622. doi:10.1136/bmjdrc-2021-002622. [Google Scholar] [PubMed] [CrossRef]
45. Jin Y, Chen Z, Li J, Zhang W, Feng S. Effects of the original gymnastics for pregnant women program on glycaemic control and delivery outcomes in women with gestational diabetes mellitus: a randomized controlled trial. Int J Nurs Stud. 2022;132:104271. doi:10.1016/j.ijnurstu.2022.104271. [Google Scholar] [PubMed] [CrossRef]
46. Zhang R, Gao X, Sun T, Li H, Yang Q, Li B, et al. Longer physical exercise duration prevents abnormal fasting plasma glucose occurrences in the third trimester: findings from a cohort of women with gestational diabetes mellitus in Shanghai. Front Endocrinol. 2023;14:1054153. doi:10.3389/fendo.2023.1054153. [Google Scholar] [PubMed] [CrossRef]
47. Brislane Á, Reid LA, Bains G, Greenwall K, Khurana R, Davenport MH. Optimizing blood glucose control through the timing of exercise in pregnant individuals diagnosed with gestational diabetes mellitus. Int J Environ Res Public Health. 2023;20(8):5500. doi:10.3390/ijerph20085500. [Google Scholar] [PubMed] [CrossRef]
48. Dipla K, Zafeiridis A, Mintziori G, Boutou AK, Goulis DG, Hackney AC. Exercise as a therapeutic intervention in gestational diabetes mellitus. Endocrines. 2021;2(2):65–78. doi:10.3390/endocrines2020007. [Google Scholar] [PubMed] [CrossRef]
49. Yew TW, Chi C, Chan SY, van Dam RM, Whitton C, Lim CS, et al. A randomized controlled trial to evaluate the effects of a smartphone application-based lifestyle coaching program on gestational weight gain, glycemic control, and maternal and neonatal outcomes in women with gestational diabetes mellitus: the SMART-GDM study. Diabetes Care. 2021;44(2):456–63. doi:10.2337/dc20-1216. [Google Scholar] [PubMed] [CrossRef]
50. Li X, Luo R, Qiao B, Ou H. Exercise intervention improves blood glucose levels and adverse pregnancy outcomes in GDM patients: a meta-analysis. Comput Math Methods Med. 2022;2022:9287737. doi:10.1155/2022/9287737. [Google Scholar] [PubMed] [CrossRef]
51. Liang Z, Wang L, Liu H, Chen Y, Zhou T, Heianza Y, et al. Genetic susceptibility, lifestyle intervention and glycemic changes among women with prior gestational diabetes. Clin Nutr. 2020;39(7):2144–50. doi:10.1016/j.clnu.2019.08.032. [Google Scholar] [PubMed] [CrossRef]
52. Ghosh C, Das N, Saha S, Kundu T, Sircar D, Roy P. Involvement of Cdkal1 in the etiology of type 2 diabetes mellitus and microvascular diabetic complications: a review. J Diabetes Metabolic Disorders. 2022;21(1):991–1001. doi:10.1007/s40200-021-00953-6. [Google Scholar] [PubMed] [CrossRef]
53. Wang Y, Li L, Li P. Novel single nucleotide polymorphisms in gestational diabetes mellitus. Clinica Chimica Acta. 2023;538(1):60–4. doi:10.1016/j.cca.2022.11.010. [Google Scholar] [PubMed] [CrossRef]
54. Pervjakova N, Moen GH, Borges MC, Ferreira T, Cook JP, Allard C, et al. Multi-ancestry genome-wide association study of gestational diabetes mellitus highlights genetic links with type 2 diabetes. Hum Mol Genet. 2022;31(19):3377–91. doi:10.1093/hmg/ddac050. [Google Scholar] [PubMed] [CrossRef]
55. Huang X, Huang J, Wu J, Li M, Yang Z, Liu L, et al. Different exercises for pregnant women with gestational diabetes: a meta-analysis of randomized controlled trials. J Sports Med Phys Fitness. 2020;60(3):464–71. doi:10.23736/S0022-4707.19.10131-4. [Google Scholar] [PubMed] [CrossRef]
56. Xie Y, Zhao H, Liu C, Huang F, Huang H, Zhao M. A meta-analysis of the effects of resistance training on blood sugar and pregnancy outcomes. Midwifery. 2020;91(1):102839. doi:10.1016/j.midw.2020.102839. [Google Scholar] [PubMed] [CrossRef]
57. Zhao H, Xie Y, Zhao M, Huang H, Liu C, Huang F, et al. Effects of moderate-intensity resistance exercise on blood glucose and pregnancy outcome in patients with gestational diabetes mellitus: a randomized controlled trial. J Diabetes Complicat. 2022;36(5):108186. [Google Scholar]
58. Duchette C, Perera M, Arnett S, White E, Belcher E, Tinius R. Benefits of resistance training during pregnancy for maternal and fetal health: a brief overview. Int J Women’s Health. 2024;16:1137–47. [Google Scholar]
59. Qazi WA, Babur MN, Malik AN, Begum R. Effects of structured exercise regime on glycosylated hemoglobin and C reactive protein in patients with gestational diabetes mellitus—a randomized controlled trial. Pak J Med Sci. 2020;36(7):1449–53. [Google Scholar] [PubMed]
60. Chaves A, Weyrauch LA, Zheng D, Biagioni EM, Krassovskaia PM, Davidson BL, et al. Influence of maternal exercise on glucose and lipid metabolism in offspring stem cells: ENHANCED by mom. J Clin Endocrinol Metab. 2022;107(8):e3353–65. [Google Scholar] [PubMed]
61. Dieberger AM, Obermayer-Pietsch B, Harreiter J, Desoye G, van Poppel MNM. Physical activity and sedentary time across pregnancy and associations with neonatal weight, adiposity and cord blood parameters: a secondary analysis of the DALI study. Int J Obes. 2023;47(9):873–81. [Google Scholar]
62. Kolseth Å J, Kulseth S, Stafne SN, Mørkved S, Salvesen K, Evensen KAI. Physical health and neurodevelopmental outcome in 7-year-old children whose mothers were at risk of gestational diabetes mellitus: a follow-up of a randomized controlled trial. Acta Obstet Gynecol Scand. 2023;102(9):1193–202. doi:10.1111/aogs.14593. [Google Scholar] [PubMed] [CrossRef]
63. Stevanović-Silva J, Beleza J, Coxito P, Rocha H, Gaspar TB, Gärtner F, et al. Exercise performed during pregnancy positively modulates liver metabolism and promotes mitochondrial biogenesis of female offspring in a rat model of diet-induced gestational diabetes. BBA—Mol Basis Dis. 2022;1868(11):166526. doi:10.1016/j.bbadis.2022.166526. [Google Scholar] [PubMed] [CrossRef]
64. Stevanović-Silva J, Beleza J, Coxito P, Pereira S, Rocha H, Gaspar TB, et al. Maternal high-fat high-sucrose diet and gestational exercise modulate hepatic fat accumulation and liver mitochondrial respiratory capacity in mothers and male offspring. Metabolism. 2021;116:154704. doi:10.1016/j.metabol.2021.154704. [Google Scholar] [PubMed] [CrossRef]
65. Bernier M, Enamorado IN, Gómez-Cabrera MC, Calvo-Rubio M, González-Reyes JA, Price NL, et al. Age-dependent impact of two exercise training regimens on genomic and metabolic remodeling in skeletal muscle and liver of male mice. npj Aging. 2022;8(1):8. doi:10.1038/s41514-022-00089-8. [Google Scholar] [PubMed] [CrossRef]
66. Beleza J, Stevanović-Silva J, Coxito P, Rocha H, Santos P, Ascensão A, et al. Gestational exercise increases male offspring’s maximal workload capacity early in life. Int J Mol Sci. 2022;23(7):3916. doi:10.3390/ijms23073916. [Google Scholar] [PubMed] [CrossRef]
67. Ding L, Liu J, Zhou L, Xiao X. Maternal exercise impacts offspring metabolic health in adulthood: a systematic review and meta-analysis of animal studies. Nutrients. 2023;15(12):2793. [Google Scholar] [PubMed]
68. Marques AH, Bjørke-Monsen AL, Teixeira AL, Silverman MN. Maternal stress, nutrition and physical activity: impact on immune function, CNS development and psychopathology. Brain Res. 2015;1617:28–46. [Google Scholar] [PubMed]
69. Taousani E, Savvaki D, Tsirou E, Grammatikopoulou MG, Tarlatzis BC, Vavilis D, et al. Effect of exercise on the resting metabolic rate and substrate utilization in women with gestational diabetes mellitus: results of a pilot study. Metabolites. 2022;12(10):998. [Google Scholar] [PubMed]
70. Merz KE, Thurmond DC. Role of skeletal muscle in insulin resistance and glucose uptake. Compr Physiol. 2020;10(3):785–809. [Google Scholar] [PubMed]
71. Halvatsiotis P, Panagiotou O, Koulouvaris P, Raptis A, Bamias A, Kalantaridou S, et al. Benefits of exercise in pregnancies with gestational diabetes. J Matern Fetal Neonatal Med. 2022;35(13):2524–9. [Google Scholar] [PubMed]
72. Narendran P, Solomon TP, Kennedy A, Chimen M, Andrews RC. The time has come to test the beta cell preserving effects of exercise in patients with new onset type 1 diabetes. Diabetologia. 2015;58(1):10–8. doi:10.1007/s00125-014-3412-8. [Google Scholar] [PubMed] [CrossRef]
73. Bergmann A, Zygmunt M, Clapp JF3rd. Running throughout pregnancy: effect on placental villous vascular volume and cell proliferation. Placenta. 2004;25(8–9):694–8. [Google Scholar] [PubMed]
74. Bhattacharjee J, Mohammad S, Goudreau AD, Adamo KB. Physical activity differentially regulates VEGF, PlGF, and their receptors in the human placenta. Physiol Rep. 2021;9(2):e14710. doi:10.14814/phy2.14710. [Google Scholar] [PubMed] [CrossRef]
75. Bolatai A, He Y, Wu N. Vascular endothelial growth factor and its receptors regulation in gestational diabetes mellitus and eclampsia. J Transl Med. 2022;20(1):400. doi:10.1186/s12967-022-03603-4. [Google Scholar] [PubMed] [CrossRef]
76. Zafaranieh S, Siwetz M, Leopold-Posch B, Kummer D, Huppertz B, Desoye G, et al. Placental structural adaptation to maternal physical activity and sedentary behavior: findings of the DALI lifestyle study. Hum Reprod. 2024;39(9):2146. [Google Scholar]
77. Bhattacharjee J, Mohammad S, Adamo KB. Does exercise during pregnancy impact organs or structures of the maternal-fetal interface? Tissue Cell. 2021;72:101543. [Google Scholar] [PubMed]
78. Fisher JJ, Vanderpeet CL, Bartho LA, McKeating DR, Cuffe JSM, Holland OJ, et al. Mitochondrial dysfunction in placental trophoblast cells experiencing gestational diabetes mellitus. J Physiol. 2021;599(4):1291–305. [Google Scholar] [PubMed]
79. Chae SA, Son JS, Du M. Prenatal exercise in fetal development: a placental perspective. FEBS J. 2022;289(11):3058–71. [Google Scholar] [PubMed]
80. Sousa RAL, Improta-Caria AC, Souza BSF. Exercise-linked Irisin: consequences on mental and cardiovascular health in type 2 diabetes. Int J Mol Sci. 2021;22(4):2199. [Google Scholar] [PubMed]
81. Wang C, Zhang X, Liu M, Qin S, He C, Liu Y, et al. Irisin participates in the beneficial effects of exercise in preventing gestational diabetes mellitus in overweight and obese pregnant women and a mouse model. Front Nutr. 2022;9:1034443. [Google Scholar] [PubMed]
82. Alizadeh Pahlavani H. Possible roles of exercise and apelin against pregnancy complications. Front Endocrinol. 2022;13:965167. doi:10.3389/fendo.2022.965167. [Google Scholar] [PubMed] [CrossRef]
83. Son JS, Zhao L, Chen Y, Chen K, Chae SA, de Avila JM, et al. Maternal exercise via exerkine apelin enhances brown adipogenesis and prevents metabolic dysfunction in offspring mice. Sci Adv. 2020;6(16):eaaz0359. doi:10.1126/sciadv.aaz0359. [Google Scholar] [PubMed] [CrossRef]
84. Pahlavani HA, Laher I, Weiss K, Knechtle B, Zouhal H. Physical exercise for a healthy pregnancy: the role of placentokines and exerkines. J Physiol Sci. 2023;73(1):30. doi:10.1186/s12576-023-00885-1. [Google Scholar] [PubMed] [CrossRef]
85. Son JS, Chae SA, Zhao L, Wang H, de Avila JM, Zhu MJ, et al. Maternal exercise intergenerationally drives muscle-based thermogenesis via activation of apelin-AMPK signaling. eBioMed. 2022;76(7):103842. doi:10.1016/j.ebiom.2022.103842. [Google Scholar] [PubMed] [CrossRef]
86. Sharma AK, Singh S, Singh H, Mahajan D, Kolli P, Mandadapu G, et al. Deep insight of the pathophysiology of gestational diabetes mellitus. Cells. 2022;11(17):2672. doi:10.3390/cells11172672. [Google Scholar] [PubMed] [CrossRef]
87. Clark E, Isler C, Strickland D, McMillan AG, Fang X, Kuehn D, et al. Influence of aerobic exercise on maternal lipid levels and offspring morphometrics. Int J Obes. 2019;43(3):594–602. [Google Scholar]
88. Anany MG, El-Kosery SM, Ashmawy HSE, Osman DA. Effect of aerobic and resistive exercise on lipid profile and quality of life in overweight breastfeeding women: a randomized controlled trial. Physiother Res Int. 2024;29(4):e2121. [Google Scholar] [PubMed]
89. Clapp JF3rd, Kiess W. Effects of pregnancy and exercise on concentrations of the metabolic markers tumor necrosis factor alpha and leptin. Am J Obstet Gynecol. 2000;182(2):300–6. [Google Scholar] [PubMed]
90. Song L, Yan J, Wang N, Wei X, Luo X, Meng K, et al. Prenatal exercise reverses high-fat-diet-induced placental alterations and alters male fetal hypothalamus during late gestation in rats. Biol Reprod. 2020;102(3):705–16. [Google Scholar] [PubMed]
91. Kojda G, Hambrecht R. Molecular mechanisms of vascular adaptations to exercise. Physical activity as an effective antioxidant therapy? Cardiovasc Res. 2005;67(2):187–97. [Google Scholar] [PubMed]
92. Kusuyama J, Alves-Wagner AB, Conlin RH, Makarewicz NS, Albertson BG, Prince NB, et al. Placental superoxide dismutase 3 mediates benefits of maternal exercise on offspring health. Cell Metab. 2021;33(5):939–56. doi:10.1016/j.cmet.2021.03.004. [Google Scholar] [PubMed] [CrossRef]
93. Xie YP, Lin S, Xie BY, Zhao HF. Recent progress in metabolic reprogramming in gestational diabetes mellitus: a review. Front Endocrinol. 2023;14:1284160. doi:10.3389/fendo.2023.1284160. [Google Scholar] [PubMed] [CrossRef]
94. Fadaei R, Goodarzi G, Yarahmadi S, Allahyari P, Fallah S, Moradi N. Circulating levels of C1q/TNF-related protein 3 (CTRP3) and CTRP9 in gestational diabetes and their association with insulin resistance and inflammatory cytokines. Lab Med. 2023;54(3):262–9. doi:10.1093/labmed/lmac096. [Google Scholar] [PubMed] [CrossRef]
95. Wasinski F, Bacurau RF, Estrela GR, Klempin F, Arakaki AM, Batista RO, et al. Exercise during pregnancy protects adult mouse offspring from diet-induced obesity. Nutr Metab. 2015;12(1):56. doi:10.1186/s12986-015-0052-z. [Google Scholar] [PubMed] [CrossRef]
96. Yang YR, Kwon KS. Potential roles of exercise-induced plasma metabolites linking exercise to health benefits. Front Physiol. 2020;11:602748. doi:10.3389/fphys.2020.602748. [Google Scholar] [PubMed] [CrossRef]
97. Li K, Schön M, Naviaux JC, Monk JM, Alchus-Laiferová N, Wang L, et al. Cerebrospinal fluid and plasma metabolomics of acute endurance exercise. FASEB J. 2022;36(7):e22408. doi:10.1096/fj.202200509R. [Google Scholar] [PubMed] [CrossRef]
98. Contrepois K, Wu S, Moneghetti KJ, Hornburg D, Ahadi S, Tsai MS, et al. Molecular choreography of acute exercise. Cell. 2020;181(5):1112–30. doi:10.1016/j.cell.2020.04.043. [Google Scholar] [PubMed] [CrossRef]
99. Schranner D, Kastenmüller G, Schönfelder M, Römisch-Margl W, Wackerhage H. Metabolite concentration changes in humans after a bout of exercise: a systematic review of exercise metabolomics studies. Sports Med—Open. 2020;6(1):11. doi:10.1186/s40798-020-0238-4. [Google Scholar] [PubMed] [CrossRef]
100. Morville T, Sahl RE, Moritz T, Helge JW, Clemmensen C. Plasma metabolome profiling of resistance exercise and endurance exercise in humans. Cell Rep. 2020;33(13):108554. doi:10.1016/j.celrep.2020.108554. [Google Scholar] [PubMed] [CrossRef]
101. Wilkinson DJ, Crossland H, Atherton PJ. Metabolomic and proteomic applications to exercise biomedicine. Transl Exerc Biomed. 2024;1(1):9–22. doi:10.1515/teb-2024-2006. [Google Scholar] [PubMed] [CrossRef]
102. da Silva ACR, Yadegari A, Tzaneva V, Vasanthan T, Laketic K, Shearer J, et al. Metabolomics to understand alterations induced by physical activity during pregnancy. Metabolites. 2023;13(12):1178. [Google Scholar] [PubMed]
103. Acosta-Manzano P, Leopold-Posch B, Simmons D, Devlieger R, Galjaard S, Corcoy R, et al. The unexplored role of sedentary time and physical activity in glucose and lipid metabolism-related placental mRNAs in pregnant women who are obese: the DALI lifestyle randomised controlled trial. BJOG: Int J Obstet Gynaecol. 2022;129(5):708–21. [Google Scholar]
104. Maitre L, Villanueva CM, Lewis MR, Ibarluzea J, Santa-Marina L, Vrijheid M, et al. Maternal urinary metabolic signatures of fetal growth and associated clinical and environmental factors in the INMA study. BMC Med. 2016;14(1):177. [Google Scholar] [PubMed]
105. Patel N, Hellmuth C, Uhl O, Godfrey K, Briley A, Welsh P, et al. Cord metabolic profiles in obese pregnant women: insights into offspring growth and body composition. J Clin Endocrinol Metab. 2018;103(1):346–55. [Google Scholar] [PubMed]
106. Man B, Koenig MD, Bernabe BP, Nagelli U, Tussing-Humphreys L. The role of the gut microbiota in the prevention and management of gestational diabetes mellitus: are we there yet? J Perinatal Neonatal Nurs. 2020;34(3):195–8. [Google Scholar] [PubMed]
107. Crusell MKW, Hansen TH, Nielsen T, Allin KH, Rühlemann MC, Damm P, et al. Comparative studies of the gut microbiota in the offspring of mothers with and without gestational diabetes. Front Cell Infect Microbiol. 2020;10:536282. doi:10.3389/fcimb.2020.536282. [Google Scholar] [PubMed] [CrossRef]
108. Song Q, Zhou T, Chen S, Liao Y, Huang H, Xiao B, et al. Association of gestational diabetes with the dynamic changes of gut microbiota in offspring from 1 to 6 months of age. J Clin Endocrinol Metab. 2023;108(9):2315–23. doi:10.1210/clinem/dgad107. [Google Scholar] [PubMed] [CrossRef]
109. Zhou L, Xiao X, Li M, Zhang Q, Yu M, Zheng J, et al. Maternal exercise improves high-fat diet-induced metabolic abnormalities and gut microbiota profiles in mouse dams and offspring. Front Cell Infect Microbiol. 2020;10:292. doi:10.3389/fcimb.2020.00292. [Google Scholar] [PubMed] [CrossRef]
110. Mahizir D, Briffa JF, Wood JL, Anevska K, Hill-Yardin EL, Jefferies AJ, et al. Exercise improves metabolic function and alters the microbiome in rats with gestational diabetes. FASEB J. 2020;34(1):1728–44. doi:10.1096/fj.201901424R. [Google Scholar] [PubMed] [CrossRef]
111. Zhang L, Zou W, Hu Y, Wu H, Gao Y, Zhang J, et al. Maternal voluntary wheel running modulates glucose homeostasis, the gut microbiota and its derived fecal metabolites in offspring. Clin Sci. 2023;137(15):1151–66. doi:10.1042/CS20230372. [Google Scholar] [PubMed] [CrossRef]
112. Johns EC, Denison FC, Norman JE, Reynolds RM. Gestational diabetes mellitus: mechanisms, treatment, and complications. Trends Endocrinol Metab: TEM. 2018;29(11):743–54. [Google Scholar] [PubMed]
113. Reichetzeder C. Overweight and obesity in pregnancy: their impact on epigenetics. Eur J Clin Nutr. 2021;75(12):1710–22. [Google Scholar] [PubMed]
114. Fujita T. Recent advances in hypertension: epigenetic mechanism involved in development of salt-sensitive hypertension. Hypertension. 2023;80(4):711–8. [Google Scholar] [PubMed]
115. Antoun E, Kitaba NT, Titcombe P, Dalrymple KV, Garratt ES, Barton SJ, et al. Maternal dysglycaemia, changes in the infant’s epigenome modified with a diet and physical activity intervention in pregnancy: secondary analysis of a randomised control trial. PLoS Med. 2020;17(11):e1003229. [Google Scholar] [PubMed]
116. Laker RC, Lillard TS, Okutsu M, Zhang M, Hoehn KL, Connelly JJ, et al. Exercise prevents maternal high-fat diet-induced hypermethylation of the Pgc-1α gene and age-dependent metabolic dysfunction in the offspring. Diabetes. 2014;63(5):1605–11. [Google Scholar] [PubMed]
117. Kusuyama J, Makarewicz NS, Albertson BG, Alves-Wagner AB, Conlin RH, Prince NB, et al. Maternal exercise-induced SOD3 reverses the deleterious effects of maternal high-fat diet on offspring metabolism through stabilization of H3K4me3 and protection against WDR82 carbonylation. Diabetes. 2022;71(6):1170–81. doi:10.2337/db21-0706. [Google Scholar] [PubMed] [CrossRef]
118. Mendham AE, Goedecke JH, Zeng Y, Larsen S, George C, Hauksson J, et al. Exercise training improves mitochondrial respiration and is associated with an altered intramuscular phospholipid signature in women with obesity. Diabetologia. 2021;64(7):1642–59. doi:10.1007/s00125-021-05430-6. [Google Scholar] [PubMed] [CrossRef]
119. Wang L, Hu J, Zhou H. Macrophage and adipocyte mitochondrial dysfunction in obesity-induced metabolic diseases. World J Men’s Health. 2021;39(4):606–14. doi:10.5534/wjmh.200163. [Google Scholar] [PubMed] [CrossRef]
120. Hözer RM, Dos Santos BG, August PM, Rodrigues KS, Maurmann RM, Flores EB, et al. Maternal exercise during pregnancy modulates mitochondrial function and redox status in a sex-dependent way in adult offspring’s skeletal muscle. J Dev Orig Health Dis. 2022;13(2):204–11. doi:10.1017/S2040174421000209. [Google Scholar] [PubMed] [CrossRef]
121. Saiyin T, Engineer A, Greco ER, Kim MY, Lu X, Jones DL, et al. Maternal voluntary exercise mitigates oxidative stress and incidence of congenital heart defects in pre-gestational diabetes. J Cell Mol Med. 2019;23(8):5553–65. doi:10.1111/jcmm.14439. [Google Scholar] [PubMed] [CrossRef]
Cite This Article
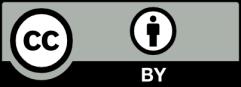
This work is licensed under a Creative Commons Attribution 4.0 International License , which permits unrestricted use, distribution, and reproduction in any medium, provided the original work is properly cited.