Open Access
ARTICLE
MiR-219a-5p exerts a protective function in a mouse model of myocardial infarction
Department of Cardiology, Zhongda Hospital, Medical School of Southeast University, Nanjing, 210009, China
* Corresponding Author: ZULONG SHENG. Email:
BIOCELL 2024, 48(9), 1369-1377. https://doi.org/10.32604/biocell.2024.049905
Received 22 January 2024; Accepted 20 July 2024; Issue published 04 September 2024
Abstract
Background: Myocardial infarction (MI) is known worldwide for its important disabling features, including myocarditis and cardiomyocyte apoptosis. It is believed that microRNA (miRNA) has a role in the cellular processes of apoptosis and myocarditis, and miR-219a-5p has been found to suppress the inflammatory response. However, unknown is the precise mechanism by which miR-219a-5p contributes to MI. Methods: We measured the expression of miR-219a-5p and evaluated its effects on target proteins, inflammatory factors, and apoptosis in a mouse model of MI. Echocardiography was utilized to examine the MI clinical index, and triphenyl tetrazolium chloride staining was employed to analyze the infarcted region. Enzyme-linked immunosorbent assay and Western blotting measured serum and molecular markers in heart tissues. To quantify the association with miR-219a-5p and ATPase sarcoplasmic/endoplasmic reticulum Ca2+ transporting 2 (ATP2A2), the luciferase activity assay and Pearson’s correlation analysis were employed. Results: MiR-219a-5p exhibited low expression in a mouse model of MI, and its amplification prevented both apoptotic and inflammatory reactions. Specifically, miR-219a-5p targeted ATP2A2. Conclusion: In a mouse model of MI, miR-219a-5p exerted a potent protective effect via direct targeting of ATP2A2.Keywords
Globally, myocardial infarction (MI) is a prevalent cardiovascular ailment. Its rates of illness and death are currently rising because of genetic diversification; therefore, MI significantly endangers human health [1–3]. MI increases the abundance of oxygen-free radicals and causes calcium overloading, aggravating apoptosis, with the clinical manifestations including deterioration of cardiac function, ventricular remodeling, arrhythmia, and expansion of the infarcted area [4]. A previous study showed that MI-induced pathological changes, such as ventricular remodeling and heart failure, involve cardiomyocyte apoptosis and inflammation, which are the leading causes of MI progression and poor prognosis [5].
MicroRNAs (miRNAs) are non-coding single-stranded small RNAs composed of 19–25 nucleotides and are tissue-specific [6,7]. MiRNAs have an irreplaceable function in the development of cardiovascular diseases, and elevated levels of some extracellular miRNAs (miR-1, -133a, -208a, -208b, -499) can be used as early clinical biomarkers of acute MI [8–11]. MiR-219a-5p was recently shown to promote oligodendrogenesis [12] and suppress osteosarcoma [13]. Moreover, the study revealed that miR-219a-5p suppressed the inflammatory response [14]. Nevertheless, to date, no research has been done to assess the mechanism underlying miR-219a-5p’s role in MI.
ATP2A2 encodes cardiac sarcoplasmic/endoplasmic reticulum calcium-ATPase 2a (SERCA2a), which modulates intracellular calcium handling. Therefore, it possesses a crucial role in cardiac contraction and relaxation. One study stated that ATP2A2 downregulation was accompanied by atrial systolic dysfunction [15]. Numerous miRNAs have been discovered to target ATP2A2, according to earlier research. MiR-200c-3p negatively regulates ATP2A2 and promotes the progression of thyroid carcinoma [16]. Moreover, miR-25 targets ATP2A2 to regulate cardiac function during heart failure [17]. However, there has never before been any research done on the relationship between miR-219a-5p and ATP2A2. Therefore, we intend to use a mouse model of acute myocardial infarction to explore the significance of this pathway.
We hypothesized that miR-219a-5p would alleviate MI in mice and may reduce the inflammatory response and apoptosis by targeting ATP2A2 and that miR-219a-5p would show potential as a possible therapeutic target for MI in our research. We suggest that the pathophysiology of MI may involve the miR-219a-5p/ATP2A2 axis, providing preliminary evidence for the identification of additional MI-targeted treatments.
Beijing Vital River Animal Co. Ltd. (Beijing, China) provided adult male BALB/c mice, weighing 23–27 g and 6–8 weeks of age. The mice were housed in a standard environment for 1 week before the experiment. The Zhongda Hospital Ethics Committee, affiliated with Southeast University, approved the protocol.
The mice were split into four groups of six mice each at random and were anesthetized via 3% pentobarbital sodium (80 mg/kg) and linked to the ventilator (110 bpm) followed by endotracheal intubation. Mice in the sham group received a 50 µL saline injection in the left ventricular apex, followed by thoracotomy without ligation. Mice in the MI group received a 50 µL saline injection in the left ventricle, followed by the establishment of MI. Mice in the MI + agomiR negative control (NC) group were injected with 50 µL agomiR NC (400 nmol/kg) in the left ventricular apex, and the MI model was established 1 day later. Mice in the MI + agomiR-219a-5p group were injected with 50 µL agomiR-219a-5p (400 nmol/kg) in the left ventricular apex, and the MI model was established 1 day later. The miRNA agomiR (RiboBio, Guangzhou, Guangdong, China) were chemically modified, double-stranded miRNA mimics with the same sequence as the miRNA of interest and with improved stability and transfection efficiency.
By clamping down on the left anterior descending (LAD) artery, the MI model was created [18]. The surgery was performed in the right lateral recumbent position. Using ophthalmic and micro scissors, the chest was sliced in the axilla of the left forelimb, between the third and fourth ribs, revealing the entire heart. The pericardial sac was cut with microsurgical forceps to fully expose the LAD artery. Then, LAD artery blood flow was completely blocked with a 7–0 needle suture. After ligation, the chest was completely closed with a 6-0 suture, and the muscles and skin were stitched from the innermost to the outermost layer.
Triphenyl tetrazolium chloride (TTC) staining
The left ventricular samples were taken once after MI establishment, washed with saline at a temperature of 4°C, dried, and frozen at −20°C for 15 min. The tissues were sliced into 1-mm-thick sections, followed by staining with 1% TTC (T8170, Solarbio, Beijing, China) for 15 min. After TTC staining, the normal area was red, the infarct area was white, and the edges were brick red. The infarct size was determined as a proportion of the total left ventricular mass utilizing Image ProPlus 5.0 (Media Cybernetics, Rockville, MD, USA).
Enzyme-linked immunosorbent assay (ELISA)
The supernatant of the serum and the left ventricular samples from each group were analyzed for markers of MI, including B-type natriuretic peptide (BNP) and cardiac troponin T (cTnT), as well as inflammatory factors, including interleukin-6 (IL-6), tumor necrosis factor-α (TNF-α), and interleukin-1β (IL-1β) following the ELISA kit manufacturer’s instructions. We bought the ELISA kits from Elabscience (E-EL-M0204c, E-EL-M1801c, E-EL-M0044c, E-EL-M3063, E-EL-M0037c; Wuhan, Hubei, China).
The high-frequency Vevo 2100 ultrasound system was used to analyze the cardiac structure (VisualSonics, Toronto, Canada). The mice were put under anesthesia and subjected to two-dimensional echocardiographic measurements. The results involved left ventricular ejection fraction (LVEF), left ventricular end-diastolic diameter (LVEDD), and left ventricular fractional shortening (LVFS), which were checked over three cardiac cycles.
Following the seeding of HEK293T cells into 96-well plates, 50 nM atomic miR-219a-5p/NC (RiboBio, Guangzhou, China) and 5 ng wild-type (WT)/mutant (MUT) ATP2A2 vectors were co-transfected with the aid of Lipofectamine 2000 (11668030, Thermo Fisher, Waltham, MA, USA). 24 h after transfection, the fluorescence activity of the cells was assessed through the use of a dual luciferase reporter assay (Promega, e1910, Madison, WI, USA).
Terminal deoxynucleotidyl transferase dUTP nick-end-labeling (TUNEL) assay
Apoptosis was measured in paraffin-embedded cardiac tissues by the TUNEL BrightRed Apoptosis Assay Kit (Vazyme, A113-01, Nanjing, Jiangsu, China) following the manufacturer’s instructions. After washing in phosphate-buffered saline, 4′,6-diamidino-2-phenylindole (DAPI) (Thermo Fisher, D1306) was used to counterstain the sections. The slides were examined by confocal microscopy (Zeiss, LSM700, Oberkochen, Germany). Both the total and apoptotic cell counts were determined. The ratio of apoptotic cells to total cells indicated the percentage of apoptotic cells.
Protein in the heart tissues from each group of mice was quantified using bicinchoninic acid protein quantitation kits (23227, Thermo Fisher) and isolated by 10% polyacrylamide gel electrophoresis. After the protein was transferred, 5% non-fat milk was used to seal the polyvinylidene fluoride membranes (Millipore, Burlington, MA, USA) for a duration of two hours at room temperature. This was followed by a 12-h incubation with primary antibodies against apoptosis-related proteins, inflammatory factors, and SERCA2a at 4°C. The membranes were treated with secondary antibodies for an hour at a temperature of 37°C. The bands were examined through a fluorescent Western blotting kit (K-12045-C20, Advansta, Menlo Park, CA, USA). The source of all antibodies, which included primary antibodies against total caspase-3 (1/2000, ab184787), IL-6 (1/1000, ab290735), Bcl-2 (1/2000, ab182858), cleaved caspase-3 (1/5000, ab214430), TNF-α (1/1000, ab183218), Bax (1/1000, ab32503), IL-1β (1/1000, ab254360), and GAPDH (1/8000, ab181602), as well as the secondary antibody (1/1000, ab96899), was Abcam (Cambridge, UK). The gray values were measured through ImageJ software 1.8.0 (National Institutes of Health, Bethesda, MD, USA), and the relative protein concentration was computed by dividing the target gray value by the GAPDH protein band’s gray value.
Quantitative real-time polymerase chain reaction (qRT-PCR)
Using Trizol (R411-01, Vazyme), total RNA was taken out of heart tissues and serum. RNA was converted into complementary DNA by reverse transcription utilizing the universal RT-PCR kit (RP1105, Solarbio, Beijing, China) and the TaqMan MicroRNA Reverse Transcription Kit (4366596, Thermo Fisher). Sangon Biotech (Shanghai, China) provided the miR-219a-5p, ATP2A2 mRNA, and U6 primers. U6 acted as an internal control to standardize the 2−ΔΔCT method, which was employed to quantify target gene expression. All primers were mouse, and the primer sequences are shown in Table 1.
The statistical analysis was conducted using SPSS 21.0 and GraphPad Prism 9.0.0 (GraphPad Software Inc., San Diego, CA, USA). The results are presented as the mean ± standard deviation. Pearson’s correlation coefficient was generated for the correlation analysis. Utilizing a one-way analysis of variance the information was contrasted among groups. The variation for the two groups was assessed using the t-test. p values less than 0.05 were regarded as statistically significant.
MiR-219a-5p was lowly expressed in the MI mouse model
MiRNA-seq data of GSE24591 was obtained from the Gene Expression Omnibus database. The data sample obtained from GSE24591 unveiled that miR-219a-5p had low expression in patients with MI (Fig. 1A). Therefore, we created mouse models of MI and divided them into four groups: sham, MI, MI + agomiR NC, and MI + agomiR-219a-5p (n = 6 per group). On days 1, 4, 7, and 14 after the MI model establishment, the level of miR-219a-5p decreased compared with the sham group in the serum (Fig. 1B). Similarly, in heart tissues, the MI group expressed less miR-219a-5p than the sham group did; however, the MI + agomir miR-219a-5p group showed significantly higher levels of miR-219a-5p when contrasted to the MI + agomir NC group (Fig. 1C). These outcomes revealed that miR-219a-5p expression was downregulated in MI.
Figure 1: MiR-219a-5p was lowly expressed in mouse models of MI. (A) The GSE24591 dataset from the GEO database performed the miR-219a-5p level. (B) On days 1, 4, 7, and 14, the level of miR-219a-5p in the serum was assessed through qRT-PCR (n = 6 mice per group). (C) The relative expression of miR-219a-5p was measured by qRT-PCR in the heart tissues of mice in each group (n = 6 mice per group). *p < 0.05, ***p < 0.001.
MI was notably impaired by miR-219a-5p overexpression
The TTC staining results showed that in the MI + agomiR-219a-5p group, the infarction area was smaller than it was in the MI + agomiR NC group (Fig. 2A). This result was reflected in the myocardial index changes, where the MI + agomiR-219a-5p group had lower BNP, heart weight/body weight ratio (HW/BW), and cTnT than the MI + agomiR NC group (Fig. 2B–D). As shown on echocardiography, the LVEDD of the MI + agomiR-219a-5p group was smaller than the one of the MI + agomiR NC group, easing the trend of ventricular enlargement. Meanwhile, LVEF and LVFS changes in the MI + agomiR-219a-5p group showed that left ventricular systolic function remained within the normal range even after establishing MI (Fig. 2E). These outcomes revealed that miR-219a-5p overexpression notably relieved MI.
Figure 2: MiR-219a-5p improved MI in mice. (A) Transverse sections of TTC-stained hearts from the four groups of mice and the analysis of the infarcted area. The red arrows represent the area of myocardial infarction. (B) ELISA was applied to determine the amount of BNP in the serum of the mice from each of the four groups. (C) The HW/BW of the four groups is shown. (D) ELISA was performed to assess the content of troponin T in the serum of the mice in each of the groups. (E) LVEDD, LVEF, and LVFS were measured by echocardiography. Scale bar = 1 mm. n = 6 per group. ***p < 0.001.
MiR-219a-5p protected the myocardium by controlling apoptosis
We assessed agomiR-219a-5p’s function in MI-affected mice. The proteins linked to apoptosis were evaluated in each of the four groups using western blotting. Bcl-2 was remarkably upregulated by overexpression of miR-219a-5p, suppressing cytotoxin-induced death of cardiomyocytes. Bax and cleaved caspase-3 were downregulated after miR-219a-5p elevation, suppressing procedural apoptosis of cardiomyocytes (Fig. 3A). In addition, TUNEL staining showed that after the overexpression of miR-219a-5p in cardiomyocytes, the rate of positive apoptotic cells decreased significantly (Fig. 3B). These outcomes implied that miR-219a-5p regulated apoptosis to protect the myocardium.
Figure 3: MiR-219a-5p reduced apoptosis in heart tissues from mice with MI. (A) The expression of apoptosis-related proteins (cleaved caspase-3, total caspase-3, Bax, and Bcl-2) in heart tissues from mice in the four groups were assessed through Western blotting. (B) The TUNEL assay was carried out to measure the apoptosis rate of cardiomyocytes in the four groups. n = 6 per group. Scale bar = 40 µm. ***p < 0.001, ****p < 0.0001.
The inflammatory response was reduced by miR-219a-5p in mice with MI
We explored how miR-219a-5p impacted the inflammatory response in mice with MI. Western blotting was used to quantify the levels of inflammatory factors (IL-6, TNF-α, and IL-1β) in cardiac tissues. Compared with the sham group, the MI group showed that all of these inflammatory factors had substantially greater levels. The level of these inflammatory markers was downregulated in the MI + agomir-219a-5p group, with levels comparable to the sham group, when contrasting the MI + agomiR NC group to the MI + agomiR-219a-5p group (Fig. 4A). The serum level of these inflammatory factors was also measured through ELISA. Consistent with the Western blotting results, ELISA also demonstrated that the inflammatory factors concentration in the MI group was higher than that in the sham group, and the inflammatory factors concentration in the MI + agomir-219a-5p group showed lower levels compared with the MI + agomir NC group (Fig. 4B).
Figure 4: MiR-219a-5p reduced inflammation in the heart tissues of mice with MI. (A) Western blotting of inflammatory factors and protein quantification of these factors in heart tissues from the four groups of mice. (B) Serum concentrations of the inflammatory factors were measured through ELISA. n = 6. *p < 0.05, **p < 0.01, ***p < 0.001.
ATP2A2 mRNA and SERCA2a were directly targeted by miR-219a-5p
The ATP2A2 mRNA and SERCA2a protein expression was elevated in MI-affected mice, while it was downregulated in the MI + agomiR-219a-5p group according to qRT-PCR and Western blotting (Fig. 5A). Thus, we postulated that miR-219a-5p can control the expression of ATP2A2. Utilizing bioinformatics software, the targeted connection between miR-219a-5p and ATP2A2 was examined. TargetScan (https://www.targetscan.org/vert_72/, accessed on 10 May 2024) projected that miR-219a-5p and ATP2A2 would bind together (Fig. 5B). The results of relative luciferase activity demonstrated that overexpression of miR-219a-5p inhibited ATP2A2-WT luciferase activity (Fig. 5C). Pearson’s correlation analysis revealed that miR-219a-5p was negatively related to ATP2A2 expression in the MI group (p < 0.05) (Fig. 5D). The above findings indicated that miR-219a-5p could target ATP2A2.
Figure 5: MiR-219a-5p targeted 3’UTR of ATP2A2. (A) The relative expression of ATP2A2 in the four groups was determined using qRT-PCR and Western blotting, and protein quantification of SERCA2a (n = 6). (B) Bioinformatics software identified the binding sites between ATP2A2 and miR-219a-5p. (C) In HEK293T cells co-transfected with agomiR-219a-5p or NC (n = 3) and WT or MUT locations of the 3′ UTR of ATP2A2, luciferase activity was observed. (D) In the MI group Pearson’s correlation analysis between ATP2A2 and miR-219a-5p (n = 6). ***p < 0.001.
MI is an ischemic heart disease in which cardiomyocytes become damaged and die because of local blood supply interruption in the heart. The high mortality and incidence rates of MI are major global health concerns [19,20].
Numerous investigations have demonstrated the significant regulatory functions miRNAs play in the pathophysiology of MI, especially cell apoptosis [21,22]. Microarray analyses of cardiac samples from patients with MI [23,24] have shown that miR-106b suppresses cardiomyocyte apoptosis through suppression of p21 expression [25]. There is also a report indicating that in models of ischemia or anoxia, miR-21 and miR-146a together repress cardiomyocyte apoptosis. The combined injection of agomiR-21 and agomiR-146a decreased the infarction area and increased LVEF and LVFS to normal values [26]. Previous studies have also shown that inflammation and apoptosis are the major factors underpinning cardiomyocyte dysfunction [27–29]; therefore, they may be beneficial targets for MI treatment. In our study, we assessed the effect of miR-219a-5p on the inflammatory response and apoptosis. We found that miR-219a-5p overexpression suppressed the inflammatory response and cardiomyocyte apoptosis in mouse models of MI, protecting the myocardial tissues from MI and promoting cardiac function.
ATP2A2 encodes SERCA2a, which facilitates the contraction of cytoplasmic centroid myocytes and the uptake of calcium back into the sarcoplasmic reticulum [30]. Cardiac contractility and relaxation are governed by SERCA2a activity, which has an impact on cardiac function [31,32]. Following MI, one of the main factors of cardiac cell death is an imbalance in calcium homeostasis, and the SERCA2a protein is crucial for controlling this homeostasis. Furthermore, ATP2A2 gene treatment has just lately become a viable therapeutic alternative [33,34]. Our results found that ATP2A2 served as the target gene of miR-219a-5p. MiR-219a-5p protected the myocardial tissue from MI by sponging its target gene ATP2A2. Consequently, we speculate that miR-219a-5p may affect the calcium homeostasis of cardiomyocytes by targeting ATP2A2, thereby regulating cardiomyocyte apoptosis and the inflammatory response. However, the control of this process involves numerous signaling channels, necessitating further exploration in the future. This finding increases the understanding of miRNAs in the context of MI and has the potential to be used in the future for the treatment of patients with MI. However, to validate the results, further clinical analysis and additional samples are needed to measure the function and mechanism of miR-219a-5p in MI.
In addition, miR-219a can target and regulate a variety of mRNAs in the cardiac field. The starBase database predicts the interaction between calcium/calmodulin-dependent kinase II delta (CAMK2D) and miR-219a, and a study showed that the cardiac myosin light chain is phosphorylated by CAMK2D for the treatment of heart failure [35]. The miR-219a/melanoma 2/Toll-like receptor 4/myeloid differentiation factor 88 axis regulates cardiomyocyte apoptosis in myocardial ischemia–reperfusion injury [36]. This evidence suggests that miR-219a not only targets ATP2A2 but it also participates in related pathways in cardiomyocytes and is widely regulated. Therefore, in the future, we must take into account miR-219a’s adjuvant and compensating effects in the regulation of MI.
MiR-219a-5p suppressed the inflammatory response and cardiomyocyte apoptosis by directly inhibiting the expression of ATP2A2 to relieve MI in mice, thus protecting cardiac function. These outcomes suggest that miR-219a-5p may represent a novel MI-regulating component. However, whether this regulatory network of miR-219a-5p in MI is appropriate for clinical use still needs further exploration.
Acknowledgement: None.
Funding Statement: This work was supported by the National Nature Science Foundation of the People’s Republic of China (No. 81400225 for Zulong Sheng and No. 82000382 for Yanru He) and the Jiangsu Provincial Medical Youth Talent (No. QNRC2016815).
Author Contributions: Study conception and design, draft manuscript preparation, manuscript revision: Zulong Sheng; Data collection: Yuqin Ji; Techninical support and supervision of the project: Yuyu Yao, Genshan Ma; Analysis and interpretation of results: Yanru He, Junyan Cai. All authors reviewed the results and approved the final version of the manuscript.
Availability of Data and Materials: The datasets generated and/or analyzed during the current study are available from the corresponding author upon reasonable request.
Ethics Approval: The experiments conformed to the Guidelines for the Care and Use of Laboratory Animals published by the US National Institutes of Health and were performed under the approval of the Zhongda Hospital Ethics Committee, Affiliated to Southeast University (2019ZDSYLL201-P01).
Conflicts of Interest: The authors declare that they have no conflicts of interest to report regarding the present study.
References
1. Koeda Y, Itoh T, Ishikawa Y, Morino Y, Mizutani T, Ako J, et al. A multicenter study on the clinical characteristics and risk factors of in-hospital mortality in patients with mechanical complications following acute myocardial infarction. Heart Vessels. 2020;35(8):1060–9. doi:10.1007/s00380-020-01586-0. [Google Scholar] [PubMed] [CrossRef]
2. Liu X, Wang L, Wang Y, Qiao X, Chen N, Liu F, et al. Myocardial infarction complexity: a multi-omics approach. Clinica Chimica Act. 2024;552:117680. doi:10.1016/j.cca.2023.117680. [Google Scholar] [PubMed] [CrossRef]
3. Shahid M, Rehman K, Akash MSH, Suhail S, Rasheed S, Imran M, et al. Biochemical association between the prevalence of genetic polymorphism and myocardial infarction. BIOCELL. 2023;47(3):473–84. doi:10.32604/biocell.2023.025930. [Google Scholar] [CrossRef]
4. Toma Y. How to bail out patients with severe acute myocardial infarction. Heart Fail Clin. 2020;16(2):177–86. doi:10.1016/j.hfc.2019.12.004. [Google Scholar] [PubMed] [CrossRef]
5. Campbell KH, Tweet MS. Coronary disease in pregnancy: myocardial infarction and spontaneous coronary artery dissection. Clin Obstet Gynecol. 2020;63(4):852–67. doi:10.1097/GRF.0000000000000558. [Google Scholar] [PubMed] [CrossRef]
6. Chatterjee B, Sarkar M, Bose S, Alam MT, Chaudhary AA, Dixit AK, et al. MicroRNAs: key modulators of inflammation-associated diseases. Semin Cell Dev Biol. 2024;154:364–73. doi:10.1016/j.semcdb.2023.01.009. [Google Scholar] [PubMed] [CrossRef]
7. Colpaert RMW, Calore M. Epigenetics and microRNAs in cardiovascular diseases. Genomics. 2021;113(2):540–51. doi:10.1016/j.ygeno.2020.12.042. [Google Scholar] [PubMed] [CrossRef]
8. Verjans R, Peters T, Beaumont FJ, Van Leeuwen R, Van Herwaarden T, Verhesen W, et al. MicroRNA-221/222 family counteracts myocardial fibrosis in pressure overload-induced heart failure. Hypertension. 2018;71(2):280–8. doi:10.1161/HYPERTENSIONAHA.117.10094. [Google Scholar] [PubMed] [CrossRef]
9. Chen Y, Zhao Y, Chen W, Xie L, Zhao ZA, Yang J, et al. MicroRNA-133 overexpression promotes the therapeutic efficacy of mesenchymal stem cells on acute myocardial infarction. Stem Cell Res Ther. 2017;8(1):268. doi:10.1186/s13287-017-0722-z. [Google Scholar] [PubMed] [CrossRef]
10. Searles CD. MicroRNAs and cardiovascular disease risk. Curr Cardiol Rep. 2024;26(2):51–60. doi:10.1007/s11886-023-02014-1. [Google Scholar] [PubMed] [CrossRef]
11. Shah AM, Giacca M. Small non-coding RNA therapeutics for cardiovascular disease. Eur Heart J. 2022;43(43):4548–61. doi:10.1093/eurheartj/ehac463. [Google Scholar] [PubMed] [CrossRef]
12. Brandi R, Fabiano M, Giorgi C, Arisi I, La Regina F, Malerba F, et al. Nerve growth factor neutralization promotes oligodendrogenesis by increasing miR-219a-5p levels. Cells. 2021;10(2):405. doi:10.3390/cells10020405. [Google Scholar] [PubMed] [CrossRef]
13. Zhang P, Li J. Down-regulation of circular RNA hsa_circ_0007534 suppresses cell growth by regulating miR-219a-5p/SOX5 axis in osteosarcoma. J Bone Oncol. 2021;27:100349. doi:10.1016/j.jbo.2021.100349. [Google Scholar] [PubMed] [CrossRef]
14. Shi Y, Dai S, Qiu C, Wang T, Zhou Y, Xue C, et al. MicroRNA-219a-5p suppresses intestinal inflammation through inhibiting Th1/Th17-mediated immune responses in inflammatory bowel disease. Mucosal Immunol. 2020;13(2):303–12. doi:10.1038/s41385-019-0216-7. [Google Scholar] [PubMed] [CrossRef]
15. Ntari L, Mantzouratou P, Katsaouni A, Pantos C, Kollias G, Mourouzis I. Changes in thyroid hormone signaling mediate cardiac dysfunction in the Tg197 mouse model of arthritis: potential therapeutic implications. J Clin Med. 2021;10(23):5512. doi:10.3390/jcm10235512. [Google Scholar] [PubMed] [CrossRef]
16. Feng YL, Ke T, Wang GL, Qi HY, Xiao Y. MicroRNA-200c-3p negatively regulates ATP2A2 and promotes the progression of papillary thyroid carcinoma. Biochem Genet. 2022;60(5):1676–94. doi:10.1007/s10528-022-10184-w. [Google Scholar] [PubMed] [CrossRef]
17. Wahlquist C, Jeong D, Rojas-Muñoz A, Kho C, Lee A, Mitsuyama S, et al. Inhibition of miR-25 improves cardiac contractility in the failing heart. Nature. 2014;508(7497):531–5. doi:10.1038/nature13073. [Google Scholar] [PubMed] [CrossRef]
18. Muthuramu I, Lox M, Jacobs F, De Geest B. Permanent ligation of the left anterior descending coronary artery in mice: a model of post-myocardial infarction remodelling and heart failure. J Vis Exp. 2014;(94):52206. [Google Scholar]
19. Krittanawong C, Khawaja M, Tamis-Holland JE, Girotra S, Rao SV. Acute myocardial infarction: etiologies and mimickers in young patients. J Am Heart Assoc. 2023;12(18):e029971. doi:10.1161/JAHA.123.029971. [Google Scholar] [PubMed] [CrossRef]
20. Avolio E, Campagnolo P, Katare R, Madeddu P. The role of cardiac pericytes in health and disease: therapeutic targets for myocardial infarction. Nat Rev Cardiol. 2024;21(2):106–18. doi:10.1038/s41569-023-00913-y. [Google Scholar] [PubMed] [CrossRef]
21. Cruz MS, da Silva AMG, de Souza KSC, Luchessi AD, Silbiger VN. miRNAs emerge as circulating biomarkers of post-myocardial infarction heart failure. Heart Fail Rev. 2020;25(2):321–9. doi:10.1007/s10741-019-09821-1. [Google Scholar] [PubMed] [CrossRef]
22. Zhan C, Liu K, Zhang Y, Zhang Y, He M, Wu R, et al. Myocardial infarction unveiled: key miRNA players screened by a novel lncRNA-miRNA-mRNA network model. Comput Biol Med. 2023;160:106987. doi:10.1016/j.compbiomed.2023.106987. [Google Scholar] [PubMed] [CrossRef]
23. Zhou J, He S, Wang B, Yang W, Zheng Y, Jiang S, et al. Construction and bioinformatics analysis of circRNA-miRNA-mRNA network in acute myocardial infarction. Front Genet. 2022;13:854993. doi:10.3389/fgene.2022.854993. [Google Scholar] [PubMed] [CrossRef]
24. Bostjancic E, Zidar N, Glavac D. MicroRNA microarray expression profiling in human myocardial infarction. Dis Markers. 2009;27(6):255–68. doi:10.1155/2009/641082. [Google Scholar] [CrossRef]
25. Liu Z, Yang D, Xie P, Ren G, Sun G, Zeng X, et al. MiR-106b and MiR-15b modulate apoptosis and angiogenesis in myocardial infarction. Cell Physiol Biochem. 2012;29(5–6):851–62. [Google Scholar] [PubMed]
26. Huang W, Tian SS, Hang PZ, Sun C, Guo J, Du ZM. Combination of microRNA-21 and microRNA-146a attenuates cardiac dysfunction and apoptosis during acute myocardial infarction in mice. Mol Ther Nucleic Acids. 2016;5(3):e296. [Google Scholar] [PubMed]
27. Liu J, Wang H, Li J. Inflammation and inflammatory cells in myocardial infarction and reperfusion injury: a double-edged sword. Clin Med Insights Cardiol. 2016;10:79–84. [Google Scholar] [PubMed]
28. Luo M, Yan D, Sun Q, Tao J, Xu L, Sun H, et al. Ginsenoside Rg1 attenuates cardiomyocyte apoptosis and inflammation via the TLR4/NF-kB/NLRP3 pathway. J Cell Biochem. 2020;121(4):2994–3004. doi:10.1002/jcb.v121.4. [Google Scholar] [CrossRef]
29. Zhang Q, Wang L, Wang S, Cheng H, Xu L, Pei G, et al. Signaling pathways and targeted therapy for myocardial infarction. Signal Transduct Target Ther. 2022;7(1):78. doi:10.1038/s41392-022-00925-z. [Google Scholar] [PubMed] [CrossRef]
30. Le Quilliec E, LeBlanc CA, Neuilly O, Xiao J, Younes R, Altuntas Y, et al. Atrial cardiomyocytes contribute to the inflammatory status associated with atrial fibrillation in right heart disease. Europace. 2024;26(4):euae082. doi:10.1093/europace/euae082. [Google Scholar] [PubMed] [CrossRef]
31. Zou Y, Li L, Li Y, Chen S, Xie X, Jin X, et al. Restoring cardiac functions after myocardial infarction-ischemia/reperfusion via an exosome anchoring conductive hydrogel. ACS Appl Mater Interfaces. 2021;13(48):56892–908. doi:10.1021/acsami.1c16481. [Google Scholar] [PubMed] [CrossRef]
32. Wang W, Tayier B, Guan L, Yan F, Mu Y. Pre-transplantation of bone marrow mesenchymal stem cells amplifies the therapeutic effect of ultrasound-targeted microbubble destruction-mediated localized combined gene therapy in post-myocardial infarction heart failure rats. Ultrasound Med Biol. 2022;48(5):830–45. doi:10.1016/j.ultrasmedbio.2022.01.004. [Google Scholar] [PubMed] [CrossRef]
33. Zhang Y, Jiao L, Sun L, Li Y, Gao Y, Xu C, et al. LncRNA ZFAS1 as a SERCA2a inhibitor to cause intracellular Ca2+ overload and contractile dysfunction in a mouse model of myocardial infarction. Circ Res. 2018;122(10):1354–68. doi:10.1161/CIRCRESAHA.117.312117. [Google Scholar] [PubMed] [CrossRef]
34. Liu ZH, Ni JY, Li L, Michael S, Guo R, Bian XY, et al. SERCA2a: a key protein in the Ca2+ cycle of the heart failure. Heart Fail Rev. 2020;25(3):523–35. doi:10.1007/s10741-019-09873-3. [Google Scholar] [PubMed] [CrossRef]
35. Chang AN, Mahajan P, Knapp S, Barton H, Sweeney HL, Kamm KE, et al. Cardiac myosin light chain is phosphorylated by Ca2+/calmodulin-dependent and-independent kinase activities. Proc Natl Acad Sci U S A. 2016;113(27):E3824–33. [Google Scholar] [PubMed]
36. Li Y, Xing N, Yuan J, Yang J. Sevoflurane attenuates cardiomyocyte apoptosis by mediating the miR-219a/AIM2/TLR4/MyD88 axis in myocardial ischemia/reperfusion injury in mice. Cell Cycle. 2020;19(13):1665–76. doi:10.1080/15384101.2020.1765512. [Google Scholar] [PubMed] [CrossRef]
Cite This Article
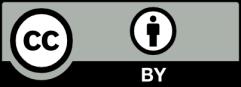
This work is licensed under a Creative Commons Attribution 4.0 International License , which permits unrestricted use, distribution, and reproduction in any medium, provided the original work is properly cited.