Open Access
ARTICLE
Blueberry anthocyanins extract attenuates oxidative stress and angiogenesis on an in vitro high glucose-induced retinopathy model through the miR-33/GLCCI1 axis
1 Department of Ophthalmology, The Second Affiliated Hospital, Jiangxi Medical College, Nanchang University, Nanchang, 330000, China
2 Department of Ophthalmology in Ocular Fundus Diseases, Affiliated Eye Hospital of Nanchang University, Nanchang, 330000, China
3 Department of Ophthalmic Treatment of Refractive Errors, Affiliated Eye Hospital of Nanchang University, Nanchang, 330000, China
* Corresponding Author: ZHIPENG YOU. Email:
BIOCELL 2024, 48(8), 1275-1284. https://doi.org/10.32604/biocell.2024.051045
Received 26 February 2024; Accepted 27 May 2024; Issue published 02 August 2024
Abstract
Background: Diabetes retinopathy (DR) is a complication of diabetes that affects patients’ vision. Previous studies have found blueberry anthocyanins extract (BAE) can inhibit the progression of DR, but its mechanism is not completely clear. Methods: To study the role of BAE in diabetes retinopathy, we treated human retinal endothelial cells (HRCECs) with 30 mM high glucose to simulate the microenvironment of diabetes retinopathy and used BAE to intervene the in vitro high glucose-induced retinopathy model. HRCEC cell viability and apoptosis rates were examined by Cell Counting Kit 8 (CCK-8) assay and flow cytometry assay. The binding sites between miR-33 and glucocorticoid-induced transcript 1 (GLCCI1) were assessed by luciferase reporter assay. Retinal neovascularization and oxidative stress contribute to diabetic retinopathy. The tubule formation assay was applied to detect the retinal neovascularization. The oxidative stress in the HRCECs was manifested by the reactive oxygen species (ROS) level, the malondialdehyde (MDA) level, and the superoxide dismutase (SOD) activity. Results: Compared with HRCECs cells cultured under normal conditions, high glucose (HG) can induce oxidative stress in HRCRCs, specifically manifested in the increase of ROS and MDA levels, and the decrease of SOD activity. BAE relieved the tubule formation in n the HRCEC. BAE also relieved the ROS and MDA levels and increased the SOD activity. Luciferase reporter assay revealed that GLCCI1 is a target molecule downstream of miR-33. In HRCEC, BAE significantly inhibited the expression of miR-33 induced by HG. miR-33 mimic inhibited the BAE’s effects on oxidative stress and angiogenesis in an in vitro high glucose-induced retinopathy model. Conclusion: BAE alleviated the oxidative stress and microangiogenesis of HRCEC by regulating the miR-33 /GLCCI1 axis.Keywords
Abbreviations
BAE | Blueberry anthocyanin extract |
BCA | Bicinchoninic acid |
DR | Diabetic retinopathy |
GLCCI1 | Glucocorticoid-induced transcript 1 |
HG | High glucose |
HRCEC | Human retinal capillary endothelial cells |
MDA | Malondialdehyde |
qRT-PCR | Quantitative real-time PCR |
ROS | Reactive oxygen species |
SOD | Superoxide dismutase |
WB | Western blot |
Diabetic retinopathy (DR) is categorized as one of the most serious microvasculature complications in early diabetes mellitus patients [1]. The incidence rate of DR has increased significantly in China year by year [2]. The prevalence rates of DR and vision-threatening DR were 16.3% and 3.2% in China [3]. In a multi-ethnic US cohort, approximately one in five participants with diabetes developed DR over 8 years [4]. DR causes serious damage to vision, and it has become one of the leading causes of blindness among working-aged adults around the world [5]. The build-up of reactive oxygen species (ROS) and neovascularization due to hyperglycemia are recognized as a primary risk factor for DR [6,7]. Reactive oxygen species (ROS) cause oxidative stres6s, and oxidative stress causes the disruption of the retinopathy endothelial cells membrane integrity and leads to DR [8–10]. A Disintegrin and Metalloproteinase with Thrombospondin motifs 5 (ADAMTS5) promotes proliferative diabetic retinopathy via neovascularization [11]. Therefore, the prevention of oxidative stress and neovascularization in patients with diabetes can delay the occurrence and development of retinopathy.
Anthocyanins are a kind of water-soluble pigment, mainly synthetic in plant cytosol [12]. It has a better therapeutic effect on diabetic cataract disease, glaucoma, and other ophthalmic diseases [13,14]. A meta-analysis shows that a 7.5 mg/day increment of dietary anthocyanin intake decreased the risk of T2DM. Higher intakes of dietary anthocyanins are associated with a lower T2DM risk [15]. Anthocyanins can prevent and treat DM, based on their effects on glucose metabolism, antioxidant, and anti-inflammatory through various mechanisms. Anthocyanins also have therapeutic effects in treating diabetic retinopathy [16]. Anthocyanin C3G suppressed the angiogenesis of human retinal endothelial cells (HRECs) [7]. Blueberries are known as the king of anthocyanins in all plants. They are extremely rich in anthocyanins (about 300 mg of anthocyanins are found in 100 g of blueberries) and have high development and utilization value [17]. Blueberry anthocyanins (BAE) have a great anti-diabetic effect manifested in the decrease of blood glucose via the increase of AMPK activity [18]. Several studies have shown that blueberry anthocyanins (BAE) have significant therapeutic effects on oxidative stress and inflammation in diabetic retinopathy [19,20]. Besides, BAE has been used in the treatment of DR in Europe with good efficacy [21]. However, the underlying mechanism of BAE in alleviating DR is still not known.
In this study, the human retinal endothelial cells (HRCECs) were processed with 30 mM high glucose to simulate the microenvironment of diabetes retinopathy. HRCECs tubule formation and the oxidative stress indexes were investigated in normal or HG-treated HRCECs. BAE was employed in an in vitro high glucose-induced retinopathy model. In the present research, the protective effects of BAE on oxidative stress and angiogenesis induced by high glucose in HRCECs were investigated to propose a preliminary mechanism for the role of antioxidants in eye nourishment.
Human Retinal Capillary Endothelial Cells (HRCECs) were acquired from the China Center for Type Culture Collection (Wuhan, China) and were cultured in high glucose Dulbecco’s Modified Eagle’s Medium (DMEM) (Gibco, 11965092, Grand Island, New York, USA) supplied with 10% Fetal Bovine Serum (FBS) (Sijiqing, 70220-8611, Hangzhou, Zhejiang, China) and 1% penicillin-streptomycin solution (Sangon-Biotech, E607011, Shanghai, China). HRCECs were incubated at 37°C in 5% CO2.
HRCECs were implanted into the 6 well plates and then processed with 10 μg/mL BAE for 24 h. Then, the HRCECs were processed with 30 mM high glucose or 5.5 mM normal glucose medium. Cells were processed with 30 mM glucose for 48 h to simulate the DR microenvironment [20]. The blueberry anthocyanins extract (BAE) was purchased from the Daxinganling Lingonberry Organic Foodstuffs Co., Ltd. (Daxinganling, China).
For cell experiments, the cells were randomly divided into 4, 5, or 6 groups. 4 groups: Control group, high glucose (HG) group, BAE group, HG + BAE group. 5 groups: Control group, HG group, HG + BAE group, HG + BAE + mimic NC group, HG + BAE + miR-33 group. 6 groups: Control group, HG group, HG + BAE group, HG + BAE + mimic NC + pcDNA-NC group, HG + BAE + miR-33 + pcDNA-NC group, HG + BAE + miR-33 + pcDNA-GLCCI1 group.
The miR-33 mimic and miR-33 inhibitor were purchased from Gene Pharm (Shanghai, China), and their sequences were as follows: hsa-miR-33a mimic: GUGCAUUGUAGUUGCAUUGCA, hsa-miR-33a inhibitor: UGCAAUGCAACUACAAUGC; The mimic NC and inhibitor NC were served as control. Cells were planted on 6-well plates for 24 h. Then the miR-33 mimic (or miR-33 inhibitor) and Lipofectamine™ 3000 (Invitrogen, L3000015, Carlsbad, CA, USA) were respectively incubated in OPTI-MEM reduced serum medium (Invitrogen, 31985070, Carlsbad, CA, USA) for 20 min. The mixture of miR-33 mimic (or miR-33 inhibitor) and Lipofectamine™ 3000 was transfected to the cells in the plate. The cells were replaced with the normal glucose medium 5 h later. 48 h later, the cells on the plates were harvested for further study. For GLCCI1 plasmid transfection, cells were transfected with a mixture of GLCCI1 plasmid and Lipofectamine™ 3000 in OPTI-MEM reduced serum medium.
Cell viability was detected using the CCK-8 kit (Yeasen, 40203ES60, Shanghai, China). 5 × 103 HRCECs were seeded into one cell of the 96 well plates and cultured for 24 h. 24 h later, the cells in each well were added 10 μL CCK-8 reagent for 4 h incubation in 5% CO2. After 4 h incubation, the optical densities (OD) at 450 nm were measured by a microplate reader (Beckman coulter, DTX 880, Brea, CA, USA).
FITC Annexin V Apoptosis Detection Kit I (BD, No. 556547, Franklin Lakes, New York, USA) was used to detect apoptosis in HRCECs. HRCECs were collected when they reached the logarithmic growth phase. 1 × 105 HRCECs were suspensed with 100 μl Binding Buffer. Next, 5 μL of Annexin-V and 5 μL of Propidium Iodide (PI) were added to the buffer and incubated at room temperature for 15 min in the darkness for Accuri C6 flow cytometer (BD Biosciences, USA) analysis.
Quantitative real-time PCR (qRT-PCR)
RNA was isolated from HRCECs with TRIzol™ reagent (Invitrogen, 15596026CN, Carlsbad, CA, USA). 1 μg isolated RNA was prepared using Prime Script™ RT reagent kit (Takara, RR037A, Shiga, Japan) or Prime Script™ miRNA RT-PCR Kit (Takara, RR716, Shiga, Japan). TB Green® Premix Ex Taq™ II (Takara, RR820Q, Shiga, Japan) was used for real-time PCR, which was run on Step One Plus™ Real-Time PCR System (Applied Biosystems, 4376600, Foster City, CA, USA). Relative gene expression was normalized with GAPDH or U6 and calculated with the 2−ΔΔCT method. The sequences of the primers used in this study are listed in Table 1.
The total protein content was extracted from HRCECs with RIPA lysis buffer (Beyotime, P0013B, Shanghai, China) and quantified. 30 μg was loaded in the concentration gel and resolved by separation gel. The objective protein was transferred to the polyvinylidene difluoride membrane (Millipore, ISEQ10100, Boston, MA, USA). Next, the membrane was blocked with 5% skim milk for 2 h. The membranes were incubated with primary antibodies anti-GLCCI1 (Abcam, ab107491, Cambridge, UK), and GAPDH (Abcam, ab181602, Cambridge, UK) at 4°C overnight. Subsequently, the membranes were washed with Tris-buffered saline tween (TBST) three times and then incubated with Goat Anti-Rabbit IgG (H + L) HRP (Abways, AB0101, Shanghai, China) at room temperature for 2 h. GAPDH served as an internal control. The protein band was visualized with an enhanced chemiluminescent detection kit (NCM Biotech, P2300, Suzhou, China) and analyzed by ImageJ software (Bio-Rad, Image Lab 6.1, Hercules, CA, USA).
Reactive oxygen species (ROS) detection
Reactive oxygen species (ROS) generation in HRCECs was examined with the Reactive Oxygen Species Assay Kit (Beyotime, S0033s, Shanghai, China). 1 × 105 HECRCs were seeded into one well of 6 well plates and then stained with 10 μM 2,7-Dichlorodi-hydro fluorescein diacetate (DCFH-DA) probes at 37°C in darkness for 30 min. The fluorescence intensity of ROS was measured at Ex/Em = 488/525 nm wavelength by fluorescence microscope (Nikon, Ts2R-FL, Tokyo, Japan).
Measurement of malondialdehyde (MDA) level and super oxide dismutase (SOD) activity in cells
The MDA level and SOD activity were detected with a Malondialdehyde (MDA) assay kit (Nanjing Jiancheng, A003-1, Nanjing, China) and Superoxide Dismutase Activity Assay kit (amyjet, STA-340, Wuhan, China) according to the manufacturer’s instructions.
We added 250 μL Matrigel (Corning, 354234, Corning, New York, USA) to pre-cooled 24-well plates for polymerization at 37°C for 60 min. A total of 1 × 105 treated HRCEC cells were plated onto the Matrigel matrix. After incubation at 37°C 48 h, the formation of tubes was counted in 6 random microscopic fields with a computer-assisted microscope (Olympus, IXplore Pro, Tokyo, Japan) and quantified using ImageJ software (Bio-Rad, Image Lab 6.1, Hercules, CA, USA).
Dual-luciferase reporter assay
Dual-luciferase reporter assay was carried out with the method in this article [22]. Briefly, the wild-type (wt) GLCCI1 3′UTR mRNA and mutated (mut) GLCCI1 3′UTR mRNA was inserted into the luciferase pmir-GLO reporter vector. Cells were co-transfected with either wt GLCCI1 or mut GLCCI1 luciferase pmir-GLO reporter vector plus NC mimic (miR-33a-5p mimic) or NC mimic (miR-33b-5p mimic) by Lipofectamin™ 3000 (Invitrogen, L3000015, Carlsbad, CA, USA). After 48 h, cells were harvested and the luciferase activities were measured using Beyotime’s Dual-Lumi™ Luciferase Assay System (Beyotime, RG088S, Shanghai, China).
The two groups’ differences were analyzed using an unpaired two-tailed Student’s t-test. If there were more than two groups, one-way ANOVA or two-way ANOVA with Tukey’s post hoc analysis was used. GraphPad Prism 9.0 software (GraphPad Software, Inc., La Jolla, CA, USA) was used for statistical analysis. All values are presented as mean ± standard deviation (SD). p values < 0.05 was considered statistically significant. Each assay was performed at least 3 times.
BAE relieved oxidative stress and angiogenesis of HG-treated HRCECs
To test the potential of BAE in alleviating DR, HRCECs were first treated with HG to simulate the DR microenvironment. Based on the findings of Huang et al., 10 mg/L BAE was selected as the optimal concentration for our subsequent experiments [20]. Proanthocyanidins inhibit the miR-33 expression in obese rats [23]. miR-33 has emerged as a drug target for a variety of metabolic diseases [24]. BAE partially inhibited the miR-33 expression increased by high-glucose treatment (Fig. 1A). The GLCCI1 level in high-glucose treated HRCECs cells was lower than the GLCCI1 in the control group, but the result was reversed after adding BAE (Fig. 1B,C). Moreover, the oxidative stress indexes in HRCECs were evaluated. Results revealed that ROS level was elevated in HG-treated HRCECs, which was abolished by BAE treatment (Fig. 1D,E). Similarly, BAE treatment reversed HG effects on the SOD activity and the MDA levels in HRCECs (Fig. 1F,G). Furthermore, the influence of BAE on cell apoptosis, cell viability, and angiogenesis of HRCECs was evaluated. As shown in Fig. 1H,I, HG treatment down-regulated the apoptosis rate of HRCECs, while BAE restored that. CCK-8 and tube formation assay results showed cell viability and angiogenesis of HRCECs were elevated by HG treatment. BAE treatment significantly reduced the proliferation and angiogenesis of HG-treated HRCECs (Fig. 1J–L). According to these data, BAE relieved oxidative stress and angiogenesis in HG-treated HRCECs.
Figure 1: BAE relieved oxidative stress and angiogenesis of HG-treated HRCECs. (A) HRCECs were examined by qRT-PCR for miR-33 expression. (B–C) qRT-PCR and WB were used to examine the GLCCI1 expression in HRCECs. (D–E) DCFH-DA staining was used to examine the levels of ROS in HRCECs. (F–G) The SOD activity and the level of MDA in HRCECs were tested. (H–I) Annexin V-FITC/PI was applied to examine apoptosis in HRCECs. (J) HRCECs cell viability was assessed by CCK-8 assay. (K–L) The angiogenesis of HRCECs was examined by the tube formation assay. **p < 0.01, ***p < 0.001 vs. Control group (normal glucose group); #p < 0.05, ##p < 0.01, ###p < 0.01 vs. HG group.
BAE relieved the oxidative stress and angiogenesis of HG-treated HRCECs by inhibiting miR-33 expression
To reveal the mechanism of miR-33 in DR, miR-33 was overexpressed in HRCECs (Fig. A1). Overexpression of miR-33 elevated miR-33 expression in HG + BAE-treated HRCECs (Fig. 2A). Compared with the HG + BAE group, transfection of miR-33 mimic decreased the GLCCI1 mRNA and protein expression (Fig. 2B,C). Significant reduction in ROS and MDA levels can be seen in the group of HG-treated HRCECs after BAE. The above results were rescued by miR-33 overexpression (Fig. 2D–G). Additionally, flow cytometry results demonstrated that HG-induced downregulation of apoptosis rate in HRCECs could be reversed by BAE combined treatment, but the effects of BAE were canceled out when miR-33 overexpression was achieved through transfection with miR-33 miRNA (Fig. 2H,I). MiR-33 overexpression increased the cell viability and angiogenesis of HRCECs compared with the HG + BAE group (Fig. 2J–L). Taken together, these data indicated that BAE alleviated the oxidative stress and angiogenesis of HG-treated HRCECs by regulating miR-33 expression.
Figure 2: BAE relieved oxidative stress and angiogenesis of HG-treated HRCECs by inhibiting miR-33 expression. HRCECs were treated with HG, HG + BAE, or combined with transfection of miR-33 mimic/mimic NC. (A) HRCECs were examined using qRT-PCR for miR-33 expression. (B–C) qRT-PCR and WB were used to assess the GLCCI1 expression in HRCECs. (D–E) DCFH-DA staining was used to examine the ROS levels of HRCECs. (F–G) The SOD activity and the level of MDA in HRCECs were detected. (H–I) Annexin V-FITC/PI was used to detect apoptosis in HRCECs. (J) CCK-8 assay was used to evaluate the proliferation of HRCECs. (K–L) The angiogenesis of HRCECs was examined by the tube formation assay. **p < 0.01, vs. Control group (normal glucose group); #p < 0.05, ##p < 0.01 vs. HG group; &p < 0.05, &&p < 0.01 vs. HG + BAE + mimic NC group.
MiR-33 interacted with GLCCI1 and repressed GLCCI1 expression
The GLCCI1 gene is located on 7p21.3, it contains eight exons and identified functional single nucleotide polymorphism [25,26]. Firstly, we used the online bioinformatics tools Target Scan (www.targetscan.org, accessed on 03/28/2024), miRDB (mirdb.org, accessed on 03/28/2024), and ENCORI (https://starbase.sysu.edu.cn/, accessed on 03/28/2024) to predict the potential target of miR-33. According to these datasets, GLCCI1 was recognized as the latent target of miR-33 and had a potential binding site of miR-33 (Fig. 3A). By dual-luciferase reporter assays, it was found that miR-33 mimic inhibited luciferase activity of the wild-type (WT) GLCCI1 reporter, but this effect was entirely canceled out in the mutant (MUT) GLCCI1 reporter group (Fig. 3B). Subsequently, HRCECs treated with miR-33 mimics exhibited significant downregulation of GLCCI1 mRNA and protein by qRT-PCR and WB. The miR-33 inhibitor led to an up-regulation of GLCCI1 in HRCECs (Fig. 3C–E). Up-regulation of GLCCI1 reversed miR-33 mimic-mediated inhibition of GLCCI1 mRNA and protein expression in HRCECs (Fig. 3F–H). HRCECs were upregulated by miR-33 inhibitors in terms of GLCCI1 mRNA and protein levels, which was abolished by GLCCI1 knockdown (Fig. 3I–K). All these data indicated that miR-33 interacted with GLCCI1 and repressed GLCCI1 expression.
Figure 3: GLCCI1 was targeted by miR-33. (A) Bioinformatics prediction of miR33 and GLCCI1 binding sites. (B) Detection of luciferase activity levels in cells co-transfected with miR33a-5p mimics or miR33b-5p mimics and WT or MUT GLCCI1 (C) In HRCECs, miR-33 expression was determined by qRT-PCR. (D–E) In HRCECs, qRT-PCR and WB analysis were used to investigate GLCCI1 expression in HRCECs. (F–H) In HRCECs, NC mimic or miR-33 mimic was transfected alone or together with pcDNA-GLCCI1 or pcDNA-NC, and miR-33 and GLCCI1 expression were determined by qRT-PCR and WB. (I–K) HRCECs were transfected with miR-33 inhibitor/inhibitor NC alone or combined with si-GLCCI1 or si-NC, qRT-PCR, and WB were used to examine the miR-33 and GLCCI1 expression in HRCECs. *p < 0.05, **p < 0.01 vs. mimic NC group; #p < 0.05, ##p < 0.01 vs. inhibitor NC group; &p < 0.05, &&p < 0.01 vs. miR-33 mimic + pcDNA-NC group; $p < 0.05 vs. miR-33 inhibitor + si-NC group.
BAE relieved the oxidative stress and angiogenesis of HG-treated HRCECs by regulating the miR-33/GLCCI1 axis
Finally, the mechanism of BAE in ameliorating HG-induced oxidative stress and angiogenesis of HRCECs was investigated. qRT-PCR and WB analysis indicated up-regulation of miR-33 and decreased GLCCI1 mRNA and protein in HRCECs treated with HG. BAE treatment inhibited the miR-33 expression while enhancing GLCCI1 mRNA and protein. In HRCECs treated with HG + BAE, miR-33 overexpression decreased the expression of GLCCI1. This effect was reversed by overexpression of GLCCI1. (Fig. 4A–C). Moreover, the oxidative stress indexes in HRCECs were evaluated. Following BAE treatment, HRCECs treated with HG showed a reduction in ROS and MDA levels and an increase in SOD activity. miR-33 overexpression elevated oxidative stress with increased levels of ROS and MDA and decreased the SOD activity in HG + BAE-treated HRCECs, which was abolished by GLCCI1 overexpression (Fig. 4D–F). Additionally, CCK-8, flow cytometry, and tube formation assay results showed that GLCCI1 overexpression reversed miR-33 mimic-mediated promotion of cell viability, angiogenesis, and inhibition of apoptosis in HG + BAE-treated HRCECs (Fig. 4G–I). Taken together, BAE alleviated the oxidative stress and neovascularization of HG-treated HRCECs by regulating the miR-33/GLCCI1 axis.
Figure 4: BAE ameliorated HG-treated HRCECs through the miR-33/GLCCI1 axis. HRCECs were treated with HG, HG + BAE, and then transfected with miR-33 mimic/mimic NC and pcDNA-GLCCI1/pcDNA-NC. (A) HRCECs were tested for miR-33 mRNA levels using qRT-PCR. (B–C) In HRCECs, GLCCI1 factors were determined by qRT-PCR and WB. (D) DCFH-DA staining was used to examine the ROS level in HRCECs. (E–F) The SOD activity and the level of MDA in HRCECs were detected. (G) HRCECs were evaluated for viability using the CCK-8 assay (H) Assays were conducted to detect apoptosis in HRCECs with Annexin V-FITC/PI (I) The tube formation assay examined the angiogenesis of HRCECs. **p < 0.01, ***p < 0.001 vs. Control group (normal glucose group); #p < 0.05, ##p < 0.01 vs. HG group; &p < 0.05, &&p < 0.01 vs. HG + BAE + mimic NC + pcDNA-NC group; $p < 0.05, $$p < 0.01 vs. HG + BAE + miR-33 mimic + pcDNA-NC group.
Diabetic retinopathy is primarily caused by high glucose-induced oxidative stress and angiogenesis. Preliminary mechanism studies showed that BAE mitigated the oxidative stress and micro-angiogenesis of HRCEC by regulating the miR-33/GLCCI1 axis. Thus, we uncovered a new means of preventing DR by BAE.
It is common knowledge that miRNAs have multiple target genes and can regulate a variety of cellular activities. It regulates vascular endothelial cell injury by influencing cell proliferation, migration, and vascular endothelial cell factors [27]. However, miRNAs play a mediating role in metabolism and cellular life cycle [28]. Preclinical studies have shown that Distel et al. reported that miR-33 is associated with energy metabolism and cell cycle regulation [29]. MicroRNA-33 inhibition overcomes diabetes mellitus on atherosclerosis plaque regression [30]. Targeting microRNA-33 can attenuate diabetic nephropathy in Wistar rats [31]. miR-33 knockdown inhibits inflammation and oxidative stress [32]. MicroRNA-33 promoted pathologic vascular proliferation [33]. However, the mechanism of microRNA-33 in diabetic retinopathy is not clear. In this study, miR-33 was highly expressed in HG-treated HRCECs, which were repressed by BAE treatment. miR-33 mimics promoted cell viability, angiogenesis, and inhibition of apoptosis in HG + BAE-treated HRCECs. miR-33 sponged GLCCI1 to repress the expression of GLCCI1. A previous study has revealed that GLCCI1 is abnormally expressed in the lacrimal gland of db/db mice, which may be a marker gene for type 2 diabetes mellitus [34]. This work first investigated the GLCCI1 overexpression reversed miR-33 mimic-mediated promotion of cell viability, angiogenesis, and oxidative stress in HG + BAE-treated HRCECs.
Extracts of blueberry anthocyanins have a higher purity of anthocyanins and can prevent diseases such as diabetes mellitus and DR [18,20]. The retina has the highest respiratory rate of any other mammalian tissue and is a significant source of oxidative stress [21]. Hyperglycemia, oxidative stress, and retinal neovascularization are key events in the pathogenesis of DR [35,36]. BAE decreased diabetes-induced oxidative stress and inflammation in rat retinas via nuclear factor erythroid-2-related actor 2/heme oxygenase 1 (Nrf2/HO-1) signaling pathway [19]. BAE also attenuated endoplasmic reticulum stress injury in the retina of diabetic rats by inhibiting miR-182 expression and promoting 8-oxo guanine DNA glycosylase (OGG1) [22]. BAE also attenuated angiogenesis by decreasing the vascular endothelial cell growth factor (VEGF) level and inhibiting the Akt pathway [20]. In this work, we treated HRCECs with HG to simulate the DR microenvironment. BAE induced apoptosis, and inhibited oxidative stress, cell viability, and angiogenesis of -treated HRCECs. BAE exhibited antioxidant capacities, which could hinder the ROS and MDA production, and increase the antioxidant enzyme SOD activities. Thus, BAE may be used as a useful adjunct for ameliorating retinal oxidative stress and retinal neovascularization by regulating the miR-33/GLCCI1 pathway (Fig. 5).
Figure 5: The mechanisms underlying BAE suppressed high glucose-induced diabetic retinopathy.
This work has shortcomings, including 1) BAE contains anthocyanins, cyanidin-3-glucoside, cyanidin 3,5-glucoside, and peonidin-3-glucoside. Although BAE mainly exerts its effects through anthocyanins, we cannot exclude other ingredients’ effects. 2) We will use surface plasmon resonance (SPR) technology and bioinformatics analysis to predict the target of BAE. Therefore, more investigations are needed in the coming future.
In conclusion, this work demonstrated that BAE relieved the tubule formation in the HRCEC. As a result of BAE, ROS and MDA levels were also reduced, and SOD activity was increased. GLCCI1 was identified as a downstream target of miR-33 by luciferase reporter assay. Besides, miR-33 mimic inhibited the BAE’s effects on oxidative stress and angiogenesis in an in vitro high glucose-induced retinopathy model. Overall, blueberry anthocyanins extract attenuates oxidative stress and angiogenesis through the miR-33/GLCCI1 axis.
Acknowledgement: None.
Funding Statement: This work was supported by the Science and Technology Project of Jiangxi Provincial Administration of Traditional Chinese Medicine (Grant Number: 2022A359).
Author Contributions: Study conception and design: Wenbin Luo, Yuling Zou; analysis and interpretation of results: Wenbin Luo, Hongxi Wu; draft the work and revise it critically for important intellectual content: Wenbin Luo, Yuling Zou, Zhongyi Yang, Zhipeng You. All authors reviewed the results and approved the final version of the manuscript.
Availability of Data and Materials: The datasets generated during and/or analyzed during the current study are not publicly available due to [REASON(S) WHY DATA ARE NOT PUBLIC], but are available from the corresponding author upon reasonable request.
Ethics Approval: Not applicable.
Conflicts of Interest: The authors declare that they have no conflicts of interest to report regarding the present study.
References
1. Beli E, Yan Y, Moldovan L, Vieira CP, Gao R, Duan Y, et al. Restructuring of the gut microbiome by intermittent fasting prevents retinopathy and prolongs survival in db/db mice. Diabetes. 2018;67(9):1867–79. doi:10.2337/db18-0158. [Google Scholar] [PubMed] [CrossRef]
2. Ting DS, Cheung GC, Wong TY. Diabetic retinopathy: global prevalence, major risk factors, screening practices and public health challenges: a review. Clin Exp Ophthalmol. 2016;44(4):260–77. doi:10.1111/ceo.12696. [Google Scholar] [PubMed] [CrossRef]
3. Hou X, Wang L, Zhu D, Guo L, Weng J, Zhang M, et al. Prevalence of diabetic retinopathy and vision-threatening diabetic retinopathy in adults with diabetes in China. Nat Commun. 2023;14(1):4296. doi:10.1038/s41467-023-39864-w. [Google Scholar] [PubMed] [CrossRef]
4. Cheung N, Chee ML, Klein R, Klein BEK, Shea S, Cotch MF, et al. Incidence and progression of diabetic retinopathy in a multi-ethnic US cohort: the multi-ethnic study of Atherosclerosis. Br J Ophthalmol. 2022;106(9):1264–8. doi:10.1136/bjophthalmol-2021-318992. [Google Scholar] [PubMed] [CrossRef]
5. Yau JW, Rogers SL, Kawasaki R, Lamoureux EL, Kowalski JW, Bek T, et al. Global prevalence and major risk factors of diabetic retinopathy. Diabetes Care. 2012;35(3):556–64. doi:10.2337/dc11-1909. [Google Scholar] [PubMed] [CrossRef]
6. Li S, Lu S, Wang L, Liu S, Zhang L, Du J, et al. Effects of amygdalin on ferroptosis and oxidative stress in diabetic retinopathy progression via the NRF2/ARE signaling pathway. Exp Eye Res. 2023;234(3):109569. doi:10.1016/j.exer.2023.109569. [Google Scholar] [PubMed] [CrossRef]
7. Zhao F, Gao X, Ge X, Cui J, Liu X. Cyanidin-3-o-glucoside (C3G) inhibits vascular leakage regulated by microglial activation in early diabetic retinopathy and neovascularization in advanced diabetic retinopathy. Bioengineered. 2021;12(2):9266–78. doi:10.1080/21655979.2021.1996512. [Google Scholar] [PubMed] [CrossRef]
8. Hammes HP. Diabetic retinopathy: hyperglycaemia, oxidative stress and beyond. Diabetologia. 2018;61(1):29–38. doi:10.1007/s00125-017-4435-8. [Google Scholar] [PubMed] [CrossRef]
9. Chang KC, Liu PF, Chang CH, Lin YC, Chen YJ, Shu CW. The interplay of autophagy and oxidative stress in the pathogenesis and therapy of retinal degenerative diseases. Cell Biosci. 2022;12(1):1. doi:10.1186/s13578-021-00736-9. [Google Scholar] [PubMed] [CrossRef]
10. Xie L, Wang Q, Ma J, Zeng Y. Hypoxia-induced reactive oxygen species in organ and tissue fibrosis. BIOCELL. 2023;47(2):261–7. doi:10.32604/biocell.2023.024738. [Google Scholar] [CrossRef]
11. Geng Z, Tan J, Xu J, Chen Q, Gu P, Dai X, et al. ADAMTS5 promotes neovascularization via autophagic degradation of PEDF in proliferative diabetic retinopathy. Exp Eye Res. 2023;234(9):109597. doi:10.1016/j.exer.2023.109597. [Google Scholar] [PubMed] [CrossRef]
12. Dabravolski SA, Isayenkov SV. The role of anthocyanins in plant tolerance to drought and salt stresses. Plants. 2023;12(13):2558. doi:10.3390/plants12132558. [Google Scholar] [PubMed] [CrossRef]
13. Natarajan SB, Hwang JW, Kim YS, Kim EK, Park PJ. Ocular promoting activity of grape polyphenols—a review. Environ Toxicol Pharmacol. 2017;50:83–90. doi:10.1016/j.etap.2016.12.004. [Google Scholar] [PubMed] [CrossRef]
14. Ogawa K, Kuse Y, Tsuruma K, Kobayashi S, Shimazawa M, Hara H. Protective effects of bilberry and lingonberry extracts against blue light-emitting diode light-induced retinal photoreceptor cell damage in vitro. BMC Complement Altern Med. 2014;14(1):120. doi:10.1186/1472-6882-14-120. [Google Scholar] [PubMed] [CrossRef]
15. Guo X, Yang B, Tan J, Jiang J, Li D. Associations of dietary intakes of anthocyanins and berry fruits with risk of type 2 diabetes mellitus: a systematic review and meta-analysis of prospective cohort studies. Eur J Clin Nutr. 2016;70(12):1360–7. doi:10.1038/ejcn.2016.142. [Google Scholar] [PubMed] [CrossRef]
16. Nabavi SF, Habtemariam S, Daglia M, Shafighi N, Barber AJ, Nabavi SM. Anthocyanins as a potential therapy for diabetic retinopathy. Curr Med Chem. 2015;22(1):51–8. [Google Scholar] [PubMed]
17. Zhu CW, Lü H, Du LL, Li J, Chen H, Zhao HF, et al. Five blueberry anthocyanins and their antioxidant, hypoglycemic, and hypolipidemic effects in vitro. Front Nutr. 2023;10:1172982. [Google Scholar] [PubMed]
18. Herrera-Balandrano DD, Chai Z, Hutabarat RP, Beta T, Feng J, Ma K, et al. Hypoglycemic and hypolipidemic effects of blueberry anthocyanins by AMPK activation: in vitro and in vivo studies. Redox Biol. 2021;46:102100. [Google Scholar] [PubMed]
19. Song Y, Huang L, Yu J. Effects of blueberry anthocyanins on retinal oxidative stress and inflammation in diabetes through Nrf2/HO-1 signaling. J Neuroimmunol. 2016;301:1–6. [Google Scholar] [PubMed]
20. Huang W, Yan Z, Li D, Ma Y, Zhou J, Sui Z. Antioxidant and Anti-inflammatory effects of blueberry anthocyanins on high glucose-induced human retinal capillary endothelial cells. Oxid Med Cell Longev. 2018;2018:1862462. [Google Scholar] [PubMed]
21. Kalt W, Cassidy A, Howard LR, Krikorian R, Stull AJ, Tremblay F, et al. Recent research on the health benefits of blueberries and their anthocyanins. Adv Nutr. 2020;11(2):224–36. doi:10.1093/advances/nmz065. [Google Scholar] [PubMed] [CrossRef]
22. Wang C, Wang K, Li P. Blueberry anthocyanins extract attenuated diabetic retinopathy by inhibiting endoplasmic reticulum stress via the miR-182/OGG1 axis. J Pharmacol Sci. 2022;150(1):31–40. doi:10.1016/j.jphs.2022.06.004. [Google Scholar] [PubMed] [CrossRef]
23. Baselga-Escudero L, Arola-Arnal A, Pascual-Serrano A, Ribas-Latre A, Casanova E, Salvadó MJ, et al. Chronic administration of proanthocyanidins or docosahexaenoic acid reverses the increase of miR-33a and miR-122 in dyslipidemic obese rats. PLoS One. 2013;8(7):e69817. doi:10.1371/journal.pone.0069817. [Google Scholar] [PubMed] [CrossRef]
24. Ouimet M, Ediriweera H, Afonso MS, Ramkhelawon B, Singaravelu R, Liao X, et al. microRNA-33 regulates macrophage autophagy in atherosclerosis. Arterioscler Thromb Vasc Biol. 2017;37(6):1058–67. doi:10.1161/ATVBAHA.116.308916. [Google Scholar] [PubMed] [CrossRef]
25. Kiuchi Z, Nishibori Y, Kutsuna S, Kotani M, Hada I, Kimura T, et al. GLCCI1 is a novel protector against glucocorticoid-induced apoptosis in T cells. Faseb J. 2019;33(6):7387–402. doi:10.1096/fj.201800344RR. [Google Scholar] [PubMed] [CrossRef]
26. Rijavec M, Žavbi M, Lopert A, Fležar M, Korošec P. GLCCI1 Polymorphism rs37973 and response to treatment of asthma with inhaled corticosteroids. J Investig Allergol Clin Immunol. 2018;28(3):165–71. [Google Scholar] [PubMed]
27. Zhao J, Zeng X, Liu J, Liu X, Liu Z, Wang B, et al. Marasmius androsaceus mitigates depression-exacerbated intestinal radiation injuries through reprogramming hippocampal miRNA expression. Biomed Pharmacother. 2023;165:115157. [Google Scholar] [PubMed]
28. Haider BA, Baras AS, McCall MN, Hertel JA, Cornish TC, Halushka MK. A critical evaluation of microRNA biomarkers in non-neoplastic disease. PLoS One. 2014;9(2):e89565. [Google Scholar] [PubMed]
29. Ortega R, Liu B, Persaud SJ. Effects of miR-33 deficiency on metabolic and cardiovascular diseases: implications for therapeutic intervention. Int J Mol Sci. 2023;24(13):10777. [Google Scholar] [PubMed]
30. Distel E, Barrett TJ, Chung K, Girgis NM, Parathath S, Essau CC, et al. miR33 inhibition overcomes deleterious effects of diabetes mellitus on atherosclerosis plaque regression in mice. Circ Res. 2014;115(9):759–69. [Google Scholar] [PubMed]
31. Ghaffari T, Moradi N, Chamani E, Ebadi Z, Fadaei R, Alizadeh-Fanalou S, et al. Captopril and spironolactone can attenuate diabetic nephropathy in wistar rats by targeting ABCA1 and microRNA-33. Curr Pharm Des. 2022;28(16):1367–72. [Google Scholar] [PubMed]
32. Wang X, Li X, Huang B, Yang L, Chen K, Zhao D, et al. Downregulation of miR-33 has protective effect against Aβ25−35-induced injury in SH-SH-SY5Y cells. Med Sci Monit. 2020;26:e921026. [Google Scholar] [PubMed]
33. Sene A, Khan AA, Cox D, Nakamura RE, Santeford A, Kim BM, et al. Impaired cholesterol efflux in senescent macrophages promotes age-related macular degeneration. Cell Metab. 2013;17(4):549–61. [Google Scholar] [PubMed]
34. Pei X, Qi D, Liu J, Si H, Huang S, Zou S, et al. Screening marker genes of type 2 diabetes mellitus in mouse lacrimal gland by LASSO regression. Sci Rep. 2023;13(1):6862. [Google Scholar] [PubMed]
35. Kang Q, Yang C. Oxidative stress and diabetic retinopathy: molecular mechanisms, pathogenetic role and therapeutic implications. Redox Biol. 2020;37:101799. [Google Scholar] [PubMed]
36. Shi W, Meng Z, Luo J. Connexin 43 (C × 43) regulates high-glucose-induced retinal endothelial cell angiogenesis and retinal neovascularization. Front Endocrinol. 2022;13:909207. doi:10.3389/fendo.2022.909207. [Google Scholar] [PubMed] [CrossRef]
Appendix A
Figure A1: The verification of miR-33 mimic. The verification of miR-33 mimic in HRCEC cell. **p < 0. 01 vs. mimic NC group.
Cite This Article
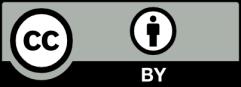
This work is licensed under a Creative Commons Attribution 4.0 International License , which permits unrestricted use, distribution, and reproduction in any medium, provided the original work is properly cited.