Open Access
ARTICLE
Oleanolic acid inhibits colon cancer cell stemness and reverses chemoresistance by suppressing JAK2/STAT3 signaling pathway
1 Department of Anus and Intestine Surgery, Shenzhen Longhua District Central Hospital, Shenzhen, China
2 Department of Urology, Shenzhen Longhua District Central Hospital, Shenzhen, China
* Corresponding Author: WEI LI. Email:
BIOCELL 2024, 48(7), 1037-1046. https://doi.org/10.32604/biocell.2024.051074
Received 27 February 2024; Accepted 17 April 2024; Issue published 03 July 2024
Abstract
Background: Oleanolic acid (OA), a pentacyclic triterpenoid exhibiting specific anti-cancer properties and highly effective antioxidant activity, was isolated from traditional Chinese medicinal herbs. Conversely, the OA that impacts colon cancer (CC) cells and its underlying mechanisms remain poorly understood. Methods: The cytotoxic effect of OA alone or OA-5-Fluorouracil (5-FU) combination on normal and CC cells was analyzed by methyl thiazolyl diphenyl-tetrazolium bromide (MTT). Then, the impact of OA on CC cell lines (LoVo and HT-29) proliferation and stemness were measured using colon formation and tumorsphere formation assays. Octamer-binding transcription factor 4 (Oct4), Prominin-1 (CD133), Nanog, and transcription factor SOX-2 (SOX2) are cell stemness-related indicators whose expression was assessed using fluorescence qPCR assay, Western blotting, and immunohistochemistry. The effect of OA on the proliferative potency of CC cells was evaluated using an in vivo model. Results: The stem-like characteristics and clone production of colon cancer cells were markedly reduced by OA alone or in combination with OA-5-FU. Moreover, OA increases the susceptibility of CC cells to 5-FU by blocking the cell stemness-related markers (CD133, Nanog, SOX2, and Oct4) expression levels both in vitro and in vivo, as well as by inactivating the activator of transcription 3 (STAT3 signaling) and Janus kinase 2/signal transducer (JAK2). Conclusion: These findings imply that oleanolic acid, both in vitro and in vivo, suppresses the JAK2/STAT3 pathway, which in turn reverses chemoresistance and decreases colon cancer cell stemness. Therefore, by reducing the recommended amount of 5-FU, this strategy may improve chemotherapeutic effectiveness and minimize undesired side effects.Keywords
Supplementary Material
Supplementary Material FileColon cancer (CC) is a highly prevalent and deadly form of cancer that is currently one of the most commonly diagnosed malignancies worldwide [1]. Even though screening and treatment strategies (surgery, chemotherapy, and targeted therapy) for colon cancer have improved in recent years, about 40% of patients experience recurrence, resistance, and even death within 5 years of treatment [1,2]. Currently, chemotherapy for colon cancer consists of two types: single-agent therapy, such as 5-FU, and multiple-agent regimens that include drugs like capecitabine, irinotecan (IRI), and oxaliplatin (OX). These treatments have enhanced the overall survival (OS) rate and quality of life (QoL) for individuals with CC [3,4]. Nevertheless, chemotherapy is hindered by particular drawbacks, including systemic toxicity, resistance, and limited tumor-specific selectivity [5]. Additionally, after long-term use of these drugs, 50% of CC patients have developed drug resistance, which is the main contributor to a poor prognosis among CC patients [6].
Moreover, prior research has demonstrated a high occurrence of abnormally activated JAK2/STAT3 in many malignancies, such as CC, which typically do not respond well to conventional treatment [7]. Recently, the JAK2/STAT3 pathway has attracted attention as a potential target for creating new anti-tumor drugs. It shows promise in treating various types of solid cancers [8]. Many studies have demonstrated that numerous bioactive compounds from natural plants or Chinese medicine have shown anti-tumor properties against multiple cancers by inhibiting the JAK2/STAT3 pathway in vivo and in intro [9–11]. Anthraquinone can hinder the growth and advancement of CC cells by targeting the JAK2/STAT3 signaling pathway [12]. Telocinobufagin, derived from the toad cake, effectively suppresses the growth and spread of osteosarcoma by blocking the JAK2/STAT3 signaling pathway [13]. Currently, a significant proportion (over 70%) of drugs approved by the FDA are made up of bioactive substances or their synthetic counterparts [14,15]. As a result, it is crucial to develop innovative and highly efficient anti-cancer medications derived from natural plants specifically for treating CC.
Oleanolic acid (OA), a natural triterpenoid isolated from traditional Chinese medicinal herbs, is receiving outstanding attention due to its biological activity against multiple diseases [16]. OA modulates human dendritic cell’s function in a fashion that favors T helper 1 cell (Th1) polarization via the activation of interleukin-12 (IL-12) dependent on Toll-like receptor-4 (TLR4) and/or Toll-like receptor-2 (TLR2) [17]. OA exhibits notable antibacterial action against many bacterial species, which makes it a promising natural source of free radical scavengers [18]. Compared to the above bioactivities, the anti-cancer activity of OA has received the most attention from researchers. Prior research has indicated that OA can inhibit tumor cells’ growth, movement, and infiltration in several forms of cancer by obstructing multiple internal biological mechanisms [19]. For instance, the growth of Hela cells is suppressed by OA, which is used in cervical cancer by modifying the Acyl-CoA synthetase long-chain family (ACSL4) ferroptosis signaling pathway [20]. The compound OA triggers apoptosis and autophagy in CC cells by blocking the adenosine monophosphate-activated protein kinase (AMPK) [21].
Additionally, OA lowers aerobic glycolysis by preventing gastric cancer cells from expressing yes-associated protein (YAP)-associated protein [22]. Apart from the direct anti-cancer activity, OA can also act synergistically with chemo and radiotherapy. When combined with radiation therapy, OA and olaparib substantially lowered the rate of cell growth in triple-negative breast cancer [23]. Additionally, OA makes doxorubicin more effective against pancreatic cancer cells [24]. Therefore, OA may be a potential compound showing outstanding clinical application prospects.
In the present research, we used 5-FU to test the effects of either combo therapy or OA alone. We investigated the fundamental processes of CC in vivo and in vitro settings.
People with stage I colon cancer (CCD 841 CoTr, No. JY771) and human adenocarcinoma cells (HT-29, No. TCHu103, and LoVo, No. TCHu 82) were obtained from the Shanghai Cell Bank at the Chinese Academy of Science in Shanghai, China. The cells were maintained alive in DMEM (Hyclone, SH30022, Shanghai, China) medium that had fetal bovine serum (FBS, 10%; #10270-106; Thermo Fisher Scientific, USA) and 100 U/mL penicillin-streptomycin. Cells were characterized and authenticated by short tandem repeats (STR). The results of the measurements revealed that no mycoplasma contamination was detected in any of the lines.
The impact of OA (No. 508-02-1, Sigma-Aldrich, USA) on HT-29 and LoVo cells was evaluated through methyl thiazolyl diphenyl-tetrazolium bromide (MTT) assay. For 12 h, 3 × 103 cells were seeded into each of the 96 wells. Subsequently, during 24, 48, and 72 hours, cells were exposed to various concentrations of dissolved OA (0, 10, 20, 40, 60, 80, and 100 μM) in 0.1% dimethyl sulfoxide (DMSO). Upon completion of the treatment, 25 μL of MTT (5 mg/mL in PBS) (No. 298-93-1, Sigma-Aldrich) was added to each well and incubated for an extra 4 h at 37°C. Then, the DMSO (100 μL) was used to dissolve the formazan residue. After that, a BIO-TEK Instruments microtiter plate reader (Winooski, VT, USA) was used to measure absorbance at 590 nm.
Following seeding onto six-well plates, LoVo and HT-29 cells were grown at a density of 600 cells per well in DMEM medium enhanced with 10% FBS. After 12 h incubation, the medium was replaced with different doses (20 and 40 μM) of OA. The control group was not treated with OA (0 μM), only with 0.1% DMSO. The colonies were fixed with paraformaldehyde (4%) (P0099, Beyotime Biotech, Shanghai, China) and stained with crystal violet (0.5%) (C0121, Beyotime Biotech) following a 14-day culture period with 5% CO2 at 37°C in an incubator. A camera captured pictures of six-well plates, and the visible colonies were tallied.
Trypsin (C0201, Beyotime Biotech, Shanghai, China) was used to digest LoVo and HT-29. The cells were then processed into a single cell suspension using serum-free DMEM medium, which was enhanced with epidermal growth factor (EGF) (354001, Corning, New York, USA) Primary fibroblast growth factor (bFGF) at 20 ng/mL concentration (CB40060, Corning, NY, USA) 10 ng/mL, N-2 Supplement (N2) (C0335, Beyotime Biotech) at 2% and 1%, and Bottenstein’s N-27 formulation (B27) (A3582801, Thermo Fisher Scientific, USA). Cells (2000/well) were seeded into 6-well ultralow attachment plates for 2 weeks and with different doses (0, 20, and 40 μM) of OA. Then, the images were taken by photography (CK40, Olympus Corporation, Tokyo, Japan), and the number of cell spheres was counted.
The FAST200 kit (220011, Feijie, Shanghai, China) extracted total RNA from CC cell lines. The NanoDrop 2000 kit (Thermo Scientific, USA) was utilized to quantify the amount and quality of RNA (at 260 and 280 nm wavelengths). Subsequently, the DNA was transcribed in the opposite direction and converted into complementary DNA (cDNA) using a qRT-PCR cDNA synthesis kit (RR067A, Takara, Dalian, China). The gene expression was measured using the Bio-Rad iQ5 Real-Time PCR System (Invitrogen Life Technologies, USA) with SYBR green detection (RR064B, Takara, Dalian, China). The cycling conditions were as follows: 95°C for 5 min, 95°C for 5 s, 60°C for 30 s, and 72°C for 34 s. The melting curve stage was then reached. The GAPDH gene served as a standard gene. Target mRNA levels were determined using the 2−ΔΔCt method with three replicates [25]. The primer sequences used in this work are as follows:
CD133 forward: 5’-GGTTTCATCCATCCGACATTG-3’;
CD133 reverse: 5’-ACACGGCAGGCATACTCATCT-3',
GAPDH, Nanog, SOX2, and Oct4 primers were synthesized [26,27].
The BCA protein assay kit (No. 23225, Pierce, Rockford, IL, USA) was used to quantify the total proteins, which were extracted using RIPA buffer with protease inhibitor (P0013C, Beyotime Biotech). The procedure for Western blotting analysis was mentioned earlier [27]. After electrophoresis using 10% SDS-PAGE, 80 μg of total protein was extracted and placed onto polyvinylidene difluoride (PVDF) membranes (No. 88518, Thermo Fisher Scientific, USA). After blocking the membrane with 5% skim milk for two hours, the membrane was incubated with primary antibodies to CD133 (1:1000, ab284389), Nanog (1:1000, ab21624, SOX2 (1:800, ab92494), Oct4 (1:800, ab184665), GADPH (1:1000, ab8245), all acquired from Abcam, UK), JAK2 (1:800, 3230, CST, USA), phosphorylated JAK2 (Y1007/1008) (1:800, 3771, CST, USA), phosphorylated STAT3 (Y705) (1:800, 9145, CST, USA), and STAT3 (1:1000, 9139, CST, USA) overnight at 4°C, followed by HRP-conjugated secondary antibodies(1:2000, ab150077, Abcam, UK) at room temperature for 1 h Enhanced Chemiluminescence (ECL) reagents (No. 32209, Thermo Fisher Scientific, Inc., USA) were used to detect the band signals. Densitometric analysis of the Western blotting was conducted with the help of Image Lab Software (v.3.0) (Bio-Rad Laboratories, USA).
Guangdong Medical University approved all in vivo investigations, which complied with the procedures established for the Care and Use of Laboratory Animals (Approval No. GDY2202009). The mice were accustomed to living in a particular pathogen-free (SPF) animal facility. The animals were cared for under constant humidity, temperature, and a regulated light-dark cycle. Male BALB/c nude mice (age = 4 to 5 weeks) received subcutaneous injections of HT-29 cells into their right flanks at a concentration of 2 × 106 cells per mouse. The mice were sourced from the Animal Center of Southern Medical University in Guangzhou, China. The tumors were randomly categorized into 4 groups (n = 5 for each group) once their volume reached 100 mm3. For three days throughout 18 days, mice received injections of DMSO (0.1%), OA (15 mg/kg), 10 mg/kg of 5-FU, and OA + 5-FU (15 mg/kg + 10 mg/kg). Every three days, the mice were observed. A sliding caliper was used to measure the tumor volumes of the xenografts after 18 days, and the tumor volume was computed as length × width2 × 0.5. After 18 days, the mice were placed in a new cage with 100% carbon dioxide for 5 min. Then, the tumors were dissected and weighed.
Immunohistochemistry (IHC) staining
Tumor were fixed for immunohistochemical experiments, as reported earlier [27]. In summary, sections were incubated with CD133 (1:150, ab284389), Nanog (1:150, ab21624, Abcam, UK), SOX2 (1:150, ab92494), Oct4 (1:100, ab184665, from Abcam, UK), phosphorylated JAK2 (Y1007/1008) (1:100, 3771, CST, USA), phosphorylated STAT3 (Y705) (1:100, 9145, CST, USA) at 4°C overnight, and then employing the streptavidin-conjugated horseradish peroxidase they were subsequently incubated. Sections were visualized with a DAB kit (P0202, Beyotime Biotech, Shanghai, China) and analyzed using a brightfield microscope (Leica DMI 40008, Germany).
The GraphPad Prism v.9.0 software (La Jolla, USA) was used to compare two groups using the student’s t-test. A p-value of <0.05 indicated a statistically significant difference in all two-sided statistical significance tests.
The impact of OA concentrations on the viability of CC cells
To ascertain the optimal concentrations of OA for CC cells (CCD 841 CoTr) and human normal colon epithelial cells. CCD 841 CoTr cells and CC cells (HT-29 and LoVo) were subjected to OA treatment at varying concentrations (0, 10, 20, 40, 60, 80, and 100 μM) for 24, 48, and 72 h. Cell viability was evaluated through the utilization of cell growth curve assays. The findings indicated that the HT-29 and LoVo cells viability remained unaffected by 10 μM OA within 48 h or less than 20 μM within 24 h (p > 0.05). Conversely, as treatment duration and OA concentration increased, cell viability decreased compared to cells not treated with OA (0 M) (Figs. 1A and 1B). Furthermore, at 24, 48, and 72 h, OA’s IC50 (inhibitory concentration) on the HT-29 cell line was 56.2, 46.3, and 31.6 M, respectively (Fig. 1A). The IC50) value of OA on the LoVo cell line was 81.4 μM at 24 h, 69.3 μM at 48 h, and 37.3 μM at 72 h (Figs. 1A and 1B). Furthermore, human normal colon epithelial cells (CCD 841 CoTr) are hazardous to higher doses of OA (above 40 μM for 24 h; Fig. 1C). The CCD 841 CoTr cell line’s IC50 values for OA were 86.3, 59.4, and 42.6 μM at 24, 48, and 72 h, respectively (Fig. 1C). HT-29 and LoVo cells, on the other hand, were more prone to OA than CCD 841 CoTr cells. These findings demonstrated that OA considerably and time-dependently reduced the CC cell growth.
Figure 1: The effects of OA on cell viability of CC cells. HT-29 and LoVo cells were treated with different doses (0, 10, 20, 40, 60, 80, and 100 μM) of OA at various times (24, 48, and 72 h), respectively. The HT-29 (A), LoVo (B), and CCD 841 CoTr (C) were tested for cell vitality using the MTT assay. The control group’s cell viability, which was treated with 0 mg/mL OA, was 100%. The cell viability percentage was computed with the control group. The mean ± SD of the proportion of viable cells from three separate trials, each with three duplicates, is represented by every single data point in the figure. *p < 0.05, **p < 0.01 in comparison to the control group (0 mg/mL OA) simultaneously.
OA inhibits proliferation and self-renewal capacity of CC cells
According to recent studies, colon cancer stem cells (CCSCs) are considered the primary initiator of cancer metastasis, recurrence, progression, or chemoresistance [28]. Thus, the sphere-formation assay and plate clone-formation assay were employed. The findings demonstrated that, in a concentration-dependent way, OA at lower doses (20 or 40 μM) effectively prevented the production of CC cell clones (Fig. 2A). OA also significantly reduced tumorsphere size in colon cancer cells (Fig. 2B).
Figure 2: OA prevents CC cells from proliferating and from being able to replenish themselves. OA was applied to CC cells at 0, 20, and 40 μM over 14 days. (A) Plate clone development To determine the inhibitory impact of OA on CC cells, an assay was utilized. (B) Utilizing a sphere-formation assay, the inhibitory effect of OA on the stemness properties of CC cells was determined. The mean ± SD of the percentage of viable cells was calculated for each of the 3 independent experiments, with triplicates of each experiment. *p < 0.05, **p < 0.01.
Additionally, the stemness property of CCSCs was assessed using real-time PCR by measuring the prominin-1 (CD133) and Octamer-binding transcription factor 4 (Oct4) expression level in Nanog. The findings demonstrated that, following a 48-h treatment, OA considerably and concentration-dependently raised the mRNA levels of stemness-related markers (CD44, Nanog, Oct4, and CD133) (Figs. 3A and 3B). These findings demonstrated that OA decreased colon cancer cells’ ability to increase.
Figure 3: The ability of CC cells to self-renew is inhibited by OA. For 48 h, HT-29 (A) and LoVo (B) cells were exposed to varying concentrations of OA (0, 20, and 40 μM), and then the stemness properties of CCSCs were measured using real-time PCR. The mean ± SD of the proportion of viable cells from 3 distinct examinations, each with three replicas, is represented by each data point in the figure. *p < 0.05, **p < 0.01.
OA promotes the CC cell’s sensitivity to 5-FU
Many studies have demonstrated that CCSCs were regarded as the key contributors to 5-FU resistance in colorectal carcinoma [6,29]. According to the above results, OA inhibited the stemness property of LoVo and HT-29 CC cells. We investigated whether OA increases the sensitivity of LoVo and HT-29 cells to 5-FU. LoVo and HT-29 cells were subjected to varying concentrations of 5-FU (0, 5, 10, and 15 μM) for distinct durations of 48 h. The findings demonstrated that the proliferation of CC cells (HT-29 and LoVo) was inhibited in a concentration-dependent fashion by 5-FU (Figs. 4A and 4B). Following 48 h, the administration of various dosages (0, 20, 40, and 60 μM) of OA effectively suppressed the CC cell development in a manner that was dependent on the concentration (Figs. 4A and 4B). OA and 5-FU, when used together, have a higher inhibitory impact than when used separately (Figs. 4A and 4B).
Figure 4: 5-FU sensitivity of CC cells is increased by OA. The sensitivity of HT-29 and LoVo cells to 5-FU was evaluated using MTT assays (A and B). The cells were cultured for a duration of 48 h with varying concentrations of 5-FU (0, 5, 10, and 15 μM), OA (0, 20, 40, and 60 μM), or a combination of OA and 5-FU. *p < 0.05, **p < 0.01.
In addition, plate clone formation assay and sphere-formation assay indicated that OA (40 μM) or 5-FU (10 μM) treatment inhibited the clone formation and tumorsphere formation ability of LoVo and HT-29 cells. But the combination of OA + 5-FU (40 μM + 10 μM) showed a dramatical inhibitory effect on clone formation and tumorsphere formation comparison with OA (40 μM) or 5-FU (10 μM) treatment (Figs. 5A and 5B). These results showed that OA improved the sensitivity of CC cells (HT-29 and LoVo) to 5-FU.
Figure 5: The inhibitory effects of OA (40 μM), 5-FU (10 μM), and OA + 5-FU (40 μM + 10 μM) combination on the proliferation of HT-29 and LoVo cells. HT-29 and LoVo cells were treated with OA (40 μM), 5-FU (10 μM), and OA + 5-FU (40 + 10 μM) for 14 d. (A) The plate clone formation test assessed the inhibitory effects on HT-29 and LoVo cells. (B) A sphere-formation assay was used to measure the self-renewal capacity of LoVo and HT-29 cells. *p < 0.05, **p < 0.01.
OA improves the CC cell’s sensitivity to 5-FU by inactivation of JAK2/STAT3 signaling
It is well known that the JAK2/STAT3 signaling activation is closely related to the stemness acquisition of cancer cells through regulating cancer stem cell markers expression [26,30,31]. To examine the mechanism by which OA increases the responsiveness of CC cells to 5-FU, we assessed the STAT3 and JAK2 protein levels using Western blot analysis. The results showed that the treatment of OA (40 μM) or 5-FU (10 μM) downregulated the p-STAT3 and p-JAK2 protein levels without affecting the total protein expression. However, when OA (40 μM) and 5-FU (10 μM) were combined at 40 μM and 10 μM concentration, respectively, there was a significant reduction in the protein levels of p-STAT3 and p-JAK2 compared to when OA or 5-FU were used alone (Figs. 6A and 6B).
Figure 6: In vitro, OA impeded the JAK2/STAT3 signaling pathway. For 48 h, HT-29 cells were exposed to OA (40 μM), 5-FU (10 μM), and OA + 5-FU (40 + 10 μM). (A) The protein was extracted and then analyzed using SDS-PAGE, followed by Western blot analysis. The internal control used was GAPDH. (B) the fold variation in protein integrated absorbance following GAPDH normalization. One was the protein level in the cells treated with 0 mg/mL OA (Control). *p < 0.05, **p < 0.01.
Furthermore, Western blot was used to assess the expression of SOX2, Oct4, Nanog, and CD133, the cancer stem cell markers. The findings demonstrated that the expression of cancer stem cell markers was suppressed by OA (40 μM) or 5-FU (10 μM) therapy. But the combination of OA + 5-FU (40 μM+10 μM) showed a dramatical decrease in the protein levels comparison with OA (40 μM) or 5-FU (10 μM) treatment alone (Figs. 7A and 7B).
Figure 7: OA suppresses the cancer stem‑like properties of CC cells through inhibited JAK2/STAT3 signaling in vitro. For 48 h, the HT-29 cells were treated with OA (40 μM), 5-FU (10 μM), and OA + 5-FU (40 + 10 μM). (A) The total protein was extracted and subjected to SDS-PAGE, followed by Western blot analysis. GAPDH was used as an internal control. (B) The fraction variation of protein integrated absorbance after GAPDH normalization. A protein concentration of 1 was established for cells treated with 0 mg/mL OA (Control). *p < 0.05, **p < 0.01.
OA enhances the CC cell’s sensitivity to 5-FU in vivo
To verify that OA increases the susceptibility of 5-FU-resistant CC cells to 5-FU in vivo, HT-29 cells were injected subcutaneously into BALB/c nude mice, and tumors were developed. The findings indicated that compared to the groups treated with OA (12 mg/kg), 10 mg/kg of 5-FU, or OA + 5-FU, the tumors in the control group (DMSO) developed more quickly (Fig. 8A). The group receiving OA with 5-FU treatment had a considerably reduced tumor volume and weight compared to those receiving 12 mg/kg of OA or 10 mg/kg of 5-FU alone (Figs. 8B and 8C).
Figure 8: OA enhances the sensitivity of CC cells to 5-FU in vivo. After the tumors reached a size of 100 mm3, the mice were split into 4 groups at random (n = 5). For eighteen days, mice in each group received injections every 3 days of control (0 mg/kg), OA (12 mg/kg), 5-FU (10 mg/kg), and OA + 5-FU (12 + 10 mg/kg). (A) The representative tumor pictures from xenografted BALB/C nude mice. (B) Tumor volumes were assessed every 3 days. (C) After 18 days of therapy with OA (12 mg/kg), 5-FU (10 mg/kg), and OA + 5-FU (12 + 10 mg/kg), tumor weights were determined. *p < 0.05, **p < 0.01.
The expression of the subcutaneous tumors of nude mice was subsequently assessed using IHC. According to the data, the subcutaneous tumors treated with the control group (DMSO) showed increased expression levels of cancer stem cell markers. A decreased expression of cancer stem cell markers CD133, Oct4, and SOX2 in the OA + 5-FU treated group than those in the OA (12 mg/kg) or 5-FU (10 mg/kg) alone group (Figs. 9A and 9B). Furthermore, we assessed the p-STAT3 and p-JAK2 expression in the corresponding subcutaneous tumors in mice without clothing. The findings demonstrated that p-STAT3 and p-JAK2 were highly expressed in the tumors in the control group (DMSO) and that these expressions were reduced in the tumors in the groups treated with 12 mg/kg of OA or 10 mg/kg of 5-FU. However, the expression of p-JAK2 and p-STAT3 levels was lower in the OA + 5-FU treated group than those in the OA (12 mg/kg) or 5-FU (10 mg/kg) alone group (Figs. 9A and 9B). These findings demonstrated that OA increases the sensitivity of CC cells to 5-FU by inhibiting JAK2/STAT3 signaling.
Figure 9: Effects of control (0), 12 mg/kg of OA, 10 mg/kg of 5-FU, and OA + 5-FU on the expression of CD133, Oct4, SOX2, p-JAK2 and p-STAT3 in HT-29 xenograft models. (A) The expression of SOX2, Oct4, CD133, p-JAK2, and p-STAT3 was found in the specified subcutaneous tumors of nude mice using immunohistochemical staining. (B) The CD133, Oct4, SOX2, p-JAK2 and p-STAT3 expression were quantified. Thus, by inhibiting the JAK2/STAT3 pathway, OA increases the sensitivity of CC cells to 5-FU and decreases their ability to improve. *p < 0.05, **p < 0.01.
Colon cancer, one of the most prevalent malignant malignancies in humans, is increasing in incidence annually worldwide [2]. Although chemotherapy is still the predominant ways for colon cancer treatments, many patients show drug resistance after long-term treatment [32]. Although they have fewer side effects and have been used widely as effective substitute treatments for various malignancies, natural products may be an option. The current study showed that OA alone, or combined with OA + 5-FU, effectively suppressed the stem-like qualities and clone generation of CC cells. OA is a naturally occurring triterpenoid extracted from traditional Chinese medicinal herbs. According to studies on the molecular mechanisms involved, OA increases the susceptibility of CC cells to 5-FU by blocking JAK2/STAT3 signaling and reducing the expression of cell stemness-related markers (CD133, Nanog, Oct4, and SOX2) in vivo and in vitro.
Cancer stem cells (CSCs) are a distinct subpopulation of cancer cells that can differentiate and self-renew, as is widely recognized [28,33]. Numerous studies conducted in recent times have provided evidence that CSCs play a role in tumorigenesis, drug resistance, and proliferation of using their persistent proliferation and resistance to traditional anti-cancer treatments [34–36]. Therefore, it has been thought that targeting CSCs is the best course of action for treating cancer in recent years. Thymoquinone, the primary active component separated from black seed oil, inhibits the characteristics of lung cancer stem cells by inducing YAP breakdown [37]. Sec62 stimulates the Wnt/β-catenin pathway, which increases colorectal cancer stemness and chemoresistance [38].
The present study showed that OA significantly inhibited CC cells’ stem-like properties and clone formation through down-regulating cell stemness-related markers (CD133, Nanog, Oct4, and SOX2) expression. Furthermore, a revolutionary method of cancer therapy that simultaneously targets various therapeutic anti-cancer pathways is combination therapy, which involves the use of two or more chemotherapeutic drugs. The natural isoflavone Biochanin-A increased 5-fluorouracil anti-cancer activity through suppression of estrogen receptor alpha (ER-α)/serine/threonine kinase (Akt) axis in vitro and in vivo [39]. Chemotherapeutic and chemopreventive effects of OA have been observed in a range of human cancer types, including prostate cancer and breast cancer [40,41]. In pancreatic cancer cells, OA also boosts doxorubicin’s anti-cancer efficacy [24].
Moreover, combined therapy of ursolic and OA prevents CC cells from migrating in vivo and in vitro [42]. In agreement with earlier findings, the current findings demonstrated that, compared to OA or 5-FU treatment, combining the OA + 5-FU demonstrated a markedly inhibitory effect on the production of tumorspheres and clones. Through its inhibition of cell stemness, our finding first demonstrated that OA may be a powerful drug against CC and offers a scientific justification for additional clinical application. Evidence shows that the JAK2/STAT3 pathway is crucial in several malignancies [7,30]. By controlling downstream gene expression, the JAK2/STAT3 pathway promotes the potential of CSCs to transition and metastasize, causing tumors to become more resistant to chemotherapy. For instance, panaxadiol downregulated the JAK2/STAT3 pathway, dramatically reducing proliferation and promoting [43]. Through the JAK2/STAT3 signaling pathway, the anthraquinone derivative C10 prevents colon cancer cells from proliferating and progressing through the cell cycle [12]. The maintenance of non-small cell lung cancer stem cells is mediated by JAK2/STAT3 signaling through the aryl hydrocarbon receptor [44]. Previous studies also showed that OA suppresses tumor cell invasion and cell proliferation by blocking STAT3, AMPK, nuclear factor kappa-B (NF-κB), and mTOR signaling pathways.
Consequently, we explore the impact of OA on JAK2/STAT3 in greater detail. By inhibiting the JAK2/STAT3 signaling pathway, OA increased the sensitivity of CC cells to 5-FU. Additionally, OA suppressed the expression of cell stemness-related markers in vitro and in vivo. Currently, no empirical evidence indicates that OA inhibits the activity of extracellular phosphatases. Prior research has demonstrated that OA can impede hepatocellular carcinoma cells’ migration and invasion capabilities by promoting inducible nitric oxide synthase (iNOS) dimerization [45]. Furthermore, OA was found to inhibit the up-regulation of vascular endothelial growth factor (VEGF) and p38 mitogen-activated protein kinase (MAPK) phosphorylation in rat models of subarachnoid hemorrhage [46].
However, mechanisms of how OA directly or indirectly regulates the JAK2/STAT3 signaling pathway need further investigation. Investigating the potential utility of OA is valuable. Therefore, the target genes of OA were analyzed using ChEMBL (https://www.ebi.ac.uk/chembl/). Analysis results show that OA may affect or interact with intracellular enzyme (DNA polymerase beta (Polb), phospholipase A2 group 1B (PLA2G1B) and aldo-keto reductase family 1 member B10 (AKR1B10)), T-cell protein-tyrosine phosphatase (PTPN2), phosphatase (Protein-tyrosine phosphatase 1B (PTPN1) or dual specificity phosphatase (CDC25B)) activity, regulate nuclear receptor (Nuclear receptor ROR gamma, ROR γ) and so on (Suppl. Fig. S1 and Suppl. Table S1). For example, Abnormal expression of PTPN1 can affect the JAK/STAT pathway in classical Hodgkin lymphoma [47]. In diabetes mellitus type 2 patients, JAK2, STAT3, and ROR-γ expression were positively related [48]. Consequently, the subsequent research will delve deeper into the target genes of OA. However, there are certain limitations to the present study: (1) The critical limitation was the investigation of only two CC cell lines. (2) We did not investigate the mechanisms of how OA directly or indirectly regulates the JAK2/STAT3 pathway. (3) although the target genes of OA were analyzed using ChEMBL, some assays will be conducted to confirm the regulatory relationship between OA and PTPN1 (or ROR γ) within the cellular context.
In summary, this research revealed that OA exhibited a substantial inhibitory effect on the stem-like characteristics and clone formation of CC cells. Furthermore, the combined administration of OA and 5-FU demonstrated a considerably stronger anti-cancer effect than either OA or 5-FU treatment alone. OA increases the CC cell’s sensitivity to 5-FU by inhibiting the JAK2/STAT3 signaling pathway and by reducing the CD133, Nanog, SOX2, and Oct4 expression levels in vitro and in vivo, according to molecular mechanism studies. As a result, these findings are expected to offer fresh perspectives on the mechanisms by which OA targets critical components of stemness and establish a scientific justification for the potential future clinical application of OA and 5-FU in combination. In addition, the current findings make it a potentially effective adjunct to chemotherapeutics for the treatment of CC.
Acknowledgement: We would like to thank all the investigators, including the physicians, nurses, and laboratory technicians in this study.
Funding Statement: The work was supported by grants from the Scientific Research Projects of Medical and Health Institutions of Longhua District, Shenzhen (2021016) and, Shenzhen Basic Research Project (JCYJ20210324125803008). The above funding listed did not have a role in the design of the study, in the collection, analysis, and interpretation of data, and in the writing of this manuscript.
Author Contributions: Study conception and design: WL, RYC, YMW; data collection: RYC, FW, JTZ; analysis and interpretation of results: RYC, HZH; draft manuscript preparation: RYC, WL. All authors reviewed the results and approved the final version of the manuscript.
Availability of Data and Materials: All data in this study are available upon request by writing to the corresponding author.
Ethics Approval: All in vivo experiments were done according to the Guidelines for the Care and Use of Laboratory Animals and were approved by the Guangdong Medical University (GDY2202009).
Conflicts of Interest: The authors declare that they have no competing interests.
Supplementary Materials: The supplementary material is available online at https://doi.org/10.32604/biocell.2024.051074.
References
1. Fabregas JC, Ramnaraign B, George TJ. Clinical updates for colon cancer care in 2022. Clin Colorectal Cancer. 2022;21(3):198–203. doi:10.1016/j.clcc.2022.05.006. [Google Scholar] [PubMed] [CrossRef]
2. Benson AB, Venook AP, Al-Hawary MM, Arain MA, Chen YJ, Ciombor KK, et al. Colon Cancer, Version 2. 2021, NCCN clinical practice guidelines in oncology. J Natl Compr Canc Netw. 2021;19(3):329–59. doi:10.6004/jnccn.2021.0012. [Google Scholar] [PubMed] [CrossRef]
3. Tie J, Cohen JD, Lahouel K, Lo SN, Wang Y, Kosmider S, et al. Circulating tumor DNA analysis guiding adjuvant therapy in stage II colon cancer. N Engl J Med. 2022;386(24):2261–72. doi:10.1056/NEJMoa2200075. [Google Scholar] [PubMed] [CrossRef]
4. Shinji S, Yamada T, Matsuda A, Sonoda H, Ohta R, Iwai T, et al. Recent advances in the treatment of colorectal cancer: a review. J Nippon Med Sch. 2022;89(3):246–54. doi:10.1272/jnms.JNMS.2022_89-310. [Google Scholar] [PubMed] [CrossRef]
5. Shin AE, Giancotti FG, Rustgi AK. Metastatic colorectal cancer: mechanisms and emerging therapeutics. Trends Pharmacol Sci. 2023;44(4):222–36. doi:10.1016/j.tips.2023.01.003. [Google Scholar] [PubMed] [CrossRef]
6. Azwar S, Seow HF, Abdullah M, Faisal Jabar M, Mohtarrudin N. Recent updates on mechanisms of resistance to 5-fluorouracil and reversal strategies in colon cancer treatment. Biology. 2021;10(9):123–31. doi:10.3390/biology10090854. [Google Scholar] [CrossRef]
7. Mengie Ayele T, Tilahun Muche Z, Behaile Teklemariam A, Bogale Kassie A, Chekol Abebe E. Role of JAK2/STAT3 signaling pathway in the tumorigenesis, chemotherapy resistance, and treatment of solid tumors: a systemic review. J Inflamm Res. 2022;15:1349–64. doi:10.2147/JIR.S353489. [Google Scholar] [PubMed] [CrossRef]
8. Kohal R, Bisht P, Gupta GD, Verma SK. Targeting JAK2/STAT3 for the treatment of cancer: a review on recent advancements in molecular development using structural analysis and SAR investigations. Bioorg Chem. 2024;143:107095. doi:10.1016/j.bioorg.2023.107095. [Google Scholar] [PubMed] [CrossRef]
9. Kubczak M, Szustka A, Rogalińska M. Molecular targets of natural compounds with anti-cancer properties. Int J Mol Sci. 2021;22(24):312–22. doi:10.3390/ijms222413659. [Google Scholar] [CrossRef]
10. Islam MR, Akash S, Rahman MM, Nowrin FT, Akter T, Shohag S, et al. Colon cancer and colorectal cancer: prevention and treatment by potential natural products. Chem Biol Interact. 2022;368:110170. doi:10.1016/j.cbi.2022.110170. [Google Scholar] [PubMed] [CrossRef]
11. Lv L, Zhao J, Wang X, Xu L, Fan Y, Wang C, et al. Fang-Xia-Dihuang decoction inhibits breast cancer progression induced by psychological stress via down-regulation of PI3K/AKT and JAK2/STAT3 pathways: an in vivo and a network pharmacology assessment. BIOCELL. 2023;47(9):1977–94. doi:10.32604/biocell.2023.030742. [Google Scholar] [CrossRef]
12. Li Y, Guo F, Chen T, Zhang L, Qin Y. Anthraquinone derivative C10 inhibits proliferation and cell cycle progression in colon cancer cells via the Jak2/Stat3 signaling pathway. Toxicol Appl Pharmacol. 2021;418:115481. doi:10.1016/j.taap.2021.115481. [Google Scholar] [PubMed] [CrossRef]
13. Ma X, Xu W, Jin X, Mu H, Wang Z, Hua Q, et al. Telocinobufagin inhibits osteosarcoma growth and metastasis by inhibiting the JAK2/STAT3 signaling pathway. Eur J Pharmacol. 2023;942:175529. doi:10.1016/j.ejphar.2023.175529. [Google Scholar] [PubMed] [CrossRef]
14. Panda SS, Thangaraju M, Lokeshwar BL. Ursolic acid analogs as potential therapeutics for cancer. Molecules. 2022;27(24):422–34. doi:10.3390/molecules27248981. [Google Scholar] [CrossRef]
15. Katz L, Baltz RH. Natural product discovery: past, present, and future. J Ind Microbiol Biotechnol. 2016;43(3):155–76. doi:10.1007/s10295-015-1723-5. [Google Scholar] [PubMed] [CrossRef]
16. Castellano JM, Ramos-Romero S, Perona JS. Oleanolic acid: extraction, characterization and biological activity. Nutrients. 2022;14(3):623. doi:10.3390/nu14030623. [Google Scholar] [PubMed] [CrossRef]
17. Jung TY, Pham TN, Umeyama A, Shoji N, Hashimoto T, Lee JJ, et al. Ursolic acid isolated from Uncaria rhynchophylla activates human dendritic cells via TLR2 and/or TLR4 and induces the production of IFN-γ by CD4+ naïve T cells. Eur J Pharmacol. 2010;643(2–3):297–303. doi:10.1016/j.ejphar.2010.06.030. [Google Scholar] [PubMed] [CrossRef]
18. Nascimento PG, Lemos TL, Bizerra AM, Arriaga ÂM, Ferreira DA, Santiago GM, et al. Antibacterial and antioxidant activities of ursolic acid and derivatives. Molecules. 2014;19(1):1317–27. doi:10.3390/molecules19011317. [Google Scholar] [PubMed] [CrossRef]
19. Baer-Dubowska W, Narożna M, Krajka-Kuźniak V. Anti-cancer potential of synthetic oleanolic acid derivatives and their conjugates with NSAIDs. Molecules. 2021;26(16):4957. doi:10.3390/molecules26164957. [Google Scholar] [PubMed] [CrossRef]
20. Jiang XF, Shi MQ, Miao S, Yuan YZ, Zhang S, et al. Oleanolic acid inhibits cervical cancer Hela cell proliferation through modulation of the ACSL4 ferroptosis signaling pathway. Biochem Biophys Res Commun. 2021;545:81–8. doi:10.1016/j.bbrc.2021.01.028. [Google Scholar] [CrossRef]
21. Hu C, Cao Y, Li P, Tang X, Yang M, Gu S, et al. Oleanolic acid induces autophagy and Apoptosis via the AMPK-mTOR signaling pathway in colon cancer. J Oncol. 2021;2021:8281718. doi:10.1155/2021/8281718. [Google Scholar] [PubMed] [CrossRef]
22. Li Y, Xu Q, Yang W, Wu T, Lu X. Oleanolic acid reduces aerobic glycolysis-associated proliferation by inhibiting yes-associated protein in gastric cancer cells. Gene. 2019;712:143956. doi:10.1016/j.gene.2019.143956. [Google Scholar] [PubMed] [CrossRef]
23. Xu AL, Xue YY, Tao WT, Wang SQ, Xu HQ. Oleanolic acid combined with olaparib enhances radiosensitization in triple negative breast cancer and hypoxia imaging with 18F-FETNIM micro PET/CT. Biomed Pharmacother. 2022;150:113007. doi:10.1016/j.biopha.2022.113007. [Google Scholar] [PubMed] [CrossRef]
24. Esmaeili H, Nasrollahzadeh Sabet M, Mosaed R, Chamanara M, Hadi S, Hazrati E, et al. Oleanolic acid increases the anticancer potency of doxorubicin in pancreatic cancer cells. J Biochem Mol Toxicol. 2023;37(10):e23426. doi:10.1002/jbt.23426. [Google Scholar] [PubMed] [CrossRef]
25. Livak KJ, Schmittgen TD. Analysis of relative gene expression data using real-time quantitative PCR and the 2−ΔΔCT method. Methods. 2001;25(4):402–8. doi:10.1006/meth.2001.1262. [Google Scholar] [PubMed] [CrossRef]
26. Liu C, Pan Z, Chen Q, Chen Z, Liu W, Wu L, et al. Pharmacological targeting PTK6 inhibits the JAK2/STAT3 sustained stemness and reverses chemoresistance of colorectal cancer. J Exp Clin Cancer Res. 2021;40(1):297. doi:10.1186/s13046-021-02059-6. [Google Scholar] [PubMed] [CrossRef]
27. Liu D, Li W, Zhong F, Yin J, Zhou W, Li S, et al. METTL7B is required for cancer cell proliferation and tumorigenesis in non-small cell lung cancer. Front Pharmacol. 2020;11:178. doi:10.3389/fphar.2020.00178. [Google Scholar] [PubMed] [CrossRef]
28. Garza Treviño EN, Quiroz Reyes AG, Rojas Murillo JA, de la Garza Kalife DA, Delgado Gonzalez P, Islas JF, et al. Cell therapy as target therapy against colon cancer stem cells. Int J Mol Sci. 2023;24(9):8163. doi:10.3390/ijms24098163. [Google Scholar] [CrossRef]
29. Wang L, Zhao L, Lin Z, Yu D, Jin M, Zhou P, et al. Targeting DCLK1 overcomes 5-fluorouracil resistance in colorectal cancer through inhibiting CCAR1/β-catenin pathway-mediated cancer stemness. Clin Transl Med. 2022;12(5):e743. doi:10.1002/ctm2.743. [Google Scholar] [PubMed] [CrossRef]
30. Huang B, Lang X, Li X. The role of IL-6/JAK2/STAT3 signaling pathway in cancers. Front Oncol. 2022;12:1023177. doi:10.3389/fonc.2022.1023177. [Google Scholar] [PubMed] [CrossRef]
31. Chang JC. Cancer stem cells: role in tumor growth, recurrence, metastasis, and treatment resistance. Medicine. 2016;95(1S):S20–5. doi:10.1097/MD.0000000000004766. [Google Scholar] [PubMed] [CrossRef]
32. Crudele F, Bianchi N, Astolfi A, Grassilli S, Brugnoli F, Terrazzan A, et al. The molecular networks of microRNAs and their targets in the drug resistance of colon carcinoma. Cancers. 2021;13(17):4355. doi:10.3390/cancers13174355. [Google Scholar] [PubMed] [CrossRef]
33. Zhou H, Tan L, Liu B, Guan XY. Cancer stem cells: recent insights and therapies. Biochem Pharmacol. 2023;209:115441. doi:10.1016/j.bcp.2023.115441. [Google Scholar] [PubMed] [CrossRef]
34. Huang T, Song X, Xu D, Tiek D, Goenka A, Wu B, et al. Stem cell programs in cancer initiation, progression, and therapy resistance. Theranostics. 2020;10(19):8721–43. doi:10.7150/thno.41648. [Google Scholar] [PubMed] [CrossRef]
35. Gao Q, Zhan Y, Sun L, Zhu W. Cancer stem cells and the tumor microenvironment in tumor drug resistance. Stem Cell Rev Rep. 2023;19(7):2141–54. doi:10.1007/s12015-023-10593-3. [Google Scholar] [PubMed] [CrossRef]
36. Dashtaki ME, Ghasemi S. CRISPR/Cas9-based gene therapies for fighting drug resistance mediated by cancer stem cells. Curr Gene Ther. 2023;23(1):41–50. doi:10.2174/1566523222666220831161225. [Google Scholar] [PubMed] [CrossRef]
37. Zhang Y, Liu X, Dang W, Liu L. Thymoquinone inhibits lung cancer stem cell properties via triggering YAP degradation. Carcinogenesis. 2023;44(5):426–35. doi:10.1093/carcin/bgad026. [Google Scholar] [PubMed] [CrossRef]
38. Liu X, Su K, Sun X, Jiang Y, Wang L, Hu C, et al. Sec62 promotes stemness and chemoresistance of human colorectal cancer through activating Wnt/β-catenin pathway. J Exp Clin Cancer Res. 2021;40(1):132. doi:10.1186/s13046-021-01934-6. [Google Scholar] [PubMed] [CrossRef]
39. Mahmoud M, Abdollah MRA, Elsesy ME, Abou El Ella DA, Zada SK, Tolba MF. The natural isoflavone Biochanin-A synergizes 5-fluorouracil anticancer activity in vitro and in vivo in Ehrlich solid-phase carcinoma model. Phytother Res. 2022;36(3):1310–25. doi:10.1002/ptr.7388. [Google Scholar] [PubMed] [CrossRef]
40. Chen H, Li R, Zhang F, Yao Q, Guo Y. A scientometric visualization analysis for natural products on cancer research from 2008 to 2020. Front Pharmacol. 2021;12:650141. doi:10.3389/fphar.2021.650141. [Google Scholar] [PubMed] [CrossRef]
41. Kim GJ, Jo HJ, Lee KJ, Choi JW, An JH. Oleanolic acid induces p53-dependent apoptosis via the ERK/JNK/AKT pathway in cancer cell lines in prostatic cancer xenografts in mice. Oncotarget. 2018;9(41):26370–86. doi:10.18632/oncotarget.25316. [Google Scholar] [PubMed] [CrossRef]
42. Piet M, Paduch R. Ursolic and oleanolic acids in combination therapy inhibit migration of colon cancer cells through down-regulation of the uPA/uPAR-dependent MMPs pathway. Chem Biol Interact. 2022;368:110202. doi:10.1016/j.cbi.2022.110202. [Google Scholar] [PubMed] [CrossRef]
43. Fan X, Fu H, Xie N, Guo H, Fu T, Shan Y. Inhibition of JAK2/STAT3 signaling pathway by panaxadiol limits the progression of pancreatic cancer. Aging. 2021;13(19):22830–42. doi:10.18632/aging.203575. [Google Scholar] [PubMed] [CrossRef]
44. Xiong J, Zhang X, Zhang Y, Wu B, Fang L, Wang N, et al. Aryl hydrocarbon receptor mediates Jak2/STAT3 signaling for non-small cell lung cancer stem cell maintenance. Exp Cell Res. 2020;396(1):112288. doi:10.1016/j.yexcr.2020.112288. [Google Scholar] [PubMed] [CrossRef]
45. Wang H, Zhong W, Zhao J, Zhang H, Zhang Q, Liang Y, et al. Oleanolic acid inhibits epithelial-mesenchymal transition of hepatocellular carcinoma by promoting iNOS dimerization. Mol Cancer Ther. 2019;18(1):62–74. doi:10.1158/1535-7163.MCT-18-0448. [Google Scholar] [PubMed] [CrossRef]
46. Han YW, Liu XJ, Zhao Y, Li XM. Role of Oleanolic acid in maintaining BBB integrity by targeting p38MAPK/VEGF/Src signaling pathway in rat model of subarachnoid hemorrhage. Eur J Pharmacol. 2018;839:12–20. doi:10.1016/j.ejphar.2018.09.018. [Google Scholar] [PubMed] [CrossRef]
47. Tiacci E, Ladewig E, Schiavoni G, Penson A, Fortini E, Pettirossi V, et al. Pervasive mutations of JAK-STAT pathway genes in classical Hodgkin lymphoma. Blood. 2018;131(22):2454–65. doi:10.1182/blood-2017-11-814913. [Google Scholar] [PubMed] [CrossRef]
48. Rezaeepoor M, Hoseini-Aghdam M, Sheikh V, Eftekharian MM, Behzad M. Evaluation of interleukin-23 and JAKs/STATs/SOCSs/ROR-γt expression in Type 2 diabetes mellitus patients treated with or without sitagliptin. J Interferon Cytokine Res. 2020;40(11):515–23. doi:10.1089/jir.2020.0113. [Google Scholar] [PubMed] [CrossRef]
Cite This Article
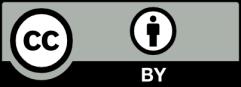
This work is licensed under a Creative Commons Attribution 4.0 International License , which permits unrestricted use, distribution, and reproduction in any medium, provided the original work is properly cited.