Open Access
REVIEW
The pathogenesis of chronic subdural hematoma in the perspective of neomembrane formation and related mechanisms
1 School of Medicine, Southern University of Science and Technology, Shenzhen, 518055, China
2 Department of Emergency, Shenzhen People’s Hospital, The Second Clinical Medical College of Jinan University, The First Affiliated Hospital of Southern University of Science and Technology, Shenzhen, 518055, China
* Corresponding Author: BO DU. Email:
BIOCELL 2024, 48(6), 889-896. https://doi.org/10.32604/biocell.2024.050097
Received 27 January 2024; Accepted 11 April 2024; Issue published 10 June 2024
Abstract
Chronic subdural hematoma (CSDH) is a disease characterized by capsuled blood products that progressively occupy the intracranial space, causing intracranial hypertension and compression in the brain. CSDH frequently occurs in all demographics, especially in the elderly, but the pathogenesis of CSDH remains unclear. In this review, we discuss the origin, development, and current treatment strategies of CSDH. For the first time, we analyzed the cellular and molecular compositions of hematoma membranes with a focus on neomembrane formation, a complex early-stage interactive event in hematoma pathogenesis. We hypothesize that in patients with CSDH, dural border cells (DBCs) might be induced to synthesize collagen or serum proteins might accumulate at the dura and arachnoid layers at the site of injury, thereby encapsulating the hemorrhage. Membrane formation may trigger inflammatory responses after subdural hemorrhage, promoting fibroblast-involved extracellular matrix (ECM) deposition and aberrant angiogenesis within the outer membrane. Consequently, ECM deposition and angiogenesis mutually influence each other and are modulated by inflammatory processes. By illustrating the complex and interactive mechanism of neomembrane formation, we aim to provide a novel insight into CSDH pathogenesis and propose directions for future research as well as advancements in treatment strategies for this disease.Keywords
Abbreviation List
PKR | Activated protein kinase |
ANG | Angiopoietin |
BHC | Burr-hole craniotomy |
bFGF | Basic fibroblast growth factor |
ICTP | Carboxyterminal telopeptide of type I collagen |
CSF | Cerebrospinal fluid |
CSDH | Chronic subdural hematoma |
DAMP | Damage-associated molecular pattern |
DBC | Dural border cell |
EC | Endothelial cell |
EDN | Eosinophil-derived neurotoxin |
ECM | Extracellular matrix |
ERK | Extracellular signal-regulated kinase |
GAG | Glycosaminoglycan |
H&E | Hematoxylin and eosin |
HIF | Hypoxia-inducible factor |
IL-5 | Interleukin-5 |
MMP | Matrix metalloproteinase |
mTOR | Mechanistic target of rapamycin |
MMA | Middle meningeal artery |
MEK | Mitogen-activated protein kinase kinase |
NF-κB | Nuclear factor-kappaB |
PI3K-Akt | Phosphoinositide 3-kinase-protein kinase B |
PlGF | Placental growth factor |
PICP | Procollagen I, C-terminal propeptide |
PIIINP | Procollagen III, N-terminal propeptide |
PKB | Protein kinase B |
Ras | Rat sarcoma |
SEM | Scanning electron microscopy |
scRNA-seq | Single-cell RNA sequencing |
sVEGFR-1 | Soluble VEGF receptor-1 |
TGF-β | Transforming growth factor-β |
TNF-α | Tumor necrosis factor-α |
TDC | Twist drill craniotomy |
VEGF | Vascular endothelial growth factor |
Chronic Subdural Hematoma (CSDH) is the persistent accumulation of blood products in the subdural space, which impairs motor function and cognition. Patients may exhibit a variety of symptoms, including falls, gait abnormalities, acute memory loss, and others [1]. The general incidence rate of CSDH is 1.7 to 20.6 per 100,000 person-years, with males affected 3 times more than females [2–4]. It is worth attention that CSDH threatens the elderly especially [3–5]. The hematoma is enclosed by an outer and an inner membrane. Substantial evidence has associated the development of CSDH with the rupture of fragile neovascular structures and inflammatory responses, although the precise etiology of CSDH remains unclear [6–8]. Various treatment strategies have been utilized for CSDH. For decades, surgical interventions have been preferred for managing CSDH, with burr-hole craniotomy (BHC), twist drill craniotomy (TDC), and minicraniotomy being the predominant approaches [9,10]. BHC and TDC aim to evacuate hematoma fluid via cranial perforation. In cases of failure, mini craniotomy would be performed as a rescue procedure, entailing both outer and inner membranectomy [3,11]. Recently, middle meningeal artery (MMA) embolism and neuroendoscopy-assisted membranectomy have been increasingly applied [7,12,13]. Perioperative MMA embolization before BHC or craniotomy is linked to reduced reoperation rates compared to BHC or craniotomy alone, with no significant increase in complication rates [14,15]. Three RCTs on MMA embolization, presented at this year’s International Stroke Conference, demonstrated promising results in reducing recurrence rates, whether as an independent treatment or as an adjunct therapy [16–18]. Neuroendoscopy-assisted membranectomy also demonstrated a significantly reduced rate of recurrence than BHC [7]. Despite various surgical approaches, the recurrent rate remains high, ranging from 5% to 33% overall, indicating a potential bottleneck in treatment effectiveness [19–21]. In recent years, the treatment paradigm has shifted from mere drainage to more precise membrane management. Specifically, emphasis is placed on the importance of proper membrane handling such as inner membranectomy, as evidenced by an increasing volume of literature demonstrating its potential to significantly reduce recurrence rates [7,22,23].
To achieve better treatment outcomes for CSDH, studying the formation of hematoma membrane structure is imperative. Such an investigation may offer insights into the mechanisms underlying hematoma formation, and potential interventions, such as inner membranectomy, to eradicate it. Therefore, in this review, we summarize the pathophysiology of CSDH, with particular emphasis on neomembrane formation. The outer membrane plays a critical role in hematoma formation and growth due to its abundance of fragile vasculatures [24]. The inner membrane, which is thinner and less vascularized compared to the outer membrane, is often overlooked but may contribute to maintaining hematoma integrity. Current surgical interventions typically involve perforating the outer membrane, a process in which the dura then seals, recreating a closed hematoma cavity. Subsequent new blood leakage into this cavity often leads to the recurrence of CSDH and necessitates reoperation. Making radical incisions in the inner membrane may disrupt hematoma integrity, leading to the discharge of freshly and gradually leaked blood from the outer membrane. We hypothesize that this blood may be reabsorbed by the arachnoid. As the hematoma diminishes in size, the outer and inner membranes adhere to the brain, allowing the brain to re-expand, thus leading to a successful recovery. In this review, we highlight that neomembrane formation may be an interactive outcome between inflammation, extracellular matrix (ECM) deposition, and aberrant angiogenesis. Our objective is to offer novel insights into understanding CSDH formation and refining surgical strategies, therefore optimizing patients’ prognosis, and enhancing preventive measures.
To the innermost of the dura is a layer of dural border cells (DBCs) occupying the subdural space, which, identified by previous reports, is not a hollow planetary between the dura and the arachnoid [25,26]. Dural border cells lack tight junctions and filamentous materials, making them easy to tear and fill with blood and cerebrospinal fluid (CSF). Head trauma is a major contributor to bleeding within the DBC layer [27]. Parenchymal atrophy may result in CSF exudation and create negative pressure around the loose dural border cells, making them susceptible to delamination by even minor additional force, thereby facilitating spontaneous bleeding [28,29]. This potentially explains why individuals over 50 years old with brain atrophy have a higher incidence of CSDH compared to younger individuals [30]. In younger individuals, the brain is tightly enclosed by the dura, leaving minimal subdural space, thus confining the hematoma to a localized area. In contrast, in the elderly, even minor bleeding tends to result in a hematoma that spreads diffusely due to increased subdural space.
In this article, we hypothesize that neomembrane formation resulted from ECM deposition by DBCs following interaction with blood products. Given that DBCs are fibroblast-like cells, we speculate that they may become activated and subsequently produce ECM to encapsulate the blood [3].
The subdural space is inherently limited by cerebral falx and tentorium cerebelli; consequently, blood accumulation within this confined area leads to expansion encompassing the hemisphere only on the affected side instead of uniform expansion across the entire skill. Hematoma membrane thickening is supposed to be associated with the following three primary factors: inflammation, ECM deposition, and aberrant angiogenesis (Fig. 1).
Figure 1: Neomembrane formation involves inflammation, ECM deposition, and aberrant angiogenesis and their interactions. When hemorrhage occurs, inflammatory cells are recruited and activated, fostering a pro-inflammatory environment at the site. This process subsequently stimulates ECM deposition and leads to aberrant angiogenesis. The primary source of IL-8 is macrophages/monocytes, with additional contributions from mast cells and neutrophils. Eosinophils produce TGF-β, which activates Smad pathways in fibroblasts, hence enhancing the production of collagen as ECM. Collagen, interspersed with fibroblasts, constitutes the primary component of hematoma membranes. Macrophages produce IL-6, while neutrophils secrete VEGF, both of which modulate aberrant angiogenesis via the NF-κB and PI3K/Akt pathways in endothelial cells.
Accumulation of blood in the DBCs initiates inflammatory responses. Upon subdural hemorrhage, fibroblasts and inflammatory cells like eosinophils, macrophages, and lymphocytes are recruited to the injured site [7,8,31]. In the outer membranes of CSDH patients, Kawaguchi et al. identified eosinophils through Giemsa staining in a study involving 38 individuals. They theorized that Interleukin-5 (IL-5) induced eosinophil infiltration into the outer membrane, leading to degranulation of eosinophil-derived neurotoxin (EDN) into the hematoma cavity [32]. Furthermore, Yamashima et al. observed eosinophils in both membranes with electron microscopy, suggesting their potential role in promoting local hyperfibrinolysis and liquefaction [33]. Sarkar et al. observed eosinophils in 60% (30 out of 50 cases) of the outer membranes in their CSDH sample, with a subset exhibiting degranulation. Infiltration severity increased with time post-trauma among CSDH cases. In a subset of CDSH patients, Sakar et al. observed infiltration of neutrophils, plasma cells, and lymphocytes by toluidine blue staining or hematoxylin and eosin (H&E) stained [34]. Loh et al. identified fibroblasts, mast cells, migrating erythrocytes, platelets, and eosinophils in CSDH outer membranes using electron microscopy [35]. Moskala et al. used scanning electron microscopy (SEM) to document vessel formation and accumulation of macrophages and leukocytes in the capsules of CSDH patients [24].
Evidence indicates a heightened pro-inflammatory state within the hematoma, characterized by significant infiltration of inflammatory cells and detection of numerous inflammatory factors in the hematoma fluid. Pro-inflammatory factors like IL-5, IL-6, IL-7, IL-8, and tumor necrosis factor-α (TNF-α) were found to be significantly elevated compared with the peripheral blood [36–38]. In one study, it was found that CSDH might be initiated by IL-8-induced neutrophil respiratory bursts. Concurrently, IL-8 has been implicated in the promotion of neovascularization. Initially, the DBCs secrete IL-8, which accumulates and promotes neovessel growth. The increase in local IL-8 concentration then recruits lymphocytes to the neomembrane. Oxidative rupture may occur in these neutrophils located in the walls of neovessels. The release of lysosomes from neutrophils can damage fragile neocapillaries, resulting in the influx of plasma and blood cells into the subdural space [39]. Anti-inflammatory mediators such as IL-10 and IL-13 were consistently found to be significantly lower [36,38,40]. Furthermore, reductions were observed in pro-inflammatory factors within the hematoma fluid, including IL-1β, IL-2, and IL-4; the implications of these changes remain unclear and require further investigation [36]. Current clinical management of chronic subdural hematoma indicates that combined therapy of atorvastatin plus low-dose dexamethasone presented more effective than atorvastatin alone [41].
Herein, we primarily focus on eosinophils, macrophages/monocytes, neutrophils, and mast cells, given the evidence linking these cell types to fibroblasts in ECM deposition and aberrant angiogenesis.
Prior studies have consistently demonstrated that hematoma membranes are composed predominantly of collagen and fibroblasts [31,42]. DBCs may undergo pathological splitting following injury, encapsulating subdural exudates and forming distinct outer and inner membranes.
Examining precursors and degradations of collagen in the hematoma membrane, researchers revealed a persistent process of collagen synthesis. In patients with CSDH, the outer membrane exhibited high levels of type 1 and type 3 procollagen [7]. Researchers observed a significant elevation in the levels of procollagen propeptides for type I and III procollagens (PICP and PIIINP, respectively) (p < 0.001), alongside a more moderate increase in the carboxyterminal telopeptide of type I collagen (ICTP) (p = 0.002), compared to serum levels. The ratio of PICP/ICTP can indicate the balance between collagen synthesis and degradation. The ratio was remarkably higher in the outer membrane than in the peripheral blood, suggesting a predominance of synthesis over degradation in hematoma fluid [43]. Furthermore, in hematoma fluid, changes in levels of carbonic anhydrase I, transforming growth factor-β (TGF-β)-induced protein ig-h3, and the component system were observed, possibly indicating a combination of inflammation and fibrosis during hematoma progression [44].
Inflammatory mediators can stimulate fibroblasts to initiate proliferation and collagen production. Osuka et al. identified TGF-β and eotaxin-3 in the outer membrane, noting higher concentrations in hematoma fluid compared to CSF, and observed altered expression of Smad proteins in fibroblasts via Western blotting and immunohistochemistry. Eotaxin-3′s chemotactic effect results in the frequent presence of eosinophils in the CSDH outer membrane, which are the primary producers of TGF-β in the hematoma. TGF-β then activates Smad pathways in fibroblasts and promotes fibrosis [45]. Fibroblasts or fibroblast-like cells can directly sense damage-associated molecular patterns (DAMPs) and activate innate and adaptive immune responses. Furthermore, activated fibroblasts or fibroblast-like cells are capable of augmenting immune cell accumulation by promoting cell adhesion [46]. During bleeding site healing, collagen might deposit at the wound site and form granulation tissue instead of repairing the DBC layer. ECM was found to be abundant in procollagen and glycosaminoglycans in the subdural fluid of patients with head injury [47]. Glycosaminoglycans (GAGs) comprise a major component of the ECM, which in turn regulates inflammation and tissue repair, influencing cell migration, proliferation, and transformation [48]. If the fluid in the capsule is not absorbed in time, the subdural space may progress into CSDH.
The cellular origin of fibroblasts in the fibrotic scar may include meningeal fibroblasts, perivascular fibroblasts, pericytes, endothelial cells, and circulating blood fibrocytes [46]. Pericyte and invading meningeal fibroblast-derived cells have been proposed to be capable of active proliferation and excessive deposition of ECM proteins such as collagen type IV, fibronectin, and laminin, thus contributing to the formation of connective tissue after brain trauma [49]. Given that DBCs are fibroblasts, they potentially participate in inflammatory activities and secrete collagen.
Clinical researchers have applied therapeutic strategies targeting ECM deposition to address disease recurrence. Patient prognosis may be improved through postoperative targeted pharmacological interventions, including antiangiogenic and antifibrinolytic medications [7].
Angiogenesis is initiated by inflammatory mediators, which may result in the proliferation of capillaries from the dura or middle meningeal artery into the neomembrane. Local hypoxia may serve as a pivotal trigger for angiogenesis as it prompts the release of IL-6, TNF-α, and hypoxia-inducible factor (HIF) [27]. Pro-inflammatory factors such as IL-1, IL-6, and IL-8 secreted by monocytes or macrophages within the outer membrane can facilitate inflammation as well as angiogenesis [13,50]. Evidence has shown that they can induce neomembrane formation and aberrant angiogenesis in CSDH [8].
Vascular endothelial growth factor (VEGF) could be secreted by neutrophils in the hematoma fluid, and by macrophages and endothelial cells (ECs) in the outer membrane. However, its receptor, VEGFR is exclusively expressed in endothelial cells [28,51,52]. A higher level of VEGF was detected in hematoma fluid than in serum [8]. VEGF modulates angiogenesis via the phosphoinositide 3-kinase-protein kinase B (PI3K-Akt), nuclear factor-kappa B (NF-κB), and rat sarcoma (Ras)/mitogen-activated protein kinase kinase (MEK)/extracellular signal-regulated kinase (ERK) pathways as part of post-injury repair in ECs [53]. Through Western blotting and immunohistochemistry, Funai et al. identified the expression of PI3K, protein kinase B (PKB), Akt, VEGF, and VE-cadherin in the outer membrane ECs of CSDH patients. These findings suggest a potential involvement of the PI3K/Akt pathway in the expansion or recurrence of CSDH [54]. In a separate investigation by Osuka et al., immunohistochemical analysis revealed that p-NF-κB levels in the outer membrane diminished following CSDH treatment and after VEGF blockade. Double-stranded RNA-activated protein kinase (PKR) may activate the NF-κB pathway in ECs via VEGF, thereby mediating EC proliferation and angiogenesis [55].
In addition to VEGF, other pro-angiogenic factors, such as angiopoietin (ANG)-1 and ANG-2 have also been detected within the hematoma. Ang-1 promotes vesicular stability by supporting ECs survival and barrier formation. In contrast, ANG-2 disrupts vascular architecture and plays a role in vessel remodeling. Both operate via their shared receptor, Tie-2 [56,57]. In the outer membranes of CSDH patients, expression of VEGF was observed only concurrently with the presence of ANG-1 and ANG-2 mRNA. The average ANG-1/ANG-2 ratio was found to be 0.48 in the hematoma membranes, in contrast to 1.9 in normal CSF, indicating a pro-angiogenic shift within the hematoma environment [58]. Tie2, Akt, and mechanistic targets of rapamycin (mTOR) mRNA were detected in the outer membrane, especially localizing in ECs [53]. The matrix metalloproteinase (MMP) family has also been recognized as a significant proangiogenic factor capable of initiating an angiogenic switch in a previously inactive vascular bed [27]. At the wound site, collagen activates fibroblasts and immune cells to engage in degradation via MMPs, which remodel the ECM structure and initiate the healing process [59]. Within the MMP family, MMP-2 and MMP-9 were only detected in the outer hematoma membrane [60]. These enzymes, when secreted into the cavity fluid, were present in higher concentrations than in serum (p < 0.01) [61]. Under stimulation by VEGF, ANG, or basic fibroblast growth factor (bFGF), MMPs facilitate the release of pericytes from the basement membrane through proteolytic degradation [27].
In addition to the enhancement of pro-angiogenic factors, the inhibition of anti-angiogenic factors has also been widely documented. Soluble VEGF receptor-1 (sVEGFR-1) acts as an antagonist of VEGF and placental growth factor (PIGF). Levels of sVEGFR-1 and PIGF were both significantly elevated in hematoma fluid compared to serum, while the ratio of sVEGFR-1 to PIGF was notably lower in hematoma fluid than in serum (p < 0.0001). This pattern reflects the active pathological neovascularization observed in CSDH cases [62].
CSDH is an intracranial hemorrhagic condition that significantly diminishes patients’ quality of life and imposes considerable medical and economic burdens on society and families [2]. Recent decades have seen progress in understanding CSDH’s pathophysiology, laying the groundwork for in-depth exploration of its neomembrane pathogenesis. The main reported cells involved in neomembrane formation include fibroblasts, eosinophils, neutrophils, macrophages, and endothelial cells. These cells collaborate to establish an interrelated network of inflammation, ECM deposition, and aberrant angiogenesis, contributing collectively to neomembrane formation.
Elevated levels of pro-inflammatory cytokines such as IL-5, IL-6, IL-7, and IL-8, alongside decreased levels of anti-inflammatory cytokines like IL-13, have been reported by many researchers [36–38]. These findings suggest a predominance of pro-inflammatory activity within the hematoma. However, data on specific inflammatory markers show variability across studies. For instance, Kitazono et al. [38] reported increased levels of TNF-α, in contrast to Stanisic et al. [36], who reported a decrease. Similarly, Wada et al. [40] found reduced levels of IL-10, while Kitazono et al. [38] reported an increase. These discrepancies could stem from limitations such as small sample sizes or differences in study design. Advanced methodologies, like single-cell RNA sequencing (scRNA-seq), or analyses involving larger sample cohorts, may help clarify these inconsistencies.
Cytokines such as IL-5 secreted by eosinophils may facilitate fibroblast deposition of ECM, which involves procollagens and glycosaminoglycans that form the matrix for neomembrane development [45]. Additionally, molecules like carbonic anhydrase I and TGF-β-induced protein ig-h3 identified in hematoma fluids could clarify ECM deposition dynamics [44]. To better understand neomembrane formation, it is necessary to deepen our knowledge of the cellular architecture of the dura mater and the outer and inner membranes. The origin of fibroblasts in CSDH remains unclear. Innovative methodologies such as scRNA-seq and lineage tracing could offer a more comprehensive view of the cellular interactions and processes underlying neomembrane formation.
Currently, knowledge about the cellular composition of the outer membrane is incomplete. There is a general agreement that hemorrhage triggers inflammation [60,63,64]. Existing research has predominantly concentrated on cytokines and the perpetuating cycle of hyperfibrinolysis, coagulation, neovascularization, and subsequent hemorrhage. Moreover, the development of animal models and statistical frameworks for CSDH could significantly contribute by delineating the chronological progression of changes in both the membrane and the cellular constituents of hematoma fluid.
With an evolving understanding of neomembrane formation, treating strategies for CSDH could be refined and tailored more effectively. Therapeutic approaches could specifically target the key mechanisms involved: inflammation, ECM deposition, and aberrant angiogenesis, aiming to alleviate symptoms at an early stage. Additionally, surgical techniques may be optimized considering an enhanced understanding of the CSDH membrane. For instance, making radial incisions in the dense inner membrane could facilitate brain re-expansion while simultaneously preventing herniation. We also hypothesize that blood leaking from the outer membrane could be drained through incisions in the inner membrane and then reabsorbed by the arachnoid layer. Some outcomes from inner membranectomy studies support this theory, but further investigation is required [7,12,13]. The absence of improved outcomes in certain studies of inner membranectomy may be attributed to non-radial incisions, potentially leading to brain herniation [22,65]. Ultimately, reconciling research discrepancies and achieving comprehensive patient outcomes in CSDH necessitates advancing our understanding of the involved membranes.
In this paper, we explore the pathogenesis of CSDH by examining the reported cellular and cytokine composition of the hematoma membranes, with a focus on the outer membrane characteristics. We aim to shed light on the origin, progression, and current treatment strategies of CSDH. Our analysis suggests that the neomembrane formation is governed by three intertwined processes: inflammation responses, ECM deposition, and aberrant angiogenesis. The interaction among these processes is intricate and dynamic, influencing the disease’s trajectory. Thus, we aim to provide means to disrupt this pathological cycle, thereby paving the way for a complete resolution of the condition.
Acknowledgement: The authors greatly appreciate Servier Medical Art (smart.servier.com/) for providing image materials for drafting the figure. The authors also thank Zeyuan Zheng from the University of Pennsylvania for enhancing the manuscript’s readability and Xuyang Chen from the Southern University of Science and Technology for helping design the graphical abstract.
Funding Statement: This research was funded by Guangdong Natural Science Foundation (2020A1515010289), Shenzhen Key Medical Discipline Construction Fund (Shenzhen Key Medical Discipline 2020–2024), and Shenzhen Science and Technology Innovation Foundation (Shenzhen Technology Key Project JSGG20211029095400001).
Author Contributions: MY.H. and JF.D. drafted and completed the manuscript. MY.H. designed the Figure. JF.D. retrieved literatures. B.D. conceived the topic and revised the manuscript critically. XL.Z., J.W. and JZ.X. supervised the manuscript. All authors contributed to the article and approved the submitted version.
Availability of Data and Materials: All data generated or analyzed during this study are included in this published article.
Ethics Approval: Not applicable.
Conflicts of Interest: The authors declare that the research was conducted in the absence of any commercial or financial relationships that could be construed as a potential conflict of interest.
References
1. Turgut M, Akhaddar A, Hall WA, Turgut AT. Subdural hematoma: past to present to future management. Available from: https://link.springer.com/10.1007/978-3-030-79371-5 [Accessed 2021]. [Google Scholar]
2. Feghali J, Yang WY, Huang J. Updates in chronic subdural hematoma: epidemiology, etiology, pathogenesis, treatment, and outcome. World Neurosurg. 2020;141:339–45. doi:10.1016/j.wneu.2020.06.140 [Google Scholar] [PubMed] [CrossRef]
3. Kolias AG, Chari A, Santarius T, Hutchinson PJ. Chronic subdural haematoma: modern management and emerging therapies. Nat Rev Neurol. 2014;10(10):570–8. doi:10.1038/nrneurol.2014.163 [Google Scholar] [PubMed] [CrossRef]
4. Zhu FL, Wang HF, Li WC, Han S, Yuan JY, Zhang CY, et al. Factors correlated with the postoperative recurrence of chronic subdural hematoma: an umbrella study of systematic reviews and meta-analyses. EClinicalMedicine. 2022;43(12):101234. doi:10.1016/j.eclinm.2021.101234 [Google Scholar] [PubMed] [CrossRef]
5. Mauricewilliams RS, Kitchen N. The scope of neurosurgery for elderly people. Age Ageing. 1993;22(5):337–42. doi:10.1093/ageing/22.5.337 [Google Scholar] [PubMed] [CrossRef]
6. Colamaria A, Sacco M, Iodice S, Fochi NP, Carbone F. Cerebrospinal fluid leak as a driving factor in chronic subdural hematoma formation: a histological study. Surg Neurol Int. 2021;12:578. doi:10.25259/SNI_950_2021 [Google Scholar] [PubMed] [CrossRef]
7. Du B, Xu JZ, Hu JT, Zhong XL, Liang J, Lei PF, et al. A clinical study of the intra-neuroendoscopic technique for the treatment of subacute-chronic and chronic septal subdural hematoma. Front Neurol. 2020;10:1408. doi:10.3389/fneur.2019.01408 [Google Scholar] [PubMed] [CrossRef]
8. Koch AE, Polverini PJ, Kunkel SL, Harlow LA, Dipietro LA, Elner VM, et al. Interleukin-8 as a macrophage-derived mediator of angiogenesis. Science. 1992;258(5089):1798–801. doi:10.1126/science.1281554 [Google Scholar] [PubMed] [CrossRef]
9. Scerrati A, Visani J, Ricciardi L, Dones F, Rustemi O, Cavallo MA, et al. To drill or not to drill, that is the question: nonsurgical treatment of chronic subdural hematoma in the elderly. A systematic review. Neurosurg Focus. 2020;49(4):E7. doi:10.3171/2020.7.FOCUS20237 [Google Scholar] [PubMed] [CrossRef]
10. Miah IP, Blanter A, Tank Y, van Zwet EW, Rosendaal FR, Peul WC, et al. Change in hematoma size after dexamethasone therapy in chronic subdural hematoma subtypes: a prospective study in symptomatic patients. J Neurotrauma. 2023;40(3–4):228–39. doi:10.1089/neu.2022.0024 [Google Scholar] [PubMed] [CrossRef]
11. Brennan PM, Kolias AG, Joannides AJ, Shapey J, Marcus HJ, Gregson BA, et al. The management and outcome for patients with chronic subdural hematoma: a prospective, multicenter, observational cohort study in the United Kingdom. J Neurosurg. 2017;127(4):732–9. doi:10.3171/2016.8.JNS16134 [Google Scholar] [PubMed] [CrossRef]
12. Onyinzo C, Berlis A, Abel M, Kudernatsch M, Maurer CJ. Efficacy and mid-term outcome of middle meningeal artery embolization with or without burr hole evacuation for chronic subdural hematoma compared with burr hole evacuation alone. J NeuroInterventional Surg. 2022;14(3):297–300. doi:10.1136/neurintsurg-2021-017450 [Google Scholar] [PubMed] [CrossRef]
13. Tamura R, Sato M, Yoshida K, Toda M. History and current progress of chronic subdural hematoma. J Neurol Sci. 2021;429:118066. doi:10.1016/j.jns.2021.118066 [Google Scholar] [PubMed] [CrossRef]
14. Sattari SA, Yang WY, Shahbandi A, Feghali J, Lee RP, Xu RS, et al. Middle meningeal artery embolization versus conventional management for patients with chronic subdural hematoma: a systematic review and meta-analysis. Neurosurg. 2023;92(6):1142–54. doi:10.1136/neurintsurg-2021-017352. [Google Scholar] [CrossRef]
15. Ironside N, Nguyen C, Do Q, Ugiliweneza B, Chen CJ, Sieg EP, et al. Middle meningeal artery embolization for chronic subdural hematoma: a systematic review and meta-analysis. J NeuroInterv Surg. 2021;13(10):951–7. doi:10.1136/neurintsurg-2021-017352 [Google Scholar] [PubMed] [CrossRef]
16. Knopman J, Davies JM, Harbaugh RE, Hassan AE, Khalessi A, Mokin M, et al. Embolization of the middle meningeal artery with onyx liquid embolic system in the treatment of subacute and chronic subdural hematoma (EMBOLISE). Available from: https://www.abstractsonline.com/pp8/#!/10942/presentation/3767 [Accessed 2024]. [Google Scholar]
17. Mao Y, Liu JM.Managing non-acute subdural hematoma using liquid materials: a chinese randomized trial of middle meningeal artery treatment (MAGIC-MT). Available from: https://www.abstractsonline.com/pp8/#!/10942/presentation/3768 [Accessed 2024]. [Google Scholar]
18. Arthur AS, Fiorella D. The SQUID trial for the embolization of the MMA for the treatment of CSDH (STEM). Available from: https://www.abstractsonline.com/pp8/#!/10942/presentation/3770 [Accessed 2024]. [Google Scholar]
19. Duerinck J, van der Veken J, Schuind S, Van Calenbergh F, van Loon J, Du Four S, et al. Randomized trial comparing burr hole craniostomy, minicraniotomy, and twist drill craniostomy for treatment of chronic subdural hematoma. Neurosurg. 2022;91(2):304–11. doi:10.1227/neu.0000000000001997 [Google Scholar] [PubMed] [CrossRef]
20. Al-Salihi MM, Al-Jebur MS, Al-Salihi Y, Saha R, Hammadi F, Al Hajali A, et al. Comparison of burr-hole craniostomy versus twist-drill craniostomy operations for patients with chronic subdural hematoma: a systematic review and network meta-analysis. World Neurosurg. 2023;176:229–36.e7. doi:10.1016/j.wneu.2023.05.022 [Google Scholar] [PubMed] [CrossRef]
21. Zhang JB, Liu XM, Fan XY, Fu K, Xu CS, Hu Q, et al. The use of endoscopic-assisted burr-hole craniostomy for septated chronic subdural haematoma: a retrospective cohort comparison study. Brain Res. 2018;1678:245–53. doi:10.1016/j.brainres.2017.10.017 [Google Scholar] [PubMed] [CrossRef]
22. Kusano Y, Horiuchi T, Seguchi T, Kakizawa Y, Tanaka Y, Hongo K. Local brain herniation after partial membranectomy for organized chronic subdural hematoma in an adult patient: case report and review of the literature. Brain Inj. 2010;24(9):1118–21. doi:10.3109/02699052.2010.490515 [Google Scholar] [PubMed] [CrossRef]
23. Hong KT, Hsu SW, Chen CH, Hueng DY, Chen YH, Ju DT, et al. Endoscope-assisted manipulation of chronic subdural hematomas provides a novel solution for eliminating the septum and inner membrane leading to reduced recurrence. In Vivo. 2022;36(6):2774–9. doi:10.21873/invivo.13014 [Google Scholar] [PubMed] [CrossRef]
24. Moskala M, Goscinski I, Kaluza J, Polak J, Krupa M, Adamek D, et al. Morphological aspects of the traumatic chronic subdural hematoma capsule: SEM studies. Microsc Microanal. 2007;13(3):211–9. doi:10.1017/S1431927607070286 [Google Scholar] [PubMed] [CrossRef]
25. Derk J, Jones HE, Como C, Pawlikowski B, Siegenthaler JA. Living on the edge of the CNS: meninges cell diversity in health and disease. Front Cell Neurosci. 2021;15:703944. doi:10.3389/fncel.2021.703944 [Google Scholar] [PubMed] [CrossRef]
26. Pietilä R, Del Gaudio F, He LQ, Vázquez-Liébanas E, Vanlandewijck M, Muhl L, et al. Molecular anatomy of adult mouse leptomeninges. Neuron. 2023;111(23):3745–64. doi:10.1016/j.neuron.2023.09.002 [Google Scholar] [PubMed] [CrossRef]
27. Weigel R, Schilling L, Krauss JK. The pathophysiology of chronic subdural hematoma revisited: emphasis on aging processes as key factor. Geroscience. 2022;44(3):1353–71. doi:10.1007/s11357-022-00570-y [Google Scholar] [PubMed] [CrossRef]
28. Edlmann E, Giorgi-Coll S, Whitfield PC, Carpenter KLH, Hutchinson PJ. Pathophysiology of chronic subdural haematoma: inflammation, angiogenesis and implications for pharmacotherapy. J Neuroinflamm. 2017;14:108. doi:10.1186/s12974-017-0881-y [Google Scholar] [PubMed] [CrossRef]
29. Santarius T, Kirkpatrick PJ, Kolias AG, Hutchinson PJ. Working toward rational and evidence-based treatment of chronic subdural hematoma. Clin Neurosurg. 2010;57:112–22 [Google Scholar] [PubMed]
30. Bartek JJr, Sjåyik K, Dhawan S, Sagberg LM, Kristiansson H, Ståhl F, et al. Clinical course in chronic subdural hematoma patients aged 18–49 compared to patients 50 years and above: a multicenter study and meta-analysis. Front Neurol. 2019;10:311. doi:10.3389/fneur.2019.00311 [Google Scholar] [PubMed] [CrossRef]
31. Sato S, Suzuki J. Ultrastructural observations of capsule of chronic subdural hematoma in various clinical stages. J Neurosurg. 1975;43(5):569–78. doi:10.3171/jns.1975.43.5.0569 [Google Scholar] [PubMed] [CrossRef]
32. Kawaguchi R, Osuka K, Aoyama M, Miyachi S, Takayasu M. Expressions of eotaxin-3, interleukin-5, and eosinophil-derived neurotoxin in chronic subdural hematoma fluids. J Neurotrauma. 2018;35(19):2242–9. doi:10.1089/neu.2018.5646 [Google Scholar] [PubMed] [CrossRef]
33. Yamashima T. The inner membrane of chronic subdural hematomas-pathology and pathophysiology. Neurosurg Clin North Am. 2000;11(3):413–24. doi:10.1016/S1042-3680(18)30103-7. [Google Scholar] [CrossRef]
34. Sarkar C, Lakhtakia R, Gill SS, Sharma MC, Mahapatra AK, Mehta VS. Chronic subdural haematoma and the enigmatic eosinophil. Acta Neurochir. 2002;144(10):983–8. doi:10.1007/s00701-002-0994-6 [Google Scholar] [PubMed] [CrossRef]
35. Loh JK, Howng SL. Electron microscopic study on the outer membrane of chronic subdural hematoma. Kaohsiung J Med Sci. 1998;14(1):25–30 [Google Scholar] [PubMed]
36. Stanisic M, Aasen AO, Pripp AH, Lindegaard KF, Ramm-Pettersen J, Lyngstadaas SP, et al. Local and systemic pro-inflammatory and anti-inflammatory cytokine patterns in patients with chronic subdural hematoma: a prospective study. Inflamm Res. 2012;61(8):845–52. doi:10.1007/s00011-012-0476-0 [Google Scholar] [PubMed] [CrossRef]
37. Bounajem MT, Campbell RA, Denorme F, Grandhi R. Paradigms in chronic subdural hematoma pathophysiology: current treatments and new directions. J Trauma Acute Care Surg. 2021;91(6):E134–41. doi:10.1097/TA.0000000000003404 [Google Scholar] [PubMed] [CrossRef]
38. Kitazono M, Yokota H, Satoh H, Onda H, Matsumoto G, Fuse A, et al. Measurement of inflammatory cytokines and thrombomodulin in chronic subdural hematoma. Neurol Med-Chir. 2012;52(11):810–5. doi:10.2176/nmc.52.810 [Google Scholar] [PubMed] [CrossRef]
39. Tao ZQ, Lin YY, Hu MT, Ding SH, Li JW, Qiu YM. Mechanism of subdural effusion evolves into chronic subdural hematoma: iL-8 inducing neutrophil oxidative burst. Med Hypotheses. 2016;86:43–6. doi:10.1016/j.mehy.2015.11.027 [Google Scholar] [PubMed] [CrossRef]
40. Wada T, Kuroda K, Yoshida Y, Ogasawara K, Ogawa A, Endo S. Local elevation of the anti-inflammatory interleukin-10 in the pathogenesis of chronic subdural hematoma. Neurosurg Rev. 2006;29(3):242–5. doi:10.1007/s10143-006-0019-7 [Google Scholar] [PubMed] [CrossRef]
41. Wang D, Gao C, Xu X, Chen T, Tian Y, Wei HJ, et al. Treatment of chronic subdural hematoma with atorvastatin combined with low-dose dexamethasone: phase II randomized proof-of-concept clinical trial. J Neurosurg. 2021;134(1):235–43. doi:10.3171/2019.11.JNS192020 [Google Scholar] [PubMed] [CrossRef]
42. Familiari P, Lapolla P, Relucenti M, Battaglione E, Cristiano L, Sorrentino V, et al. Cortical atrophy in chronic subdural hematoma from ultra-structures to physical properties. Sci Rep. 2023;13(1):3400. doi:10.1038/s41598-023-30135-8 [Google Scholar] [PubMed] [CrossRef]
43. Heula AL, Sajanti J, Majamaa K. Procollagen propeptides in chronic subdural hematoma reveal sustained dural collagen synthesis after head injury. J Neurol. 2009;256(1):66–71. doi:10.1007/s00415-009-0048-6 [Google Scholar] [PubMed] [CrossRef]
44. Heula AL, Ohlmeier S, Sajanti J, Majamaa K. Characterization of chronic subdural hematoma fluid proteome. Neurosurg. 2013;73(2):317–31. doi:10.1227/01.neu.0000430323.24623.de [Google Scholar] [PubMed] [CrossRef]
45. Osuka K, Watanabe Y, Usuda N, Aoyama M, Takeuchi M, Takayasu M. Eotaxin-3 activates the smad pathway through the transforming growth factor beta 1 in chronic subdural hematoma outer membranes. J Neurotrauma. 2014;31(16):1451–6. doi:10.1089/neu.2013.3195 [Google Scholar] [PubMed] [CrossRef]
46. Ayazi M, Zivkovic S, Hammel G, Stefanovic B, Ren Y. Fibrotic scar in CNS injuries: from the cellular origins of fibroblasts to the molecular processes of fibrotic scar formation. Cells. 2022;11(15):2371. doi:10.3390/cells11152371 [Google Scholar] [PubMed] [CrossRef]
47. Lin MS. Subdural lesions linking additional intracranial spaces and chronic subdural hematomas: a narrative review with mutual correlation and possible mechanisms behind high recurrence. Diagn. 2023;13(2):235. doi:10.3390/diagnostics13020235 [Google Scholar] [PubMed] [CrossRef]
48. Wang QC, Chi LL. The alterations and roles of glycosaminoglycans in human diseases. Polym. 2022;14(22):5014. doi:10.3390/polym14225014 [Google Scholar] [PubMed] [CrossRef]
49. Dias DO, Göritz C. Fibrotic scarring following lesions to the central nervous system. Matrix Biol. 2018;68–69:561–70. doi:10.1016/j.matbio.2018.02.009 [Google Scholar] [PubMed] [CrossRef]
50. Rehman K, Afzaal A, Akash MSH, Imran M, Assiri MA. Interleukin-1 receptor antagonist: from synthesis to therapeutic applications. Biocell. 2023;47(4):809–23. doi:10.32604/biocell.2023.025850. [Google Scholar] [CrossRef]
51. Takei J, Tanaka T, Yamamoto Y, Hatano K, Ichinose D, Maruyama F, et al. Significantly high concentrations of vascular endothelial growth factor in chronic subdural hematoma with trabecular formation. Clin Neurol Neurosurg. 2021;202:106458. doi:10.1016/j.clineuro.2020.106458 [Google Scholar] [PubMed] [CrossRef]
52. Duffy AM, Bouchier-Hayes DJ, Harmey JH. Vascular endothelial growth factor (VEGF) and its role in non-endothelial cells: autocrine signalling by VEGF. Available from: https://www.ncbi.nlm.nih.gov/books/NBK6482/ [Accessed 2013]. [Google Scholar]
53. Isaji T, Osuka K, Ohmichi Y, Ohmichi M, Naito M, Nakano T, et al. Expression of angiopoietins and angiogenic signaling pathway molecules in chronic subdural hematomas. J Neurotrauma. 2020;37(23):2493–8. doi:10.1089/neu.2020.7042 [Google Scholar] [PubMed] [CrossRef]
54. Funai M, Osuka K, Usuda N, Atsuzawa K, Inukai T, Yasuda M, et al. Activation of PI3 Kinase/Akt signaling in chronic subdural hematoma outer membranes. J Neurotrauma. 2011;28(6):1127–31. doi:10.1089/neu.2010.1498 [Google Scholar] [PubMed] [CrossRef]
55. Osuka K, Watanabe Y, Usuda N, Atsuzawa K, Aoyama M, Niwa A, et al. Activation of Ras/MEK/ERK signaling in chronic subdural hematoma outer membranes. Brain Res. 2012;1489:98–103. doi:10.1016/j.brainres.2012.10.013 [Google Scholar] [PubMed] [CrossRef]
56. Moss A. The angiopoietin: tie 2 interaction: a potential target for future therapies in human vascular disease. Cytokine Growth Factor Rev. 2013;24(6):579–92. doi:10.1016/j.cytogfr.2013.05.009 [Google Scholar] [PubMed] [CrossRef]
57. Khan M, Aziz AA, Shafi NA, Abbas T, Khanani AM. Targeting angiopoietin in retinal vascular diseases: a literature review and summary of clinical trials involving faricimab. Cells. 2020;9(8):1869. doi:10.3390/cells9081869 [Google Scholar] [PubMed] [CrossRef]
58. Hohenstein A, Erber R, Schilling L, Weigel R. Increased mRNA expression of VEGF within the hematoma and imbalance of angiopoietin-1 and -2 mRNA within the neomembranes of chronic subdural hematoma. J Neurotrauma. 2005;22(5):518–28. doi:10.1089/neu.2005.22.518 [Google Scholar] [PubMed] [CrossRef]
59. Sharma S, Rai VK, Narang RK, Markandeywar TS. Collagen-based formulations for wound healing: a literature review. Life Sci. 2022;297:120096. doi:10.1016/j.lfs.2021.120096 [Google Scholar] [PubMed] [CrossRef]
60. Nakagawa T, Kodera T, Kubota T. Expression of matrix metalloproteinases in the chronic subdural haematoma membrane. Acta Neurochir. 2000;142(1):61–6. doi:10.1007/s007010050008 [Google Scholar] [PubMed] [CrossRef]
61. Li FB, Hua C, Feng Y, Yuan HY, Bie L. Correlation of vascular endothelial growth factor with magnetic resonance imaging in chronic subdural hematomas. J Neurol Sci. 2017;377:149–54. doi:10.1016/j.jns.2017.04.013 [Google Scholar] [PubMed] [CrossRef]
62. Kalamatianos T, Stavrinou LC, Koutsarnakis C, Psachoulia C, Sakas DE, Stranjalis G. PlGF and sVEGFR-1 in chronic subdural hematoma: implications for hematoma development. J Neurosurg. 2013;118(2):353–7. doi:10.3171/2012.10.JNS12327 [Google Scholar] [PubMed] [CrossRef]
63. Yang L, Li N, Yang LJ, Wang D, Qiang SK, Zhao ZM. Atorvastatin-induced absorption of chronic subdural hematoma is partially attributed to the polarization of macrophages. J Mol Neurosci. 2022;72(3):565–73. doi:10.1007/s12031-021-01910-x [Google Scholar] [PubMed] [CrossRef]
64. Wang JL, Chen Y, Liang JJ, Cao MS, Shen JB, Ke KF. Study of the pathology and the underlying molecular mechanism of tissue injury around hematoma following intracerebral hemorrhage. Mol Med Rep. 2021;24(4):702. doi:10.3892/mmr.2021.12341 [Google Scholar] [PubMed] [CrossRef]
65. Haciyakupoglu E, Yilmaz DM, Kinali B, Arpaci T, Akbas T, Haciyakupoglu S. Recurrent chronic subdural hematoma: report of 13 cases. Open Med. 2018;13(1):520–7. doi:10.1515/med-2018-0076 [Google Scholar] [PubMed] [CrossRef]
Cite This Article
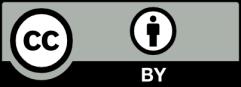