Open Access
ARTICLE
Puerarin mediated miR-30b-5p targeting fibroblast activation protein against oral submucous fibrosis
1 Department of Periodontal Mucosa, Hunan University of Chinese Medicine, Changsha, China
2 Department of Periodontal Mucosa, Changsha Stomatology Hospital, Changsha, China
3 Department of Pharmacy, Changsha Stomatology Hospital, Changsha, China
4 Department of Stomatology, The First Hospital of Hunan University of Chinese Medicine, Changsha, China
* Corresponding Author: HUI XIE. Email:
BIOCELL 2024, 48(4), 591-599. https://doi.org/10.32604/biocell.2024.046691
Received 11 October 2023; Accepted 11 December 2023; Issue published 09 April 2024
Abstract
Background: Puerarin (Pue) has been reported to be a natural active ingredient with multiple antifibrotic properties. This work aimed at exploring the function of Pue in oral submucous fibrosis (OSF) treatment. Methods: Human oral mucosa fibroblasts (hOMF) were induced with transforming growth factor beta1 (TGF-β1) and intervened with Pue. Expressions of fibrosis-related markers were analyzed by Western blot and IF staining. Cell viability was characterized by the CCK-8 assay. Expressions of miR-30 family members were quantified by qRT-PCR. The correlation between fibroblast activation protein (FAP) and miR-30 family expression was evaluated by the Pearson correlation coefficient. Bioinformatics prediction and dual-luciferase reporter assay were employed to verify the regulation between FAP and miR-30b-5p. The specific mechanism of Pue on OSF was explored through the promotion or inhibition of miR-30b-5p. Results: After induction by TGF-β1, hOMF showed upregulated Collagen I, Collagen III, and FAP expressions, while miR-30 family expression was downregulated with miR-30b-5p being the most significant. Pue intervention inhibited the excessive proliferation of TGF-β1-induced hOMF, downregulated FAP, collagen type 3 (COL3A1), collagen type 1 (COL1A1), matrix metalloproteinase 1 (MMP1), and matrix metalloproteinase 3 (MMP3) expressions, and restored miR-30 family expression. Bioinformatics prediction and dual-luciferase reporter assay revealed that miR-30b-5p selectively inhibited FAP expression. Mechanistically, miR-30b-5p mimic suppressed the excessive proliferation of TGF-β1-induced hOMF and declined fibrosis levels. Pue intervention significantly reversed the promotion of TGF-β1-induced OSF by miR-30b-5p inhibition. Conclusion: Pue mediated miR-30b-5p targeting FAP against OSF, which provided a theoretical basis for the pathogenesis research and Pue application in OSF.Graphical Abstract
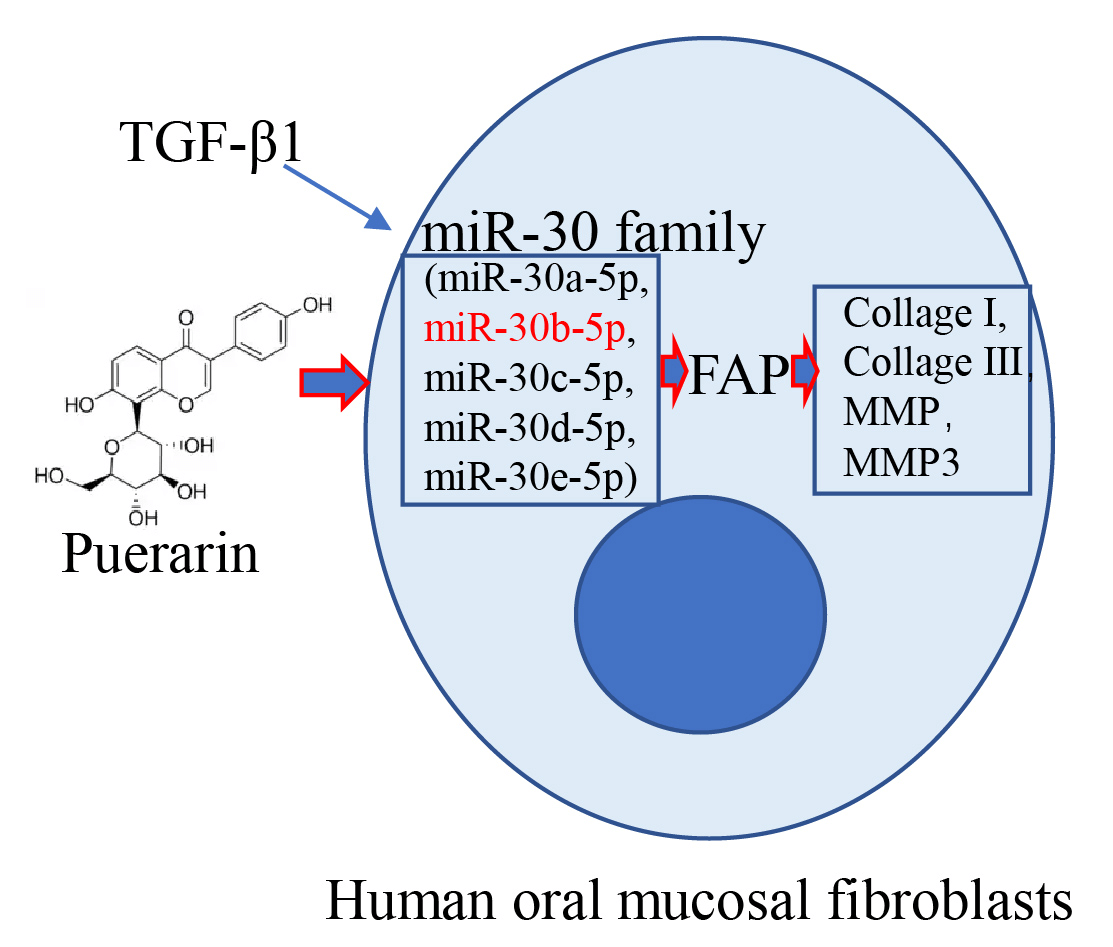
Keywords
Oral submucous fibrosis (OSF) is an insidious disease with various clinical manifestations, such as recurrent oral ulcers, intolerance to spicy food, and decreased mouth opening [1]. Studies have shown that OSF is a collagen deposition disorder that affects oral function and the life quality of patients. Additionally, it carries a risk of developing into oral squamous cell carcinoma (OSCC) ranging from 7% to 30% [2,3]. Extracting active ingredients from Chinese medicine for antifibrosis treatment is currently a research hotspot. It has been found that puerarin (Pue) has a good antifibrotic effect on multiple organs [4–6]. However, the function of Pue on OSF remains to be studied.
microRNAs (miRNAs), a group of non-coding RNAs, can control gene expression. They are highly stable in human body fluids and hold great potential as biomarkers for diagnosing and predicting the prognosis of various diseases [7]. New evidence has suggested that dysregulated miRNAs may be associated with the development of OSF [8]. The downregulation of miR-200b may promote the pathogenesis of Betel Quid-related OSF by regulating expressions of ZEB2 and myofibroblast markers [9]. Additionally, miR-1246 expression is upregulated in OSF tissues, making it a therapeutic target in OSF pathogenesis [10]. The miR-30 family consists of five members and holds great potential as a developmental and disease-regulatory factor [11]. miR-30 may inhibit the development of liver fibrosis through the inhibition of transforming growth factor beta (TGF-β) signal [12]. Further studies have revealed that miR-30a can counteract Wnt1-inducible signaling pathway protein 1 (WISP1) expression induced by transforming growth factor beta1 (TGF-β1), thus influencing the progression of pulmonary fibrosis [13]. However, the specific mechanism by which the miR-30 family participates in OSF remains uncertain.
Fibroblast activation protein (FAP) is one of the most representative secreted products of cancer-associated fibroblasts (CAFs), closely related to tumor growth and invasion [14]. Currently, FAP has attracted great interest as a potential biomarker for cancer detection and a drug target for cancer treatment [15]. Overexpression of FAP in fibroblasts leads to the production of extracellular matrix, which accelerates the development of ovarian cancer and pancreatic cancer [16]. Studies have shown that the downregulation of FAP inhibits cell proliferation and metastasis in OSCC through two signaling pathways [17]. In addition, differential methylation or expression of FAP is significantly associated with survival in OSCC patients [18]. In fibrosis-related disease research, miRNA-30a can downregulate FAP-α expression in lung fibroblasts induced by TGF-β1 [19]. miR-30a-5p directly targets FAP and inhibits cell viability, proliferation, invasion, and migration in OSCC [20]. Therefore, miR-30a-5p may serve as an inhibitor of the occurrence and progression of oral diseases and cancer.
Based on these findings, TGF-β1-induced human oral mucosal fibroblasts (hOMF) were treated with Pue to investigate the role of Pue in regulating FAP expression via miR-30b-5p in OSF, aiming to enrich insights into the application of Pue in OSF treatment.
hOMF (CP-H205, Procell, Wuhan, China) were cultured in a specialized culture medium (CM-H205, Procell, Wuhan, China) under the condition of 5% CO2 at 37°C. In addition, hOMF slides were prepared for immunofluorescence (IF) staining. The miR-30b-5p mimic (HG-MI029666) and its control, and miR-30b-5p inhibitor (HG-IN029666) and its control were all bought from HonorGene (Changsha, China).
In the OSF group, hOMF were treated with TGF-β1 (10 ng/mL) (100-21, Peprotech, Suzhou, China) for 48 h [21]. To determine the suitable concentration of Pue, hOMF were treated with 0.05, 0.1, 0.15, 0.2, and 0.25 mmol/L (mM) of Pue (3681-99-0, Chengdu Yuancheng Biotechnology Co., Ltd., China) for 48 h [22]. In the Pue group, hOMF were treated with TGF-β1, followed by treatment with Pue (0.2 mM) for 48 h. In mimic NC and miR-30b-5p mimic groups, hOMF were treated with TGF-β1, followed by transfection with mimic NC and miR-30b-5p mimic using lipofectamine 2000 (11668019, Invitrogen, Carlsbad, CA, USA), respectively. In Pue+inhibitor NC and Pue+miR-30b-5p inhibitor groups, hOMF were treated with TGF-β1, transfected with inhibitor NC and miR-30b-5p inhibitor respectively, and treated with Pue.
Quantitative real-time polymerase chain reaction (qRT-PCR)
Total RNA was extracted from hOMF using Trizol reagent (15596026, Thermo, USA). Reverse transcription was carried out using an mRNA reverse transcription kit (CW2569, CWBIO, China), followed by qRT-PCR with UltraSYBR Mixture (CW2601, CWBIO, China). All primers used are displayed in Table 1. The mRNA expression was quantified by 2−ΔΔCt, with either β-actin or U6 serving as the internal reference.
Proteins were extracted from hOMF using RIPA lysate (AWB0136, Abiowell, Changsha, China) and transferred onto nitrocellulose (NC) membranes after electrophoretic separation. After being blocked with 5% skimmed milk powder, NC membranes were mixed with primary antibodies overnight at 4°C, including Collagen I (14695-1-AP, Proteintech, Chicago, IL, USA), Collagen III (ab7778, Abcam, Cambridge, UK), FAP (15384-1-AP, Proteintech, Chicago, IL, USA), and β-actin (66009-1-Ig, Proteintech, Chicago, IL, USA). Then, NC membranes were cultured with HRP-labeled secondary antibodies. Next, they were incubated with Super ECL Plus detection reagent (AWB0005, Abiowell, Changsha, China) for imaging in a chemiluminescence imaging system (ChemiScope6100, CLINX, Shanghai, China). The gray values of bands were read by Quantity One 4.6.6 (Bio-Rad Inc., Hercules, CA, USA). Finally, protein expression was calculated with β-actin being the internal reference.
Cell counting kit-8 (CCK-8) assay
hOMF were digested and cultured at 37°C (5 × 103 cells/well). After adhesion, they were treated accordingly for 48 h. Next, 10 μL of CCK-8 reagent (NU679, DOJINDO, Kumamoto, Japan) was added to the medium. Finally, hOMF were cultured for 4 h before detection of optical density (OD) at 450 nm by a multifunctional microplate reader (MB-530, HEALES, Shenzhen, China).
After being fixed with 4% paraformaldehyde, cell slides were mixed with 0.3% Triton X-100 at 37°C. Cell slides were sealed with 5% BSA and cultured with the primary antibody of FAP (Ab40778, Abcam, Cambridge, UK) overnight at 4°C. Next, 100 μL of CoraLite 488-conjugated Affinipure Goat Anti-Rabbit IgG (H+L) (SA00013-2, Proteintech, Chicago, IL, USA) was added and incubated for 1.5 h. Nuclei were stained with DAPI reagent (AWI0331a, Abiowell, Changsha, China) for 20 min. Cell slides were sealed with glycerin before observation under a fluorescence microscope.
Dual-luciferase reporter assay
The possible binding sites between FAP and miR-30b-5p were analyzed by TargrtScan (https://www.targetscan.org/). psiCHECK-2-Hsa-FAP-WT and psiCHECK-2-Hsa-FAP-MUT were purchased from HonorGene (Changsha, China). HEK-293T cells were bought from Abiowell (AW-CNH086, Changsha, China). In our study, HEK-293T cells were co-transfected with psiCHECK-2-Hsa-FAP-WT or psiCHECK-2-Hsa-FAP-MUT along with mimic NC or miR-30b-5p mimic by using lipofectamine 2000. After being cultured for 48 h, cells were lysed and treated by using a dual-luciferase detection kit (E1910, Promega, Beijing, China). Subsequently, the activity of both firefly luciferase and Renilla luciferase was measured consecutively using a chemiluminescence detector (GloMax 20/20, Promega, Beijing, China).
Experimental data were processed by GraphPad Prism 8.0 (GraphPad Software Inc., San Diego, CA, USA) and represented as mean±SD. t-test and one-way analysis of variance (ANOVA) were employed for comparison. The correlation between FAP and miR-30 family expression was evaluated by the Pearson correlation coefficient. Statistical significance was determined at p < 0.05.
The miR-30 family participated in the disease process of OSF
To establish an in vitro model of OSF, hOMF were induced with TGF-β1. After induction, Collagen I and Collagen III expressions in hOMF were significantly upregulated (Fig. 1A). Furthermore, miR-30 family expression was downregulated in hOMF after TGF-β1 induction, with miR-30b-5p showing the most significant decrease. Conversely, FAP expression was upregulated after TGF-β1 induction (Figs. 1B and 1C). Finally, the analysis of the Pearson correlation coefficient displayed a negative correlation between FAP and miR-30 family expression (Fig. 1D). These results evidenced that the miR-30 family was involved in the disease process of OSF.
Figure 1: The miR-30 family participated in the disease process of OSF. (A) Collagen I and Collagen III expressions in hOMF were analyzed by Western blot. (B) miR-30 family and FAP mRNA expressions in hOMF were evaluated by qRT-PCR. (C) FAP expression in hOMF was evaluated by Western blot. (D) Pearson correlation coefficient analysis of relationship between expression of FAP mRNA and expression of miR-30 family. The experiment was repeated three times. The data were presented as the mean ± SD. *p < 0.05 vs. Control. n = 3.
Pue affected the expression of miR-30/FAP axis in OSF
To screen for suitable experimental concentrations, hOMF was treated with 0, 0.05, 0.1, 0.15, 0.2, and 0.25 mM of Pue for 48 h, and the cell viability was analyzed by CCK-8 assay. As a result, Pue showed a proliferation-promoting effect on hOMF when the concentration of Pue was 0.05–0.2 mM. However, when the concentration of Pue was 0.25 mM, the pro-proliferative extent of hOMF was significantly reduced. Therefore, 0.2 mM Pue was chosen for subsequent experiments (Fig. 2A). After TGF-β1 induction, hOMF exhibited excessive proliferation. However, this excessive proliferation was inhibited with Pue intervention (Fig. 2B). qRT-PCR analysis revealed that TGF-β1 induction reduced miR-30 family expressions. However, Pue intervention restored miR-30 family expression, with miR-30b-5p exhibiting the greatest recovery. Combined with the results in Fig. 1B, miR-30b-5p was selected for subsequent experiments. In addition, Pue intervention also reversed the TGF-β1-induced upregulation of FAP mRNA expression (Fig. 2C). Furthermore, analysis of fibrosis-related genes revealed that TGF-β1 induction promoted the high expressions of COL3A1, COL1A1, MMP1, and MMP3. However, Pue intervention inhibited TGF-β1-induced fibrosis promotion (Fig. 2D). Consistent with the results in Fig. 2C, IF staining showed that Pue intervention also reversed the TGF-β1-induced promotion of FAP expression (Fig. 2E). In general, Pue affected the miR-30/FAP axis in OSF.
Figure 2: Pue affected the miR-30/FAP axis in OSF. (A) The toxic effects of Pue on hOMF were evaluated using the CCK-8 assay. &p < 0.05 vs. 0 mM. (B) The proliferation of hOMF was assessed by CCK-8 assay. (C) miR-30 family and FAP mRNA expressions in hOMF were determined by qRT-PCR. (D) mRNA expressions of COL3A1, COL1A1, MMP1, and MMP3 in hOMF were analyzed by qRT-PCR. (E) FAP protein expression in hOMF was identified by IF staining. Scale bar: 25 μm. Magnification: 400 ×. The experiment was repeated three times. The data were presented as the mean ± SD. *p < 0.05 vs. Control. #p < 0.05 vs. OSF. n = 3.
miR-30b-5p regulated FAP expression
miR-30b-5p was proved to have binding sites on the 3′-UTR of FAP according to TargetScan (Fig. 3A). To confirm its targeting effect on FAP, HEK-293T cells were transfected with FAP-WT or FAP-MUT, along with either mimic NC or miR-30b-5p mimic. When co-transfected with FAP-WT, the relative luciferase activity was reduced in the miR-30b-5p mimic group. Nevertheless, it was not changed in the miR-30b-5p mimic group when co-transfected with FAP-MUT (Fig. 3B). The above proved that miR-30b-5p could regulate FAP expression.
Figure 3: miR-30b-5p regulated FAP expression. (A) TargetScan prediction of binding sites between miR-30b-5p and FAP. (B) The regulation of FAP by miR-30b-5p was confirmed through a dual-luciferase reporter assay. The experiment was repeated three times. The data were presented as the mean ± SD. *p < 0.05 vs. FAP-WT+mimic NC. n = 3.
miR-30b-5p targetedly regulated FAP to affect TGF-β1-induced OSF
To explain the function of miR-30b-5p in OSF, hOMF were induced by TGF-β1 and transfected with miR-30b-5p mimic or its control. miR-30b-5p mimic transfection effectively inhibited the excessive proliferation of hOMF induced by TGF-β1 (Fig. 4A). Moreover, miR-30b-5p overexpression led to the downregulation of COL3A1, COL1A1, MMP1, and MMP3 mRNA expression, as well as the decrease in FAP protein expression (Figs. 4B–4D). The above identified that miR-30b-5p targetedly regulated FAP to affect TGF-β1-induced OSF.
Figure 4: miR-30b-5p targetedly regulated FAP to affect TGF-β1-induced OSF. (A) The proliferation of hOMF was measured by CCK-8 assay. (B) miR-30b-5p expression in hOMF was analyzed by qRT-PCR. (C) mRNA expressions of COL3A1, COL1A1, MMP1, and MMP3 in hOMF were determined by qRT-PCR. (D) FAP protein expression in hOMF was analyzed by Western blot. The experiment was repeated three times. The data were presented as the mean ± SD. *p < 0.05 vs. mimic NC. n = 3.
Pue inhibited TGF-β1-induced OSF through the miR-30b-5p/FAP axis
To investigate the regulatory mechanism of Pue on TGF-β1-induced OSF, hOMF were induced with TGF-β1, transfected with miR-30b-5p inhibitor or its control, and then treated with Pue. Compared with the Pue+inhibitor NC group, hOMF proliferation was enhanced in the Pue+miR-30b-5p inhibitor group (Fig. 5A). Additionally, miR-30b-5p expression was downregulated in hOMF of the Pue+miR-30b-5p inhibitor group, confirming the successful silencing of miR-30b-5p (Fig. 5B). In hOMF of the Pue+miR-30b-5p inhibitor group, COL3A1, COL1A1, MMP1, and MMP3 mRNA expressions were upregulated compared with the Pue+inhibitor NC group (Fig. 5C). Moreover, FAP, Collagen I, and Collagen III expressions were also upregulated in hOMF of the Pue+miR-30b-5p inhibitor group in contrast to the Pue+inhibitor NC group (Figs. 5D–5E). The above illustrated that Pue inhibited TGF-β1-induced OSF through the miR-30b-5p/FAP axis.
Figure 5: Pue inhibited TGF-β1-induced OSF through the miR-30b-5p/FAP axis. (A) The proliferation of hOMF was detected by CCK-8 assay. (B) miR-30b-5p expression in hOMF was assessed by qRT-PCR. (C) mRNA expressions of COL3A1, COL1A1, MMP1, and MMP3 in hOMF were determined by qRT-PCR. (D) Protein expression of FAP in hOMF was measured by IF staining. Scale bar: 25 μm. Magnification: 400×. (E) Protein expressions of Collage I and Collage III in hOMF were assessed by Western blot. The experiment was repeated three times. The data were presented as the mean ± SD. *p < 0.05 vs. Pue+inhibitor NC. n = 3.
OSF is a potential oral malignancy involving multiple factors such as chronic trauma, infection, and inflammation, and is characterized by progressive fibrosis of the submucosal tissue [23]. Recently, natural active ingredients have shown potential in OSF treatment [24–26]. This study demonstrated that Pue was a promising alternative drug for OSF treatment, the benefits of which were achieved through miR-30b-5p targeted inhibition of FAP expression.
TGF-β regulates important physiological processes in normal cells and tissues, such as tumor suppression. However, the occurrence of tumors counteracts the antitumor effect of TGF-β [27]. Furthermore, in the later stages of the tumor, TGF-β can promote metastasis by enhancing epithelial-mesenchymal transition and angiogenesis [28]. A study has found that TGF-β1 is a key regulatory factor in OSF pathogenesis. TGF-β1 induces fibrotic gene expression and collagen production in buccal mucosal fibroblasts (BMF) [29]. In fibrosis-related diseases, compared to healthy tissues, the expression of MMP2, actin alpha cardiac muscle 3 (ACTA3), COL1A2, and MMP1 is significantly higher in fibrotic tissues [30]. Among various etiological factors, betel nut is the major causative factor in OSF development. Betel nut alkaloids can increase TGF-β secretion, and TGF-β can activate the differentiation of myofibroblasts in the oral mucosa [31]. In this study, TGF-β1 induced upregulation of COL3A1, COL1A1, MMP1, MMP3, and FAP expressions in hOMF. These suggested that TGF-β1 induced the activation and differentiation of hOMF, leading to an imbalance between collagen degradation and synthesis, thus promoting the development of OSF.
The miR-30 family plays an essential role in cancer, bone metabolism, pulmonary fibrosis, and so on. They influence important biological processes like proliferation, differentiation, and metastasis by controlling multiple target genes [32–34]. Upregulation of miR-30 expression can suppress the motility and metastasis of cancer, thereby improving the survival and outcomes of patients [35]. Additionally, miR-30 suppresses the progression of breast cancer via the regulation of FK506-binding protein 3 (FKBP3) expression [36]. Members of the miR-30 family can inhibit breast cancer invasion by regulating genes related to bone metastasis [33]. Moreover, the miR-30 family inhibits excessive lung vascular permeability in the pre-metastatic stage by directly targeting Skp2 [37]. In this study, bioinformatics prediction revealed binding sites between miR-30b-5p and the 3′-UTR of FAP. Furthermore, dual-luciferase reporter assay results displayed miR-30b-5p inhibition on the downstream target FAP expression. Pearson correlation coefficient further validated the significant negative regulation of FAP expression by miR-30 family members. FAP-α is selectively expressed in sites of tissue remodeling and repair, and it promotes tumorigenesis. FAP-α was regarded as a therapeutic target for diseases associated with fibrosis dysregulation [38]. Studies have shown that miR-30a attenuates TGF-β1-induced activation of lung fibroblasts by targeting FAP-α [39]. In this study, successful transfection of miR-30b-5p mimic resulted in downregulation of TGF-β1-induced FAP accumulation in hOMF, identifying the negative regulation of miR-30b-5p on FAP expression in OSF.
Pue, a bioactive component of Radix puerariae, has various pharmacological effects, including vasodilation, neuroprotection, cardioprotection, bone protection, anticancer, and so on [40]. Research has revealed that Pue can reduce proliferation and induce apoptosis of bladder cancer cells by upregulating miR-16 and consequently inhibit the nuclear factor-kappa B (NF-κB) pathway [41]. Additionally, Pue upregulates the expression of miR-155-3p, promoting the differentiation and bone formation of marrow mesenchymal stem cells in rats undergoing bone transplantation [42]. Pue and its derivatives have also been studied for their inhibitory effects on organ fibrosis. It has been found that the extract of Radix puerariae can improve paraquat-induced pulmonary fibrosis by downregulating the expression of miR-21 [43]. Furthermore, Pue has been shown to prevent myocardial fibrosis in mice by reducing NF-κB and TGF-β1 expressions in the heart [44]. In type 2 diabetic rats, Pue alleviates liver fibrosis by the TGF-β signaling pathway inhibition [45]. However, there have been few reports on the role and mechanism of Pue in OSF. In this study, 0.05–0.2 mM Pue had no cytotoxicity on hOMF, which was consistent with literature reports [22,46]. 0.2 mM Pue intervention effectively suppressed the excessive proliferation of TGF-β1-induced hOMF, alleviated collagen deposition, upregulated miR-30 family expression, and downregulated FAP expression, thereby delaying the progression of fibrosis. In subsequent mechanistic studies, Pue intervention significantly reversed the promotion of TGF-β1-induced OSF by the miR-30b-5p inhibitor. These findings suggested that Pue had the potential to treat OSF by modulating the miR-30b-5p/FAP axis, making it a promising treatment for OSF.
This study still has some limitations. Firstly, we have investigated the in vitro effect of Pue on OSF, and further in vivo studies are needed to clarify its therapeutic potential. Secondly, the exact molecular mechanisms underlying the interaction between Pue, miR-30b-5p, and FAP in OSF require further exploration. For example, the mechanism by which Pue promotes miR-30b-5p expression and whether Pue regulates the miR-30b-5p/FAP axis by interfering with other signaling pathways need to be explored through functional analysis. Thirdly, the potential side effects and toxicological profiles of Pue should be further investigated in pre-clinical studies before clinical application. Fourthly, MMP1 and MMP3 have been widely studied in fibrotic diseases [47,48]. However, other members in MMP family may participate in OSF. Due to the limit of source and time, we have only assessed the two molecules here. The function of other members in MMP family on OSF will inspire us to do the work ahead. Finally, Pue has a short half-life, fast elimination in vivo, and short binding time with the target. Therefore, the structural modification of Pue to improve its efficacy and bioavailability is a research direction for the later development of new drugs against OSF.
In summary, our study demonstrated that Pue had a significant inhibition on OSF through the downregulation of FAP and its downstream targets, including COL3A1, COL1A1, MMP1, and MMP3. This effect was mainly achieved through the upregulation of miR-30b-5p and inhibition of FAP signaling. These findings will enrich the understanding of the application of Pue in OSF and other fibrotic diseases.
Acknowledgement: None.
Funding Statement: This work was supported by the National Natural Science Foundation of China (Nos. 81874496, 82374530), the Clinical Medical Technology Innovation Guide Project of Hunan Province (No. 2020SK53206), the Natural Science Foundation of Hunan Province (No. 2021JJ70062), the Changsha Natural Science Foundation Project (No. kq2014019), the Health Special Fund Research Project of Hunan Province (No. B2020-07), and the Clinical Pharmaceutical Research Fund of Hunan Medical Association (No. B202012).
Author Contributions: All authors contributed to the study conception and design. Conceptualization, data curation, validation, writing of the original draft and review: SX; Formal analysis, investigation and software: JG, YY, MZ, SW and JT. Funding acquisition, project administration, supervision, and review: HX. All authors reviewed the results and approved the final version of the manuscript.
Availability of Data and Materials: The datasets used and analyzed during the current study are available from the corresponding author on reasonable request.
Ethics Approval: Not applicable.
Conflicts of Interest: The authors declare that they have no conflicts of interest to report regarding the present study.
References
1. Chaudhry K, Khatana S, Bali R, Kaur A, Dutt N. Quality of life in oral submucous fibrosis: a systematic review. J Maxillofac Oral Surg. 2022;21(1):14–24. doi:10.1007/s12663-020-01507-8. [Google Scholar] [PubMed] [CrossRef]
2. Shen YW, Shih YH, Fuh LJ, Shieh TM. Oral submucous fibrosis: a review on biomarkers, pathogenic mechanisms, and treatments. Int J Mol Sci. 2020;21(19):7231. doi:10.3390/ijms21197231. [Google Scholar] [PubMed] [CrossRef]
3. Peng Q, Li H, Chen J, Wang Y, Tang Z. Oral submucous fibrosis in Asian countries. J Oral Pathol Med. 2020;49(4):294–304. doi:10.1111/jop.12924. [Google Scholar] [PubMed] [CrossRef]
4. Wang J, Ge S, Wang Y, Liu Y, Qiu L, Li J, et al. Puerarin alleviates UUO-induced inflammation and fibrosis by regulating the NF-κB P65/STAT3 and TGFβ1/smads signaling pathways. Drug Design, Dev Therapy. 2021;15:3697–708. doi:10.2147/dddt.S321879. [Google Scholar] [PubMed] [CrossRef]
5. Ni SY, Zhong XL, Li ZH, Huang DJ, Xu WT, Zhou Y, et al. Puerarin alleviates lipopolysaccharide-induced myocardial fibrosis by inhibiting PARP-1 to prevent HMGB1-mediated TLR4-NF-κB signaling pathway. Cardiovasc Toxicol. 2020;20(5):482–91. doi:10.1007/s12012-020-09571-9. [Google Scholar] [PubMed] [CrossRef]
6. Xue Z, Zhao F, Sang X, Qiao Y, Shao R, Wang Y, et al. Combination therapy of tanshinone IIA and puerarin for pulmonary fibrosis via targeting IL6-JAK2-STAT3/STAT1 signaling pathways. Phytother Res PTR. 2021;35(10):5883–98. doi:10.1002/ptr.7253. [Google Scholar] [PubMed] [CrossRef]
7. Ho PTB, Clark IM, Le LTT. MicroRNA-based diagnosis and therapy. Int J Mol Sci. 2022;23(13):7167. doi:10.3390/ijms23137167. [Google Scholar] [PubMed] [CrossRef]
8. Jishnu PV, Shenoy SU, Sharma M, Chopra A, Radhakrishnan R. Comprehensive analysis of microRNAs and their target genes in oral submucous fibrosis. Oral Dis. 2023;29(5):1894–904. doi:10.1111/odi.14219. [Google Scholar] [PubMed] [CrossRef]
9. Liao YW, Yu CC, Hsieh PL, Chang YC. miR-200b ameliorates myofibroblast transdifferentiation in precancerous oral submucous fibrosis through targeting ZEB2. J Cell Mol Med. 2018;22(9):4130–8. doi:10.1111/jcmm.13690. [Google Scholar] [PubMed] [CrossRef]
10. Liu CM, Liao YW, Hsieh PL, Yu CH, Chueh PJ, Lin T, et al. miR-1246 as a therapeutic target in oral submucosa fibrosis pathogenesis. J Formos Med Assoc, 118(7):1093–8. doi:10.1016/j.jfma.2019.02.014. [Google Scholar] [PubMed] [CrossRef]
11. Huang J, Li Y, Zhu S, Wang L, Yang L, He C. MiR-30 family: a novel avenue for treating bone and joint diseases? Int J Med Sci. 2023;20(4):493–504. doi:10.7150/ijms.81990. [Google Scholar] [PubMed] [CrossRef]
12. Tu X, Zheng X, Li H, Cao Z, Chang H, Luan S, et al. MicroRNA-30 protects against carbon tetrachloride-induced liver fibrosis by attenuating transforming growth factor beta signaling in hepatic stellate cells. Toxicol Sci. 2015;146(1):157–69. doi:10.1093/toxsci/kfv081. [Google Scholar] [PubMed] [CrossRef]
13. Berschneider B, Ellwanger DC, Baarsma HA, Thiel C, Shimbori C, White ES, et al. miR-92a regulates TGF-β1-induced WISP1 expression in pulmonary fibrosis. Int J Biochem Cell Biol. 2014;53:432–41. doi:10.1016/j.biocel.2014.06.011. [Google Scholar] [PubMed] [CrossRef]
14. Dendl K, Koerber SA, Kratochwil C, Cardinale J, Finck R, Dabir M, et al. FAP and FAPI-PET/CT in malignant and non-malignant diseases: a perfect symbiosis? Cancers. 2021;13(19):4946. doi:10.3390/cancers13194946. [Google Scholar] [PubMed] [CrossRef]
15. Kim J, Seki E. FAP: not just a biomarker but druggable target in liver fibrosis. Cell Mol Gastroenterol Hepatol. 2023;15(4):1018–9. doi:10.1016/j.jcmgh.2022.12.018. [Google Scholar] [PubMed] [CrossRef]
16. Fitzgerald AA, Weiner LM. The role of fibroblast activation protein in health and malignancy. Cancer Metastasis Rev. 2020;39(3):783–803. doi:10.1007/s10555-020-09909-3. [Google Scholar] [PubMed] [CrossRef]
17. Wang H, Wu Q, Liu Z, Luo X, Fan Y, Liu Y, et al. Downregulation of FAP suppresses cell proliferation and metastasis through PTEN/PI3K/AKT and Ras-ERK signaling in oral squamous cell carcinoma. Cell Death Dis. 2014;5(4):e1155. doi:10.1038/cddis.2014.122. [Google Scholar] [PubMed] [CrossRef]
18. Zhao C, Zou H, Zhang J, Wang J, Liu H. An integrated methylation and gene expression microarray analysis reveals significant prognostic biomarkers in oral squamous cell carcinoma. Oncol Rep. 2018;40(5):2637–47. doi:10.3892/or.2018.6702. [Google Scholar] [PubMed] [CrossRef]
19. Han Y, Chen L, Liu H, Jin Z, Wu Y, Wu Y, et al. Airway epithelial cGAS is critical for induction of experimental allergic airway inflammation. J Immunol. 2020;204(6):1437–47. doi:10.4049/jimmunol.1900869. [Google Scholar] [PubMed] [CrossRef]
20. Ruan P, Tao Z, Tan A. Low expression of miR-30a-5p induced the proliferation and invasion of oral cancer via promoting the expression of FAP. Biosci Rep. 2018;38(1):BSR20171027. doi:10.1042/BSR20171027. [Google Scholar] [PubMed] [CrossRef]
21. Liu J, Li F, Liu B, Yao Z, Li L, Liu G, et al. Adipose-derived mesenchymal stem cell exosomes inhibit transforming growth factor-β1-induced collagen synthesis in oral mucosal fibroblasts. Exp Ther Med. 2021;22(6):1419. doi:10.3892/etm.2021.10854. [Google Scholar] [PubMed] [CrossRef]
22. Li Y, Hong L, Liu C, Min J, Hong S, Hu M, et al. Effect of puerarin on collagen metabolism of fibroblasts in pelvic tissue of women with pelvic organ prolapse. Mol Med Rep. 2018;17(2):2705–11. doi:10.3892/mmr.2017.8112. [Google Scholar] [PubMed] [CrossRef]
23. Chen X, Xie H, Guo J. Drug treatment for oral submucous fibrosis: an update. BMC oral health. 2023;23(1):748. doi:10.1186/s12903-023-03488-9. [Google Scholar] [PubMed] [CrossRef]
24. James A, Gunasekaran N, Krishnan R, Arunachalam P, Mahalingam R. Anti-fibrotic activity of licorice extract in comparison with colchicine on areca nut-induced fibroblasts: an in vitro study. J Oral Maxillofacial Pathol. 2022;26(2):173–8. doi:10.4103/jomfp.jomfp_110_21. [Google Scholar] [PubMed] [CrossRef]
25. Zhang L, Tan J, Liu Y, Luo M. Curcumin relieves arecoline-induced oral submucous fibrosis via inhibiting the LTBP2/NF-κB axis. Oral Dis. 2023;8:7500. doi:10.1111/odi.14656. [Google Scholar] [PubMed] [CrossRef]
26. Cai X, Zhang H, Li T. Multi-target pharmacological mechanisms of Salvia miltiorrhiza against oral submucous fibrosis: a network pharmacology approach. Arch Oral Biol. 2021;126(4):105131. doi:10.1016/j.archoralbio.2021.105131. [Google Scholar] [PubMed] [CrossRef]
27. Tian M, Neil JR, Schiemann WP. Transforming growth factor-β and the hallmarks of cancer. Cell Signal. 2011;23(6):951–62. doi:10.1016/j.cellsig.2010.10.015. [Google Scholar] [PubMed] [CrossRef]
28. Fernandes S, Cruz JO, Morazzo S, Niro F, Cassani M, Ďuríková H, et al. TGF-β induces matrisome pathological alterations and EMT in patient-derived prostate cancer tumoroids. Matrix Biol J Int Soc Matrix Biol. 2023;125(2):12–30. doi:10.1016/j.matbio.2023.11.001. [Google Scholar] [PubMed] [CrossRef]
29. Hsieh YP, Chen HM, Lin HY, Yang H, Chang JZ. Epigallocatechin-3-gallate inhibits transforming-growth-factor-β1-induced collagen synthesis by suppressing early growth response-1 in human buccal mucosal fibroblasts. J Formosan Med Assoc Taiwan Yi Zhi. 2017;116(2):107–13. doi:10.1016/j.jfma.2016.01.014. [Google Scholar] [PubMed] [CrossRef]
30. Mirzavand S, Rafiei A, Teimoori A, Khorsandi L, Bahreini A, Motamedfar A, et al. Gene expression in human liver fibrosis associated with Echinococcus granulosus sensu lato. Parasitol Res. 2020;119(7):2177–87. doi:10.1007/s00436-020-06700-9. [Google Scholar] [PubMed] [CrossRef]
31. Yu CC, Liao YW, Hsieh PL, Chang YC. Targeting lncRNA H19/miR-29b/COL1A1 axis impedes myofibroblast activities of precancerous oral submucous fibrosis. Int J Mol Sci. 2021;22(4):2216. doi:10.3390/ijms22042216. [Google Scholar] [PubMed] [CrossRef]
32. Zhao Q, Yuan X, Zheng L, Xue M. miR-30d-5p: a non-coding RNA with potential diagnostic, prognostic and therapeutic applications. Front Cell Dev Biol. 2022;10:829435. doi:10.3389/fcell.2022.829435. [Google Scholar] [PubMed] [CrossRef]
33. Croset M, Pantano F, Kan CWS, Bonnelye E, Descotes F, Alix-Panabières C, et al. miRNA-30 family members inhibit breast cancer invasion, osteomimicry, and bone destruction by directly targeting multiple bone metastasis-associated genes. Cancer Res. 2018;78(18):5259–73. doi:10.1158/0008-5472.CAN-17-3058. [Google Scholar] [PubMed] [CrossRef]
34. Perera UE, Derseh HB, Dewage SNV, Stent A, Wijayarathna R, Snibson KJ. Evaluation of microRNA expression in a sheep model for lung fibrosis. BMC Genom. 2021;22(1):827. doi:10.1186/s12864-021-08073-4. [Google Scholar] [PubMed] [CrossRef]
35. Jamshidi M, Fagerholm R, Muranen TA, Kaur S, Potdar S, Khan S, et al. High miR-30 expression associates with improved breast cancer patient survival and treatment outcome. Cancers. 2021;13(12):2907. doi:10.3390/cancers13122907. [Google Scholar] [PubMed] [CrossRef]
36. Liu P, Xie X, Yang A, Kong Y, Allen-Gipson D, Tian Z, et al. Melatonin regulates breast cancer progression by the lnc010561/miR-30/FKBP3 axis. Mol Ther Nucleic Acids. 2020;19:765–74. doi:10.1016/j.omtn.2019.12.019. [Google Scholar] [PubMed] [CrossRef]
37. Qi F, He T, Jia L, Song N, Guo L, Ma X, et al. The miR-30 family inhibits pulmonary vascular hyperpermeability in the premetastatic phase by direct targeting of Skp2. Clin Cancer Res. 2015;21(13):3071–80. doi:10.1158/1078-0432.Ccr-14-2785. [Google Scholar] [PubMed] [CrossRef]
38. Schmidkonz C, Kuwert T, Atzinger A, Cordes M, Schett G, Ramming A, et al. Fibroblast activation protein inhibitor imaging in nonmalignant diseases: a new perspective for molecular imaging. J Nuclear Med Official Publ Soc Nuclear Med. 2022;63(12):1786–92. doi:10.2967/jnumed.122.264205. [Google Scholar] [PubMed] [CrossRef]
39. Wu G, Xie B, Lu C, Chen C, Zhou J, Deng Z. microRNA-30a attenuates TGF-β1-induced activation of pulmonary fibroblast cell by targeting FAP-α. J Cell Mol Med. 2020;24(6):3745–50. doi:10.1111/jcmm.15020. [Google Scholar] [PubMed] [CrossRef]
40. Wang D, Bu T, Li Y, He Y, Yang F, Zou L. Pharmacological activity, pharmacokinetics, and clinical research progress of puerarin. Antioxidants. 2022;11(11):2121. doi:10.3390/antiox11112121. [Google Scholar] [PubMed] [CrossRef]
41. Liu X, Li S, Li Y, Cheng B, Tan B, Wang G. Puerarin inhibits proliferation and induces apoptosis by upregulation of miR-16 in bladder cancer cell line T24. Oncol Res. 2018;26(8):1227–34. doi:10.3727/096504018X15178736525106. [Google Scholar] [PubMed] [CrossRef]
42. Zhou Y, Lian H, Liu K, Wang D, Xiu X, Sun Z. Puerarin improves graft bone defect through microRNA‐155‐3p‐mediated p53/TNF‐α/STAT1 signaling pathway. Int J Mol Med. 2020;46(1):239–51. doi:10.3892/ijmm.2020.4595. [Google Scholar] [PubMed] [CrossRef]
43. Liu MW, Liu R, Wu HY, Li YY, Su MX, Dong MN, et al. Radix puerariae extracts ameliorate paraquat-induced pulmonary fibrosis by attenuating follistatin-like 1 and nuclear factor erythroid 2p45-related factor-2 signalling pathways through downregulation of miRNA-21 expression. BMC Complement Altern Med. 2016;16(1):11. doi:10.1186/s12906-016-0991-6. [Google Scholar] [PubMed] [CrossRef]
44. Chen R, Xue J, Xie M. Puerarin prevents isoprenaline-induced myocardial fibrosis in mice by reduction of myocardial TGF-β1 expression. J Nutr Biochem. 2012;23(9):1080–5. doi:10.1016/j.jnutbio.2011.05.015. [Google Scholar] [PubMed] [CrossRef]
45. Hou B, Zhao Y, Qiang G, Yang X, Xu C, Chen X, et al. Puerarin mitigates diabetic hepatic steatosis and fibrosis by inhibiting tgf-β signaling pathway activation in type 2 diabetic rats. Oxid Med Cell Longev. 2018;2018:4545321. doi:10.1155/2018/4545321. [Google Scholar] [PubMed] [CrossRef]
46. Mo Q, Li S, You S, Wang D, Zhang J, Li M, et al. Puerarin reduces oxidative damage and photoaging caused by UVA radiation in human fibroblasts by regulating Nrf2 and MAPK signaling pathways. Nutrients. 2022;14(22):4724. doi:10.3390/nu14224724. [Google Scholar] [PubMed] [CrossRef]
47. Chuang HM, Chen YS, Harn HJ. The versatile role of matrix metalloproteinase for the diverse results of fibrosis treatment. Mol. 2019;24(22):4188. doi:10.3390/molecules24224188. [Google Scholar] [PubMed] [CrossRef]
48. Giannandrea M, Parks WC. Diverse functions of matrix metalloproteinases during fibrosis. Dis Model Mech. 2014;7(2):193–203. doi:10.1242/dmm.012062. [Google Scholar] [PubMed] [CrossRef]
Cite This Article
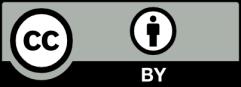