Open Access
REVIEW
Exploring the molecular mechanisms and potential therapeutic strategies of ferroptosis in ovarian cancer
1 Department of Gynecology, Jiaxing University Master Degree Cultivation Base, Zhejiang Chinese Medical University, Jiaxing, China
2 Department of Gynecology, Jiaxing University Affiliated Women and Children’s Hospital, Jiaxing, China
* Corresponding Author: WEILI ZHU. Email:
# Equal contribution
(This article belongs to the Special Issue: Cell Death in Health and Disease: Diversity, Complexity, and Dynamics)
BIOCELL 2024, 48(3), 379-386. https://doi.org/10.32604/biocell.2024.047812
Received 18 November 2023; Accepted 22 January 2024; Issue published 15 March 2024
Abstract
The morbidity rate of ovarian cancer, a malignant tumour in gynaecological tumours, is rising, and it is considered to be the most lethal cancer. The majority of patients are typically diagnosed during the advanced stages of the illness due to the elusive characteristics of ovarian cancer and an absence of highly sensitive and specific diagnostic indicators. Surgical excision of the lesions, along with chemotherapy, is the conventional treatment for ovarian cancer; however, resistance to platinum-based chemotherapeutic drugs and molecular targeted therapies frequently arises. Improving the survival rate and prognosis of patients with end-stage or recurring ovarian cancer requires the identification of new therapeutic targets due to the absence of efficient medications, and this has emerged as a highly demanding issue. Studies have demonstrated that ferroptosis effectively hinders the proliferation of ovarian cancer and induces the demise of malignant cells. Ferroptosis is composed of the cystine/glutamate antiporter system (the system Xc-) and glutathione peroxidase 4 (GPX4). Solute carrier family 7 member 11 (SLC7A11) and solute carrier family 3 member 2 (SLC3A2) play crucial roles in the regulation of ferroptosis by facilitating the uptake of cystine into cells and the efflux of glutamate out of cells, respectively. In cells, GPX4 is the exclusive enzyme employed for reducing liposomal peroxide through glutathione peroxidase activity. The occurrence of ferroptosis in ovarian cancer is strongly associated with three main pathways, namely, the GPX4-glutathione (GSH) protective pathway, the ferroptosis suppressor protein 1 (FSP1)- coenzyme Q10 (CoQ10) protective pathway, and the guanosine 5'-triphosphate cyclohydrolase I (GCH1) protective pathway. In ovarian cancer cells, the postsynaptic density-95, discs-large, zona occludens 1 (PDZ)-binding motif-angiopoietin-like 4-nicotinamide adenine dinucleotide phosphate oxidases 2 (TAZ-ANGPTL4-NOX2) pathway can be regulated by Yes-associated protein (YAP)/TAZ, a downstream component of the Hippo pathway, leading to the modulation of ferroptosis. By targeting microRNA-587, lncRNA ADAMTS9 antisense RNA 1 (ADAMTS9-AS1) can modulate the expression of SLC7A11 and reduce the occurrence of ferroptosis. Although ferroptosis holds promise in overcoming the resistance mechanism, there remain obstacles in utilizing it as a cancer treatment, including the potential harm of drugs to healthy cells. Hence, additional investigations are required to formulate safer and more efficient chemotherapy protocols for the treatment of ovarian cancer and other malignancies.Keywords
Among gynaecological tumours, ovarian cancer has the highest fatality rate in terms of mortality [1]. Each year, approximately 240,000 women worldwide receive a diagnosis of ovarian cancer, and the incidence has been on the rise in recent times [2]. Ovarian cancer typically develops subtly. The reason is a lack of comprehensive comprehension regarding the occurrence and progression of ovarian cancer. Moreover, due to the absence of highly sensitive and specific diagnostic indicators, the majority of patients are detected in the advanced phase of the illness, either with localized or distant metastasis, resulting in a mere 46% survival rate after five years [3]. The current primary treatment for ovarian cancer primarily relies on the surgical excision of the tumours, with chemotherapy as a supplementary measure [4]. Despite the fact that over 50% of patients can attain remission through standard treatment procedures [5], resistance to platinum-based chemotherapeutic agents and molecular targeted therapeutic drugs remain a common occurrence. However, there are restrictions on the variety and effectiveness of suitable targeted medications, and ovarian cancer has a tendency to relapse and spread following the initial treatment recovery [6]. Treating end stage or recurring ovarian cancer has become increasingly challenging due to the absence of efficient medications. Hence, there remains a requirement for novel therapeutic targets to enhance the survival rate and prognosis of these individuals.
The identification of new therapeutic targets or agents is closely linked to the investigation of the molecular mechanism that underlies tumour formation and progression. The cause of ovarian cancer remains unclear, but it is thought to be associated with gonadotropin, genetic factors, inflammation, and other factors [7]. First, existing theory suggests that gonadotrophins and oestrogens act on the ovarian surface epithelium to accumulate DNA damage that eventually leads to malignant transformation [8]. Further, alterations in the breast-cancer susceptibility gene 1 (BRCA1) gene are linked to approximately 90% of familial instances of ovarian cancer [9]. The presence of the BRCA1 protein in ovarian serous cystadenocarcinoma is greatly diminished, resulting in increased cell growth and decreased cell death, ultimately causing cancer [10]. In addition, research indicates that pelvic inflammatory disease significantly elevates the likelihood of developing ovarian cancer. During the immune response, immune cells have the ability to stimulate the growth of blood vessels and the transformation of epithelial cells into mesenchymal cells, which ultimately contributes to the formation and progression of tumours [11,12].
In the last few years, an increasing number of scientists have directed their attention towards the correlation involving ferroptosis and ovarian cancer. The term ferroptosis was introduced by Dixon and colleagues. A decade prior, non-apoptotic cell death was found to rely on iron. An abnormal buildup of intracellular reactive oxygen species (ROS) and cell membrane lipid peroxidation are the defining features [13]. Mitochondria are rich in iron and are the primary organelles that produce ROS. Hence, mitochondria are regarded as a crucial location for the incidence of ferroptosis. Ferroptosis is characterized by decreased mitochondria, altered permeability of the outer mitochondrial membranes, and heightened membrane density. ROS can influence cell membrane fluidity and permeability via lipid peroxidation that leads to cell death [4]. Prior research has indicated that ferroptosis can potentially be linked to several types of malignancies, including hepatocellular carcinoma [14], colorectal cancer [15], and gastric cancer [16]. This article outlines the process of ferroptosis in ovarian cancer.
Ferroptosis in the Ovarian Cancer (OVCA) Mechanism
Major mechanism of ferroptosis
The regulatory mechanism of ferroptosis involves the system Xc- and GPX4 [17,18]. The Xc- system is made up of dimers of SLC7A11 and SLC3A2 that function as a cystine-glutamate anti-transporter system. SLC7A11 has the ability to carry cystine into cells, supplying the necessary components for the synthesis of GSH [19]. Therefore, inhibiting SLC7A11 expression can reduce cystine uptake and lead to decreased glutathione peroxidase activity. Further, the cells experience a decrease in their antioxidant capacity, while their susceptibility to iron ptosis is heightened. P53, a crucial gene for suppressing tumours, plays a significant role in the development of cancer in healthy cells. During ferroptosis, p53 has the ability to interact with certain genes downstream in order to control the advancement of ferroptosis [20]. Moreover, it has been discovered that p53 targets SLC7A11 in ferroptosis. By downregulating the expression of SLC7A11, the tumour suppressor gene, p53, promotes ferroptosis induced by ROS. SLC3A2 transports a cystine molecule into the cell and exports a glutamate molecule out of the cell. Once inside cells, cystine undergoes conversion to cysteine that utilized for the synthesis of glutathione and subsequent production of GPX4. Suppression of SLC3A2 manifestation leads to reduced levels of cysteine within cells, hindering the lipid restoration capability of GPX4 and impeding ferroptosis [21]. The GPX4 enzyme is the exclusive glutathione peroxidase employed in cellular liposomal peroxide reduction. GPX4 prevents the accumulation of lipid free radicals by reducing toxic lipid peroxides into harmless fatty alcohols. Inactivation of GPX4 leads to the conversion of accumulated lipid peroxide into a harmful lipid free radical, resulting in the degradation of polyunsaturated fatty acids. Finally, ferroptosis is induced [22–24]. Fig. 1 illustrates that the RAS-specific deadly 3 (RSL3) protein binds directly to the GPX4 protein, leading to its deactivation, thus resulting in the formation of lipid ROS and ultimately inducing cell ferroptosis. Further, cells that overexpress GPX4 exhibit resistance to RSL3 and hinder the occurrence of ferroptosis induced by it [25].
Figure 1: Mechanisms of ferroptosis. Tumor cells induce ferroptosis by causing lipid ROS accumulation via system Xc- and GPX4.
The mechanism of ferroptosis, a novel form of programmed cell death, remains incompletely understood. Current research is still actively investigating the mechanism of ferroptosis. In addition to the major mechanism of ferroptosis, the Hippo pathway is currently the most studied in ovarian cancer, as described below. Yang et al. discovered that the density of cells in ovarian cancer has an impact on their vulnerability to ferroptosis. Ovarian cancer cells with increased confluence exhibit reduced vulnerability to ferroptosis [26]. Research indicates that YAP/TAZ, an effector of the Hippo pathway, has the capability to perceive cell density. NOX2 is an enzyme complex. It can consume NADPH to produce oxidizing radicals and induce ferroptosis. A genetic analysis revealed that TAZ has the ability to control ferroptosis in ovarian cancer cells through the TAZ-ANGPTL4-NOX2 pathway [27]. Moreover, TAZ is employed to eliminate the transcription coactivator, resulting in a reduction in vulnerability to ferroptosis. Increased cellular density induces an upregulation of TAZ expression, indicating that cells modulate their density via ferroptosis. Cancerous cells proliferate quickly and possess a high level of density. Consequently, an elevated level of TAZ is associated with a heightened susceptibility of cancerous cells to ferroptosis. Further, it was discovered that patients experiencing a relapse of ovarian cancer exhibited reduced TAZ levels and a diminished susceptibility to ferroptosis as a result of their chemical resistance. Similary, an investigation of YAP demonstrated empirical proof that indicated the elimination of YAP resulted in reduced susceptibility of ovarian cancer cells to ferroptosis. YAP controls the regulation of S-phase kinase-associated protein 2 (SKP2) in ferroptosis, and the elimination of YAP suppresses the expression of SKP2. In addition, YAP or SKP2 knockout eliminates erastin-induced lipid peroxidation. Together, YAP has the ability to control the expression of the YAP direct target gene, SKP2, in order to determine ferroptosis [23]. In summary, the Hippo pathway has the ability to control the activity of NOX2, thereby influencing the cell’s susceptibility to ferroptosis (Fig. 2). The significance of the Hippo pathway effectors and ferroptosis in therapy is crucial.
Figure 2: Ovarian cancer cells sense cell density and regulate ferroptosis through the Hippo pathway.
The ferroptosis mechanism in ovarian carcinoma is also associated with many other pathways. In addition to these two pathways, researchers have also found a number of independent lipid peroxide-clearing pathways within cells. For example, there is a FSP1-CoQ10 protection pathway. When FSP1 undergoes myristoylation, it can also inhibit lipid peroxidation and ferroptosis. Moreover, in the GCH1 protection pathway, GCH1 inhibits ferroptosis by limiting the rate of biosynthesis of tetrahydrobipterin (BH4), a metabolite of lipid peroxides (Fig. 1) [28]. There are many such pathways, but the mechanisms of these pathways need to be confirmed by more studies that will not be described in detail in this paper. Currently, many scientific studies are still slowly enriching our knowledge of the mechanism of ferroptosis.
LncRNA and miRNAs in ferroptosis
Discovering the molecular mechanisms behind ovarian cancer will enable us to uncover more efficient therapies. In epithelial ovarian carcinoma, Cai et al. found a significant increase in the expression of long non-coding RNA (LncRNA) ADAMTS9-AS1 [29]. Upregulating the expression of ADAMTS9-AS1 leads to a decrease in microRNA-587 expression and an increase in SLC7A11 expression. System Xc- includes SLC7A11 as one of its constituents. SLC7A11 alters the role of GPX4 by transporting cystine into cells, thereby converting it into GSH via a sequence of chemical reactions [21]. This mechanism promotes ferroptosis and inhibits cell proliferation. In summary, LncRNA ADAMTS9-AS1 has the ability to control SLC7A11 expression by targeting microRNA-587, thus reducing the impact of ferroptosis [29].
MicroRNAs, also known as miRNAs, are RNA molecules that are not coded by genes and consist of single-stranded RNA molecules. By interacting with mRNA, they have the ability to control gene expression after transcription [30]. Ferroptosis also encompasses the participation of miRNAs. Prior experiments conducted by researchers have discovered that the induction of intracellular ROS accumulation through the use of lidocaine hinders the proliferation and invasiveness of ovarian cancer cells. Further, lidocaine led to an increase in the expression of miR-382-5p, whereas SLC7A11 exhibited a decrease. Generally speaking, lidocaine can regulate ferroptosis via miR-382-5P/SLC7A11 [31]. Mashima et al. demonstrated that the expression of ferroptosis is regulated by miR-424-5p via direct targeting of the acyl-CoA synthetase long-chain family member 4 (ACSL4). ACSL4 belongs to the long chain fatty acyl coenzyme family. To create associated lipids, it combines CoA with long-chain fatty acids to produce long-chain acyl-CoA [32]. Over-expression of ACSL4 leads to the presence of oxidized phospholipids on cancer cell surfaces, enhancing their susceptibility to ferroptosis. Ferroptosis inducers, such as erastin and RSL3, have the ability to selectively eliminate tumour cells with RAS mutations. Elevating the expression of miR-424-5p hinders the expression of ACSL4 and decreases the sensitivity of ovarian cancer cells to erastin and RSL3. In addition, an increase in ACSL4 expression can lead to a reduction in miR-424-5p expression, potentially resulting in the heightened sensitivity of ovarian cancer cells (Fig. 3) [30]. These findings all suggest potential therapeutic approaches for ovarian cancer.
Figure 3: The abnormal expression of lncRNA and miRNA can lead to ferroptosis of tumor cells.
Nuclear factor erythroid 2-related factor 2 (NRF2) inhibits ferroptosis
Prior studies have demonstrated that NRF2 can impede ferroptosis in cancer cells. Several academics have suggested that NRF2 has the ability to facilitate the production and operation of GPX4, eliminate lipid peroxidation, and uphold the balance of iron within cells [33]. Cancer cells develop resistance to ferroptosis with prolonged utilization of erastin. The primary reason for this resistance was determined to be an increase in expression of cystathionine β-synthase (CBS). Liu et al. found that NRF2 upregulated CBS, and CBS knockdown by RNA interference (RNAi) results in ferroptosis. The researchers concluded that erastin-induced ferroptosis resistance is conferred by NRF2/CBS activation [34]. Carboxymethylated pachyman (CMP), when administered in the correct dosage, has the ability to enhance the levels of superoxide dismutase (SOD) and Fe2+ while also impeding the formation of GSH in ovarian carcinoma cells. Ovarian cancer cells generate GSH. Further, CMP also results in a noteworthy decrease in NRF2, heme oxygenase-1 (HO-1), cystine glutamate reverse transporter (xCT), and GPX4 expression. Ferroptosis is influenced by both of these elements in cells affected by ovarian cancer [35].
According to You et al., the involvement of ferroptosis in ovarian cancer progression and invasion may be attributed to its impact on both tumour metastasis and immune responses [36]. The action of a platinum medication involves inducing cell damage and cell death by producing ROS within cells [37]. Wang et al. discovered that in ovarian cancer cells that can tolerate platinum (Pt-T), the presence of Frizzled-7 increases the likelihood of triggering ferroptosis via the FZD7–β-catenin–Tp63–GPX4 pathway. First, they found that Frizzled-7 (FZD7) is a receptor, and platinum-resistant cancer cells are plentiful. In ovarian cancer cells, the sensitivity to platinum is enhanced by the knockout of FZD7. Pt-T tumour cells exhibit an upregulation of GPX4. Increased levels of FZD7 trigger the activation of the cancer-causing agent Tp63, leading to an elevation in GPX4 expression and ultimately causing resistance to platinum in cancer cells [38].
The downregulation of GPX4 and SLC7A11 expression, promotion of lipid peroxidation, accumulation of large amounts of ROS, and subsequent occurrence of ferroptosis have been observed in human serum incubated- superparamagnetic iron oxides (SPIO-Serum). Further, the upregulation of the p53 tumour suppressor gene facilitates the buildup of lipid peroxidation substances caused by SPIO-serum, contributing to the development of ferroptosis and the suppression of proliferation in ovarian cancer cells [39].
Jin et al. disclosed that by promoting ferroptosis, snail homolog 2 (SNAI2) knockout prevented ovarian cancer’s emergence and development. Moreover, it was demonstrated that the direct association between SNAI2 and the SLC7A11 promoter prompted the onset of ferroptosis in ovarian cancer. In ovarian cancer, the anti-tumour activity of erastin is ultimately diminished by the excessive expression of SNAI2 [40].
One study provided evidence that sodium molybdate (Na2MoO4), a soluble Mo compound, produces nitrous oxide (NO) dependence on Mo-enzymes sulfite oxidase (SUOX), aldehyde oxidase (AOX), and the mitochondrial amidoxime-reducing component (mARC1). In addition, the high production of NO depletes GSH. Conversely, the labile iron pool (LIP) is augmented by sodium molybdate, leading to ferroptosis of ovarian cancer cells [41].
Ferroptosis has many intricate regulatory mechanisms, but there exists only a rudimentary grasp of the primary regulatory mechanisms. By delving deeper into the study of ferroptosis and incorporating advancements in technology, it is anticipated that scientists will gain a more comprehensive understanding of the regulatory mechanism behind ferroptosis, ultimately leading to potential benefits for individuals diagnosed with ovarian cancer.
The primary challenge in the study of cancer medications is finding a way to efficiently eliminate cancerous cells while minimizing harm to healthy cells. There is currently a lack of a particular specialized medication for ovarian cancer in the clinical setting. Finding a novel treatment method is crucial for lowering the mortality rate associated with ovarian cancer.
Impact of traditional Chinese medicine on ferroptosis
Over the last several years, researchers have gradually uncovered the anti-cancer properties of traditional Chinese medicine (TCM), including its ability to prevent drug resistance and minimize side effects. The concept of ferroptosis additionally provides a fresh perspective and pathway for investigating the potential of TCM for cancer treatment. Artesunate (ART) is a natural classic anti-malarial drug derived from Artemisia annua, a TCM [42]. Significant inhibitory effects on ovarian carcinoma cells and notable cytotoxicity were observed upon treatment with ART. ART-treated ovarian cancer cells exhibited robust ROS induction, leading to ferroptosis activation and the cancer cell growth suppression [43]. Further, the use of ferroptosis inhibitors did not entirely suppress the cytotoxic effects of artemisinin on ovarian cancer cell lines. This suggests that multiple mechanisms play a role in the cytotoxic effects of artemisinin on ovarian cancer cells [44]. The results suggested a prospective role for ART in treating ovarian cancer [45]. In addition, baicalein was also found to be a natural inhibitor of ferroptosis. It primarily inhibits Fe2+ generation, reducing glutathione consumption, and also prevents lipid peroxidation, inhibits ROS production, and eventually reduces cell death [46].
Solution of the chemical resistance problem
Ovarian cancer is typically treated with chemotherapy drugs such as cisplatin. By forming crosslinks with DNA, cisplatin has the ability to induce DNA damage in tumour cells and trigger mitochondrial apoptosis, ultimately leading to its anti-tumour effects [47]. However, some patients may develop multidrug resistance with varying degrees of use and duration of cisplatin. Drug resistance to chemotherapy contributes toa low survival rate among individuals with ovarian cancer. Compounds that trigger ferroptosis have the ability to cause the demise of cancer cells, offering a novel approach to address the issue of drug resistance. Research conducted in China discovered that erastin enhanced the cytotoxic impact of cisplatin on cells of ovarian cancer. In human epithelial ovarian cancer cells (EOC), erastin, a ferroptosis inducer with low concentration, can enhance the number of apoptotic cells induced by cisplatin. As a result, research has demonstrated that the combination of erastin and cisplatin effectively suppresses the growth of ovarian cancer cells [48]. Erastin and its analogs act as inhibitors of System Xc-, leading to the induction of ferroptosis. Hence, the utilization of ferroptosis inducing substances or inhibitors to trigger ferroptosis has emerged as a fresh therapeutic approach for treating ovarian cancer.
The multi-kinase inhibitor, sorafenib, is a clinically approved anticancer drug that can induce ferroptosis. By inhibiting System Xc-, it has the ability to activate the NRF2/SLC7A11 signaling pathway, thereby inducing ferroptosis in cancer cells. Nevertheless, the efficacy and safety of these medications are currently being researched [49]. The existence of peripheral cancer cells (PPCs) [50] is primarily responsible for the development of drug resistance in most cases. Gao et al. suggested that initiated ferroptosis polymer micelles caused the prompt discharge of payload, subsequently exhausting GSH [50]. As a result, the capacity of ferroptosis to eradicate dormant PCCs is heightened, leading to an enhancement in its anti-tumour efficacy [50]. This outcome has the potential to serve as a viable therapeutic strategy for addressing drug resistance in ovarian cancer, mitigating tumour recurrence, and offering a novel resolution to the issue of multidrug resistance in cancer. Zhou et al. provided evidence suggesting a potential correlation between the overexpression of adenosine triphosphate-binding cassette subfamily B member 1 (ABCB1) and the reoccurrence of resistance to docetaxel [51]. Erastin can reverse the resistance to docetaxel mediated by ABCB1 in individuals diagnosed with ovarian cancer. The implication was that a combination of erastin and docetaxel might be advantageous for ovarian cancer patients in overcoming drug resistance [51].
Effectiveness of ovarian cancer treatment is improved by the use of combination therapies
According to a report by Chan et al., the combination of momordica anti-HIV protein of 30 kDa (MAP30), a biologically active protein derived from bitter melon seeds, and a small amount of cisplatin, effectively suppressed the proliferation and metastasis of cancer [52]. Experiments on cellular function confirmed that MAP30 had a suppressive impact on the growth of cancerous ovarian cells, while it had no influence on normal ovarian epithelial cells. Additional animal trials revealed that MAP30 did not have any impact on the functioning of the liver and kidneys. These studies suggested that MAP30, as a nontoxic supplement, might enhance chemotherapy efficacy in advanced ovarian cancer. A study that examined the role of iron found that a high LIP content promoted the Fenton response that generates significant amounts of ROS and triggers ferroptosis [53]. Erastin failed to trigger ferroptosis in ovarian cancer cells that exhibited low intracellular LIP levels. The administration of Ferlixit increased the susceptibility of ovarian cancer cells to erastin, resulting in the induction of ferroptosis. They demonstrated a small dose of Ferlixit improved erastin-based ovarian cancer treatments [54].
In ovarian cancers, it was discovered that there is a significant presence of stearoyl CoA desaturase (SCD1) expression that effectively shields ovarian cancer cells from undergoing apoptosis [55]. Blocking SCD1 can trigger the onset of ferroptosis. The combination of an SCD1 inhibitor and ferroptosis could potentially reduce the likelihood of ovarian cancer development. This may lead to the development of a new therapy for advanced ovarian cancer [56]. The Menin-MLL inhibitor has shown some effectiveness in treating patients with MLL-retime. Researchers accidentally discovered that it can induce ferroptosis in cell lines of ovarian cancer; it might be associated with SCD1 inhibition. Research conducted in Japan has indicated that the pairing of Menin-MLL inhibitors, such as MI-463, with auranofin (a substance that inhibits thioredoxin reductase), could potentially serve as a successful therapy for ovarian cancer by triggering ferroptosis [57].
Role of other molecules in ferroptosis therapy
Further, certain molecules possess the capability to influence the proliferation of neoplastic cells via ferroptosis, thereby presenting a promising avenue for cancer therapy. Tagitinin C, for instance, has the ability to stimulate ferroptosis-associated pathways within the endoplasmic reticulum, thereby expediting the demise of malignant cells. Consequently, Tagitinin C can be considered a chemical sensitizer, augmenting the efficacy of chemotherapy agents and enabling them to exert a more potent impact on neoplastic cells [58]. Moreover, the inhibition of tumor growth is facilitated by beclin 1 (BECN1) through the suppression of the system Xc- activity, ultimately resulting in the induction of ferroptosis [59]. Moreover, the suppression of CDGSH iron sulfur domain 1 (CISD1) expression has been observed to induce mitochondrial lipid peroxidation in cancer cells, thereby playing a role in the ferroptosis induced by erastin [60]. Numerous ferroptosis-related molecules have been identified as potential agents for cancer therapy. Nevertheless, the existing body of research provides limited evidence, necessitating further investigations to elucidate the precise mechanism underlying their therapeutic application.
In summary, despite being in the early stages of research, the study of ferroptosis in cancer therapy shows immense potential for application. Although the exact mechanism of its effectiveness against different types of cancer remains unclear, it is expected to emerge as a promising new avenue for cancer treatment in the future.
Researchers have become increasingly interested in ferroptosis as a cancer-related topic. Multiple studies have demonstrated that ferroptosis possesses an anti-cancer impact by impeding the growth of ovarian cancer and inducing the demise of tumour cells. Currently, a preliminary framework has been established that is comprised of System Xc-, GPX4, and other regulatory systems, while additional crucial mechanisms require further investigation. Currently, platinum and paclitaxel remain the primary chemotherapy treatments for ovarian cancer. Nevertheless, patients with advanced ovarian cancer are highly likely to experience recurrence and develop resistance to chemotherapy. It is crucial to tackle the resistance to chemotherapy in order to treat and decrease the likelihood of the return of ovarian cancer, and ferroptosis holds promise in reversing the mechanism that causes resistance. It is hoped that ferroptosis will become a new therapeutic approach for ovarian cancer. However, targeting ferroptosis for cancer diseases such as ovarian cancer still has problems that require solutions. Although drugs targeting ferroptosis speed up the demise of cancer cells, they also exhibit significant toxicity towards healthy cells. Hence, enhancing the security of medications is a significant obstacle that must be confronted. Additional investigation is anticipated to result in the rational utilization of substances that induce and hinder ferroptosis in the near future, thus leading to the development of novel and efficient chemotherapy protocols for the treatment of ovarian cancer and various other malignancies.
Acknowledgement: We thank LetPub (www.letpub.com) for its linguistic assistance during the preparation of this manuscript. All of the figures in the article were drawn by Figdraw.
Funding Statement: The authors received no specific funding for this study.
Author Contributions: The authors confirm contribution to the paper as follows: study conception and design: Lisha Ma; data collection: Weili Zhu; analysis and interpretation of results: Wanqi Shao; draft manuscript preparation: Lisha Ma. All authors reviewed the results and approved the final version of the manuscript.
Availability of Data and Materials: Data sharing not applicable to this article as no datasets were generated or analyzed during the current study.
Ethics Approval: Not applicable.
Conflicts of Interest: The authors declare that they have no conflicts of interest to report regarding the present study.
References
1. Siegel RL, Miller KD, Fuchs HE, Jemal A. Cancer statistics, 2021. CA: Cancer J Clin. 2021;71(1):7–33, 2021. [Google Scholar] [PubMed]
2. Sung H, Ferlay J, Siegel RL, Laversanne M, Soerjomataram I, Jemal A, et al. Global Cancer Statistics 2020: GLOBOCAN estimates of incidence and mortality worldwide for 36 cancers in 185 countries. CA: Cancer J Clin. 2021;71(3):209–49. [Google Scholar] [PubMed]
3. Armstrong DK, Alvarez RD, Bakkum-Gamez JN, Barroilhet L, Behbakht K, Berchuck A, et al. Ovarian cancer, Version 2.2020, NCCN clinical practice guidelines in oncology. J Natl Compr Canc Netw. 2021;19(2):191–226. [Google Scholar] [PubMed]
4. Cortez AJ, Tudrej P, Kujawa KA, Lisowska KM. Advances in ovarian cancer therapy. Cancer Chemother Pharmacol. 2018;81(1):17–38. [Google Scholar] [PubMed]
5. Yang L, Xie HJ, Li YY, Wang X, Liu XX, Mai J. Molecular mechanisms of platinum‐based chemotherapy resistance in ovarian cancer. Oncol Rep. 2022;47(4):82. [Google Scholar] [PubMed]
6. Valenti G, Sopracordevole F, Chiofalo B, Forte S, Ciancio F, Fiore M, et al. Parenchymal liver metastasis in advanced ovarian cancer: can bowel involvement influence the frequency and the related mortality rate. Eur J Obstet Gynecol Reprod Biol. 2023;280:48–53. [Google Scholar] [PubMed]
7. Zhao X, Liu Z, Chen T. Potential role of vaginal microbiota in ovarian cancer carcinogenesis, progression and treat. Pharm. 2023;15(3):948. [Google Scholar]
8. Loizzi V, Dellino M, Cerbone M, Arezzo F, Cazzato G, Damiani GR, et al. The role of hormonal replacement therapy in brca mutated patients: llights and shadows. Int J Mol Sci. 2023;24(1):764. [Google Scholar] [PubMed]
9. Ueki A, Yoshida R, Kosaka T, Matsubayashi H. Clinical risk management of breast, ovarian, pancreatic, and prostatic cancers for BRCA1/2 variant carriers in Japan. J Hum Genet. 2023;68(8):517–26. [Google Scholar] [PubMed]
10. Liu Y, Shen Z, Zhu T, Lu W, Fu Y. Curcumin enhances the anti-cancer efficacy of paclitaxel in ovarian cancer by regulating the miR-9-5p/BRCA1 axis. Front Pharmacol. 2022;13:1014933. [Google Scholar] [PubMed]
11. Lo HW, Weng SF, Chen HS, Tsai EM. Pelvic inflammatory disease is associated with ovarian cancer development in women with endometriosis: a cohort study in Taiwan. Int J Gynaecol Obstet. 2022;158(1):145–52. [Google Scholar] [PubMed]
12. Cheng L, Zhou S, Zhou S, Shi K, Cheng Y, Cai MC, et al. Dual inhibition of CDK12/CDK13 targets both tumor and immune cells in ovarian cancer. Cancer Res. 2022;82(19):3588–602. [Google Scholar] [PubMed]
13. Yin J, Meng X, Peng L, Xie W, Liu X, He W, et al. Ferroptosis and cancer immunotherapy. Curr Mol Med. 2023;23(5):401–9. [Google Scholar] [PubMed]
14. Tan J, Xiang Y, Xiong Y, Zhang Y, Qiao B, Zhang H. Crebanine induces ROS-dependent apoptosis in human hepatocellular carcinoma cells via the AKT/FoxO3a signaling pathway. Front Pharmacol. 2023;14:1069093. doi:10.3389/fphar.2023.1069093. [Google Scholar] [PubMed] [CrossRef]
15. Kwak AW, Kim WK, Lee SO, Yoon G, Cho SS, Kim KT, et al. Licochalcone B induces ROS-dependent apoptosis in oxaliplatin-resistant colorectal cancer cells via p38/JNK MAPK signaling. Antioxidants. 2023;12(3):656. [Google Scholar] [PubMed]
16. Cen K, Wu Z, Mai Y, Dai Y, Hong K, Guo Y. Identification of a novel reactive oxygen species (ROS)-related genes model combined with RT-qPCR experiments for prognosis and immunotherapy in gastric cancer. Front Genet. 2023;14:1074900. [Google Scholar] [PubMed]
17. Xue Q, Yan D, Chen X, Li X, Kang R, Klionsky DJ, et al. Copper-dependent autophagic degradation of GPX4 drives ferroptosis. Autophagy. 2023;19(7):1982–96. [Google Scholar] [PubMed]
18. Koeberle SC, Kipp AP, Stuppner H, Koeberle A. Ferroptosis-modulating small molecules for targeting drug-resistant cancer: challenges and opportunities in manipulating redox signaling. Med Res Rev. 2023;43(3):614–82. [Google Scholar] [PubMed]
19. Heit BS, Chu A, Sane A, Featherstone DE, Park TJ, Larson J. Tonic extracellular glutamate and ischaemia: glutamate antiporter system xc− regulates anoxic depolarization in hippocampus. J Physiol. 2023;601(3):607–29. [Google Scholar] [PubMed]
20. Liu Y, Gu W. p53 in ferroptosis regulation: the new weapon for the old guardian. Cell Death Differ. 2022;29(5):895–910. [Google Scholar] [PubMed]
21. Viktorinova A. Future perspectives of oxytosis/ferroptosis research in neurodegeneration diseases. Cell Mol Neurobiol. 2023;43(6):2761–8. [Google Scholar] [PubMed]
22. Tang D, Kang R, Berghe TV, Vandenabeele P, Kroemer G. The molecular machinery of regulated cell death. Cell Res. 2019;29(5):347–64. [Google Scholar] [PubMed]
23. Yang WH, Lin CC, Wu J, Chao PY, Chen K, Chen PH, et al. The hippo pathway effector YAP promotes ferroptosis via the E3 ligase SKP2. Mol Cancer Res. 2021;19(6):1005–14. doi:10.1158/1541-7786.MCR-20-0534. [Google Scholar] [PubMed] [CrossRef]
24. Zhang J, Wang X, Guan B, Wang X, An X, Wang T, et al. Qing-Xin-Jie-Yu Granule inhibits ferroptosis and stabilizes atherosclerotic plaques by regulating the GPX4/xCT signaling pathway. J Ethnopharmacol. 2023;301:115852. doi:10.1016/j.jep.2022.115852. [Google Scholar] [PubMed] [CrossRef]
25. Cheff DM, Huang C, Scholzen KC, Gencheva R, Ronzetti MH, Cheng Q, et al. The ferroptosis inducing compounds RSL3 and ML162 are not direct inhibitors of GPX4 but of TXNRD1. Redox Biol. 2023;62:102703. [Google Scholar] [PubMed]
26. Yang WH, Huang Z, Wu J, Ding CC, Murphy SK, Chi JT. A TAZ-ANGPTL4-NOX2 axis regulates ferroptotic cell death and chemoresistance in epithelial ovarian cancer. Mol Cancer Res. 2020;18(1):79–90. [Google Scholar] [PubMed]
27. Yang WH, Ding CC, Sun T, Rupprecht G, Lin CC, Hsu D, et al. The hippo pathway effector TAZ regulates ferroptosis in renal cell carcinoma. Cell Rep. 2019;28(10):2501–8. [Google Scholar] [PubMed]
28. Li L, Qiu C, Hou M, Wang X, Huang C, Zou J, et al. Ferroptosis in ovarian cancer: a novel therapeutic strategy. Front Oncol. 2021;11:665945. [Google Scholar] [PubMed]
29. Cai L, Hu X, Ye L, Bai P, Jie Y, Shu K. Long non-coding RNA ADAMTS9-AS1 attenuates ferroptosis by Targeting microRNA-587/solute carrier family 7 member 11 axis in epithelial ovarian cancer. Bioengineered. 2022;13(4):8226–39. [Google Scholar] [PubMed]
30. Ma LL, Liang L, Zhou D, Wang SW. Tumor suppressor miR-424-5p abrogates ferroptosis in ovarian cancer through targeting ACSL4. Neoplasma. 2021;68(1):165–73. [Google Scholar] [PubMed]
31. Sun D, Li YC, Zhang XY. Lidocaine Promoted ferroptosis by targeting miR-382-5p/SLC7A11 Axis in ovarian and breast cancer. Front Pharmacol. 2021;12:681223. [Google Scholar] [PubMed]
32. Mashima T, Seimiya H, Tsuruo T. De novo fatty-acid synthesis and related pathways as molecular targets for cancer therapy. Br J Cancer. 2009;100(9):1369–72. [Google Scholar] [PubMed]
33. Song X, Long D. Nrf2 and ferroptosis: a new research direction for neurodegenerative diseases. Front Neurosci. 2020;14:267. [Google Scholar] [PubMed]
34. Liu N, Lin X, Huang C. Activation of the reverse transsulfuration pathway through NRF2/CBS confers erastin-induced ferroptosis resistance. Br J Cancer. 2020;122(2):279–92. [Google Scholar] [PubMed]
35. Jing T, Guo Y, Wei Y. Carboxymethylated pachyman induces ferroptosis in ovarian cancer by suppressing NRF1/HO-1 signaling. Oncol Lett. 2022;23(5):161. [Google Scholar] [PubMed]
36. You Y, Fan Q, Huang J, Wu Y, Lin H, Zhang Q. Ferroptosis-related gene signature promotes ovarian cancer by influencing immune infiltration and invasion. J Oncol. 2021;2021:9915312. [Google Scholar] [PubMed]
37. Santos I, Ramos C, Mendes C, Sequeira CO, Tomé CS, Fernandes D, et al. Targeting glutathione and cystathionine β-synthase in ovarian cancer treatment by selenium-chrysin polyurea dendrimer nanoformulation. Nutrients. 2019;11(10):2523. [Google Scholar] [PubMed]
38. Wang Y, Zhao G, Condello S, Huang H, Cardenas H, Tanner EJ, et al. Frizzled-7 identifies platinum-tolerant ovarian cancer cells susceptible to ferroptosis. Cancer Res. 2021;81(2):384–99. [Google Scholar] [PubMed]
39. Zhang Y, Xia M, Zhou Z, Hu X, Wang J, Zhang M, et al. p53 promoted ferroptosis in ovarian cancer cells treated with human serum incubated-superparamagnetic iron oxides. Int J Nanomedicine. 2021;16:283–96. [Google Scholar] [PubMed]
40. Jin Y, Chen L, Li L, Huang G, Huang H, Tang C. SNAI2 promotes the development of ovarian cancer through regulating ferroptosis. Bioeng. 2022;13(3):6451–63. [Google Scholar]
41. Mao G, Xin D, Wang Q, Lai D. Sodium molybdate inhibits the growth of ovarian cancer cells via inducing both ferroptosis and apoptosis. Free Radic Biol Med. 2022;182:79–92. [Google Scholar] [PubMed]
42. Huang QF, Li YH, Huang ZJ, Jun M, Wang W, Chen XL, et al. Artesunate carriers induced ferroptosis to overcome biological barriers for anti-cancer. Eur J Pharm Biopharm. 2023;190:284–93. [Google Scholar] [PubMed]
43. McDowellJr A, Hill KS, McCorkle JR, Gorski J, Zhang Y, Salahudeen AA, et al. Preclinical evaluation of artesunate as an antineoplastic agent in ovarian cancer treatment. Diagnostics. 2021;11(3):395. [Google Scholar] [PubMed]
44. Efferth T. From ancient herb to modern drug: Artemisia annua and artemisinin for cancer therapy. Semin Cancer Biol. 2017;46:65–83. [Google Scholar] [PubMed]
45. Greenshields AL, Shepherd TG, Hoskin DW. Contribution of reactive oxygen species to ovarian cancer cell growth arrest and killing by the anti-malarial drug artesunate. Mol Carcinog. 2017;56(1):75–93. [Google Scholar] [PubMed]
46. Perez CA, Wei Y, Guo M. Iron-binding and anti-fenton properties of baicalein and baicalin. J Inorg Biochem. 2009;103(3):326–32. [Google Scholar] [PubMed]
47. Dasari S, Njiki S, Mbemi A, Yedjou CG, Tchounwou PB. Pharmacological effects of cisplatin combination with natural products in cancer chemotherapy. Int J Mol Sci. 2022;23(3):1532. [Google Scholar] [PubMed]
48. Cheng Q, Bao L, Li M, Chang K, Yi X. Erastin synergizes with cisplatin via ferroptosis to inhibit ovarian cancer growth in vitro and in vivo. J Obstet Gynaecol Res. 2021;47(7):2481–91. [Google Scholar] [PubMed]
49. Nassar D, Blanpain C. Cancer stem cells: basic concepts and therapeutic implications. Annu Rev Pathol. 2016;11:47–76. [Google Scholar] [PubMed]
50. Gao M, Deng J, Liu F, Fan A, Wang Y, Wu H, et al. Triggered ferroptotic polymer micelles for reversing multidrug resistance to chemotherapy. Biomater. 2019;223:119486. [Google Scholar]
51. Zhou HH, Chen X, Cai LY, Nan XW, Chen JH, Chen XX, et al. Erastin reverses ABCB1-mediated docetaxel resistance in ovarian cancer. Front Oncol. 2019;9:1398. [Google Scholar] [PubMed]
52. Chan DW, Yung MM, Chan YS, Xuan Y, Yang H, Xu D, et al. MAP30 protein from Momordica charantia is therapeutic and has synergic activity with cisplatin against ovarian cancer in vivo by altering metabolism and inducing ferroptosis. Pharmacol Res. 2020;161:105157. [Google Scholar] [PubMed]
53. Battaglia AM, Sacco A, Perrotta ID, Faniello MC, Scalise M, Torella D, et al. Iron administration overcomes resistance to erastin-mediated ferroptosis in ovarian cancer cells. Front Oncol. 2022;12:868351. [Google Scholar] [PubMed]
54. Tesfay L, Paul BT, Konstorum A, Deng Z, Cox AO, Lee J, et al. Stearoyl-CoA desaturase 1 protects ovarian cancer cells from ferroptotic cell death. Cancer Res. 2019;79(20):5355–66. [Google Scholar] [PubMed]
55. Liu Y, Liu X, Wang H, Ding P, Wang C. Agrimonolide inhibits cancer progression and induces ferroptosis and apoptosis by targeting SCD1 in ovarian cancer cells. Phytomed. 2022;101:154102. [Google Scholar]
56. Kato I, Kasukabe T, Kumakura S. Menin‐MLL inhibitors induce ferroptosis and enhance the anti‐proliferative activity of auranofin in several types of cancer cells. Int J Oncol. 2020;57(4):1057–71. [Google Scholar] [PubMed]
57. Chekerov R, Hilpert F, Mahner S, El-Balat A, Harter P, De Gregorio N, et al. Sorafenib plus topotecan versus placebo plus topotecan for platinum-resistant ovarian cancer (TRIASa multicentre, andomized, double-blind, placebo-controlled, phase 2 trial. Lancet Oncol. 2018;19(9):1247–58. [Google Scholar] [PubMed]
58. Wei R, Zhao Y, Wang J, Yang X, Li S, Wang Y, et al. Tagitinin C induces ferroptosis through PERK-Nrf2-HO-1 signaling pathway in colorectal cancer cells. Int J Biol Sci. 2021;17(11):2703–17. [Google Scholar] [PubMed]
59. Song X, Zhu S, Chen P, Hou W, Wen Q, Liu J, et al. AMPK-mediated BECN1 phosphorylation promotes ferroptosis by directly blocking system Xc− activity. Curr Biol. 2018;28(15):2388–2399.e5. [Google Scholar] [PubMed]
60. Yuan H, Li X, Zhang X, Kang R, Tang D. CISD1 inhibits ferroptosis by protection against mitochondrial lipid peroxidation. Biochem Biophys Res Commun. 2016;478(2):838–44. [Google Scholar] [PubMed]
Cite This Article
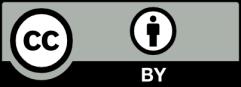