Open Access
ARTICLE
Kaempferol ameliorated levodopa-induced dyskinesia in experimental rats: A role of brain monoamines, cFOS, FosB, Parkin, Pdyn, TH, and p-JNK
Geriatrics Department, Chengde Central Hospital, Chengde, 067000, China
* Corresponding Author: JIANHUA MA. Email:
BIOCELL 2024, 48(3), 513-523. https://doi.org/10.32604/biocell.2023.045640
Received 02 September 2023; Accepted 21 November 2023; Issue published 15 March 2024
Abstract
Background: L-dopa (Levodopa) is well known for managing PD (Parkinson’s disease); however, its prolonged use caused dyskinesia (LID). Due to the varied presentation of LID, effective treatment options are scarce. Flavonoids reported their neuroprotective activity by ameliorating acetylcholinesterase, monoamine oxidase, and neuroinflammation. Kaempferol is another flavonoid bearing these potentials. Aim: To evaluate neuroprotective activity of kaempferol in dyskinetic rats. Methods: PD was developed in Sprague-Dawley rats by injecting combination of L-ascorbic acid (10 µL) + 6-OHDA (12 µg) in medial forebrain bundle to induce neuronal damage in substantial nigra (SNr). LID was induced by administrating combination of L-dopa (20 mg/kg) + benserazide HCl (5 mg/kg) for 42 days. Rats were concomitantly treated with amantadine (40 mg/kg) or kaempferol (25, 50, and 100 mg/kg, p.o.). Results: Kaempferol (50 and 100 mg/kg) markedly (p < 0.05) inhibited LID-induced abnormal involuntary movements (AIMs) and alternation in motor function. Kaempferol administration considerably (p < 0.05) inhibited reduced mitochondrial complex activities, serotonin and dopamine levels, Bcl-2, and Tyrosine hydroxylase protein expressions in SNr. Additionally, kaempferol considerably (p < 0.05) attenuated increased cFOS, FosB, Parkin, and Pdyn mRNAs expressions, Bax, cleaved caspase-3, caspase-3, and pJNK proteins levels; DOPAC and 5-HIAA levels in SNr. A positive correlation was reported between cFOS, FosB, Parkin, Pdyn, apoptosis, and TH with AIMs. Conclusion: Kaempferol effectively attenuated L-dopa-induced AIMs and dyskinesia via amelioration of alterations in cFOS, FosB, Parkin, Pdyn, Tyrosine hydroxylase, and apoptosis in the brain SNr.Graphical Abstract
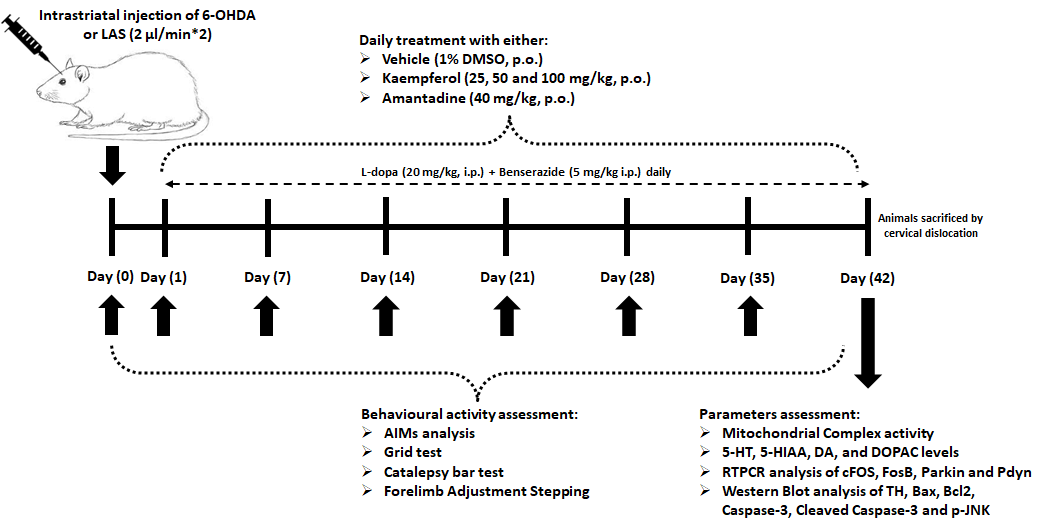
Keywords
Parkinson’s disease (PD) is a degenerative condition of neuros mainly affecting males (4%) and females (2%) aged 85 years or older [1,2]. The prevalence of PD will be increased twice in the next two decades globally [1]. The characteristic features of PD are motor dysfunction, which includes resting tremors, muscle rigidity, bradykinesia, postural instability, and gait disturbances [3]. These symptoms are linked to the selective degeneration of dopamine-specific neurons at the substantia nigra (SNr), neurodegeneration, and SNr dopamine (DA) depletion. Studies documented that PD is linked to significant clinical and economic burdens, impacting the QoL (quality of life) of both patients suffering from PD and their caregivers [4]. The average annual treatment cost for PD in China is $3,226 for individual patients [5].
Current treatment of PD includes levodopa, dopamine activators (such as apomorphine, pramipexole, etc.), monoamine oxidase (MAO)-B inhibitors (including selegiline, rasagiline, etc.) mainly focuses on improving motor symptoms by restoring DA levels [3]. L-dopa (Levodopa), a dopamine precursor, is widely used as a treatment for the management of PD. Levodopa therapy causes dopamine restoration in dopamine-deprived areas of the forebrain (striatum), thereby restoring neurotransmission. This causes a drastic symptomatic relief in the motor symptoms during the early days of the treatment; however, prolonged use of L-dopa may lead to re-emergence or even worsening symptoms due to the “wearing off” phenomenon [3]. Patients are also prone to developing dyskinesia induced by Levodopa (LID), which is more prominent in early-onset PD patients [6]. The complications may be diphasic dyskinesias, chorea, and dystonic movements. Due to the varied presentation of the LID associated with L-dopa plasma levels and abnormalities in neurotransmitter systems of LID, only amantadine has been able to be established for treating LID [6]. However, it has side effects, including ankle swelling, hallucinations, and skin allergies. Therefore, new therapies are required to treat LID.
Flavonoids are naturally occurring compounds found in plants, vegetables, and fruits. These compounds reported various pharmacological benefits, including neuroprotective, antioxidant, anti-inflammatory, and neurotrophic effects [7]. Thus, flavonoids have been researched as a potential treatment option for neurodegenerative disorders, including PD [7]. One of the flavonoids, quercetin, has been shown to have antioxidant and neuroprotective potential against rotenone-induced toxicity in experimental PD [8]. Another flavonoid, kaempferol, mainly found in broccoli, beans, tea, leeks, and onions, has been found to inhibit acetylcholinesterase and monoamine oxidase, attenuate cognitive deficit and neuroinflammation, increase memory retention and regulate antioxidants [9]. Sloley et al. reported that kaempferol in Ginkgo biloba extract is responsible for its MAO-inhibiting activity [10]. Furthermore, kaempferol has been suggested as an important selective human MAO-A inhibitor present in various plant foods, including Calabrian red wines [11]. Recently, researchers reported the anti-apoptotic potential of kaempferol via amelioration of caspase-3 and Bcl-2 Associated X-protein (Bax) expressions [12]. Kaempferol showed elevated tyrosine hydroxylase (TH) expression and decreased Caspase-3 activity in transgenic Drosophila PD model [13]. Additionally, molecular docking and pharmacophore modeling studies revealed that hydroxyl groups in kaempferol played an important role in amino acid residue interactions to inhibit the COMT (catechol-O methyltransferase inhibitors) that reduce dopamine levels [14]. These findings suggested the neuroprotective potential of kaempferol; thus, the current study aimed to determine the potential of kaempferol in dyskinetic rat.
Male SD (Sprague-Dawley) rats (220–250 g, 8–11 weeks) received from animal house facility of Chengde Central Hospital (Chengde, China) and were maintained at 23°C ± 2°C under a 12 h light/12 h dark cycle with 50% ± 10% relative humidity, provided with a sufficient food and water. The Chengde Central Hospital ethical committee for the welfare and ethics of experimental animals approved the protocol for this study (no. CDCH20230522).
A previously reported method was used to induce PD in SD rats by Unilateral administration of 6-hydroxydopamine (6-OHDA) [15]. On the day of induction of PD, rats were intraperitoneally (i.p.) injected with desipramine HCl at a dose of 25 mg/kg (obtained from Sigma-Aldrich, St. Louis, MO, USA; Catalogue number: D3900-1G) 30 min before administration of 6-OHDA. 6-OHDA (12 µg; Sigma-Aldrich, St. Louis, MO, USA; H4381-100MG) in a mixture of NaCl (0.9%, MP Biomedicals India Private Limited, Mumbai, Maharashtra, India; MB130-100G) and L-ascorbic acid [0.1%; Sigma-Aldrich, St. Louis, MO, USA; A7631-25G]) was administered in the right medial forebrain bundle of anesthetized rats using thiopental sodium at an intraperitoneal dose of 45 mg/kg (Pharmaffiliates, Panchkula, Haryana, India; PA 203080030) using stereotaxic apparatus (Harvard Apparatus, MA, Massachusetts, USA; 75-1818) with predefined coordinates as −2.5, +2.0, −9.0 mm (anterior-posterior, Medial-Lateral, and dorsal-ventral, respectively) which is relative to bregma. A Hamilton syringe (Sigma-Aldrich, St. Louis, MO, USA; HAM80075) was used to administer 6-OHDA (4 µL) at a 2 µL/min rate.
After 4 weeks of surgery, rats were injected with apomorphine at an intraperitoneal dose of 0.5 mg/kg (Sigma-Aldrich, St. Louis, MO, USA; 41372-20-7) to induce and assess asymmetry rotations (a minimum of 7 full-body rotations per minute) contra to the lesioned side [16]. Rats depicting less than seven full-body rotations per minute were grouped as hemiparkinsonian rats. Rats with more than 7 asymmetry rotations were selected for induction of LID.
Rats were randomly assigned into following groups consisted 14 rats in each group. They injected with L-dopa (20 mg/kg; Sigma-Aldrich, St. Louis, MO, USA; D9628-25G) and benserazide HCl (5 mg/kg; Sigma-Aldrich, St. Louis, MO, USA; B7283-5G) daily for 42 days. Concomitant treatment of vehicle (Dimethyl sulfoxide [DMSO; Sigma-Aldrich, St. Louis, MO, USA; D8418], 10 mL/kg, named “LID control”) or amantadine (40 mg/kg; Sigma-Aldrich, St. Louis, MO, USA; A4393-100MG) [15] or kaempferol (25, 50, and 100 mg/kg; Sigma-Aldrich, St. Louis, MO, USA; purity ≥97.0%; 60010-25G) [17,18] was provided orally for 42 days. A separate group of rats was maintained during the study period, namely sham, hemiparkinsonian, and Perse (sham treatment with kaempferol [100 mg/kg]).
A previously reported method was used to assess each abnormal involuntary movements (AIMs) subtype score (limb, axial, orolingual and total) and total AIMs [2,19]. A rota rod test (Techno Rotarod apparatus, Techno Electronics, Lukhnow, India) was used to evaluate neurological impairments and muscular coordination [20], whereas the forepaw Adjusting Steps (FAS) test was used determine akinesia [15]. A previously described method was used to evaluate the catalepsy test [15] (Graphical abstract).
Biochemical and molecular assessment
On day 43, cervical decapitation method was used to sacrificed rats. An ipsilateral portion brain SNr (n = 6) was isolated to prepare a homogenate to determine mitochondrial complex (I–IV) activities, monoamines (Dopamine [DA]; 5-hydroxytryptamine [5-HT]), respective metabolites (5-Hydroxyindoleacetic acid [5-HIAA]; 3,4-Dihydroxyphenylacetic acid [DOPAC]) [15]. Another brain SNr (n = 6) was used to determine the mRNA expressions of cFOS (Forward: 5′-AGTGGTGAAGACCATGTCAGG-3′, Reverse: 5′-CATTGGGGATCTTGCAGG-3′, base pair: 521), FosB (Forward: 5′-GTGAGAGATTTGCCAGGGTC-3′, Reverse: 5′-AGAGAGAAGCCGTCAGGTTG-3′, base pair: 245), Parkin (Forward: 5′-ACACCCAACCTCAGACAAGG-3′, Reverse: 5′-ATCAGGGAGTTGGGACAGC-3′, base pair: 252), Prodynorphin (Pdyn; Forward: 5′-TTTGCCATCTGCATAACTTTATTT-3′, Reverse: 5′-GCTCTTCATGTGTTCTGAAATGTG-3′, base pair: 164) and β-actin (Forward: 5′-GTCACCCACACTGTGCCCATCT-3′, Reverse: 5′-ACAGAGTACTTGCGCTCAGGAG-3′, base pair: 764) using reverse-transcriptase polymerase chain reaction kits (MP Biomedicals India Private Limited, Mumbai, Maharashtra, India) [15]. Protein expression of Bax (ab216494, 1:1000, Abcam, Cambridge, MA, USA), Bcl-2 (B-cell lymphoma 2; EPR17509, ab182858, 1:2000), Caspase-3 (ab32351, 1:5000), cleaved Caspase-3 (ab2302, 1:500), TH (ab6211, 1:500), p-JNK (c-Jun N-terminal Kinase; ab307802, 1:1000), and GAPDH (Glyceraldehyde 3-phosphate dehydrogenase; EPR16891, ab181602, 1:10000) was estimated using Western blot analysis [21]. Another SNr specimen from the respective group was stored for 24 h in 10% formalin (Sigma-Aldrich, St. Louis, MO, USA; HT5011) for histological examination. They were stained using Hematoxylin and eosin (H&E; Sigma-Aldrich, St. Louis, MO, USA; 1.02439) and examined under Olympus light microscope (DP-BSW Ver.03.03, Olympus Medical Systems India Private Limited, India).
During the sample size calculation an attrition was expected as 30%. Thus, a power analysis method was used to calculate the sample size using a formula: Corrected sample size = Sample size/(1−[% attrition/100]) [22]. A GraphPad Prism 5.0 software (San Diego, California, USA) was used for data analysis and reported as mean ± SEM (standard error of the mean). A one- or two-way ANOVA (analysis of variance) was used whereas Mann-Whitney (non-parametric) and Tukey’s (parametric) multiple range test was used during post-hoc analysis. A p value corresponding to <0.05 was consider as statistically significant.
Abnormal involuntary movements
The LID control group had effective increased (p < 0.05) AIMs scale scores (limb, axial, orolingual, and total) on the respective days than the sham group. Kaempferol, at a dose of 50 and 100 mg/kg, effectively (p < 0.05) inhibits LID-induced AIMs than the LID control group. The reduction in AIMs was seen with kaempferol treatment (25 mg/kg), but it was statistically non-significant. Amantadine at 40 mg/kg dose markedly reduces (p < 0.05) LID-induced AIMs than the vehicle control group. Amelioration of AIMs was more prominent (p < 0.05) in amantadine than kaempferol. No difference in AIMs (limb, axial, orolingual, and total) was observed for hemiparkinsonian and sham rats treated with kaempferol at a dose of 100 mg/kg on the respective days compared to sham group (Figs. 1a–1d).
Figure 1: Behavior alterations of axial (a), limb (b), orolingual (c), and total (d) AIM scores its amelioration by kaempferol. The inset represents data in the Area Under the Curve (AUC) for the respective graph. Data analysis: Two-way ANOVA (post-hoc test: non-parametric Mann-Whitney) and one-way ANOVA (post-hoc test: Tukey’s multiple range). Data reported as mean ± SEM (n = 6). Statistically significant compared with #,&sham rats, *LID control rats, and $one another (amantadine vs. kaempferol). A (Amantadine treated group); AIMs (Abnormal Involuntary Movement Scale); K (Kaempferol treated group); LID (L-dopa Induced Dyskinesia).
Motor coordination and catalepsy bar test
The LID control group had an effective (p < 0.05) decreased fall-off time on the grid test and effectively spent more time on the catalepsy bar than sham rats. Compared to LID control group, kaempferol at a dose of 50 and 100 mg/kg effectively (p < 0.05) inhibited LID-induced alteration in motor coordination and catalepsy bar time spent. Amantadine at 40 mg/kg dose effectively (p < 0.05) inhibited LID-induced reduction in the fall-off time and catalepsy bar time spent than LID control group. Moreover, amantadine more efficiently (p < 0.05) inhibited decreased fall-off time and higher catalepsy bar time spent than kaempferol treatment. The fall-off time and catalepsy bar time spent did not differ in hemiparkinsonian and sham rats treated with kaempferol (100 mg/kg) compared to sham group (Figs. 2a and 2b).
Figure 2: Alterations in fall-off time in grid test (a), time spent on catalepsy bar (b), numbers of steps in FAS (c), and percent intact stepping in FAS (d) its amelioration by kaempferol. Inset represents the area under the curve (AUC) data for a respective graph. Two-way ANOVA (post-hoc test: non-parametric Mann-Whitney) and one-way ANOVA (post-hoc test: Tukey’s multiple range). Data reported as mean ± SEM (n = 6). Statistically significant compared with #,&sham rats, *LID control rats, and $one another (amantadine vs. kaempferol). A (Amantadine treated group); FAS (Forelimb Adjustment Stepping); K (Kaempferol treated group); LID (L-dopa Induced Dyskinesia).
The LID control group rats demonstrated markedly lower (p < 0.05) steps and a lower percentage of intact stepping in forepaw adjusting steps (FAS) than sham group rats. Compared to LID control group, kaempferol at a dose of 50 and 100 mg/kg effectively augmented (p < 0.05) the steps and the percent of intact stepping in FAS. Amantadine at 40 mg/kg also augmented the steps and the percent of intact stepping in FAS more effectively (p < 0.05) vs. LID control group. The number of steps and percent intact stepping did not differ effectively in hemiparkinsonian and sham rats administered with kaempferol (100 mg/kg) vs. sham group (Figs. 2c and 2d).
Mitochondrial complex levels in SNr
The LID control and hemiparkinsonian groups had markedly suppressed (p < 0.05) mitochondrial complex (I–IV) levels in SNr than sham rats. Kaempferol at a dose of 50 and 100 mg/kg effectively inhibited LID-induced reduction in mitochondrial complex levels (p < 0.05) in SNr vs. LID control group. Amantadine at 40 mg/kg dose also augmented mitochondrial complex levels effectively (p < 0.05) in SNr vs. LID control group. Amantadine-treated rats had more effective increased (p < 0.05) mitochondrial complex levels in SNr than kaempferol treated group (Table 1).
Brain monoamines and their metabolites
The LID control and hemiparkinsonian groups had effectively decreased (p < 0.05) brain SNr monoamines (5-HT and DA) and elevated levels of DOPAC and 5-HIAA than the sham group. Also, there was an effective increase (p < 0.05) in DA and 5-HT turnover in the LID control group and the hemiparkinsonian group vs. sham group. Kaempferol at a dose of 50 and 100 mg/kg effectively (p < 0.05) elevated brain SNr monoamines, whereas it decreased their metabolites and turnover than LID control group. Amantadine at 40 mg/kg dose also effectively increased (p < 0.05) 5-HT and DA levels, diminished 5-HIAA and DOPAC levels, DA and 5-HT turnover in SNr than the LID control group. No significant differences were noted in brain monoamines and their turnover in the sham rats administered with kaempferol (100 mg/kg) compared to sham group (Table 2).
FosB, cFOS, Pdyn, and Parkin in SNr
The LID control and hemiparkinsonian groups had effectively (p < 0.05) up-regulated cFOS, FosB, Pdyn and Parkin mRNA expressions in SNr than the sham group. Kaempferol at a dose of 50 and 100 mg/kg markedly (p < 0.05) decreased cFOS, FosB, Parkin, and Pdyn mRNA expressions than LID control group. Amantadine at 40 mg/kg dose markedly (p < 0.05) reduced LID-induced increased FosB, cFOS, Pdyn and Parkin mRNA expressions in SNr than LID control group. No significant differences were noted for these mRNA expressions of FosB, cFOS, Pdyn and Parkin in SNr of sham rats administered with kaempferol (100 mg/kg) compared to sham group (Figs. 3a–3d).
Figure 3: Alterations in brain SNr mRNA expression of cFOS (a), FosB (b), Parkin (c), and Pdyn (d) its amelioration by kaempferol. One-way ANOVA (post-hoc test: Tukey’s multiple range). Data reported as mean ± SEM (n = 6). Statistically significant compared with #,&sham rats, *LID control rats, and $one another (amantadine vs. kaempferol). Ladder 1000 bp (Lane 1); mRNA expression of sham rats; hemiparkinsonian rats; sham + K (100, kaempferol [100 mg/kg, p.o.]); LID control rats; LID + A (40, amantadine [40 mg/kg, p.o.]); LID + K (25, kaempferol [25 mg/kg, p.o.]); LID + K (50, kaempferol [50 mg/kg, p.o.]) and LID + K (100, kaempferol [100 mg/kg, p.o.]) treated rats (Lanes 2–9). A (Amantadine treated group); K (Kaempferol treated group); LID (L-dopa Induced Dyskinesia); Pdyn (Prodynorphin).
There was a positive correlation between mRNA expressions of cFOS (R2 = 0.5927, Fig. 4a), FosB (R2 = 0.7272, Fig. 4b), Parkin (R2 = 0.6895, Fig. 4c), Pdyn (R2 = 0.7867, Fig. 4d), and total AIMs score.
Figure 4: A simple regression of SNr’s cFOS (a), FosB (b), Parkin (c), and Pdyn (d) on the cumulative AIMs score in L-dopa treated rats. Correlation coefficients were determined using a two-sided Fisher test.
Apoptosis, TH, and p-JNK in SNr
Marked (p < 0.05) elevation in apoptosis (cleaved caspase-3, caspase-3 and Bax), p-JNK protein expressions, and a decrease in Bcl-2 and TH protein expressions in SNr were noted in the LID control group, and hemiparkinsonian group than sham group. Kaempferol effectively (p < 0.05) attenuated LID-induced increase (p < 0.05) apoptotic and p-JNK protein expressions and decreased protein expressions of Bcl-2 and TH in SNr than LID control group. Amantadine efficiently (p < 0.05) decreased apoptotic and p-JNK protein expressions and increased protein expressions of Bcl-2 and TH in SNr than in the LID control group. No significant differences were noted in cleaved caspase-3, caspase-3 and Bax, Bcl-2, TH, and p-JNK protein expressions in SNr of sham rats administered with kaempferol (100 mg/kg) compared to sham group (Figs. 5a–5g).
Figure 5: Alterations in brain SNr Bax, Bcl-2, Caspase-3, cleaved Caspase-3, TH and p-JNK protein expressions of rats and its amelioration by kaempferol (a), quantitative representation of the protein expression of Bax (b), Bcl-2 (c), Caspase-3 (d), cleaved Caspase-3 (e) TH (f) and p-JNK (g). One-way ANOVA (post-hoc test: Tukey’s multiple range). Data reported as mean ± SEM (n = 6). Statistically significant compared with #,&sham rats, *LID control rats, and $one another (amantadine vs. kaempferol). Lanes 1–8: protein expression of sham rats; hemiparkinsonian rats; sham + K (100, kaempferol [100 mg/kg, p.o.]); LID control rats; LID + A (40, amantadine [40 mg/kg, p.o.]); LID + K (25, kaempferol [25 mg/kg, p.o.]); LID + K (50, kaempferol [50 mg/kg, p.o.]) and LID + K (100, kaempferol [100 mg/kg, p.o.]) treated rats. A (Amantadine treated group); Bax (Bcl-2-associated X); Bcl-2 (B-cell lymphoma 2); JNK (c-Jun N-terminal Kinase); K (Kaempferol treated group); LID (L-dopa Induced Dyskinesia); TH (Tyrosine hydroxylase); 5-HIAA (5-Hydroxyindoleacetic acid); 5-HT (5-hydroxytryptamine).
A positive correlation was established between apoptosis (Bax [R2 = 0.7318, Fig. 6a], Bcl2 [R2 = 0.5942, Fig. 6b]), Bax/Bcl2 [R2 = 0.5859, Fig. 6c]), caspase-3 [R2 = 0.7241, Fig. 6d], TH (R2 = 0.6036, Fig. 6e), p-JNK (R2 = 0.7894, Fig. 6f), and total AIMs score.
Figure 6: A simple regression of SNr Bax (a), Bcl-2 (b), Bax: Bcl-2 (c), cleaved Caspase-3 (d) TH (e), and p-JNK (f) on the cumulative AIMs score in L-dopa treated rats. Correlation coefficients were determined using a two-sided Fisher test.
Figs. 7a and 7b illustrated the normal histological structure of SNr neurons from the sham rats and sham rats treated with kaempferol (100 mg/kg), whereas SNr section from LID control rats showed neuronal degeneration, nuclear pyknosis, and necrosis (Fig. 7c). SNr sections from amantadine-treated rats showed reduced striatal neuronal degeneration and necrosis, with few cells showing pyknotic nuclei (Fig. 7d). SNr section from kaempferol (50 and 100 mg/kg) administered rats showed the presence of mild to moderate neuronal degeneration, nuclear pyknosis, and necrosis (Figs. 7e and 7f).
Figure 7: Alteration in SNr histopathology in LID rats. Photomicrograph of sections of SNr tissue from sham (a), sham + K (100, kaempferol [100 mg/kg, p.o.]) treated rats (b), LID control rats (c), LID + A (40, amantadine [40 mg/kg, p.o.]) treated rats (d), LID + K (50, kaempferol [50 mg/kg, p.o.]) treated rats (e), and LID + K (100, kaempferol [100 mg/kg, p.o.]) (f) (H&E stain; 40 X). Neuronal degeneration (black arrow), necrosis (blue arrow), and pyknosis (yellow arrow).
Parkinson’s disease is a chronic, progressive, motor deficit incapacitating situation that occurs due to a lack of availability of DA; thus, L-Dopa is vital therapeutic regimen in managing PD [4]. However, chronic L-Dopa administration caused rapid elevation of DA levels followed by uncontrolled DA receptor stimulation, resulting in L-Dopa-induced dyskinesia (LID) [23]. The current investigation determine efficacy of kaempferol on LID-induced PD in dyskinetic rats. The AIMs scale is implemented for dystonic-like orolingual, forelimb, and axial movements [15]. The L-dopa-induced AIMs have previously been reported to alter the activities of postsynaptic neurons caused by both L-dopa and denervation to DA [15]. Additionally, repetitive L-dopa administration caused up-regulated prodynorphin (PDyn) and pre-proenkephalin mRNA expression in the striatum [24]. In this investigation, the administration of kaempferol effectively reduced PDyn and Parkin mRNA expression levels, which in turn ameliorated AIMs. Furthermore, correlation analysis also suggested a positive correlation between PDyn, Parkin, and AIMs. Previous researchers also documented the efficacy of flavonoids against LID via downregulation of PDyn and Parkin [15]. The results of current study align with previous researcher results [15].
Studies have well-documented altered FosB expression in animals with dyskinesia [2,25]. Additionally, recent advances in biochemical evidence have strengthened the role of 5-HT in PD. Munoz et al. reported that combined 5-HT (1A and 1B) receptor activators significantly decreased FosB-positive cells [25]. Furthermore, this researcher documented a linking of FosB-positive cells and AIMs score [25]. Similarly, up-regulated expression of cFos in dyskinetic rats has been well correlated with the severity of AIMS [15]. Findings from the present investigation suggested kaempferol treatment effectively decreases FosB and cFos mRNA expression, which further contributes to the amelioration of AIMs, and these results align with the results of previous researchers [15]. These findings were further supported by correlation analysis where cFos and FosB showed a positive correlation with AIMs, suggesting the vital role of kaempferol in ameliorating AIMs.
It has been reported that anpirtoline, a 5-HT (1A and 1B) receptor activator, significantly ameliorates LID [26]. These findings suggested 5-HT (1A and 1B) receptors activator induced a decrease in 5-HT neuronal activity, which might be the key to the reported anti-dyskinetic potential of anpirtoline [26]. Therefore, 5-HT (1A and 1B) receptor agonists are a potent treatment option for PID due to their selective effect on the 5-HT (1A and 1B) receptors without decreasing the release of other DA neurons or 5-HT levels [27]. Furthermore, several studies have documented that LID might result from the unregulated DA release as a false neurotransmitter from SNr serotonin terminals [27]. Additionally, 5-HT and DA turnover is an important factor during LID as increased DA turnover followed by elevated DOPAC levels were reported in patients with LID [28]. The alteration in the level of monoamine results in the induction of catalepsy measured using the bar test. The previous researchers showed that 5-HT1A receptor agonists ameliorated the degree of catalepsy during LID in 6-OHDA-Lesioned rats [29]. In the present study, kaempferol attenuated LID without affecting 5-HT and dopamine release. This observation might be due to a possible activity of kaempferol on 5-HT (1A and 1B) receptors confirmed by decreased DA turnover and DOPAC levels. Moreover, increased 5-HT levels in kaempferol also contributed to its anti-cataleptic potential during the catalepsy bar test.
In this study, the L-dopa-treated group had lowered mitochondrial activities, suggesting a potential detrimental effect of L-dopa on mitochondrial activities and neuromotor dysfunction. Previous studies also described the role of a mitochondrial complex during the induction and maintenance of Parkinsonism [30]. Administration of kaempferol alleviated L-dopa-induced alterations in the mitochondrial complex, depicting its ameliorative potential against mitochondrial complex activities. Researchers reported a neuroprotective effect of flavonoid quercetin against neurotoxicity-induced neuromotor alterations [31]. Findings from this study also depicted the potential of kaempferol during neuromotor coordination, suggesting its important role in alleviating LID-induced neuromotor deficits.
The study suggested that apoptotic proteins, including Bax and Bcl-2, play vital regulatory role in the induction of PD [20]. Increased Bax/Bcl-2 ratio in PD suggested an elevated apoptotic rate due to increased Bax levels [20]. Caspase-3 is another crucial protein in the apoptotic pathway, and its increased level has been noted in LID rats during PD [20]. Furthermore, L-dopa actively participates in the activation of caspase-3 to cleaved caspase-3 [32]. In the present study, decreased apoptosis levels and increased Bcl-2 levels post-kaempferol treatment demonstrated its protective role against LID. The findings of our investigation are consistent with the results of previous researchers reporting that the administration of flavonoid Icariin ameliorated LID due to its anti-apoptotic potential [2,33]. Interestingly, phosphorylated p-JNK is a protein kinase that plays a central dogma role during PD [33]. Studies have shown that JNK activation is increased in PD, and inhibition in the activation of JNK is considered a potential target for PD management [33]. Our study has reported decreased levels of p-JNK in the kaempferol-treated group, further demonstrating its neuroprotective potential. Previously, kaempferol has been reported to exert its protective potential via modulation of JNK and p38-MAPK pathways, and the findings from this study also corroborated the results of earlier investigator [34].
Recently therapeutic moieties of herbal origin, such as Mucuna pruriens and Hyoscyamus niger, have been used in the management of neurodegenerative disorders, including PD [35]. Furthermore, daily intake of dietary flavonoids has been reported to reduce the risk of Parkinson’s disease [36]. A systematic review of experimental studies documented the neuroprotective potential of kaempferol via its anti-inflammatory, antioxidant, and anti-apoptotic properties [37]. Recently, a double-blind, randomized, placebo-controlled study reported the kaempferol safety over a 4-week duration [38]. Thus, kaempferol can be considered for further clinical evaluation in the PD management.
The findings of the current investigation documented the efficacy of kaempferol during LID in an experimental model of PD. The putative mechanism for the neuroprotective efficacy of kaempferol may be due to the amelioration of alterations in cFOS, FosB, Parkin, Pdyn, Tyrosine hydroxylase, and apoptosis in the brain SNr.
Acknowledgement: None.
Funding Statement: The authors received no specific funding for this study.
Author Contributions: Concept: Pei Qin, Miao Liu, and Jianhua Ma; design: Pei Qin and Jianhua Ma; data acquisition: Miao Liu; data analysis: Pei Qin, Xin Wang, and Jianhua Ma; literature search: Pei Qin and Xin Wang; manuscript editing: Pei Qin, Miao Liu, Xin Wang, and Jianhua Ma. All authors reviewed the results and approved the final version of the manuscript.
Availability of Data and Materials: The datasets generated during and/or analyzed during the current study are not publicly available due to confidentiality agreements, supporting data can only be made available to bona fide researchers subject to a non-disclosure agreement.
Ethics Approval: The Chengde Central Hospital Ethical Committee for the welfare and ethics of experimental animals approved the protocol for this study (No. CDCH20230522).
Conflicts of Interest: The authors declare that they have no conflicts of interest to report regarding the present study.
References
1. Simon DK, Tanner CM, Brundin P. Parkinson disease epidemiology, pathology, genetics, and pathophysiology. Clin Geriatr Med. 2020;36(1):1–12. doi:10.1016/j.cger.2019.08.002. [Google Scholar] [PubMed] [CrossRef]
2. Zhang SF, Xie CL, Lin JY, Wang MH, Wang XJ, Liu ZG. Lipoic acid alleviates L‐DOPA‐induced dyskinesia in 6‐OHDA parkinsonian rats via anti‐oxidative stress. Mol Med Rep. 2018;17(1):1118–24. doi:10.3892/mmr.2017.7974. [Google Scholar] [PubMed] [CrossRef]
3. Prasad EM, Hung SY. Behavioral tests in neurotoxin-induced animal models of Parkinson’s disease. Antioxidants. 2020;9(10):1007. doi:10.3390/antiox9101007. [Google Scholar] [PubMed] [CrossRef]
4. Armstrong MJ, Okun MS. Diagnosis and treatment of Parkinson disease: a review. J Am Med Assoc. 2020;323(6):548–60. doi:10.1001/jama.2019.22360. [Google Scholar] [PubMed] [CrossRef]
5. Yang JX, Chen L. Economic burden analysis of Parkinson’s disease patients in China. Parkinsons Dis. 2017;2017(3):8762939. doi:10.1155/2017/8762939. [Google Scholar] [PubMed] [CrossRef]
6. Martinez-Carrasco A, Real R, Lawton M, Iwaki H, Tan MMX, Wu L, et al. Genetic meta-analysis of levodopa induced dyskinesia in Parkinson’s disease. NPJ Parkinsons Dis. 2023;9(1):128. doi:10.1038/s41531-023-00573-2. [Google Scholar] [PubMed] [CrossRef]
7. de Andrade Teles RB, Diniz TC, Costa Pinto TC, de Oliveira Junior RG, Gama ESM, de Lavor EM, et al. Flavonoids as therapeutic agents in Alzheimer’s and Parkinson’s diseases: a systematic review of preclinical evidences. Oxid Med Cell Longev. 2018;2018:7043213. doi:10.1155/2018/7043213. [Google Scholar] [PubMed] [CrossRef]
8. Madiha S, Batool Z, Tabassum S, Liaquat L, Sadir S, Shahzad S, et al. Quercetin exhibits potent antioxidant activity, restores motor and non-motor deficits induced by rotenone toxicity. PLoS One. 2021;16(11):e0258928. doi:10.1371/journal.pone.0258928. [Google Scholar] [PubMed] [CrossRef]
9. Ayaz M, Sadiq A, Junaid M, Ullah F, Ovais M, Ullah I, et al. Flavonoids as prospective neuroprotectants and their therapeutic propensity in aging associated neurological disorders. Front Aging Neurosci. 2019;11:155. doi:10.3389/fnagi.2019.00155. [Google Scholar] [PubMed] [CrossRef]
10. Sloley BD, Urichuk LJ, Morley P, Durkin J, Shan JJ, Pang PK, et al. Identification of kaempferol as a monoamine oxidase inhibitor and potential neuroprotectant in extracts of Ginkgo biloba leaves. J Pharm Pharmacol. 2000;52(4):451–9. doi:10.1211/0022357001774075. [Google Scholar] [PubMed] [CrossRef]
11. Herraiz T, Flores A, Fernandez L. Analysis of monoamine oxidase (MAO) enzymatic activity by high-performance liquid chromatography-diode array detection combined with an assay of oxidation with a peroxidase and its application to MAO inhibitors from foods and plants. J Chromatogr B Biomed Appl. 2018;1073:136–44. doi:10.1016/j.jchromb.2017.12.004. [Google Scholar] [PubMed] [CrossRef]
12. Feng Z, Wang C, Jin Y, Meng Q, Wu J, Sun H. Kaempferol-induced GPER upregulation attenuates atherosclerosis via the PI3K/AKT/Nrf2 pathway. Pharm Biol. 2021;59(1):1106–16. doi:10.1080/13880209.2021.1961823. [Google Scholar] [PubMed] [CrossRef]
13. Rahul NF, Jyoti S, Siddique YH. Effect of kaempferol on the transgenic Drosophila model of Parkinson’s disease. Sci Rep. 2020;10(1):13793. doi:10.1038/s41598-020-70236-2. [Google Scholar] [PubMed] [CrossRef]
14. Mahfudin U, Subarnas A, Wilar G, Hermanto F. Potential activity of kaempferol as anti-Parkinson’s; molecular docking and pharmacophore modelling study. Int J Appl Pharm. 2023;15(3):43–8. doi:10.22159/ijap.2023v15i3.47355. [Google Scholar] [CrossRef]
15. Cao W, Liang S, Yang Y, Zhu C, Sun L, Zhang L. Fisetin ameliorates levodopa-induced dyskinesia in experimental model Parkinson’s disease: role of mitochondrial activities and monoamines turnover. Nat Prod Commun. 2022;17(11):1934578X221136674. doi:10.1177/1934578x221136674. [Google Scholar] [CrossRef]
16. Bayo-Olugbami AA, Nafiu AB, Amin A, Ogundele OM, Lee CC, Owoyele BV. Amelioration of L-DOPA-induced dyskinesia with vitamin D3 in Parkinsonian mice model. bioRxiv. 2021;2012:920953. doi:10.1101/2021.09.13.459937. [Google Scholar] [CrossRef]
17. Pan X, Liu X, Zhao H, Wu B, Liu G. Antioxidant, anti-inflammatory and neuroprotective effect of kaempferol on rotenone-induced Parkinson’s disease model of rats and SH-S5Y5 cells by preventing loss of tyrosine hydroxylase. J Funct Foods. 2020;74(9):104140. doi:10.1016/j.jff.2020.104140. [Google Scholar] [CrossRef]
18. Li S, Pu XP. Neuroprotective effect of kaempferol against a 1-methyl-4-phenyl-1,2,3,6-tetrahydropyridine-induced mouse model of Parkinson’s disease. Biol Pharm Bull. 2011;34(8):1291–6. doi:10.1248/bpb.34.1291. [Google Scholar] [PubMed] [CrossRef]
19. Zheng CQ, Fan HX, Li XX, Li JJ, Sheng S, Zhang F. Resveratrol alleviates levodopa-induced dyskinesia in rats. Front Immunol. 2021;12:683577. doi:10.3389/fimmu.2021.683577. [Google Scholar] [PubMed] [CrossRef]
20. Kishore Kumar SN, Deepthy J, Saraswathi U, Thangarajeswari M, Yogesh Kanna S, Ezhil P, et al. Morinda citrifolia mitigates rotenone-induced striatal neuronal loss in male Sprague-Dawley rats by preventing mitochondrial pathway of intrinsic apoptosis. Redox Rep. 2017;22(6):418–29. doi:10.1080/13510002.2016.1253449. [Google Scholar] [PubMed] [CrossRef]
21. Yao X, Li L, Kandhare AD, Mukherjee-Kandhare AA, Bodhankar SL. Attenuation of reserpine-induced fibromyalgia via ROS and serotonergic pathway modulation by fisetin, a plant flavonoid polyphenol. Exp Ther Med. 2020;19(2):1343–55. doi:10.3892/etm.2019.8328. [Google Scholar] [PubMed] [CrossRef]
22. Charan J, Kantharia ND. How to calculate sample size in animal studies? J Pharmacol Pharmacother. 2013;4(4):303–6. doi:10.4103/0976-500X.119726. [Google Scholar] [PubMed] [CrossRef]
23. Warren Olanow C, Kieburtz K, Rascol O, Poewe W, Schapira AH, Emre M, et al. Factors predictive of the development of Levodopa-induced dyskinesia and wearing-off in Parkinson’s disease. Mov Disord. 2013;28(8):1064–71. doi:10.1002/mds.25364. [Google Scholar] [PubMed] [CrossRef]
24. Hulme H, Fridjonsdottir E, Vallianatou T, Shariatgorji R, Nilsson A, Li Q, et al. Basal ganglia neuropeptides show abnormal processing associated with L-DOPA-induced dyskinesia. NPJ Parkinsons Dis. 2022;8(1):41. doi:10.1038/s41531-022-00299-7. [Google Scholar] [PubMed] [CrossRef]
25. Munoz A, Li Q, Gardoni F, Marcello E, Qin C, Carlsson T, et al. Combined 5-HT1A and 5-HT1B receptor agonists for the treatment of L-DOPA-induced dyskinesia. Brain. 2008;131:3380–94. doi:10.1093/brain/awn235. [Google Scholar] [PubMed] [CrossRef]
26. Ślifirski G, Król M, Turło J. 5-HT receptors and the development of new antidepressants. Int J Mol Sci. 2021;22(16):9015. doi:10.3390/ijms22169015. [Google Scholar] [PubMed] [CrossRef]
27. Farajdokht F, Sadigh-Eteghad S, Majdi A, Pashazadeh F, Vatandoust SM, Ziaee M, et al. Serotonergic system modulation holds promise for L-DOPA-induced dyskinesias in hemiparkinsonian rats: a systematic review. EXCLI J. 2020;19:268–95. doi:10.17179/excli2020-1024. [Google Scholar] [PubMed] [CrossRef]
28. Scheffer DDL, Freitas FC, AguiarJr AS, Ward C, Guglielmo LGA, Prediger RD, et al. Impaired dopamine metabolism is linked to fatigability in mice and fatigue in Parkinson’s disease patients. Brain Commun. 2021;3(3):fcab116. doi:10.1093/braincomms/fcab116. [Google Scholar] [PubMed] [CrossRef]
29. Fisher R, Hikima A, Morris R, Jackson MJ, Rose S, Varney MA, et al. The selective 5-HT1A receptor agonist, NLX-112, exerts anti-dyskinetic and anti-parkinsonian-like effects in MPTP-treated marmosets. Neuropharmacol. 2020;167(3):107997. doi:10.1016/j.neuropharm.2020.107997. [Google Scholar] [PubMed] [CrossRef]
30. Subrahmanian N, LaVoie MJ. Is there a special relationship between complex I activity and nigral neuronal loss in Parkinson’s disease? A critical reappraisal. Brain Res. 2021;1767:147434. doi:10.1016/j.brainres.2021.147434. [Google Scholar] [PubMed] [CrossRef]
31. Wróbel-Biedrawa D, Grabowska K, Galanty A, Sobolewska D, Podolak I. A flavonoid on the brain: quercetin as a potential therapeutic agent in central nervous system disorders. Life. 2022;12(4). doi:10.3390/life12040591. [Google Scholar] [PubMed] [CrossRef]
32. Lai CY, Lin CY, Wu CR, Tsai CH, Tsai CW. Carnosic acid alleviates levodopa-induced dyskinesia and cell death in 6-hydroxydopamine-lesioned rats and in SH-SY5Y cells. Front Pharmacol. 2021;12:703894. doi:10.3389/fphar.2021.703894. [Google Scholar] [PubMed] [CrossRef]
33. Lu DS, Chen C, Zheng YX, Li DD, Wang GQ, Liu J, et al. Combination treatment of icariin and L-DOPA against 6-OHDA-lesioned dopamine neurotoxicity. Front Mol Neurosci. 2018;11:155. doi:10.3389/fnmol.2018.00155. [Google Scholar] [PubMed] [CrossRef]
34. Xie B, Zeng Z, Liao S, Zhou C, Wu L, Xu D. Kaempferol ameliorates the inhibitory activity of dexamethasone in the osteogenesis of MC3T3-E1 cells by JNK and p38-MAPK pathways. Front Pharmacol. 2021;12:739326. doi:10.3389/fphar.2021.739326. [Google Scholar] [PubMed] [CrossRef]
35. Rahman MM, Wang X, Islam MR, Akash S, Supti FA, Mitu MI, et al. Multifunctional role of natural products for the treatment of Parkinson’s disease: at a glance. Front Pharmacol. 2022;13:976385. doi:10.3389/fphar.2022.976385. [Google Scholar] [PubMed] [CrossRef]
36. Gao X, Cassidy A, Schwarzschild MA, Rimm EB, Ascherio A. Habitual intake of dietary flavonoids and risk of Parkinson disease. Neurology. 2012;78(15):1138–45. doi:10.1212/WNL.0b013e31824f7fc4. [Google Scholar] [PubMed] [CrossRef]
37. Dong X, Zhou S, Nao J. Kaempferol as a therapeutic agent in Alzheimer’s disease: evidence from preclinical studies. Ageing Res Rev. 2023;87:101910. doi:10.1016/j.arr.2023.101910. [Google Scholar] [PubMed] [CrossRef]
38. Akiyama M, Mizokami T, Ito H, Ikeda Y. A randomized, placebo-controlled trial evaluating the safety of excessive administration of kaempferol aglycone. Food Sci Nutr. 2023;11(9):5427–37. doi:10.1002/fsn3.3499. [Google Scholar] [PubMed] [CrossRef]
Cite This Article
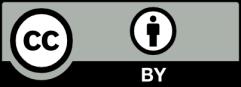