Open Access
ARTICLE
Immune checkpoint receptors and their ligands on CD8 T cells and myeloma cells in extramedullary multiple myeloma
1 Department of Hematology, Third Hospital of Hebei Medical University, Shijiazhuang, 050051, China
2 Department of Bone and Soft Tissue Oncology, Third Hospital of Hebei Medical University, Shijiazhuang, 050051, China
* Corresponding Authors: GUOCHUAN ZHANG. Email: ; LIXIA SUN. Email:
# Lead contact
BIOCELL 2024, 48(2), 303-311. https://doi.org/10.32604/biocell.2023.046640
Received 09 October 2023; Accepted 05 December 2023; Issue published 23 February 2024
Abstract
Background: Prognosis of multiple myeloma (MM) patients with extramedullary disease (EMD) remains poor. T cell dysfunction and an immunosuppressive environment have been reported in the bone marrow (BM) of MM patients. However, the immunosuppressive microenvironment and immune checkpoint receptors (ICRs) on CD8 T cells in the EMD tissue of newly diagnosed MM (NDMM) patients have not been thoroughly studied. Methods: We investigated the expression levels of T cell immunoglobulin mucin-domain-containing-3 (TIM-3) and T-cell immunoglobulin and ITIM domain (TIGIT) on CD8 T cells and the expression of their ligands (Galectin-9 and CD155) on myeloma cells in EMD tissue of NDMM patients. The expression levels of TIM-3 and TIGIT on T cells and their ligands (Galectin-9 and CD155) on myeloma cells were analyzed by multicolored fluorescent flow cytometry in the peripheral blood (PB), BM, and EMD tissue. Additionally, the expression of ICRs was compared among PB, BM, and EMD tissue. Results: The expression levels of TIGIT and TIM-3 on CD8 T cell subsets, including CD8 Naïve T (T) cells and CD8 terminally differentiated effector memory T (T) cells, were significantly higher in EMD than in PB ( < 0.05 for all). The expression levels of TIGIT and TIM-3 on CD8 T cells were higher in EMD than in BM ( < 0.05 for all). There were no differences in Galectin-9 and CD155 expression on myeloma cells among PB, BM, and EMD tissue. Conclusion: Our data add new evidence that T cells express increased TIGIT and TIM-3, suggesting that there may be T-cell exhaustion in EMD of patients with MM.Graphic Abstract
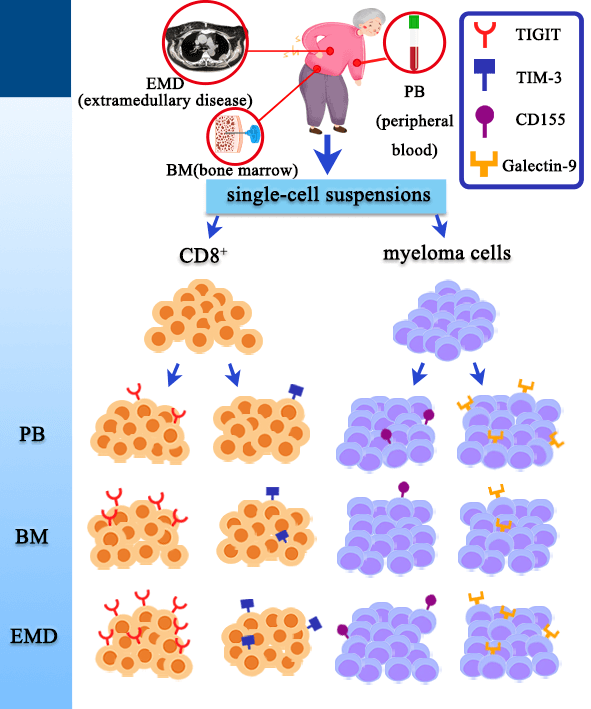
Keywords
Supplementary Material
Supplementary Material FileMultiple myeloma (MM) is a hematological malignancy featured by localized and unlimited reproduction of malignant plasma cells in the bone marrow (BM) [1]. In some cases, myeloma cells may become more invasive, resistant to treatment, and able to directly penetrate bone cortex or metastasize to distant tissues or organs through the circulation, resulting in the formation of soft tissue masses known as extramedullary disease (EMD) [2]. Despite advances in treatment, including new drugs and autologous hematopoietic stem-cell transplantation, the prognosis in patients with EMD remains poor [3,4]. However, the exact pathogenesis of EMD remains unclear [2].
The simultaneous presence of growing tumors and T cells implies that CD8+ T cells with tumor reactivity gradually experience a decline in functionality throughout tumorigenesis [5]. Cancer immunology and immunotherapy have emphasized the significant role of CD8+ T cells and the tumor microenvironment, supporting the crucial role of tumor-specific CD8+ T cells in antitumor immune responses [6].
Many studies [7–9] have demonstrated a highly immunosuppressive BM environment and T cell dysfunction in patients with MM. T-cell exhaustion, resulting from expression or coexpression of multiple immune checkpoint receptors (ICRs) on T cells, such as programmed cell death receptor-1 (PD-1), T-cell immunoglobulin mucin-domain-containing-3 (TIM-3), and T cell immunoglobulin and ITIM domain (TIGIT), leads to T cell functional immunosuppression and promotes immune escape of MM cells [10–12]. Both murine and human studies [13,14] on MM have indicated that TIGIT is more frequently expressed in CD8+ T cells during myeloma progression, which is associated with compromised effector functions of these T cells. Zelle-Rieser et al. [15] discovered that CD8+ T cells derived from the tumor site (BM) of myeloma patients expressed multiple molecules related to T-cell exhaustion, such as PD-1, cytotoxic T-lymphocyte-associated protein (CTLA-4), CD244. These cells were unable to produce interferon-γ following in vitro restimulation with CD3/CD28 and exhibited a diminished capacity to degranulate in response to T cell stimuli. All of these studies focused on CD8+ T cells and ICRs within myeloma microenvironment. However, anti–PD-1 monotherapy has shown limited benefit in MM patients. Furthermore, clinical trials of PD-1 inhibitors plus immunomodulators were stopped due to an imbalance in the proportion of the death group, indicating that this ICR may not be the major mediator of failing anti-MM immunity [16]. In previous studies [17,18], the effectiveness of CTLA-4 blockade or lymphocyte-activated gene 3 (LAG-3) blockade in patients with MM has not been demonstrated. For example, in a phase 1b study [18], Armand et al. reported that seven MM patients were treated with nivolumab plus ipilimumab, but none of these cases showed an objective response. Thus, it is important to investigate new applicable ICR inhibitors and therapeutic strategies for overcoming immune suppression in MM.
A few studies to date have reported the expression of ICRs in EMD. Crescenzi et al. [19] performed immunohistochemical staining for PD1 and programmed death ligand-1 (PD-Ll) in 12 EMD patients. They found PD-L1 expression in 4/12 cases of extraosseous myeloma and the presence of PD1+ infiltrating lymphocytes in all examined cases. Although the expression of PD1/PD-L1 was compared between EMD tissue and BM, there has been no comparison of the expression levels or expression patterns between different T cell subpopulations, and the expression of other ICRs and their ligands has not been examined. Lee et al. [20] examined expression of Galectin-9 in four extramedullary plasmacytoma tissues located in the skull, neck node, chest wall, and scalp using immunofluorescence assays. They found that none of the samples expressed Galectin-9, and indicated that the lack of Galectin-9 expression might be associated with EMD; however, they did not compare the expression levels of Galectin-9 between EMD tissue and BM, and they did not detect its ligand TIM-3. Thus far, there has been no examination of the Galectin-9 and TIM-3 expression in EMD tissue or analysis of the differences in the expression levels between EMD tissue, BM, and peripheral blood (PB).
Available studies suggest that ICRs might be involved in the pathogenesis of EMD and that their expression might differ among PB, BM, and EMD. Nevertheless, expression of multiple ICRs in the EMD microenvironment has not been fully elucidated. Considering the major role of CD8+ T cell dysfunction in patients with MM and limited benefit of PD-1 inhibitors in MM, here we investigated the expression of TIM-3 and TIGIT on CD8+ T cells and the expression of their ligands (Galectin-9 and CD155) on myeloma cells in the EMD tissue of newly diagnosed MM (NDMM) patients. Additionally, we analyzed differences in the expression if ICRs among PB, BM, and EMD tissue to reveal molecules associated with EMD pathogenesis.
Patients and sample collection
A total of nineteen NDMM patients were enrolled in this study, including eleven men and eight women, with a median age of 62 years (range, 36–72 years). They were diagnosed in accordance with the International Myeloma Working Group (IMWG) guidelines [21]. Among them, nine NDMM patients had EMD, including seven men and two women, with a median age of 63 years (range, 36–71 years). EMD was defined as the presence of plasma-cell tumors outside the BM, either in the form of soft tissue mass arising from bone lesions or not contiguous with bone lesions [2]. Diagnosis of EMD was confirmed by computed tomography, magnetic resonance imaging, or positron emission tomography/computed tomography scans, and biopsies were carried out whenever possible. We collected BM aspirates and paired PB samples from all 19 NDMM patients, and we obtained EMD tissue samples (n = 6) by surgery or puncture from nine NDMM patients with EMD. Furthermore, we collected PB samples from 10 age- and sex-matched healthy donors (HD) (four men and six women; median age: 61 years [range, 43–79 years]) and BM samples from 10 age- and sex-matched healthy donors (six men and four women; median age: 54 years [range, 39–77 years]) as controls. The study was conducted in accordance with the Declaration of Helsinki. All of the subjects provided informed consent, and ethical approval was obtained from the Ethics Committee of the Third Affiliated Hospital of Hebei Medical University.
Fresh EMD tissues were collected on the day of surgery or puncture. The tissues were first manually minced into smaller pieces, which were then transferred to a 300-μm steel mesh. Single-cell suspensions were obtained by mechanical grinding and continual washing with cell staining buffer. The prepared cell suspension was then filtered through a 100-μm filter screen, followed by centrifugation at 1000 g for 5 min. After complete aspiration of the supernatant, the tumor cell suspensions were resuspended in cell staining buffer and centrifuged at 1000 g for 5 min. The supernatant was discarded, and the tumor cells were kept for flow cytometry analysis.
Extracellular staining was performed in accordance with manufacturer’s instructions. Briefly, the single-cell suspensions prepared from EMD tissue, BM, and PB were washed with phosphate-buffered saline (PBS) and centrifuged at 1200 g for 5 min. The supernatant was discarded, and this process was repeated twice. Lymphoid cells were stained for surface markers using anti–CD3-PerCP, anti–CD8-PE-Cy7, anti–CD45RA-PE, anti–CD197-APC-Cy7, anti–TIGIT-APC, and anti–TIM-3-APC, as well as relevant isotype controls. Plasma cells were first stained with surface markers, including anti–CD155-FITC, anti–CD45-PerCP, and anti–CD38-APC, in the dark at 4°C for 30 min. Then, plasma cells were stained with intracellular markers, including anti–Galectin-9-FITC, anti–Lambda-APC-Cy7, and anti–Kappa-PE-Cy7. Briefly, the cells were fixed in 0.5 mL/tube Fixation Buffer in the dark for 20 min at room temperature. Next, the fixed cells were washed three times with 1× Intracellular Staining Perm Wash Buffer and centrifuged at 400 g for 5 min. Finally, the cells were incubated with anti–Galectin-9-FITC, anti–Lambda-APC-Cy7, and anti–Kappa-PE-Cy7 antibodies and washed twice with 1× Intracellular Staining Perm Wash Buffer before being resuspended in 0.5 mL staining buffer. The BD FACS Canto II flow cytometer was used to collect the cells and Flowjo software (Flowjo LLC, USA) was used to analyze the expression of immune checkpoint molecules. FSC-A/FSC-H gating was used to wipe off the dead and sticky cells.
We analyzed TIGIT and TIM-3 expression levels on CD3+ T and CD3+ CD8+ T cell differentiation subsets. T cell differentiation subsets were defined as follows: terminally differentiated effector memory T cells (TEMRA, CD8+ and CD45RA+CCR7−), Naïve T cells (TN, CD8+ and CD45RA+CCR7+), central memory T cells (TCM, CD8+ and CD45RA−CCR7+), effector memory T cells (TEM, CD8+ and CD45RA−CCR7−) [22,23]. We also analyzed the expression of Galectin-9 and CD155 in normal plasma cells (CD38+CD45+Kappa+Lambda+) and MM cells (CD38+ CD45−/+Kappa+Lambda− or CD38+CD45−/+Kappa− Lambda+). The antibodies used in this study were purchased from Biolegend (San Diego, CA, USA), and their essential information is shown in Suppl. Table S1.
SPSS software was used for statistical analyses. Shapiro-Wilk test was used to assess the normality of each variable. Student’s t test or one-way analysis was used to analyze the normal distribution data. Mann-Whitney U test or Kruska-Wallis was used to analyze the nonnormal distribution data. Bonferroni’s test was used for all post-hoc analyses. All data are presented as the median (Q1–Q3). p-value < 0.05 was regarded as statistical significance statistically significant (ns: not significant).
Differences in percentages of CD8+ T cell subsets and MM cells among PB, BM, and EMD tissue of NDMM patients
The gating strategies for dividing subpopulations of CD8+ T cells using flow cytometry is depicted in Fig. 1A. The differences in CD8+ T cell subsets of PB, BM, and EMD tissue of NDMM patients are listed in Suppl. Table S2. Percentages of CD3+ T cells in patients with NDMM were significantly lower in EMD than in PB (1.16% vs. 16.88%, p < 0.001) and BM (1.16% vs. 8.74%, p = 0.023). Percentages of CD8+ TCM cells among CD8+ T cells were higher in EMD than in PB (3.75% vs. 1.00%, p = 0.002) and BM (3.75% vs. 1.07%, p = 0.006). Percentages of MM cells were significantly higher in EMD than in PB (49.24% vs. 0.18%, p < 0.001) and BM (49.24% vs. 7.40%, p = 0.044). These results indicate that compared with PB and BM, EMD from patients with NDMM contains more CD8+ TCM and myeloma cells but fewer CD3+ T cells.
Figure 1: The gating strategies for the immunophenotyping analyses of CD8+ T cells and myeloma plasma cells by flow cytometry from a patient with MM. (A) The analytic logic of flow cytometry detection of TIGIT expression in CD8+ T cells and the subpopulations of CD8+ T cells. (B) The analytic logic of flow cytometry detection of Galectin-9 expression in myeloma plasma cells.
Differences in TIGIT expression on CD8+ T cells and CD155 expression on myeloma cells among PB, BM, and EMD tissue of NDMM patients
The gating strategies for the immunophenotyping analyses of CD8+ T cells and myeloma plasma cells by flow cytometry from a patient with MM is depicted in Figs. 1A and 1B. The percentages and mean fluorescence intensity of differences in TIGIT expression on CD8+ T cells and CD155 expression on plasma cells in PB and BM between HD and NDMM patients are listed in Suppl. Tables S3–6.
Fig. 2 shows a representative chart of TIGIT expression on CD8+ T cells in PB, BM, and EMD tissue of NDMM patients. Compared with PB (n = 19), the percentages of TIGIT expression on CD3+ T cells, CD8+ T cells, CD8+ TN cells, CD8+ TEM cells, and CD8+ TEMRA cells were higher in EMD (n = 6) of NDMM patients. The percentages of TIGIT expression on CD3+ T cells and CD8+ TN cells were also higher in EMD (n = 6) than in BM (n = 19). There were no statistical differences in CD155 expression on myeloma cells in EMD compared with BM and PB (Fig. 3 and Suppl. Table S7).
Figure 2: Representative graph of TIGIT expression on CD3+ T cells and CD8+ T cells in PB, BM, and EMD of NDMM patients.
Figure 3: Percentage of TIGIT expression on T cell subsets and CD155 expression on myeloma cells in PB (n = 19), BM (n = 19), and EMD (n = 6) of NDMM patients. (A and B) Comparison of the percentage of TIGIT expression on CD3+ and CD8+ T cells in PB, BM, and EMD from NDMM patients. (C–F) Comparison of the percentage of TIGIT expression on CD8+ TEMRA, CD8+ TN, CD8+ TCM, and CD8+ TEM cells in PB, BM, and EMD of NDMM patients. (G) Comparison of the percentage of CD155 on myeloma cells in PB, BM, and EMD of NDMM patients. TEMRA: terminally differentiated effector memory T cells (CD8+ and CD45RA+CCR7−), TN, Naïve T cells (CD8+ and CD45RA+CCR7+), TCM: central memory T cells (CD8+ and CD45RA−CCR7+), TEM: effector memory T cells (CD8+ and CD45RA−CCR7−); MM cells, myeloma cells (CD38+CD45−/+Kappa+Lambda− or CD38+CD45−/+Kappa−Lambda+); PB, peripheral blood; BM, bone marrow; EMD, extramedullary disease; NDMM, newly diagnosed multiple myeloma; MM cells, myeloma cells. A, B, E and F: One-way analysis of variance (ANOVA) test for three-group comparison, with Bonferroni’s test used for post hoc analysis. C, D, and G: Kruskal‒Wallis test for three-group comparison, with Bonferroni's test used for post hoc analysis. All data are shown as the median.
Subgroup analysis showed that compared with PB (n = 9), the percentages of TIGIT expression on CD3+ T cells, CD8+ T cells, CD8+ TN cells, CD8+ TEM cells, and CD8+ TEMRA cells were higher in EMD (n = 6) of NDMM patients with EMD. The percentages of TIGIT expression on CD3+ T cells and CD8+ TN cells were higher in EMD (n = 6) than in BM (n = 9). There were no differences in CD155 expression on myeloma cells in EMD compared with BM and PB (Table 1 and Suppl. Table S8).
Differences in TIM-3 expression on CD8+ T cells and Galectin-9 expression on myeloma cells among PB, BM, and EMD tissue of NDMM patients
The differences in TIM-3 expression on T cells and Galectin-9 expression on plasma cells of PB and BM between HD and NDMM patients are listed in Suppl. Tables S9–12. A representative scatter chart of TIM-3 expression on T cells in PB, BM, and EMD tissue of NDMM patients is demonstrated in Fig. 4. Compared with PB (n = 19), the percentages of TIM-3 expression on CD3+ T cells, CD8+ T cells, CD8+ TN cells, CD8+ TEM cells, and CD8+ TEMRA cells were higher in EMD (n = 6) of NDMM patients. There were no differences in Galectin-9 expression on myeloma cells in EMD compared with BM and PB (Fig. 5 and Suppl. Table S13).
Figure 4: Representative graph of TIM-3 expression on CD3+ T cells and CD8+ T cells in PB, BM, and EMD of NDMM patients.
Figure 5: Percentage of TIM-3 expression on T cell subsets and Galectin-9 expression on myeloma cells in PB (n = 19), BM (n = 19), and EMD (n = 6) of NDMM patients. (A and B): Comparison of the percentage of TIM-3 expression on CD3+ and CD8+ T cells in PB, BM, and EMD of NDMM patients. (C–F): Comparison of the percentage of TIM-3 expression on CD8+ TEMRA, CD8+ TN, CD8+ TCM, and CD8+ TEM cells in PB, BM, and EMD of NDMM patients. (G) Comparison of the percentage of Galectin-9 expression on myeloma cells in PB, BM, and EMD of NDMM patients. TEMRA, terminally differentiated effector memory T cells (CD8+ and CD45RA+CCR7−), TN, Naïve T cells (CD8+ and CD45RA+CCR7+), TCM, central memory T cells (CD8+ and CD45RA−CCR7+); TEM, effector memory T cells (CD8+ and CD45RA−CCR7−); MM cells, myeloma cells (CD38+CD45−/+Kappa+Lambda− or CD38+CD45−/+Kappa−Lambda+); PB, peripheral blood; BM, bone marrow; EMD, extramedullary disease; NDMM, newly diagnosed multiple myeloma; MM cells, myeloma cells. A–G: Kruskal‒Wallis test for three-group comparison, with Bonferroni’s test used for post hoc analysis. All data are shown as the median.
Subgroup analysis showed that compared with PB (n = 9), the percentages of TIM-3 expression on CD3+ T cells, CD8+ T cells, CD8+ TN cells, and CD8+ TEMRA cells were higher in EMD (n = 6) of NDMM patients with EMD. The percentages of TIM-3 expression on CD3+ T cells and CD8+ TN cells were higher in EMD (n = 6) than in BM (n = 9). There were no differences in Galectin-9 expression on myeloma cells among PB, BM, and EMD of NDMM patients with EMD (Table 2 and Suppl. Table S14).
Several studies have confirmed the role of BM exhausted T cells in the pathogenesis of MM [7,9,15]. In the present study, for the first time, we demonstrated higher percentages of myeloma cells and CD8+ TCM cells and a lower percentage of total CD3+ T cells in EMD than in PB and BM of NDMM patients. Importantly, we found higher expression levels of TIGIT and TIM-3 on CD8+ T cell subsets in EMD than in either BM or PB, though there were no differences in CD155 and Galectin-9 expression on myeloma cells in EMD compared with BM and PB. Overall, we provided further evidence for the existence of exhausted T cells in EMD of NDMM patients, although the quantities of total CD3+ T cells in EMD were lower than those in PB and BM.
CD8+ T cells can be classified as CD8+ TN, CD8+ TCM, CD8+ TEM, and CD8+ TEMRA cells. After infiltrating the tumor, Naïve CD8+ T cells undergo differentiation into effector T cells. Then, the effector cells further differentiate and become activated into cytotoxic and memory T cells, which perform their unique functions at the site of the tumor microenvironment [24,25]. In our study, the higher level of CD8+ TCM cells in EMD compared with PB and BM suggests that more CD8+ TCM cells are recruited into EMD to kill myeloma cells in patients with MM.
TIGIT was first identified as a novel member of the CD28 family. TIGIT has the ability to bind two ligands, CD155 and Nectin-2. These ligands are expressed on different cell types such as antigen-presenting cells (APCs), T cells, and various non-hematopoietic cell types, including tumor cells. In addition to its direct effect on T and natural killer (NK) cells, TIGIT indirectly suppresses immune responses by activating CD155 on dendritic cells (DCs). Thus, TIGIT plays a negative regulatory role in antitumor responses [26]. Importantly, TIGIT expression is low in the peripheral lymphoid organs of mice with tumors, but it is highly abundant in tumor tissue. This observation suggests that TIGIT plays an especial role in regulating immune responses within the tumor microenvironment [27]. Guillerey et al. [13] linked high expression of TIGIT on CD8+ T cells to the progression of MM in both mice and humans. Moreover, it has been demonstrated that blocking TIGIT can heighten the effector function of CD8+ T cells in MM patients and effectively inhibit the progression of MM. TIGIT can promote myeloma growth directly through elevated interleukin (IL)-10 expression [28]. Blocking TIGIT delays MM progression and increases the number of interferon (IFN)-γ–producing CD4+ and CD8+ T cells [29]. Frequencies of tumor-infiltrating CD8+ T cells that express IL-2/tumor necrosis factor (TNF)-α/IFN-γ and CD4+ T cells that express IL-2 are significantly increased after anti-TIGIT monoclonal antibody treatment [30]. We found significantly higher expression of TIGIT on T cells in EMD than in PB and BM, but there was no difference in CD155 expression on myeloma cells. The results reported by other researchers and the results of this study suggest the existence of T-cell exhaustion in EMD in view of the TIGIT–CD155 receptor and ligand and their role in the onset of T-cell exhaustion.
TIM-3 is a transmembrane protein that consists of a signal peptide sequence, an immunoglobulin-like V-type domain, a mucin domain, and a cytoplasmic tail. It is selectively expressed on CD4+ T helper 1 and CD8+ T cytotoxic 1 T cells that produce IFN-γ. Many studies have demonstrated that TIM-3 is a pivotal immune checkpoint molecule for tumor-induced immune suppression [31]. We found higher expression of TIM-3 on CD8+ T cells in EMD than in PB, though there was no difference in Galectin-9 expression on myeloma cells. However, Lee et al. [20] examined Galectin-9 expression in four tissues of extramedullary plasmacytoma using immunofluorescence assays and found that none of them expressed Galectin-9. These inconsistencies may be related to the differences in the patients enrolled, detection methods, and EMD tissue types. Overall, the results reported by other researchers and the results of this study provide additional evidence for the existence of T-cell exhaustion in EMD in view of the TIM-3–Galectin-9 receptor and ligand and their role in the onset of T-cell exhaustion.
There are several limitations to our study. First, we did not detect expression of Nectin-2 (another ligand of TIGIT) on myeloma cells. Second, the number of samples was relatively limited, and the relationship with clinical outcome was not investigated. Third, the mechanisms of T-cell exhaustion in the immunosuppressive microenvironment were not explored in the present study. Therefore, further studies are required to clarify these aspects.
In summary, the results of this study add new evidence suggesting that T-cell exhaustion exists in EMD from the points of view of both the TIGIT–CD155 receptor and ligand and the TIM-3–Galectin-9 receptor and ligand. Our results provide preliminary data for further elucidating the underlying mechanisms by which myeloma cells in EMD induce T-cell exhaustion. Furthermore, a study involving a larger population of MM patients with EMD is necessary to elucidate the underlying mechanisms of TIGIT–CD155 and TIM-3–Galectin-9 in the microenvironment of EMD.
Acknowledgement: The authors thank all patients and healthy donors participating in this study. The authors thank Jiang-Chao Liu for his technical support.
Funding Statement: The authors declare that this study was supported by S&T Program of Hebei, China (Grant Number 21377754D) and the 2021 Hebei Provincial Government-funded Clinical Medicine Talents Training Project (Grant Number 2021-99).
Author Contributions: The authors confirm contribution to the paper as follows: study conception and design: L.-X. S, G.-C. Z; data collection: Z. Z, H. L, M.-R. Z, J.-Q. Z, J.-N. L, J.-P. L; analysis and interpretation of results: X. Z, J.-Z. W, M.-M. H; draft manuscript preparation: X. Z, L.-X. S. All authors reviewed the results and approved the final version of the manuscript.
Availability of Data and Materials: All data generated or analyzed during this study are included in this published article (and its supplementary information files).
Ethics Approval: The study was conducted according to the guidelines of the Declaration of Helsinki. All patients and subjects provided informed consent, and ethical approval was obtained from the Ethics Committee of the Third Affiliated Hospital of Hebei Medical University (No. K2022-003-1).
Conflicts of Interest: The authors declare that they have no conflicts of interest to report regarding the present study.
Supplementary Materials: The supplementary material is available online at https://doi.org/10.32604/biocell.2023.046640.
References
1. Owens B. Outlook: multiple myeloma. Nature. 2020;587(7835):S55. [Google Scholar] [PubMed]
2. Blade J, Beksac M, Caers J, Jurczyszyn A, von Lilienfeld-Toal M, Moreau P, et al. Extramedullary disease in multiple myeloma: a systematic literature review. Blood Cancer J. 2022;12(3):45. [Google Scholar] [PubMed]
3. Bhutani M, Foureau DM, Atrash S, Voorhees PM, Usmani SZ. Extramedullary multiple myeloma. Leukemia. 2020;34(1):1–20. [Google Scholar] [PubMed]
4. Beksac M, Seval GC, Kanellias N, Coriu D, Rosiñol L, Ozet G, et al. A real world multicenter retrospective study on extramedullary disease from Balkan Myeloma Study Group and Barcelona University: analysis of parameters that improve outcome. Haematologica. 2020;105(1):201–8. [Google Scholar] [PubMed]
5. Philip M, Schietinger A. CD8+ T cell differentiation and dysfunction in cancer. Nat Rev Immunol. 2022;22(4):209–23. [Google Scholar] [PubMed]
6. Zhang J, Lei F, Tan H. The development of CD8 T-cell exhaustion heterogeneity and the therapeutic potentials in cancer. Front Immunol. 2023;14:1166128. [Google Scholar] [PubMed]
7. Kwon M, Kim CG, Lee H, Cho H, Kim Y, Lee EC, et al. PD-1 blockade reinvigorates bone marrow CD8+ T cells from patients with multiple myeloma in the presence of TGFbeta inhibitors. Clin Cancer Res. 2020;26(7):1644–55. [Google Scholar] [PubMed]
8. Alrasheed N, Lee L, Ghorani E, Henry JY, Conde L, Chin M, et al. Marrow-infiltrating regulatory T cells correlate with the presence of dysfunctional CD4+PD-1+ cells and inferior survival in patients with newly diagnosed multiple myeloma. Clin Cancer Res. 2020;26(13):3443–54. [Google Scholar] [PubMed]
9. Giannotta C, Castella B, Tripoli E, Grimaldi D, Avonto I, D’Agostino M, et al. Immune dysfunctions affecting bone marrow Vγ9Vδ2 T cells in multiple myeloma: role of immune checkpoints and disease status. Front Immunol. 2022;13:1073227. [Google Scholar] [PubMed]
10. Huang S, Liang C, Zhao Y, Deng T, Tan J, Zha X, et al. Increased TOX expression concurrent with PD-1, Tim-3, and CD244 expression in T cells from patients with acute myeloid leukemia. Cytometry Part B, Clin Cytometry. 2022;102(2):143–52. [Google Scholar]
11. Zhao Y, Liao P, Huang S, Deng T, Tan J, Huang Y, et al. Increased TOX expression associates with exhausted T cells in patients with multiple myeloma. Exp Hematol Oncol. 2022;11(1):12. [Google Scholar] [PubMed]
12. Lee MY, Park CJ, Cho YU, You E, Jang S, Seo EJ, et al. Immune checkpoint programmed cell death protein-1 (PD-1) expression on bone marrow T cell subsets in patients with plasma cell myeloma. Ann Lab Med. 2021;41(3):259–67. [Google Scholar] [PubMed]
13. Guillerey C, Harjunpaa H, Carrie N, Kassem S, Teo T, Miles K, et al. TIGIT immune checkpoint blockade restores CD8+ T-cell immunity against multiple myeloma. Blood. 2018;132(16):1689–94. [Google Scholar] [PubMed]
14. Lee BH, Kim JH, Kang KW, Lee SR, Park Y, Sung HJ, et al. PVR (CD155) expression as a potential prognostic marker in multiple myeloma. Biomedicines. 2022;10(5):1099. [Google Scholar] [PubMed]
15. Zelle-Rieser C, Thangavadivel S, Biedermann R, Brunner A, Stoitzner P, Willenbacher E, et al. T cells in multiple myeloma display features of exhaustion and senescence at the tumor site. J Hematol Oncol. 2016;9(1):116. [Google Scholar] [PubMed]
16. Le Calvez B, Moreau P, Touzeau C. Immune checkpoint inhibitors for the treatment of myeloma: novel investigational options. Expert Opin Investig Drugs. 2021;30(9):965–73. [Google Scholar] [PubMed]
17. Jing W, Gershan JA, Weber J, Tlomak D, McOlash L, Sabatos-Peyton C, et al. Combined immune checkpoint protein blockade and low dose whole body irradiation as immunotherapy for myeloma. J Immunother Cancer. 2015;3(1):2. [Google Scholar] [PubMed]
18. Armand P, Lesokhin A, Borrello I, Timmerman J, Gutierrez M, Zhu L, et al. A phase 1b study of dual PD-1 and CTLA-4 or KIR blockade in patients with relapsed/refractory lymphoid malignancies. Leukemia. 2021;35(3):777–86. [Google Scholar] [PubMed]
19. Crescenzi A, Annibali O, Bianchi A, Pagano A, Donati M, Grifoni A, et al. PD-1/PD-L1 expression in extra-medullary lesions of multiple myeloma. Leuk Res. 2016;49:98–101. [Google Scholar] [PubMed]
20. Lee BH, Park Y, Kim JH, Kang KW, Lee SJ, Kim SJ, et al. Prognostic value of galectin-9 relates to programmed death-ligand 1 in patients with multiple myeloma. Front Oncol. 2021;11:669817. [Google Scholar] [PubMed]
21. Rajkumar SV. Multiple myeloma: 2020 update on diagnosis, risk-stratification and management. Am J Hematol. 2020;95(5):548–67. [Google Scholar] [PubMed]
22. Lucas F, Pennell M, Huang Y, Benson DM, Efebera YA, Chaudhry M, et al. T cell transcriptional profiling and immunophenotyping uncover LAG3 as a potential significant target of immune modulation in multiple myeloma. Biol Blood Marrow Transplant. 2020;26(1):7–15. [Google Scholar] [PubMed]
23. Cooke RE, Quinn KM, Quach H, Harrison S, Prince HM, Koldej R, et al. Conventional treatment for multiple myeloma drives premature aging phenotypes and metabolic dysfunction in T cells. Front Immunol. 2020;11:2153. [Google Scholar] [PubMed]
24. Maimela NR, Liu S, Zhang Y. Fates of CD8+ T cells in tumor microenvironment. Comput Struct Biotechnol J. 2019;17:1–13. [Google Scholar] [PubMed]
25. Anandappa AJ, Wu CJ, Ott PA. Directing traffic: how to effectively drive T cells into tumors. Cancer Discov. 2020;10(2):185–97. [Google Scholar] [PubMed]
26. Jantz-Naeem N, Böttcher-Loschinski R, Borucki K, Mitchell-Flack M, Böttcher M, Schraven B, et al. TIGIT signaling and its influence on T cell metabolism and immune cell function in the tumor microenvironment. Front Oncol. 2023;13:1060112. [Google Scholar] [PubMed]
27. Annese T, Tamma R, Ribatti D. Update in TIGIT immune-checkpoint role in cancer. Front Oncol. 2022;12:871085. [Google Scholar] [PubMed]
28. Jin S, Zhang Y, Zhou F, Chen X, Sheng J, Zhang J. TIGIT: a promising target to overcome the barrier of immunotherapy in hematological malignancies. Front Oncol. 2022;12:1091782. [Google Scholar] [PubMed]
29. Minnie SA, Kuns RD, Gartlan KH, Zhang P, Wilkinson AN, Samson L, et al. Myeloma escape after stem cell transplantation is a consequence of T-cell exhaustion and is prevented by TIGIT blockade. Blood. 2018;132(16):1675–88. [Google Scholar] [PubMed]
30. Ge Z, Peppelenbosch MP, Sprengers D, Kwekkeboom J. TIGIT, the next step towards successful combination immune checkpoint therapy in cancer. Front Immunol. 2021;12:699895. [Google Scholar] [PubMed]
31. Cao Y, Li Q, Liu H, He X, Huang F, Wang Y. Role of Tim-3 in regulating tumorigenesis, inflammation, and antitumor immunity therapy. Cancer Biomark. 2021;32(2):237–48. [Google Scholar] [PubMed]
Cite This Article
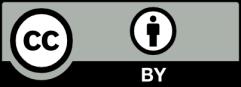
This work is licensed under a Creative Commons Attribution 4.0 International License , which permits unrestricted use, distribution, and reproduction in any medium, provided the original work is properly cited.