Open Access
REVIEW
Farnesol as a multifunctional candidate for treatment development
1 Department of Epidemiology and Health Statistics, Xiangya School of Public Health, Central South University, Changsha, 410017, China
2 Department of Chemistry, Sri Krishnadevaraya University, Anantapur, 515003, India
3 School of Food Science and Nutrition, University of Leeds, Leeds, LS2 9JT, UK
* Corresponding Author: WING-FU LAI. Email:
# These authors contributed equally to this article
BIOCELL 2024, 48(2), 163-171. https://doi.org/10.32604/biocell.2023.043839
Received 14 July 2023; Accepted 30 November 2023; Issue published 23 February 2024
Abstract
Farnesol is a C15 isoprenyl alcohol generated from the hydrolysis of farnesyl diphosphate under the action of farnesol synthase in plants. Although this sesquiterpenoid alcohol has been exploited in a wide variety of industrial applications, serious discussions on the possible use of this agent in disease treatment have been scant until now. In this article, we review the current understanding of the physiological properties of farnesol and the potential use of the agent as a candidate for treatment development. In fact, since the turn of the last century, farnesol has been found not only to trigger apoptosis in tumor cells but also to be able to function as a quorum-sensing molecule in Candida albicans to influence infection dynamics. By offering a snapshot of recent advances in research on farnesol, it is expected that this article can provide more insights into future research on farnesol for the development of different treatment strategies.Keywords
Plants and related botanical products possess antioxidative properties and can lead to various health-promoting effects [1]. Over the last several decades, research on the therapeutic potential of essential oil extracts from plants has gained increasing interest [2,3]. Essential oil extracts are volatile liquids consisting of a multitude of aromatic substances, with terpenes being the most prominent class of aromatic agents [4]. Common types of terpenes are monoterpenes and sesquiterpenes. Both of which can be found in essential oils [5]. They have been extensively studied because of their possible clinical applications, partly resulting from their antimicrobial, antitumor, anti-parasitic, hypoglycemic, and anti-inflammatory properties [6–9]. Considering the therapeutic potential of aromatic plants, more research is highly warranted to explore the properties of those phytochemicals. This review focuses on the potential of one of these agents, namely farnesol, as a candidate for treatment development.
Farnesol is a sesquiterpene alcohol found in various natural sources, including the oils of seeds, aromatic plants, and citrus fruits [10]. Under ambient conditions, farnesol is a colorless liquid with a mild, pleasant aroma [11]. Industrially, it is often added to deodorants, soaps, and perfumes [12]. Farnesol is regarded by the Food and Drug Administration (FDA) of the United States to be generally safe for use as an ingredient in flavoring products for human consumption [11]. It can be found in greater amounts in soil because it is not vulnerable to photolysis and has low volatility in arid soils [13,14]. Till now, most research has focused on the effectiveness of farnesol in serving as a biocontrol agent against phytopathogenic nematodes [15], as an antimicrobial agent against bacteria (e.g., Staphylococcus epidermidis) [16], and as an antifungal agent against yeasts (e.g., Saccharomyces cerevisiae) [17]. In fact, farnesol can function as an extracellular trigger for apoptosis in human tumor cells. It preferentially acts on neoplastic cells. Its ability to stop the production of phosphatidylcholine and its role in diacylglycerol signaling pathways have been found to cause apoptosis [18,19]. Furthermore, farnesol has been identified as a quorum-sensing molecule produced by Candida albicans. It can control the population density through quorum sensing, affecting the infection process [20,21]. Apart from the above-mentioned functions, farnesol exhibits antidiarrheal activity in rodents partly via its ability to interact with the monosialotetrahexosylganglioside receptor and with cholera toxin, though its interactions with the muscarinic receptor type 3 may play a role as well [22]. This warrants further exploitation of farnesol for use in the treatment of diarrheal diseases. Apart from this, farnesol has been investigated for use in cancer treatment and neuroprotection. Some of the relevant evidence will be highlighted in the following sections of this article.
Use of farnesol as an anticancer agent
Farnesol is an apoptosis inducer and has been shown to inhibit the growth of several tumor cells in vitro [23]. The oncogenic signaling pathways modulated by farnesol are shown in Fig. 1 [10]. As shown by Öztürk and coworkers [24], farnesol can modulate the activity of tumorigenic proteins (as well as signal transduction cascades) in various cancer cells and can downregulate the expression level of proteins involved in cell proliferation. It can also lead to downregulation of the Ras-extracellular signal-regulated kinase (ERK)1/2 signaling pathway, resulting in cell growth inhibition and cell death. Ras, a diminutive GTPase, is the primary regulator of the ERK/mitogen-activated protein kinase (MAPK) pathway [25]. It and its downstream signaling counterpart, ERK/MAPK, can influence the activation of the phosphatidylinositol-3-kinase (PI3K) pathway, the mammalian target of rapamycin (mTOR) pathway, and the AMP-activated protein kinase pathway. Ras, therefore, plays a fundamental role in overseeing various cellular activities, including cell growth and differentiation, programmed cell death, and even the onset and progression of cancer [26]. The antitumor effect of farnesol is also mediated directly via downregulation of the PI3K/protein kinase B (Akt) signaling pathway [27]. Based on this property, a nebulized aerosol formulation of farnesol has been developed to induce cell death in human lung cancer cells [27]. Recently, one study has evaluated the cytotoxic effects of nebulized farnesol on cancer cell lines (viz., H460 and CALU6) [24]. Nebulized farnesol (at a concentration above 120 μM) has been shown to lead to 100% loss of viability of H460 cells [24].
Figure 1: Major oncogenic signaling pathways modulated by the action of farnesol (Reproduced from [10] with permission from MDPI).
In both in vitro and in vivo contexts, treatment with farnesol has been found to promote the expression of p53, phosphorylated c-Jun N-terminal kinase (JNK), cleaved caspase-3, Bax, and cleaved caspase-9, while reducing the expression of p-PI3K, p-Akt, p-p38, and Bcl-2. This, in turn, increases the proportion of apoptotic cells [28]. The protein p53, produced by the TP53 gene located on the human chromosome 17, is pivotal in preventing tumor growth. It intricately interacts with signaling pathways crucial for fundamental cellular functions such as cell division. Such an interaction effectively hinders the physical and genetic changes associated with cancer development [29]. Bax and Bak are vital regulators of proapoptotic signaling, with their disruption linked to cancer development. p53 can regulate genes for cell growth arrest and can also activate proapoptotic BH3-only Bcl-2 family members. Evasion of apoptosis plays an important role in tumorigenesis [30,31].
Apart from the anticancer action delineated above, farnesol can induce cell death in a human T-lymphocytic leukemia cell line (Molt4) by activating apoptosomes [32]. The decrease in the mitochondrial membrane potential (ΔΨm) of farnesol-treated Molt4 cells suggests that treatment with farnesol leads to the opening of the permeability transition pore, thereby allowing cytochrome c to be released from mitochondria into the cytoplasm, activating caspases 9 and 3 and ultimately inducing the onset of apoptosis. Furthermore, apoptosis in farnesol-treated Molt4 cells is shown to be accelerated by externalization of phosphatidylserine, depolarization of the plasma membrane, and loss of intracellular potassium. All these are associated with the activation of the PERK-eIF2α-ATF3/4 cascade [32]. In recent years, farnesol has been exploited for its chemopreventive potential on 9,10-dimethylbenz(a)anthracene-initiated and 12-O-tetradecanoylphorbol-13-acetate-promoted skin tumorigenesis in Swiss albino mice [33]. At a low concentration, farnesol inhibits the Ras/Raf/ERK1/2 signaling pathway, whereas at a high dose, the opposite effect prevails. Nonetheless, all concentrations of farnesol have been shown to inhibit the induction of apoptosis by modifying the Bax/Bcl-2 ratio. This reveals the application potential of farnesol in the treatment of cancer.
Use of farnesol as an antioxidant
The antioxidative effect of farnesol has partially explained the anti-cancer capacity of the agent. This is attributed to the fact that oxidative stress is an important factor underlying the onset of cancer. Apart from this, upon treatment with farnesol, the activity of glutathione (GSH) (as well as its dependent enzymes) and catalase in mice (suffering from cadmium toxicity induced by administration of cadmium chloride) has been restored [23]. Cadmium-induced microcrack formation and chromosomal aberrations have also been found to be significantly alleviated [23]. The restoration of the second-stage enzymes and the enhancement of the antioxidant status are thought to partially explain the anticarcinogenic property of farnesol [23].
By inhibiting oxidative stress and restoring antioxidant capacity, farnesol can protect against high cholesterol diet-induced liver damage, too [34]. Numerous studies have reported that cholesterol-enriched diets can induce oxidative stress in various tissues [35–37]. Hypercholesterolaemia has been shown to be triggered by oxidative modifications of low-density lipoproteins, protein glycation, and glucose auto-oxidation [38,39]. On the other hand, high cholesterol levels can promote reactive oxygen species (ROS) leakage from the mitochondrial electronic system and can cause activation of NADPH oxidase and xanthine oxidase, ultimately leading to hepatic lipid peroxidation and protein oxidation [38,40]. In the in vivo context, farnesol has been shown to be able to reduce hepatic lipid peroxidation, increase the levels of reduced GSH and antioxidant enzymes in the liver, and attenuate hepatic oxidative stress [34]. It can, therefore, protect the liver from cyclophosphamide-induced oxidative damage in mice [41]. With such antioxidative capacity, farnesol has also been reported to protect the prostate tissue from cigarette smoke-induced oxidative stress [42].
Use of farnesol in neuro- and cardio-protection
The neuroprotective capacity of farnesol comes partly from its anti-neuroinflammatory effect on chronic sleep deprivation-induced cognitive impairment, its ability to inhibit microglia activation, and its capacity to reduce the secretion of pro-inflammatory cytokines [43]. Sleep deprivation-induced cognitive impairment is associated with a decrease in the level of antioxidants and an increase in the ROS level [44]. As shown by earlier studies [43,45], farnesol can increase the activity of superoxide dismutase and protect the central nervous system from the action of certain toxic agents. There is ample evidence showing that a decline in the level of Sirt1 contributes to the development of sleep deprivation-induced cognitive impairment [46,47], with the Sirt1/Nrf2/HO-1/Gpx4 signaling pathway being a plausible therapeutic target [43]. HO-1 promotes antioxidative and anti-inflammatory effects through its action on Sirt1 [48–50]. On the other hand, Nrf2 and HO-1 can be induced to modulate Gpx4 synthesis to regulate iron toxicity [51]. Furthermore, inhibiting iron denaturation in the hippocampus can attenuate sleep deprivation-induced memory loss [52,53]. Upon treatment with farnesol, the decline in Sirt1 expression in the hippocampus of mice suffering from sleep deprivation-induced cognitive impairment has been restored [43]. The reduction in the levels of Nrf2, HO-1, and Gpx4 in chronically sleep-deprived mice has also been shown to be reversed [43]. All these result in neuroprotection.
Besides neuroprotection, farnesol can reverse impaired vascular tone in resistance arteries caused by chronic β-adrenergic stimulation [54], inhibit pro-inflammatory cytokine expression [55], and reduce the activity of nuclear factor kappa B (NF-κB) [55]. In spontaneously hypertensive rats, farnesol serves as an inhibitor of vascular smooth muscle Ca2+ signaling [56]. It exhibits plasma membrane Ca2+ channel blocking capacity [56] and produces a hypotensive effect by blocking L-type calcium channels in smooth muscle cells [54]. In addition, treatment with farnesol can attenuate the extent of lipid peroxidation during cardiac hypertrophy [54], thereby alleviating cellular damage. Cell membrane damage is postulated to be partly caused by oxidative stress. If the ROS fails to be neutralized by antioxidant molecules and reacts with lipids, it results in an increase in the production of lipid peroxides [57–59]. Taking this into consideration, one may infer that the mechanism by which farnesol attenuates cardiac cell damage is through its ability to serve as an antioxidant molecule to neutralize the ROS, thereby blocking further damage experienced by the cardiac myocyte membrane. Furthermore, farnesol is cardioprotective partly because of its ability to reduce the activation of ERK1/2, Bax, and caspase 3, and to increase the expression of Akt and Bcl-2 proteins [54].
Apart from the activities described above, farnesol improves arrhythmia scores and reduces the incidence of the most severe arrhythmias [60]. During Ca2+ overload, NADPH oxidase is produced in different cell types [61]. The high level of Ca2+ in mitochondria also leads to the production of multiple oxidation products [61]. As shown in previous studies [62,63], excessive oxidative stress caused by Ca2+ overload subjects cardiomyocytes to cellular damage and promotes phospholipid acylation through oxidation of methionine in the Ca2+/calmodulin-dependent protein kinase II (CaMKII) regulatory domain. In addition, once Ca2+ waves are triggered, arrhythmias is resulted [62–64]. Farnesol prevents arrhythmias by blocking multiple cardiac ionic currents, including L-type Ca2+ currents and transient outward K+ currents [60]. This renders farnesol effective in cardioprotection.
Recent advances in the development of farnesol-based treatment
Owing to the variety of physiological activities exhibited by farnesol, different farnesol-based treatment approaches have been exploited in preclinical trials. For instance, a gel composed of hydroxypropyl methylcellulose (HPMC) and liposomal farnesol was developed for wound treatment [65]. In a rat model of third-degree burns, the gel was found to be more effective than the commercial silver sulfadiazine cream (as well as HPMC alone) in triggering tissue repair [65]. Apart from this, farnesol has been adopted as a booster for antibiotic therapy [66–68]. In one recent report, the adherence of vaginal Lactobacillus cells to vaginal epithelial cells was significantly reduced upon treatment with farnesol [69]. This reveals the potential of farnesol as an antimicrobial agent for the treatment of vaginitis.
Recently, the influence of farnesol on planktonic development and cell permeability was investigated by Namba and colleagues [70], who examined the capacity of farnesol to enhance the effect of antimicrobial photodynamic inactivation (aPDI) on Enterococcus faecalis. During the study, E. faecalis planktonic cells and biofilms were treated with farnesol (0.25 mM) for 2 h prior to treatment with aPDI [70]. In combination with aPDI, farnesol significantly improved the antibacterial capacity, leading to a remarkable decline in the colony-forming units. The potentiating activity of farnesol was proposed to be related to the effect of farnesol on the permeability of the bacterial cell. This finding demonstrates the possible use of farnesol in combination with PDI to fight against infection [70]. The antibacterial activity of farnesol was further corroborated by Castelo-Branco and co-workers [71], who studied the effect of farnesol (either alone or in combination with antibacterial drugs such as ceftazidime, doxycycline, amoxicillin, and sulfamethoxazole-trimethoprim) on Burkholderia pseudomallei biofilms. Farnesol inhibits the growth of B. pseudomallei biofilms and increases the antimicrobial effect of antibiotics [71]. The effectiveness of co-formulating farnesol with other agents in combating biofilm formation was also demonstrated by using C. albicans and Streptococcus mutans dual-species biofilms grown on saliva-coated hydroxyapatite discs [72]. Biofilms treated with 4′-hydroxychalcone (C135) alone exhibited a 3D structure different from those treated with C135 in combination with farnesol and/or sodium fluoride (Fig. 2) [72]. In addition, co-formulation of C135 with farnesol was more effective than C135 alone in inhibiting the production of insoluble exopolysaccharides to reduce the biomass of the biofilms [72].
Figure 2: Confocal microscopy images of 43 h-old Candida albicans and Streptococcus mutans dual-species biofilms. The biofilms were treated with 4′-hydroxychalcone (C135), tt-farnesol (Far), sodium fluoride (F), vehicle (V) (consisting of ethanol and dimethyl sulfoxide), or a combination of these agents. The arrows indicate the C. albicans cells. Yellow ones show the hyphal cells. The white ones show the cells in the yeast morphology. Green fluorescence comes from SYTO9 labeling, whereas red fluorescence shows the exopolysaccharides in the extracellular matrix stained with Alexa Fluor 647. The larger images give an overlay of the red and green fluorescence images. Reproduced from [72] with permission from the American chemical society.
To improve drug retention in areas where biofilms grow, pH-responsive nanoparticles have been adopted as carriers of farnesol (Fig. 3) [73]. These cationic nanoparticles are generated by using diblock copolymers consisting of 2-(dimethylamino)ethyl methacrylate (DMAEMA), butyl methacrylate, and 2-propylacrylic acid. The generated nanoparticles were shown to exhibit high adsorption affinity to negatively charged hydroxyapatite, saliva-coated hydroxyapatite (sHA), and exopolysaccharide-coated sHA [73]. Such affinity is thought to result from strong electrostatic interactions mediated by multivalent tertiary amines of p(DMAEMA) [73]. Topical treatment with farnesol-loaded nanoparticles was reported to be four times more effective than that with free farnesol in disrupting S. mutans biofilms [73]. Contrary to free farnesol, which showed no effect on carious lesions, treatment with farnesol-loaded nanoparticles significantly reduced the number of carious lesions [73]. All these demonstrate the role of nanotechnologies in enhancing the therapeutic activity of farnesol. The viability of using nanotechnologies in the development of farnesol-based treatment was further evidenced in a more recent study [74], in which biofilms of Pseudomonas aeruginosa were treated with liposomes loaded with farnesol and ciprofloxacin. Those liposomes successfully inhibited the growth of the biofilms. The presence of farnesol enhanced the antimicrobial action of ciprofloxacin [74], leading to a larger degree of biofilm disruption, a higher ratio of dead cells, and a deeper level of biofilm destruction [74]. More recently, coformulation of farnesol with β-cyclodextrin was developed for the treatment of hypertension [3]. Compared to using farnesol alone, the coformulation displayed a stronger effect in combating hypertension in vivo [3]. Molecular docking revealed that farnesol forms hydrogen bonds with the ligand-binding residues on M3 and M2 muscarinic and nicotinic receptors [3]. This suggests that farnesol may cause hypotension (and bradycardia) via its action on these receptors. Regarding the fact that the antihypertensive effect of farnesol can be enhanced in the presence of β-cyclodextrin, new farnesol-based cardiovascular therapies may be developed in the future via formulation strategies (e.g., inclusion complexation) [3].
Figure 3: (A) The synthesis and self-assembly of the diblock copolymer. (B) Chemical structures of the control polymers adopted to demonstrate the role of p (DMAEMA) coronas, and nanoparticle structures, in binding to dental surfaces. (C) The mode of action of the pH-responsive nanoparticles for tackling biofilm formation. Abbreviations: AIBN, 2, 2-azobis(isobutyronitrile); BMA, butyl methacrylate; CTA, chain transfer agent; DMAEMA, 2-(dimethylamino)ethyl methacrylate; DMF, dimethylformamide; DP, degree of polymerization; ECT, ethylsulfanylthiocarbonyl sulfanylpentanoic acid; EPS, exopolysaccharides; NPC, nanoparticle carrier; PAA, 2-propylacrylic acid. Reproduced from [73] with permission from the American chemical society.
Apart from tackling biofilm formation and diseased conditions, farnesol has the potential to ameliorate the effect of toxic agents. This was demonstrated by Araghi and colleagues [41], who studied the capacity of farnesol to reduce the toxicity of cyclophosphamide. Upon treatment with cyclophosphamide (200 mg/kg) for 7 days, blood samples, as well as tissues from different organs were collected from the treated mice. Pretreating the mice with farnesol before cyclophosphamide treatment reduced the severity of tissue damage, particularly in the testis, liver, and spleen [41]. This finding confirms the protective effect of farnesol against oxidative stress caused by cyclophosphamide in tissues, suggesting that farnesol may benefit patients who are undergoing treatment with cyclophosphamide [41]. In another study, farnesol was shown to protect the lungs from the effect of toxic agents in cigarette smoke [1]. Upon administration of a single gavage dose of farnesol to mice for 7 days, intratracheal instillation of aqueous cigarette smoke extract (CSE) was given to mice to induce lung damage, followed by measurement of the H2O2 levels and the activity of antioxidant enzymes (including glutathione peroxidase, glutathione reductase, and catalase). Farnesol protected the lung by decreasing the malondialdehyde level in bronchoalveolar lavage fluid [1]. It also restored the activity of the tested antioxidant enzymes in the lung tissue and decreased the H2O2 content. All these reveal the effect of farnesol in acting against CSE-induced lung inflammation [1].
Toxicity and adverse effects caused by farnesol-based treatment
Over the years, extensive research efforts have explored the therapeutic potential of farnesol in vitro and in vivo. Despite the promising potential of farnesol as delineated in the preceding sections, few incidents of adverse effects caused by farnesol have been reported. Allergic contact dermatitis is currently reported as a possible adverse reaction caused by farnesol-based treatment. Schnuch and coworkers noted that farnesol can be an important allergen [75]. In a local lymph node assay, farnesol was shown to be a skin sensitizer [76]. The possible adverse effect led by improper use of farnesol was further corroborated by Silva and colleagues [3], who reported the occurrence of dose-dependent hypotension and bradycardia in farnesol-treated normotensive rats. This finding demonstrates the need to further investigate the adverse physiological effect brought about by improper use of farnesol before farnesol-based treatment is widely adopted in routine clinical practice.
Notwithstanding the potential toxicity of farnesol, neoplastic cells appear to be more sensitive to farnesol toxicity than non-neoplastic ones [77]. This was demonstrated by the fact that the effect of farnesol on cell growth inhibition and cell death is more significant in neoplastic cells than in cells derived from normal tissues [77]. In addition, farnesol-induced inhibition of cell growth can be potentially reversed by administration of exogenous diacylglycerol, a phosphocholine-derived metabolite and a second messenger in protein kinase C (PKC)-dependent signal transduction. This suggests that PKC may play a role in farnesol-triggered apoptotic cell death [77]. The selective toxicity of farnesol was also observed by Eugenia and colleagues [78], who noted that compared to the viability of cells obtained from normal tissues, cultured cells generated from neoplastic tissues experience a more significant drop in viability upon treatment with farnesol. After 1 h treatment with farnesol, translocation of the PKC in HeLa cells from the membrane fraction to the cytoplasm was reported [78]. On the other hand, the effect of farnesol on the localization of PKC in CF-3 fibroblasts was found to be negligible [78]. Such selectivity was corroborated recently by Ozturk and colleagues [24], who investigated the in vitro effect of farnesol on cancer cell lines and on healthy human lung epithelial BEAS-2B cells. They found that farnesol has a dose-dependent effect on cancer cell lines, but the effect of farnesol on the growth of BEAS-2B cells is not significant [24].
Farnesol possesses multifunctional properties that render it potentially applicable to the treatment of various diseases, ranging from cancer to inflammatory diseases [10]. It exhibits a broad spectrum of antimicrobial activity. This makes farnesol useful for either preventing the growth of microorganisms or making the microbes more susceptible to the action of other antibiotics [18,79–82]. Farnesol can exert neuro- and cardioprotective action, too. Not only can it mitigate cognitive impairment by reducing neuroinflammation and by modulating key signaling pathways, but it can also improve vascular tone and reduce inflammation in the cardiovascular system. All these demonstrate the potential use of farnesol as a multifunctional candidate for treatment development. For further research, apart from studying the mechanism and therapeutic action of farnesol per se, developing co-formulations consisting of farnesol and other bioactive agents may turn out to be a rewarding research direction. To streamline the translation of farnesol-based treatment from the preclinical context to routine clinical practice, fully understanding the mechanisms of action of farnesol, optimizing the dose, and evaluating the short-term and long-term toxicity appear to be some major directions that are worth investigating. Finally, farnesol actually has four stereoisomers (Fig. 4). Among them, (2E,6E)-farnesol used to be the only commercially available one that can be readily attained with high isomeric purity [83]. With advances in synthetic chemistry, right now all the four olefin stereoisomers of farnesol can be synthesized from nerylacetone or geranylacetone [83]. Yet, variations in the therapeutic action and properties of the stereoisomers of farnesol are still poorly understood. Addressing this question may help elucidate the structure-activity relationship of farnesol and identify more rational strategies to manipulate the chemical structure of farnesol to enhance the selectivity and efficiency in treatment development in the future.
Figure 4: Isomers of farnesol.
Acknowledgement: The authors would like to thank Melody Fong for helpful discussions during the preparation of this manuscript.
Funding Statement: There was no external funding to support this work.
Author Contributions: The authors confirm contribution to the paper as follows: Conceptualization: WFL; data curation: AA, SRO, WFL; writing-original draft: SRO, WFL; writing-review & editing: AA, WFL; supervision: WFL. All authors reviewed and approved the final version of the manuscript.
Availability of Data and Materials: Data sharing is not applicable as no new data were created or analyzed in this article.
Ethics Approval: Not applicable.
Conflicts of Interest: The authors have no relevant declarations of interest.
References
1. Qamar W, Sultana S. Farnesol ameliorates massive inflammation, oxidative stress and lung injury induced by intratracheal instillation of cigarette smoke extract in rats: an initial step in lung chemoprevention. Chem Biol Interact. 2008;176(2):79–87. [Google Scholar]
2. Alkhanjaf AAM, Athar MT, Ullah Z, Alsayhab AMH, Umar A, Shaikh IA. Farnesol protects against cardiotoxicity caused by doxorubicin-induced stress, inflammation, and cell death: an in vivo study in wistar rats. Mol. 2022;27(23):8589. [Google Scholar]
3. Silva EAP, Carvalho JS, dos Santos DM, Oliveira AMS, de Souza Araújo AA, Serafini MR, et al. Cardiovascular effects of farnesol and its β-cyclodextrin complex in normotensive and hypertensive rats. Eur J Pharmacol. 2021;901:174060. [Google Scholar]
4. Bakkali F, Averbeck S, Averbeck D, Idaomar M. Biological effects of essential oils—A review. Food Chem Toxicol. 2008;46(2):446–75. [Google Scholar]
5. de Araújo Delmondes G, Bezerra DS, de Queiroz Dias D, de Souza Borges A, Araújo IM, da Lins Cunha G, et al. Toxicological and pharmacologic effects of farnesol (C15H26Oa descriptive systematic review. Food Chem Toxicol. 2019;129:169–200. [Google Scholar]
6. Paduch R, Kandefer-Szerszeń M, Trytek M, Fiedurek J. Terpenes: substances useful in human healthcare. Arch Immunol Ther Exp. 2007;55(5):315–27. [Google Scholar]
7. Prado-Audelo D, Luisa M, Cortés H, Caballero-Florán IH, González-Torres M, Escutia-Guadarrama L, et al. Therapeutic applications of terpenes on inflammatory diseases. Front Pharmacol. 2021;12:704197. [Google Scholar]
8. Masyita A, Mustika Sari R, Dwi Astuti A, Yasir B, Rahma Rumata N, Emran TB, et al. Terpenes and terpenoids as main bioactive compounds of essential oils, their roles in human health and potential application as natural food preservatives. Food Chem: X. 2022;13:100217. [Google Scholar]
9. Yang W, Chen X, Li Y, Guo S, Wang Z, Yu X. Advances in pharmacological activities of terpenoids. Nat Prod Commun. 2020;15(3):1934578X20903555. [Google Scholar]
10. Jung YY, Hwang ST, Sethi G, Fan L, Arfuso F, Ahn KS. Potential anti-inflammatory and anti-cancer properties of farnesol. Mol. 2018;23(11):2827. [Google Scholar]
11. Lapczynski A, Bhatia SP, Letizia CS, Api AM. Fragrance material review on farnesol. Food Chem Toxicol. 2008;46(11):S149–S56. [Google Scholar]
12. Sarkic A, Stappen I. Essential oils and their single compounds in cosmetics—A critical review. Cosmet. 2018;5(1):11. [Google Scholar]
13. Duke JA. Handbook of phytochemical constituent grass, herbs and other economic plants. Boca Raton, Florida: CRC Press; 1992. [Google Scholar]
14. Hawker DW, Boonsaner M, Connell DW, Sripongpun G. The role of organic matter components in soil on partitioning of chlorohydrocarbons. Toxicol Environ Chem. 1999;71(3–4):289–307. [Google Scholar]
15. Rowat AC, Keller D, Ipsen JH. Effects of farnesol on the physical properties of DMPC membranes. Biochim Biophys Acta Biomembr. 2005;1713(1):29–39. [Google Scholar]
16. Gomes FIA, Teixeira P, Azeredo J, Oliveira R. Effect of farnesol on planktonic and biofilm cells of staphylococcus epidermidis. Curr Microbiol. 2009;59(2):118–22. [Google Scholar]
17. Fairn GD, MacDonald K, McMaster CR. A chemogenomic screen in saccharomyces cerevisiae uncovers a primary role for the mitochondria in farnesol toxicity and its regulation by the Pkc1 pathway. J Biol Chem. 2007;282(7):4868–74. [Google Scholar]
18. Rao CV, Newmark HL, Reddy BS. Chemopreventive effect of farnesol and lanosterol on colon carcinogenesis. Cancer Detect Prev. 2002;26(6):419–25. [Google Scholar]
19. Voziyan PA, Haug JS, Melnykovych G. Mechanism of farnesol cytotoxicity: further evidence for the role of pkc-dependent signal transduction in farnesol-induced apoptotic cell death. Biochem Biophys Res Commun. 1995;212(2):479–86. [Google Scholar]
20. Ramage G, Saville Stephen P, Wickes Brian L, López-Ribot José L. Inhibition of candida albicans biofilm formation by farnesol, a quorum-sensing molecule. Appl Environ Microbiol. 2002;68(11):5459–63. [Google Scholar] [PubMed]
21. Scheper MA, Shirtliff ME, Meiller TF, Peters BM, Jabra-Rizk MA. Farnesol, a fungal quorum-sensing molecule triggers apoptosis in human oral squamous carcinoma cells. Neoplasia. 2008;10(9):954–63. [Google Scholar] [PubMed]
22. da Costa DS, Negreiros PDS, da Silva VG, Nunes DB, Acha BT, Quintans-Junior LJ, et al. Antidiarrheal activity of farnesol in rodents: pharmacological actions and molecular docking. Eur J Pharmacol. 2020;874:172986. [Google Scholar] [PubMed]
23. Jahangir T, Khan TH, Prasad L, Sultana S. Alleviation of free radical mediated oxidative and genotoxic effects of cadmium by farnesol in Swiss albino mice. Redox Rep. 2005;10(6):303–10. [Google Scholar]
24. Yilmaz Öztürk B, Feyzullazade N, Dağ İ, Şengel T. The investigation of in vitro effects of farnesol at different cancer cell lines. Microsc Res Tech. 2022;85(8):2760–75. [Google Scholar]
25. Chaudhary SC, Siddiqui MS, Athar M, Alam MS. Geraniol inhibits murine skin tumorigenesis by modulating COX-2 expression, Ras-ERK1/2 signaling pathway and apoptosis. J Appl Toxicol. 2013;33(8):828–37. [Google Scholar]
26. Xu X, Yu H, Xu Y. Ras-ERK1/2 signaling promotes the development of osteosarcoma by regulating H2BK12ac through CBP. Cancer Manag Res. 2020;12:2501–2. [Google Scholar]
27. Wang YL, Liu HF, Shi XJ, Wang Y. Antiproliferative activity of Farnesol in HeLa cervical cancer cells is mediated via apoptosis induction, loss of mitochon-drial membrane potential (ΛΨm) and PI3K/Akt signalling pathway. Methods. 2018;8(11):752–7. [Google Scholar]
28. Park JS, Kwon JK, Kim HR, Kim HJ, Kim BS, Jung JY. Farnesol induces apoptosis of DU145 prostate cancer cells through the PI3K/Akt and MAPK pathways. Int J Mol Med. 2014;33(5):1169–76. [Google Scholar]
29. Marei HE, Althani A, Afifi N, Hasan A, Caceci T, Pozzoli G, et al. p53 signaling in cancer progression and therapy. Cancer Cell Int. 2021;21(1):703. [Google Scholar]
30. Degenhardt K, Chen G, Lindsten T, White E. BAX and BAK mediate p53-independent suppression of tumorigenesis. Cancer Cell. 2002;2(3):193–203. [Google Scholar]
31. Emily HYAC, Wei MC, Weiler S, Flavell RA, Mak TW, Lindsten T, et al. BCL-2, BCL-XL sequester BH3 domain-only molecules preventing BAX-and BAK-mediated mitochondrial apoptosis. Mol Cell. 2001;8(3):705–11. [Google Scholar]
32. Joo JH, Ueda E, Bortner CD, Yang XP, Liao G, Jetten AM. Farnesol activates the intrinsic pathway of apoptosis and the ATF4-ATF3-CHOP cascade of ER stress in human T lymphoblastic leukemia Molt4 cells. Biochem Pharmacol. 2015;97(3):256–68. [Google Scholar]
33. Chaudhary SC, Alam MS, Siddiqui MS, Athar M. Chemopreventive effect of farnesol on DMBA/TPA-induced skin tumorigenesis: involvement of inflammation, Ras-ERK pathway and apoptosis. Life Sci. 2009;85(5–6):196–205. [Google Scholar]
34. Abukhalil MH, Hussein OE, Bin-Jumah M, Saghir SAM, Germoush MO, Elgebaly HA, et al. Farnesol attenuates oxidative stress and liver injury and modulates fatty acid synthase and acetyl-CoA carboxylase in high cholesterol-fed rats. Environ Sci Pollut Res Int. 2020;27(24):30118–32. [Google Scholar]
35. Abbas AM, Sakr HF. Simvastatin and vitamin E effects on cardiac and hepatic oxidative stress in rats fed on high fat diet. J Physiol Biochem. 2013;69(4):737–50. [Google Scholar]
36. Bin-Jumah MN. Monolluma quadrangula protects against oxidative stress and modulates LDL receptor and fatty acid synthase gene expression in hypercholesterolemic rats. Oxid Med Cell Longev. 2018;2018:3914384. [Google Scholar]
37. Kocsis GF, Csont T, Varga-Orvos Z, Puskas LG, Murlasits Z, Ferdinandy P. Expression of genes related to oxidative/nitrosative stress in mouse hearts: effect of preconditioning and cholesterol diet. Med Sci Monit. 2010;16(1):Br32–9. [Google Scholar] [PubMed]
38. Singh UN, Kumar S, Dhakal S. Study of oxidative stress in hypercholesterolemia. Int J Contemp Med Res. 2017;4(5):1204–7. [Google Scholar]
39. Yang RL, Shi YH, Hao G, Li W, Le GW. Increasing oxidative stress with progressive hyperlipidemia in human: relation between malondialdehyde and atherogenic index. J Clin Biochem Nutr. 2008;43(3):154–8. [Google Scholar]
40. Varga ZV, Kupai K, Szűcs G, Gáspár R, Pálóczi J, Faragó N, et al. MicroRNA-25-dependent up-regulation of NADPH oxidase 4 (NOX4) mediates hypercholesterolemia-induced oxidative/nitrative stress and subsequent dysfunction in the heart. J Mol Cell Cardiol. 2013;62:111–21. [Google Scholar]
41. Araghi A, Golshahi H, Baghban F, Abouhosseini Tabari M. Ameliorative action of farnesol on cyclophosphamide induced toxicity in mice. J Herbmed Pharmacol. 2018;7(1):37–43. [Google Scholar]
42. Lateef A, Rehman MU, Tahir M, Khan R, Khan AQ, Qamar W, et al. Farnesol protects against intratracheally instilled cigarette smoke extract-induced histological alterations and oxidative stress in prostate of wistar rats. Toxicol Int. 2013;20(1):35–42. [Google Scholar]
43. Li Y, Xie Z, Luo X, Wang X, Wang Y, Guo M, et al. Farnesol exerts protective effects against chronic sleep deprivation-induced cognitive impairment via activation SIRT1/Nrf2 pathway in the hippocampi of adult mice. Mol Nutr Food Res. 2023;67(11):e2200735. [Google Scholar]
44. Xue R, Wan Y, Sun X, Zhang X, Gao W, Wu W. Nicotinic mitigation of neuroinflammation and oxidative stress after chronic sleep deprivation. Front Immunol. 2019;10:2546. [Google Scholar]
45. Santhanasabapathy R, Vasudevan S, Anupriya K, Pabitha R, Sudhandiran G. Farnesol quells oxidative stress, reactive gliosis and inflammation during acrylamide-induced neurotoxicity: behavioral and biochemical evidence. Neurosci. 2015;308:212–27. [Google Scholar]
46. Chang HM, Wu UI, Lan CT. Melatonin preserves longevity protein (sirtuin 1) expression in the hippocampus of total sleep-deprived rats. J Pineal Res. 2009;47(3):211–20. [Google Scholar]
47. Gao S, Tang YY, Jiang L, Lan F, Li X, Zhang P, et al. H2S attenuates sleep deprivation-induced cognitive impairment by reducing excessive autophagy via hippocampal Sirt-1 in Wistar rats. Neurochem Res. 2021;46(8):1941–52. [Google Scholar]
48. Dang R, Wang M, Li X, Wang H, Liu L, Wu Q, et al. Edaravone ameliorates depressive and anxiety-like behaviors via Sirt1/Nrf2/HO-1/Gpx4 pathway. J Neuroinflammation. 2022;19(1):41. [Google Scholar]
49. Xu C, Song Y, Wang Z, Jiang J, Piao Y, Li L, et al. Pterostilbene suppresses oxidative stress and allergic airway inflammation through AMPK/Sirt1 and Nrf2/HO-1 pathways. Immun Inflamm Dis. 2021;9(4):1406–17. [Google Scholar]
50. Zhang J, Cai X, Zhang Q, Li X, Li S, Ma J, et al. Hydrogen sulfide restores sevoflurane postconditioning mediated cardioprotection in diabetic rats: role of SIRT1/Nrf2 signaling-modulated mitochondrial dysfunction and oxidative stress. J Cell Physiol. 2021;236(7):5052–68. [Google Scholar]
51. Song X, Long D. Nrf2 and ferroptosis: a new research direction for neurodegenerative diseases. Front Neurosci. 2020;14:267. [Google Scholar] [PubMed]
52. Wang X, Wang Z, Cao J, Dong Y, Chen Y. Melatonin alleviates acute sleep deprivation-induced memory loss in mice by suppressing hippocampal ferroptosis. Front Pharmacol. 2021;12:708645. [Google Scholar] [PubMed]
53. Yang Y, Wang X, Xiao A, Han J, Wang Z, Wen M. Ketogenic diet prevents chronic sleep deprivation-induced Alzheimer’s disease by inhibiting iron dyshomeostasis and promoting repair via Sirt1/Nrf2 pathway. Front Aging Neurosci. 2022;14:998292. [Google Scholar] [PubMed]
54. Souza DS, Barreto TO, Menezes-Filho JER, Heimfarth L, Rhana P, Rabelo TK, et al. Myocardial hypertrophy is prevented by farnesol through oxidative stress and ERK1/2 signaling pathways. Eur J Pharmacol. 2020;887:173583. [Google Scholar] [PubMed]
55. Rabkin SW. Considerations in understanding the coronary blood flow-left ventricular mass relationship in patients with hypertension. Curr Cardiol Rev. 2017;13(1):75–83. [Google Scholar] [PubMed]
56. Roullet JB, Luft UC, Xue H, Chapman J, Bychkov R, Roullet CM, et al. Farnesol inhibits L-type Ca2+ channels in vascular smooth muscle cells. J Biol Chem. 1997;272(51):32240–6. [Google Scholar] [PubMed]
57. Durço AO, de Souza DS, Heimfarth L, Miguel-Dos-Santos R, Rabelo TK, Oliveira Barreto T, et al. d-Limonene ameliorates myocardial infarction injury by reducing reactive oxygen species and cell apoptosis in a murine model. J Nat Prod. 2019;82(11):3010–9. [Google Scholar]
58. Gioda CR, de Oliveira Barreto T, Prímola-Gomes TN, de Lima DC, Campos PP, Capettini Ldos S, et al. Cardiac oxidative stress is involved in heart failure induced by thiamine deprivation in rats. Am J Physiol Heart Circ Physiol. 2010;298(6):H2039–45. [Google Scholar] [PubMed]
59. Zhang GX, Kimura S, Nishiyama A, Shokoji T, Rahman M, Yao L, et al. Cardiac oxidative stress in acute and chronic isoproterenol-infused rats. Cardiovasc Res. 2005;65(1):230–8. [Google Scholar] [PubMed]
60. Souza DS, Menezes-Filho JER, Santos-Miranda A, Jesus ICG, Silva Neto JA, Guatimosim S, et al. Calcium overload-induced arrhythmia is suppressed by farnesol in rat heart. Eur J Pharmacol. 2019;859:172488. [Google Scholar] [PubMed]
61. Demaurex N, Rosselin M. Redox control of mitochondrial calcium uptake. Mol Cell. 2017;65(6):961–2. [Google Scholar] [PubMed]
62. Purohit A, Rokita AG, Guan X, Chen B, Koval OM, Voigt N, et al. Oxidized Ca2+/calmodulin-dependent protein kinase II triggers atrial fibrillation. Circ. 2013;128(16):1748–57. [Google Scholar]
63. Sovari AA. Cellular and molecular mechanisms of arrhythmia by oxidative stress. Cardiol Res Pract. 2016;2016:9656078. [Google Scholar] [PubMed]
64. Tse G. Mechanisms of cardiac arrhythmias. J Arrhythm. 2016;32(2):75–81. [Google Scholar] [PubMed]
65. Wu YC, Wu GX, Huang HH, Kuo SM. Liposome-encapsulated farnesol accelerated tissue repair in third-degree burns on a rat model. Burns. 2019;45(5):1139–51. [Google Scholar] [PubMed]
66. Nagy F, Tóth Z, Daróczi L, Székely A, Borman AM, Majoros L, et al. Farnesol increases the activity of echinocandins against Candida auris biofilms. Med Mycol. 2020;58(3):404–7. [Google Scholar] [PubMed]
67. Fernandes RA, Monteiro DR, Arias LS, Fernandes GL, Delbem ACB, Barbosa DB. Biofilm formation by Candida albicans and Streptococcus mutans in the presence of farnesol: a quantitative evaluation. Biofouling. 2016;32(3):329–38. [Google Scholar] [PubMed]
68. Katragkou A, McCarthy M, Alexander EL, Antachopoulos C, Meletiadis J, Jabra-Rizk MA, et al. In vitro interactions between farnesol and fluconazole, amphotericin B or micafungin against Candida albicans biofilms. J Antimicrob Chemother. 2015;70(2):470–8. [Google Scholar] [PubMed]
69. Wang F, Liu Z, Zhang D, Niu X. In vitro activity of farnesol against vaginal Lactobacillus spp. Eur J Obstet Gynecol Reprod Biol. 2017;212:25–9. [Google Scholar] [PubMed]
70. Namba AM, Santos ELdS, Garcia MT, Ribeiro FdC, Figueiredo-Godoi LMA, Rossoni RD, et al. Farnesol as a potentiator of antimicrobial photodynamic inactivation on Enterococcus faecalis. Photodiagnosis Photodyn Ther. 2022;39:102928. [Google Scholar] [PubMed]
71. Castelo-Branco DSCM, Riello GB, Vasconcelos DC, Guedes GMM, Serpa R, Bandeira TJPG, et al. Farnesol increases the susceptibility of Burkholderia pseudomallei biofilm to antimicrobials used to treat melioidosis. J Appl Microbiol. 2016;120(3):600–6. [Google Scholar] [PubMed]
72. Lobo CI, Barbugli PA, Rocha GR, Klein MI. Topical application of 4'-hydroxychalcone in combination with tt-farnesol is effective against candida albicans and streptococcus mutans biofilms. ACS Omega. 2022;7(26):22773–86. [Google Scholar] [PubMed]
73. Horev B, Klein MI, Hwang G, Li Y, Kim D, Koo H, et al. pH-activated nanoparticles for controlled topical delivery of farnesol to disrupt oral biofilm virulence. ACS Nano. 2015;9(3):2390–404. [Google Scholar] [PubMed]
74. Bandara HMHN, Herpin MJ, Kolacny Jr D, Harb A, Romanovicz D, Smyth HDC. Incorporation of farnesol significantly increases the efficacy of liposomal ciprofloxacin against pseudomonas aeruginosa biofilms in vitro. Mol Pharm. 2016;13(8):2760–70. [Google Scholar] [PubMed]
75. Schnuch A, Uter W, Geier J, Lessmann H, Frosch PJ. Contact allergy to farnesol in 2021 consecutively patch tested patients. Results of the IVDK. Contact Dermatitis. 2004;50(3):117–21. [Google Scholar] [PubMed]
76. Gilpin S, Maibach H. Allergic contact dermatitis caused by farnesol: clinical relevance. Cutan Ocul Toxicol. 2010;29(4):278–87. [Google Scholar] [PubMed]
77. Adany I, Yazlovitskaya EM, Haug JS, Voziyan PA, Melnykovych G. Differences in sensitivity to farnesol toxicity between neoplastically-and non-neoplastically-derived cells in culture. Cancer Lett. 1994;79(2):175–9. [Google Scholar] [PubMed]
78. Yazlovitskaya EM, Melnykovych G. Selective farnesol toxicity and translocation of protein kinase C in neoplastic HeLa-S3K and non-neoplastic CF-3 cells. Cancer Lett. 1995;88(2):179–83. [Google Scholar] [PubMed]
79. Kovács R, Bozó A, Gesztelyi R, Domán M, Kardos G, Nagy F, et al. Effect of caspofungin and micafungin in combination with farnesol against Candida parapsilosis biofilms. Int J Antimicrob Agents. 2016;47(4):304–10. [Google Scholar]
80. Luft UC, Bychkov R, Gollasch M, Gross V, Roullet JB, McCarron DA, et al. Farnesol blocks the L-type Ca2+ channel by targeting the α1C subunit. Arterioscler Thromb Vasc Biol. 1999;19(4):959–66. [Google Scholar] [PubMed]
81. Constantino JA, Delgado-Rastrollo M, Pacha-Olivenza MA, Pérez-Giraldo C, Quiles M, González-Martín ML, et al. In vivo bactericidal efficacy of farnesol on Ti6Al4V implants. Rev Esp Cir Ortop Traumatol. 2016;60(4):260–6. [Google Scholar] [PubMed]
82. Bozó A, Domán M, Majoros L, Kardos G, Varga I, Kovács R. The in vitro and in vivo efficacy of fluconazole in combination with farnesol against Candida albicans isolates using a murine vulvovaginitis model. J Microbiol. 2016;54(11):753–60. [Google Scholar]
83. Yu JS, Kleckley TS, Wiemer DF. Synthesis of farnesol isomers via a modified wittig procedure. Org Lett. 2005;7(22):4803–6. [Google Scholar] [PubMed]
Cite This Article
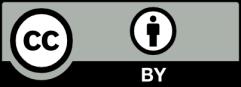