Open Access
ARTICLE
Effects of Exendin-4 on diabetic wounds: Direct action on proliferative phase of wound healing
1 Department of Diagnostics and Public Health, University of Verona, Verona, 37134, Italy
2 Department of Neurofarba, University of Florence, Florence, 50100, Italy
3 Department of Biology, University of Florence, Florence, 50100, Italy
* Corresponding Author: STEFANO BACCI. Email:
(This article belongs to the Special Issue: Understanding Cellular Mechanisms in Wound Healing During Therapeutic Interventions)
BIOCELL 2024, 48(12), 1751-1759. https://doi.org/10.32604/biocell.2024.057904
Received 30 August 2024; Accepted 25 October 2024; Issue published 30 December 2024
Abstract
Background: Impaired wound healing is one of the most well-known complications of type 2 diabetes mellitus. Experimental evidence suggested that treatment with Exendin-4, a glucagon-like peptide-1 agonist displaying a wide range of antidiabetic effects, can promote tissue regeneration. Objectives: Thus, this study aimed to examine the efficacy of topical treatment with Exendin-4 in accelerating wound healing in normoglycemic and hyperglycemic mice. Methods: For this purpose, two wounds inflicted on the back skin of 12 normo- and 12 hyperglycemic mice were injected intradermally with either saline solution or Exendin-4. Wounds were collected at the time of abrasion (T0), 48 h (T1), 96 h (T2), and 144 h (T3) and cryosections were analyzed by histological and histochemical methods. Results: In wounds treated with Exendin-4, an 85% reduction in size was observed at T3, and the thickness of the epidermis was statistically lower than in untreated wounds. The significative difference was observed in Exendin-4 treated wounds at T2 and T3 in the infiltration of granulocyte populations and at T3 in the degranulation index by mast cells and in the increase in vessels. Fibroblasts were significantly increased in Exendin-4 treated wounds of normo- and hyperglycemic mice at T3, which began to differentiate into myofibroblasts, from T2, and at T3 reached significant values. Conclusion: Taken all together, these results indicate that the topical administration of Exendin-4 had beneficial effects on the acceleration of the healing process of skin wounds in both normo- and hyperglycemic mice.Keywords
Diabetes mellitus is a chronic metabolic disorder, characterized by elevated blood glucose levels, or hyperglycemia, resulting from dysfunction in insulin secretion, insulin action, or both [1,2]. Impaired wound healing is a frequent and very severe problem in patients with diabetes mellitus, resulting from a complex pathophysiology involving vascular, neuropathic, immune, and biochemical components [3,4]. Essential stages of normal wound healing include inflammation, cell migration and proliferation, angiogenesis, and re-epithelialization [5].
Poor granulation tissue development and remodeling—events in which fibroblasts are crucial—are the hallmarks of diabetic skin ulcers [6], as well as a longer inflammatory phase during the healing process, potentially resulting in chronic wounds and pathological scarring [7–9]. In diabetic wounds, macrophages generate an overabundance of proinflammatory cytokines and fail to efficiently convert to anti-inflammatory macrophages [10,11]. Neutrophils also contribute to inflammation by producing cytotoxic enzymes, inflammatory mediators, and free radicals that worsen oxidative stress. In diabetic wounds, oxidative stress results in further tissue damage and delayed pathological repair. In addition, neutrophil extracellular traps, or NETs, are produced in excess by neutrophils in diabetic wounds, sustaining an inflammatory state that impedes wound healing [7]. Dysregulation of transcription factors and epigenetic mechanisms exacerbates pathological inflammation in diabetic wounds [10,12].
Chronic inflammation is a primary factor contributing to compromised healing in diabetic wounds, which also increases the risk of aberrant scarring and chronic wounds [13]. Scarring in diabetic wounds results from less collagen synthesis and alterations in the scar’s structure, adversely affecting optimal wound healing [14–16].
In diabetic wounds, inadequate angiogenesis significantly impedes wound healing, attributable to a deficiency of essential proangiogenic factors, an increase in antiangiogenic factors, a decrease in capillary maturation factors, miRNAs, and matrix metalloproteinases [7,17]. A lack of maturation factors slows down the healing process and increases the risk that the wound may become chronic or recurrent [7].
Exendin-4 (Exe-4) is a bioactive polypeptide of 39 amino acid residues (Fig. 1) isolated from the saliva of the Gila monster (Heloderma suspectum). Exe-4 is a natural potent and long-acting agonist of the glucagon-like peptide-1 (GLP-1), the insulinotropic intestinal peptide belonging to the incretin hormones, evaluated for the regulation of plasma glucose in type 2 diabetes [18–21]. GLP-1, by binding to the GLR-1 receptor (GLR-1R) stimulates the release of insulin on pancreatic beta cells [18–22]. GLP-1 exerts an important post-prandial insulinotropic effect, secreting approximately 60% of the post-prandial insulin. GLP-1 effects are secondary to the activation of pancreatic G-protein coupled receptors disseminated extra pancreas too. In patients with type 2 diabetes, the insulin-secreting efficiency of GLP-1 results is strongly reduced [20,21,23]. Exenatide, Exe-4 synthetic form, increases cyclic AMP levels in pancreatic acinar cells and acts as a GLP-1 agonist and incretin mimetic, enhancing insulin secretion in response to increased glucose levels; it also suppresses inappropriate glucagon secretion and slows gastric emptying. Thus, since Exe-4, mimics most of GLP-1 effects by activating GLP-1R, it is used as an anti-diabetic, especially for type 2 diabetes, as well as an anti-obesity agent [18–21].
Figure 1: Aminoacidic structure of Exendin-4 [24].
Experimental evidence suggests a possible role for Exe-4 in accelerating wound healing [25], both in diabetic rats [18] and in acute mouse wounds [23]. In particular, Exe-4 was reported to exert an angiogenic effect both in vitro and in vivo [26], and to enhance dermal and epidermal regeneration, also showing an increment of cell proliferation and tissue regeneration markers (Ki67 and TGF-β1, respectively) [18]. Moreover, an increment of fibroblast/myofibroblast populations was also reported after Exe-4 treatment in acute wounds, along with the mast cells (MCs) degranulation index and early activation of MCs, critical in wound healing acceleration.
Thus, in this study we investigated the effects of Exe-4 in acute wounds of normo- or hyperglycemic mouse model, analyzing parameters fundamental for the wound healing process: a) degranulation index of MCs, b) response of cellular infiltrate, c) angiogenesis, d) epithelization, e) reactivity of fibroblasts.
In this investigation, 24 male CD1 strain mice (Harlan Laboratories s.r.l., Udine, Italy), weighing between 18 and 20 g, were maintained in individual cages with unrestricted access to food and water. The mice were maintained at a stable temperature of 24°C–25°C and exposed to a regulated 12-h light-dark cycle.
Experimental and animal utilization protocols adhered to the National Institutes of Health Guide for the Care and Use of Laboratory Animals (NIH Publications no. 80-23, revised 1996). The Animal Care Committee of the Department of Pharmacology at the University of Florence approved the experimental protocols in accordance with the European Convention for the Protection of Vertebrate Animals used for Experimental and Other Scientific Purposes (ETS no. 123) and the European Communities Council Directive of 24 November 1986 (86/609/EEC). The authors assert that all measures were taken to reduce the number of animals utilized and to alleviate their suffering.
Diabetes was induced by injecting intraperitoneally (i.p.) streptozotocin (S0130, Sigma, Milan, Italy, 150 mg/kg, twice 48 h apart) to 12 mice, as reported before [18,27–29]. The presence of diabetes in the mice was verified after 3 and 10 days using a glucose refractometer. In non-diabetic mice classified as ‘healthy’, a fasting blood glucose level ranging from 80 to 100 mg/dL was recorded [30,31]. Nonfasting blood glucose >250 mg/dL was considered hyperglycemic. A glucose tolerance test (GTT) was performed to confirm hyperglycemia [32].
Following two weeks of hyperglycemia, hyperglycemic mice (n = 12) and age-matched normoglycemic mice (n = 12), maintained at a constant temperature of 37.5°C, were sedated with a single intraperitoneal injection of ketamine (80 mg/kg body weight) and xylazine (10 mg/kg body weight). Two circular abrasions, each with a diameter of 1 cm and a minimum separation of 1 cm, were created on the back by abrading undamaged skin with commercial sandpaper (grain size 68 µm; KWH Mirka Ltd., Oravaisfabrik, Finland) (Fig. 2A,B).
Figure 2: In vivo experiments set up. (A) Commercial cutter and sandpaper used for dermabrasion. (B) Macroscopical aspects of dorsal (upper; “saline”) and (lower; “Exe-4”) wounds in the normoglycemic mouse at the time of abrasion (T0). (C) Normo- and (D) hyperglycemic mice at T3 showing untreated (“saline”) and treated (“Exe-4”) wounds. Scale bar = 1 cm.
Based on their proximity to the mouse’s head, the wounds were defined as ‘upper’ and ‘lower’. Dermabrasion was performed until the epidermis was completely removed while avoiding deep wounds. Following the treatment, a minimal quantity of Streptosil (Boehringer Ingelheim Italia spa, Milan, Italy) was administered topically to avert the onset of infections. During the experiments, the wound remained exposed. Twenty microliters of saline solution (NT) or Exe-4 (62 ng; Sigma-Aldrich, St. Louis, MO, USA) were administered into the dermis of the upper and lower wounds of each animal, respectively. Immediately after the treatment, 2 normo- and 2 hyperglycemic mice were sacrificed by cervical dislocation. Pictures were taken from all the wounds before collecting skin biopsies (T0) and after 48 (2 days; T1), 96 (4 days; T2), and 144 h (6 days; T3) from wound induction. Biopsies were stored at −80°C until further evaluations.
The specimens embedded in frozen tissue medium (Killik; BioOptica, Milan, Italy, catalog number: 05-9801) were cryosectioned and post-fixed in cold acetone after being. Individual sections from each specimen were stained with hematoxylin and eosin (HE) (Sigma-Aldrich, Milan, Italy, catalog number HT 110132/H3136), Picrosirius Red (PSR) (Sigma-Aldrich, Milan, Italy, catalog number 365548) or paraldehyde-fuchsin (PF) (BioOptica, Milan, Italy, catalog number: 04-045872). HE was used to perform the analyses described below such as the detection of the cellular infiltrate response, while the measurement of epithelialization and the gross morphology of connective fibers was performed with PSR to specifically detect collagen fibers and PF for elastic fibers.
In other sections, indirect immunofluorescence techniques involving primary antibodies were used to detect various antigens (see Table 1). Appropriate fluorescein isothiocyanate labeled polyclonal rabbit (Sigma, Milan, Italy, catalog number: F0382) or mouse secondary antibodies (Sigma, Milan, Italy; catalog number: F2012) were incubated for 2 h at 37°C. The omission of the primary antibody and substitution with an irrelevant one were used as controls for the immunohistochemical reaction.
About the techniques of immunofluorescence that we used, to minimize intra- or extracellular background signals, nonspecific antigens were blocked by incubating the sample in bovine serum albumin (BSA) (Sigma-Aldrich, Milan, Italy, catalog number: A7641). Typical blocking times ranged from 30 min to approximately one hour. After incubation with the primary antibody, samples were washed 3 times for 5 min in a wash solution (PBS) to avoid background fluorescence. After incubation with the secondary antibody, samples were washed again with PBS and water. Samples were then mounted with a mounting medium characterized by low autofluorescence (Fluoromount: Sigma-Aldrich, Milan, Italy, catalog number: F4680).
The detection of mast cells or vessels was also performed using affinity cytochemistry techniques. Avidin is able to tag mast cell granules in a precise manner and with a high degree of awareness. The binding of the molecule is due to electrostatic attraction, which is why it has the same meaning as basophilia. However, the sensitivity of Avidin is far higher than that of standard microscopic dyes such as Giemsa or toluidine blue [33]. Griffonia (Bandeiraea) Simplicifolia Lectin II (GSL II) simplicifolia was used to stain endothelial cells. This is a glycoprotein that is formed of two subunits that are substantially equal in size, with each subunit including chains that are linked by disulfide and a binding site for N-acetylglucosamine residues that are linked by either α or β. The ability of GSL II to detect exclusively α- or β-linked N-acetylglucosamine residues on the nonreducing terminal of oligosaccharides has been considered to be a distinctive characteristic [34].
Images were captured using either a light microscope equipped with the tablet camera VisiCam TC10 (VWR International, Milan, Italy) or a Zeiss Axioskop microscope suitable for epifluorescence equipped with a digital camera (Zeiss, Jena, Germany).
For the epithelialization, wounds were digitally acquired at x 25 magnification, and the maximum epidermal thickness was measured in 10 epidermal tract frames for each specimen using ImageJ 1.37v software (National Institutes of Health, Bethesda, MD, USA), as previously reported [23,35]. Cellular infiltration evaluation, as shown in hematoxylin and eosin-stained sections, was graded on a 0–3 arbitrary scale for each biopsy site, as reported [23,35]. At least 10 nonoverlapping microscopical fields, from immunostained sections (one section per staining), were acquired at x 40 magnification. Immunoreactive cells, as well as MCs degranulation, were counted using ImageJ 1.37v software. For each comparison, the average value for each specimen was assumed as a sample unit. All differences were subjected to analysis of variance, and differences were considered statistically significant when p < 0.05. In the case of significant results among all experimental groups, the values at each time point were compared with controls by student’s t-test for unpaired values with two tails, and differences were considered statistically significant when p < 0.05.
Macroscopical evaluations of wounds
The initial wound area measured 0.78 cm2, derived from the assumption that the wounds were circular with a diameter of 1 cm (Fig. 2B). After six days (T3), the wounds’ size was once again measured to ascertain the effect of the treatments on the healing process. At this time point, we observed that both normo- (NUW) and hyperglycemic (HUW) un-treated (“saline”) wounds had an area of 0.50 cm2 (radius 0.4 cm) while in Exe-4-treated wounds the area was only of 0.12 cm2 (radius 0.2 cm), both in normo- (NTW) and hyperglycemic (HTW) mice. Thus, it resulted that untreated and Exe-4-treated wounds healed up to 36% and 85% respectively of their initial size (Fig. 2C,D).
Histological and immunohistochemical findings
The re-epithelialization or the thickness of the epidermis after the abrasion shows significant differences at T3 of both normo- or hyperglycemic mice treated with Exe-4 compared to untreated mice (Fig. 3). No significant differences were reported at T1 and T2 in Exe-4-treated or untreated wounds of both normo- and hyperglycemic mice.
Figure 3: Re-epithelialization of untreated and treated acute wounds in normo- and hyperglycemic mice. Measures of the thickness of epidermis at T0, T1, T2, and T3. Data are represented as mean ± SEM of 10 non-overlapping microscopical fields (*p < 0.05).
The evaluation of cellular infiltrate response performed by hematoxylin and eosin (HE) staining reported no differences in all conditions and time points analyzed (Fig. 4A,B). In accordance, the analysis of the content, organization, and orientation of the connective fibers, collagen fibers and elastic fibers by Picrosirius Red (PSR) or Paraldehyde Fuchsin (PF) staining respectively (Fig. 4C), did not show any difference.
Figure 4: Cellular infiltrate in untreated and treated acute wounds in normo- and hyperglycemic mice. (A) Cellular infiltrate evaluation at T0, T1, T2, and T3, graded on a 0–3 arbitrary scale. Data are represented as mean ± SEM of 10 non-overlapping microscopical fields. (B) Representative HE-stained sections of treated wounds collected at T3 from normo- (NTW) and hyperglycemic (HTW) mice: identification of the inflammatory infiltrate. (C) Representative sections of treated wounds collected at T3 from hyperglycemic (HTW) stained with picrosirius red (PSR, specific staining for collagen fibers) and paraldehyde fuchsin (PF, specific staining for connective fibers). Light microscopy, scale bar = 10 microns.
On the contrary, Ly6g immunostaining showed higher values in treated wounds, both for normo- and hyperglycemic mice from T1 to T3, when the difference resulted statistically significant (Fig. 5A). In treated wounds, figures of diapedesis were observed at T3 (Fig. 5B) (*p < 0.05).
Figure 5: Response of granulocytes in untreated and treated acute wounds in normo- and hyperglycemic mice. (A) Density of granulocytes at different time points of analysis. Data are represented as mean ± SEM of 10 non-overlapping microscopical fields (*p < 0.05). (B) Representative Ly6g+ fluorescence staining of granulocytes from treated wounds collected at T0 and T3 from injury from normo- (NTW) and hyperglycemic (HTW) mice. Fluorescence microscopy, scale bar = 10 microns.
At T2, in Exe-4-treated both normo- and hyperglycemic wounds, the degranulation index of MCs was increased compared to untreated ones (Fig. 6). At T3, the difference between treated and untreated wounds was statistically significant (*p < 0.05). Moreover, in this last condition, several cellular interactions between these MCs and fibroblast or vessels were observed (not shown).
Figure 6: Degranulation index of MCs in untreated and treated acute wounds in normo- and hyperglycemic mice. MCs degranulation was calculated by dividing the number of granules for the number of cells at T0, T1, T2 and T3. Data are represented as mean ± SEM of 10 non-overlapping microscopical fields (*p < 0.05).
Quantitative evaluation of the vessels shows differences starting from T2 in Exe-4-treated wounds compared to untreated ones. These differences are strengthened at T3, reaching levels of significance (Fig. 7) (*p < 0.05).
Figure 7: Angiogenesis in untreated and Exe-4-treated acute wounds in normo- and hyperglycemic mice. Angiogenesis was represented as the number of vessels for mm2 at T0, T1, T2 and T3. Data are represented as mean ± SEM of 10 non-overlapping microscopical fields (*p < 0.05).
The presence of fibroblasts did not increase significantly in treated normo- and hyperglycemic wounds up to T2 while the differences were statistically significant at T3 compared to untreated ones (Fig. 8A,B).
Figure 8: Response of fibroblasts in untreated and treated acute wounds in normo- and hyperglycemic mice. (A) Fibroblasts count at T0, T1, T2 and T3. Data are represented as mean ± SEM of 10 non-overlapping microscopical fields (*p < 0.05). (B) Representative Hsp47 fluorescence staining of fibroblasts from Exe-4-treated wounds collected at T0 and T3 from normo- (NTW) and hyperglycemic (HTW) mice. Fluorescence microscopy, scale bar = 10 µm.
The evaluation of the myofibroblast population revealed a statistically significant increment in both normo- and hyperglycemic Exe-4-treated wounds compared to untreated once only after 6 days (T3) (Fig. 9A). This suggests that both normo- and hyperglycemic Exe-4-treated wounds exhibit late myofibroblast development, as detected by ACTA 2 staining, which only becomes noticeable at T3 (Fig. 9B).
Figure 9: Response of myofibroblasts in untreated and treated acute wounds in normo- and hyperglycemic mice. (A) Myofibroblasts count at T2 and T3. Data are represented as mean ± SEM of 10 non-overlapping microscopical fields (*p < 0.05). (B) Representative Acta2 fluorescence staining of myofibroblasts from treated wounds collected at T3 from normo- (NTW) and hyperglycemic (HTW) mice. Fluorescence microscopy, scale bar = 10 microns.
In this manuscript we compare the effects of Exe-4, a natural potent and long-acting agonist of GLP-1, on the cellular response of acute wounds in normo- or hyperglycemic mice to assess whether the topical administration of this drug is able to accelerate the wound healing process.
The re-epithelialization data showed that the epidermis thickness reached normal values only after 6 days in Exe-4-treated wounds of both normo- and hyperglycemic mice. At the same time point of analysis, the re-epithelialization was still incomplete in untreated wounds.
These findings are probably related to the different cellular infiltrate responses in this context. In particular, mast cells play a coordinating activity in triggering cellular responses [33]. It has been previously reported that MCs express GLP1-R in their cytoplasm [35] leaving us to speculate that these cells are also capable of activating keratinocytes involved in the re-epithelialization process [5].
Analysis of HE-stained sections revealed that all the lesions had a corneum stratum, similar extent of both inflammatory cell infiltrate and connective fibers, regardless of the treatment received. About the fibers, the same results were obtained with the staining carried out for the evaluation of collagen fibers (Picrosirius Red) and elastic fiber (Paraldehyde Fuchsin). Conversely, the expansion of granulocyte populations in the treated lesions may be interpreted as an early inflammatory response induced by Exe-4 [18,23,35].
During inflammatory phase of normal wound healing, the secretion of histamine or serotonin by MCs induces vasodilation, involving the diapedesis of neutrophil granulocytes and monocytes, which are ready to differentiate into macrophages [36]. This leads to an increase in phagocytosis within the lesion, which is necessary to combat infections or damaged cells. The proliferative phase is initiated by the secretion of cytokines and growth factors by the leukocytes. Keratinocytes, among other cell types, contribute to this process by secreting inflammatory cytokines [37,38]. Additionally, it is important to acknowledge the involvement of additional molecules in the inflammatory phase, including cytokines, matrix proteins, and enzymes [39]. Chemokines are essential especially for attracting neutrophils and lymphocytes to promote the early stages of wound healing [36]. Indeed, the evidence that at 6 days in untreated wound the density of granulocytes was still lower compared to Exe-4-treated wound, supported this hypothesis.
In our study, MCs degranulation index reached the maximum values at T3 in treated wounds in both normo- and hyperglycemic mice, in accordance with the literature about the role of MCs in wound healing [33,40] or in diabetic wounds [41,42]. However, these data conflict with those of Tellechea et al. [43] supporting that appropriate wound healing depends on the existence of non-degranulated MCs in unwounded skin, thus therapies inhibiting MCs degranulation could improve wound healing in diabetes. Indeed, they reported that the inhibition of pre-wounding mast cell degranulation using disodium cromoglycate, a mast cell stabilizing agent, enhances wound healing in diabetic mice, but exerts no influence on nondiabetic mice.
Even though our results about the role of MCs in wound healing are in contrast with those of Tellechea and colleagues [43] we can hypothesize that, since MCs showed GLP1-R expression after Exe-4 treatment, this drug could activate MCs pathways. This hypothesis needs to be further investigated.
Regarding angiogenesis, the increment of vessels inside the dermis of the wounds treated with Exe-4, regardless of whether they are diabetic, demonstrates once again how the drug is able to act by stimulating the formation of new vessels and therefore confirming the previously published data, albeit under different conditions [18,26].
Among the various cells that participate in the inflammatory infiltrate there are fibroblasts whose secretion of extracellular matrix seems to be stimulated also by other factors present in the cutaneous microenvironment and by other cells that participate in the formation of infiltrates, such as MCs [33]. The fact that interactions have been found between these two cell types seems to confirm this hypothesis. Subsequent analysis has shown that fibroblasts actively participate in the stimulus caused by the drug, since, in the various experimental time points, their density is significantly higher in normo- and hyperglycemic treated wounds compared to untreated ones. In particular, the density of fibroblasts increases, already at T2, while a significative high number of these cells is observed at T3 in treated wounds. This data is probably closely related to the differentiation of these cell types in myofibroblasts. Thus, these cells are evidently affected by the stimulus evoked by Exe-4 as they differentiate only at T3 in treated wounds. This observation is surprising considering the significance of the various densities with respect to those obtained in untreated wounds.
In conclusion, it appears that Exe-4 accelerates wound healing kinetics acting particularly in the proliferative phase, regardless of whether the mice analyzed are normo- or hyperglycemic.
However, it is imperative to underscore that this investigation is subject to numerous limitations. First, most of the human type 2 diabetes is not clinically compatible with streptozotocin-induced diabetes, but this experimental model is considered valid for wound healing. Second, the closure of excisional wounds in this study may be influenced by wound contracture rather than the genuine effect of re-epithelialization. Third, the restricted quantity of mice. Fourth, when applied intradermally, it is impossible to exclude that Exe-4 has some degree of systemic bioavailability.
Acknowledgement: We would like to thank Prof. Laura Raimondi for her teachings and the following students for their help during the writing of the manuscript: Caciolli Adele, Macchi Sara, Magnolfi Vittorio, Napoli Alessandra, Nocentini Camilla, Raimondo Laura, Ricciardi Veronica, Ristori Sara, Salvo Maria, Sgattoni Chiara, Zepponi Francesca.
Funding Statement: The authors received no specific funding for this study.
Author Contributions: The authors confirm contribution to the paper as follows: study conception and design: Virginia Lotti, Stefano Bacci, Gaetano De Siena; data collection: Virginia Lotti, Stefano Bacci, Gaetano De Siena; analysis and interpretation of results: Virginia Lotti, Stefano Bacci; draft manuscript preparation: Virginia Lotti, Stefano Bacci. All authors reviewed the results and approved the final version of the manuscript.
Availability of Data and Materials: All data generated or analyzed during this study are included in this published article.
Ethics Approval: Experimental and animal utilization protocols adhered to the National Institutes of Health Guide for the Care and Use of Laboratory Animals (NIH Publications no. 80-23, revised 1996). The Animal Care Committee of the Department of Pharmacology at the University of Florence approved the experimental protocols in accordance with the European Convention for the Protection of Vertebrate Animals used for Experimental and Other Scientific Purposes (ETS no. 123) and the European Communities Council Directive of 24 November 1986 (86/609/EEC). The authors assert that all measures were taken to reduce the number of animals utilized and to alleviate their suffering.
Conflicts of Interest: Preliminary results were presented in a patent: Raimondi L, Bacci S, Mannucci E. Glucagon like peptide (GLP-1) agonists for use in topical applications. Filed with the Chamber of Commerce, Industry, Crafts, and Agriculture of Florence, application number: 00259 Application submission date 11.27.2012, Registration 19 May 2015, No. 001414583).
References
1. Banday MZ, Sameer AS, Nissar S. Pathophysiology of diabetes: an overview. Avicenna J Med. 2020;10(4):174–88. doi:10.4103/ajm.ajm_53_20. [Google Scholar] [PubMed] [CrossRef]
2. Diagnosis and classification of diabetes mellitus. Diabetes Care. 2014;37(Suppl 1):S81–90. Available from: https://diabetesjournals.org/care/article/37/Supplement_1/S81/37753/Diagnosis-and-Classification-of-Diabetes-Mellitus. [Accessed 2024]. [Google Scholar]
3. Greenhalgh DG. Wound healing and diabetes mellitus. Clin Plast Surg. 2003;30(1):37–45. doi:10.1016/S0094-1298(02)00066-4. [Google Scholar] [PubMed] [CrossRef]
4. Mieczkowski M, Mrozikiewicz-Rakowska B, Kowara M, Kleibert M, Czupryniak L. The problem of wound healing in diabetes-from molecular pathways to the design of an animal model. Int J Mol Sci. 2022;23(14):7930. doi:10.3390/ijms23147930. [Google Scholar] [PubMed] [CrossRef]
5. Raziyeva K, Kim Y, Zharkinbekov Z, Kassymbek K, Jimi S, Saparov A. Immunology of acute and chronic wound healing. Biomolecules. 2021;11(5):700. doi:10.3390/biom11050700. [Google Scholar] [PubMed] [CrossRef]
6. Tsourdi E, Barthel A, Rietzsch H, Reichel A, Bornstein SR. Current aspects in the pathophysiology and treatment of chronic wounds in diabetes mellitus. Biomed Res Int. 2013; 2013;2013:385641. doi:10.1155/2013/385641. [Google Scholar] [PubMed] [CrossRef]
7. Dasari N, Jiang A, Skochdopole A, Chung J, Reece EM, Vorstenbosch J, et al. Updates in diabetic wound healing, inflammation, and scarring. Semin Plast Surg. 2021;35(3):153–8. doi:10.1055/s-0041-1731460. [Google Scholar] [PubMed] [CrossRef]
8. Zhao R, Liang H, Clarke E, Jackson C, Xue M. Inflammation in chronic wounds. Int J Mol Sci. 2016;17(12):2085. doi:10.3390/ijms17122085. [Google Scholar] [PubMed] [CrossRef]
9. Holzer-Geissler JCJ, Schwingenschuh S, Zacharias M, Einsiedler J, Kainz S, Reisenegger P, et al. The impact of prolonged inflammation on wound healing. Biomedicines. 2022;10(4):856. doi:10.3390/biomedicines10040856. [Google Scholar] [PubMed] [CrossRef]
10. Boniakowski AE, Kimball AS, Jacobs BN, Kunkel SL, Gallagher KA. Macrophage-mediated inflammation in normal and diabetic wound healing. J Immunol. 2017;199(1):17–24. doi:10.4049/jimmunol.1700223. [Google Scholar] [PubMed] [CrossRef]
11. Wolf SJ, Melvin WJ, Gallagher K. Macrophage-mediated inflammation in diabetic wound repair. Semin Cell Dev Biol. 2021;119:111–8. doi:10.1016/j.semcdb.2021.06.013. [Google Scholar] [PubMed] [CrossRef]
12. den Dekker A, Davis FM, Kunkel SL, Gallagher KA. Targeting epigenetic mechanisms in diabetic wound healing. Transl Res. 2019;204:39–50. doi:10.1016/j.trsl.2018.10.001. [Google Scholar] [PubMed] [CrossRef]
13. Mahdavian Delavary B, van der Veer WM, van Egmond M, Niessen FB, Beelen RH. Macrophages in skin injury and repair. Immunobiology. 2011;216(7):753–62. doi:10.1016/j.imbio.2011.01.001. [Google Scholar] [PubMed] [CrossRef]
14. Spampinato SF, Caruso GI, De Pasquale R, Sortino MA, Merlo S. The treatment of impaired wound healing in diabetes: looking among old drugs. Pharmaceuticals. 2020;13(4):60. doi:10.3390/ph13040060. [Google Scholar] [PubMed] [CrossRef]
15. Sawaya AP, Stone RC, Brooks SR, Pastar I, Jozic I, Hasneen K, et al. Deregulated immune cell recruitment orchestrated by FOXM1 impairs human diabetic wound healing. Nat Commun. 2020;11(1):4678. doi:10.1038/s41467-020-18276-0. [Google Scholar] [PubMed] [CrossRef]
16. Huang Y, Kyriakides TR. The role of extracellular matrix in the pathophysiology of diabetic wounds. Matrix Biol Plus. 2020;6–7:100037. [Google Scholar] [PubMed]
17. Patel S, Srivastava S, Singh MR, Singh D. Mechanistic insight into diabetic wounds: pathogenesis, molecular targets and treatment strategies to pace wound healing. Biomed Pharmacother. 2019;112:108615. doi:10.1016/j.biopha.2019.108615. [Google Scholar] [PubMed] [CrossRef]
18. Roan JN, Cheng HN, Young CC, Lee CJ, Yeh ML, Luo CY, et al. Exendin-4, a glucagon-like peptide-1 analogue, accelerates diabetic wound healing. J Surg Res. 2017;208:93–103. doi:10.1016/j.jss.2016.09.024. [Google Scholar] [PubMed] [CrossRef]
19. Müller TD, Finan B, Bloom SR, D’Alessio D, Drucker DJ, Flatt PR, et al. Glucagon-like peptide 1 (GLP-1). Mol Metab. 2019;30:72–130. doi:10.1016/j.molmet.2019.09.010. [Google Scholar] [PubMed] [CrossRef]
20. Zhao X, Wang M, Wen Z, Lu Z, Cui L, Fu C, et al. GLP-1 receptor agonists: beyond their pancreatic effects. Front Endocrinol. 2021;12:721135. doi:10.3389/fendo.2021.721135. [Google Scholar] [PubMed] [CrossRef]
21. Dankner R, Murad H, Agay N, Olmer L, Freedman LS. Glucagon-like peptide-1 receptor agonists and pancreatic cancer risk in patients with type 2 diabetes. JAMA Network Open. 2024;7(1):e2350408-e. doi:10.1001/jamanetworkopen.2023.50408. [Google Scholar] [PubMed] [CrossRef]
22. Zheng Z, Zong Y, Ma Y, Tian Y, Pang Y, Zhang C, et al. Glucagon-like peptide-1 receptor: mechanisms and advances in therapy. Signal Transduct Target Ther. 2024;9(1):234. doi:10.1038/s41392-024-01931-z. [Google Scholar] [PubMed] [CrossRef]
23. Bacci S, Laurino A, Manni ME, Landucci E, Musilli C, De Siena G, et al. The pro-healing effect of exendin-4 on wounds produced by abrasion in normoglycemic mice. Eur J Pharmacol. 2015;764:346–52. doi:10.1016/j.ejphar.2015.06.056. [Google Scholar] [PubMed] [CrossRef]
24. PubChem Compound Summary for CID 56927919, Exendin 4.: National Center for Biotechnology Information. 2024. Available from: https://pubchem.ncbi.nlm.nih.gov/compound/Exendin-4. [Accessed 2024]. [Google Scholar]
25. Wolak M, Drobnik J, Bojanowska E. Exendin-4 differentially modulates essential functions of human dermal fibroblasts under normoglycemic and hyperglycemic conditions. J Physiol Pharmacol. 2021;72(3). [Google Scholar]
26. Kang H-M, Kang Y, Chun HJ, Jeong J-W, Park C. Evaluation of the in vitro and in vivo angiogenic effects of exendin-4. Biochem Biophys Res Commun. 2013;434(1):150–4. doi:10.1016/j.bbrc.2013.03.053. [Google Scholar] [PubMed] [CrossRef]
27. Novelli M, Bonamassa B, Masini M, Funel N, Canistro D, De Tata V, et al. Persistent correction of hyperglycemia in streptozotocin-nicotinamide-induced diabetic mice by a non-conventional radical scavenger. Naunyn Schmiedebergs Arch Pharmacol. 2010;382(2):127–37. doi:10.1007/s00210-010-0524-7. [Google Scholar] [PubMed] [CrossRef]
28. Tomić MA, Vucković SM, Stepanović-Petrović RM, Micov AM, Ugresić ND, Prostran MS, et al. Analysis of the antinociceptive interactions in two-drug combinations of gabapentin, oxcarbazepine and amitriptyline in streptozotocin-induced diabetic mice. Eur J Pharmacol. 2010;628(1–3):75–82. doi:10.1016/j.ejphar.2009.11.016. [Google Scholar] [PubMed] [CrossRef]
29. Tahara A, Matsuyama-Yokono A, Shibasaki M. Effects of antidiabetic drugs in high-fat diet and streptozotocin-nicotinamide-induced type 2 diabetic mice. Eur J Pharmacol. 2011;655(1–3):108–16. doi:10.1016/j.ejphar.2011.01.015. [Google Scholar] [PubMed] [CrossRef]
30. Jensen TL, Kiersgaard MK, Sørensen DB, Mikkelsen LF. Fasting of mice: a review. Lab Anim. 2013;47(4):225–40. doi:10.1177/0023677213501659. [Google Scholar] [PubMed] [CrossRef]
31. Kondo SY, Kropik J, Wong MA. Effect of bedding substrates on blood glucose and body weight in mice. J Am Assoc Lab Anim Sci. 2022;61(6):611–4. doi:10.30802/AALAS-JAALAS-22-000047. [Google Scholar] [PubMed] [CrossRef]
32. Zhu YX, Zhou YC, Zhang Y, Sun P, Chang XA, Han X. Protocol for in vivo and ex vivo assessments of glucose-stimulated insulin secretion in mouse islet β cells. STAR Protoc. 2021;2(3):100728. doi:10.1016/j.xpro.2021.100728. [Google Scholar] [PubMed] [CrossRef]
33. Bacci S. Fine regulation during wound healing by mast cells, a physiological role not yet clarified. Int J Mol Sci. 2022;23(3):1820. doi:10.3390/ijms23031820. [Google Scholar] [PubMed] [CrossRef]
34. Bomfim BCM, Azevedo-Silva J, Caminha G, Santos JPR, Pelajo-Machado M, de Paula Ayres-Silva J. Lectin-based carbohydrate profile of megakaryocytes in murine fetal liver during development. Sci Rep. 2023;13(1):6729. doi:10.1038/s41598-023-32863-3. [Google Scholar] [PubMed] [CrossRef]
35. Paroli G, Murciano N, Mancini C, Soldaini M, Rijli S, DeSiena G, et al. The role of mast cells in cellular modifications evoked by Exendin-4 in treated wounds: a preclinical study. J Wound Care. 2022;31(8):701–8. doi:10.12968/jowc.2022.31.8.701. [Google Scholar] [PubMed] [CrossRef]
36. Fernández-Guarino M, Hernández-Bule ML, Bacci S. Cellular and molecular processes in wound healing. Biomedicines. 2023;11(9):2526. doi:10.3390/biomedicines11092526. [Google Scholar] [PubMed] [CrossRef]
37. Cañedo-Dorantes L, Cañedo-Ayala M. Skin acute wound healing: a comprehensive review. Int J Inflamm. 2019;2019:3706315. [Google Scholar]
38. Peña OA, Martin P. Cellular and molecular mechanisms of skin wound healing. Nat Rev Mol Cell Biol. 2024;25(8):599–616. doi:10.1038/s41580-024-00715-1. [Google Scholar] [PubMed] [CrossRef]
39. Olson TS, Ley K. Chemokines and chemokine receptors in leukocyte trafficking. Am J Physiol Regul Integr Comp Physiol. 2002;283(1):R7–28. doi:10.1152/ajpregu.00738.2001. [Google Scholar] [PubMed] [CrossRef]
40. Ud-Din S, Wilgus TA, Bayat A. Mast cells in skin scarring: a review of animal and human research. Front Immunol. 2020;11:552205. doi:10.3389/fimmu.2020.552205. [Google Scholar] [PubMed] [CrossRef]
41. Albanesi C, Madonna S, Gisondi P, Girolomoni G. The interplay between keratinocytes and immune cells in the pathogenesis of psoriasis. Front Immunol. 2018;9:1549. doi:10.3389/fimmu.2018.01549. [Google Scholar] [PubMed] [CrossRef]
42. Dong J, Chen L, Zhang Y, Jayaswal N, Mezghani I, Zhang W, et al. Mast cells in diabetes and diabetic wound healing. Adv Therapy. 2020;37(11):4519–37. doi:10.1007/s12325-020-01499-4. [Google Scholar] [PubMed] [CrossRef]
43. Tellechea A, Leal EC, Kafanas A, Auster ME, Kuchibhotla S, Ostrovsky Y, et al. Mast cells regulate wound healing in diabetes. Diabetes. 2016;65(7):2006–19. doi:10.2337/db15-0340. [Google Scholar] [PubMed] [CrossRef]
Cite This Article
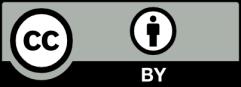
This work is licensed under a Creative Commons Attribution 4.0 International License , which permits unrestricted use, distribution, and reproduction in any medium, provided the original work is properly cited.