Open Access
COMMENTARY
A commentary on the interplay of biomaterials and cell adhesion: new insights in bone tissue regeneration
1 Departamento de Química, Universidad Nacional del Sur (UNS), Bahía Blanca, 8000, Argentina
2 INQUISUR-CONICET, Universidad Nacional del Sur (UNS), Bahía Blanca, 8000, Argentina
3 Departamento de Biología, Bioquímica y Farmacia, Universidad Nacional del Sur (UNS), Bahía Blanca, 8000, Argentina
* Corresponding Author: LUCIANO A. BENEDINI. Email:
BIOCELL 2024, 48(11), 1517-1520. https://doi.org/10.32604/biocell.2024.055513
Received 28 June 2024; Accepted 04 September 2024; Issue published 07 November 2024
Abstract
This article navigates the relationship between biomaterials and osteogenic cell adhesion, highlighting the importance of mimicking the physiological response for bone tissue regeneration. Within this spirit is an initial description of the interaction between osteoblasts and osteoprogenitor cells with the extracellular matrix, explaining the leading role of integrins and cadherins in cell adhesion, and the intracellular signaling pathways elicited. Additionally, there is a focus on the strategies of advanced biomaterials that foster osteogenesis by replicating the native environment, taking advantage of these known specific signaling pathways. The final remarks lay on the need for careful consideration of in-vitro and in-vivo complexities in biomaterial development.Keywords
Abbreviation List
FERM | 4.1 ezrin, radixin, moesin |
Axin-GSK3β | Axin and glycogen synthase kinase 3 beta |
BMP-2 | Bone morphogenetic protein 2 |
Cas | CT10 Regulator of Kinase-associated substrate |
ECM | Extracellular matrix |
ERK1/2 | Extracellular signal-regulated kinases 1 and 2 |
F-actin | Filamentous actin |
FAK | Focal adhesion kinase |
Grb2 | Growth factor receptor-bound protein 2 |
GTPase | Guanosine triphosphatase |
ILK | Integrin-linked kinase |
LRP5/6 | Low-density lipoprotein receptor-related proteins 5 and 6 |
mTOR | Mammalian target of rapamycin |
MAPK | Mitogen-activated protein kinase |
PI3K | Phosphoinositide 3-kinase |
AKT | Protein kinase B |
Rho | Ras homolog gene family |
p130 | Retinoblastoma-like protein 2 |
ROCK | Rho-associated protein kinase |
Src | Sarcoma family of non-receptor tyrosine kinases |
Ras | Small GTPases |
SOS | Son of sevenless |
Wnt | Wingless-related integration site proteins |
Osteogenic Cells’ Adhesion and Regulation
When considering regeneration, it becomes evident that there is no better response than that of our own body. Therefore, mimicking its strategies appears to be the best approach for developing successful biomaterials. This proposal requires a thorough and deep understanding of how this perfect system works. With this aim, a closer look at osteoprogenitor cells’ interactions with the extracellular matrix (ECM) is the natural first step. We will focus on cell adhesion, the kickoff of osteospecific differentiation, and subsequent osteogenesis. This communication occurs via intracellular signaling pathways related to cadherins and integrins receptors, both mechanotransducers. Cadherins facilitate cell-cell communication, while integrins are behind the interaction between cells and their ECM. The integrin receptor pathway presents two main types: those dependent on the focal adhesion kinase (FAK) complex and those independent of the FAK complex. Also, different phosphorylation sites on FAK can trigger cascades, such as the PI3K-Akt-mTOR, Ras-MAPK-ERK1/2, and p130Cas-RhoA GTPase pathways [1] (Fig. 1). The protagonism of FAK is beyond doubt regarding the intricate mechanisms involved in stiffness detection and surface texture of ECM by osteoprogenitor cells. Its three-domain structure of a kinase active domain capped by a FERM domain associated with the cell membrane at the focal adhesion and by the FAT domain on the opposite direction, related to the filamentous actin (F-actin) cytoskeleton fiber, is the cue. The native conformation hides this active domain. Still, in the presence of sufficiently high tension between the F-actin cytoskeleton and the focal adhesion, FAK reversely unfolds and elongates, exposing the kinase domain, causing its phosphorylation and subsequent complexation with Src protein-tyrosin kinase, leading to the activation of the FAK complex. If tensions cease or are insufficient, FAK will fold back and remain in its inactive low-energy conformation [2].
Figure 1: Intracellular signaling pathways related to integrins and cadherins receptors that could be activated in the biomaterial-cell interface. Adapted from [1]. The figure was created using free Inkscape software. Abbreviation list: axin and glycogen synthase kinase 3 beta (Axin-GSK3β), CT10 Regulator of Kinase-associated substrate (Cas), extracellular signal-regulated kinases 1 and 2 (ERK1/2), focal adhesion kinase (FAK), growth factor receptor-bound protein 2 (Grb2), guanosine triphosphatase (GTPase), integrin-linked kinase (ILK), low-density lipoprotein receptor-related proteins 5 and 6 (LRP5/6), mammalian target of rapamycin (mTOR), mitogen-activated protein kinase (MAPK), phosphoinositide 3-kinase (PI3K), protein kinase B (AKT), Ras homolog gene family member A (RhoA), Retinoblastoma-like protein 2 (p130), small GTPases (Ras), sarcoma family of non-receptor tyrosine kinases (Src), son of sevenless (SOS).
The subcellular mechanisms involved in cell adhesion present a collaborative association. In particular, the adhesion behavior of osteoblastic cells is interconnected rather than isolated. As such, some of the signaling molecules, scaffolding and cytoskeletal elements described at cell-ECM adhesions are also present in adherent junctions (AJ) of cell-cell adhesions, forming a converging signaling network referred to as “adhesive crosstalk” between integrins and cadherins [3]. Activated osteoblasts shape cadherin-connected monolayers after stimulation of β-integrin by ECM microenvironment via stimulation of common signaling proteins like FAK and Rho family GTPases [4]. Although the full extent of their regulation remains unclear, it is increasingly evident that their interaction affects cell spreading, growth, and survival.
Advanced Biomaterials Promoting Osteogenic Cell Adhesion
Functional tissue engineering scaffolds require biomaterials that closely mimic the native extracellular matrix of the target tissue. In this regard, numerous approaches focused on natural ECM molecules incorporation into biomaterials to promote cell adhesion, such as arginine–glycine–aspartic acid (RGD) ligands [5,6]. However, current research focuses on enhancing specific adhesion sites for endogenous cells involved in bone formation. Unlike the non-specific RGD peptide frequently used, a biomaterial could be modified with synthetic ligands with high specificity to osteogenic cells integrins (such as integrins α4β1 and αvβ3), showing increased mesenchymal stem cells and osteoblasts cells adhesion [7]. Moreover, certain soluble growth factors can orchestrate integrin and cadherin activation, giving tissue engineers more powerful tools to control cell fate. Such is the case of a recently developed polyelectrolyte matrix loaded with bone morphogenetic protein 2 (BMP-2), able to induce muscle to-osteoblast-transdifferentiation via N-cadherin/β3-integrin and Cadherin11/β1-integrin crosstalk to regulate transcriptional activities, after cell adhesion [8].
Considering the type of ligand presented and how the overall biomaterial structural design activates intracellular pathways and influences cell behavior is crucial. FAK complex function might be of interest as a target for biomaterial development since its sensibility to the substrate topographical and stiffness information is recognized [2,9]. At this point, there is a common consensus that nanofibrous topographies are much more effective for osteogenic cell adhesion, migration, and proliferation than flat surfaces. The leading hypothesis underlying the intracellular mechanism relies on the fact that osteoprogenitor cells and nanofibers have less focal adhesion, causing lower FAK activity, lower RhoA/ROCK activity, and in turn, decreasing actin polymerization required for translocation of YAP to the nucleus; this, ultimately, impairs the inhibition of Runx2, giving free road to initiate the synthesis of alkaline phosphatase (ALP), the induction of osteogenesis and bone maturation [7]. Moreover, recent findings demonstrate that interconnected porous structures enhance MSCs adhesion and spreading by synergic induction of subunit α5 of α5β1-integrin, cadherin11, and gap-junction gene expression, plus activation of Wnt pathway, involved in mechanical stretching-induced osteogenesis. The Osteonectin gene expression augmented, while the ALP activity increased by a factor of 4 compared to the non-porous substrate [10].
Integrin-related signaling pathways triggered by biomaterials are widely studied. However, adhesion doesn’t stop at integrins. Tissue engineers must expand their designs beyond the integrin family to fully unlock the potential of biomimetic approaches. In this regard, developing tunable biomaterials aimed at cadherin-related osteoblasts signaling remains a potential research area.
Along these lines, we discussed the immense importance of cognizance of the underlying mechanisms of cell behavior and communication with their microenvironment. We have emphasized that multiple pathways can be activated by the same surface parameter, providing a bird’s eye view of the beautiful complexity of intracellular communication.
The current challenge to our understanding is to investigate how specific biomaterial properties, when studied individually or combined, affect osteogenesis. A critical point is that many studies using biomaterials lack a comprehensive analysis of the biological pathways they activate. Addressing these gaps in research through methods like three-dimensional cell cultures providing more robust organotypic models and high-throughput fabrication with detailed characterization of arrays of engineered biomaterials will enhance our understanding of the key biomaterial parameters necessary for regenerating bone tissue with precision and predictability.
A final remark: in-vitro tests offer initial insights into biocompatibility but may only partially capture in-vivo complexities, such as systemic factors influence. Different processes such as neo-vascularization, immune response, and degradation are frequently slurred over. Also, proper animal selection is a sensitive issue when reflecting human responses, especially in load-bearing conditions assays. Lastly, navigating the regulatory landscape for new biomaterials represents a bureaucratic challenge that cannot be avoided when the goal is the translation of bench to bedside.
Acknowledgement: The authors acknowledge CONICET for the financial support and Dr. Javier Sartuqui for his valuable insight in reading the manuscript.
Funding Statement: The authors received no specific funding for this study.
Author Contributions: The authors confirm their contribution to the paper as follows: study conception and design: Luciano A. Benedini, A. Noel Gravina and Noelia D´Elía; draft manuscript preparation: Luciano A. Benedini, A. Noel Gravina; review and editing: Luciano A. Benedini, A. Noel Gravina; visualization: A. Noel Gravina, Noelia D´Elía; supervision: Paula Messina, Luciano A. Benedini. All authors reviewed the results and approved the final version of the manuscript.
Availability of Data and Materials: All data generated or analyzed during this study are included in this published article.
Ethics Approval: Not applicable.
Conflicts of Interest: The authors declare that they have no conflicts of interest to report regarding the present study.
References
1. Chen S, Guo Y, Liu R, Wu S, Fang J, Huang B, et al. Tuning surface properties of bone biomaterials to manipulate osteoblastic cell adhesion and the signaling pathways for the enhancement of early osseointegration. Colloids Surf B Biointerfaces. 2018;164(1):58–69. doi:10.1016/j.colsurfb.2018.01.022. [Google Scholar] [PubMed] [CrossRef]
2. Woodbury SM, Swanson WB, Mishina Y. Mechanobiology-informed biomaterial and tissue engineering strategies for influencing skeletal stem and progenitor cell fate. Front Physiol. 2023;14:1220555. doi:10.3389/fphys.2023.1220555. [Google Scholar] [PubMed] [CrossRef]
3. Barcelona-Estaje E, Dalby MJ, Cantini M, Salmeron-Sanchez M. You talking to me? Cadherin and integrin crosstalk in biomaterial design. Adv Healthc Mater. 2021;10(6):e2002048. doi:10.1002/adhm.202002048. [Google Scholar] [PubMed] [CrossRef]
4. Zhu S, Chen W, Masson A, Li YP. Cell signaling and transcriptional regulation of osteoblast lineage commitment, differentiation, bone formation, and homeostasis. Cell Discov. 2024;10(1):71. doi:10.1038/s41421-024-00689-6. [Google Scholar] [PubMed] [CrossRef]
5. Dhavalikar P, Robinson A, Lan Z, Jenkins D, Chwatko M, Salhadar K, et al. Review of integrin-targeting biomaterials in tissue engineering. Adv Healthc Mater. 2020;9(23):e2000795. doi:10.1002/adhm.202000795. [Google Scholar] [PubMed] [CrossRef]
6. Nitti P, Narayanan A, Pellegrino R, Villani S, Madaghiele M, Demitri C. Cell-tissue interaction: the biomimetic approach to design tissue engineered biomaterials. Bioengineering. 2023;10(10):1122. doi:10.3390/bioengineering10101122. [Google Scholar] [PubMed] [CrossRef]
7. Hao D, Liu R, Fernandez TG, Pivetti C, Jackson JE, Kulubya ES, et al. A bioactive material with dual integrin-targeting ligands regulates specific endogenous cell adhesion and promotes vascularized bone regeneration in adult and fetal bone defects. Bioact Mater. 2022;20(2):179–93. doi:10.1016/j.bioactmat.2022.05.027. [Google Scholar] [PubMed] [CrossRef]
8. Valat A, Fourel L, Sales A, Machillot P, Bouin AP, Fournier C, et al. Interplay between integrins and cadherins to control bone differentiation upon BMP-2 stimulation. Front Cell Dev Biol. 2023;10:1027334. doi:10.3389/fcell.2022.1027334. [Google Scholar] [PubMed] [CrossRef]
9. Vermeulen S, Tahmasebi Birgani Z, Habibovic P. Biomaterial-induced pathway modulation for bone regeneration. Biomaterials. 2022;283:121431. doi:10.1016/j.biomaterials.2022.121431. [Google Scholar] [PubMed] [CrossRef]
10. Edwards SD, Ganash M, Guan Z, Lee J, Kim YJ, Jeong KJ. Enhanced osteogenesis of mesenchymal stem cells encapsulated in injectable microporous hydrogel. Sci Rep. 2024;14(1):14665. doi:10.1038/s41598-024-65731-9. [Google Scholar] [PubMed] [CrossRef]
Cite This Article
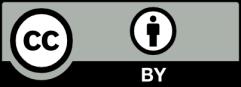
This work is licensed under a Creative Commons Attribution 4.0 International License , which permits unrestricted use, distribution, and reproduction in any medium, provided the original work is properly cited.