Open Access
ARTICLE
Tanshinone IIA inhibits NLRP3 activation and attenuates alveolar macrophage pyroptosis via the TREM2/β-catenin pathway
1 Department of Respiratory, Hunan Provincia Hospital of Integrated Traditional Chinese and Western Medicine (The Affiliated Hospital of Hunan Academy of Traditional Chinese Medicine), Changsha, 410006, China
2 Hunan Academy of Traditional Chinese Medicine, Changsha, 410006, China
* Corresponding Author: MIN LIU. Email:
(This article belongs to the Special Issue: Cell Death and Inflammation in Signaling and Diseases)
BIOCELL 2024, 48(10), 1475-1487. https://doi.org/10.32604/biocell.2024.053227
Received 28 April 2024; Accepted 28 August 2024; Issue published 02 October 2024
Abstract
Background: Alveolar macrophage pyroptosis exacerbates inflammatory lung diseases, and tanshinone IIA is known for its anti-inflammatory properties. Thus, understanding how tanshinone IIA affects alveolar macrophage pyroptosis is essential. Methods: NR8383 cells were exposed to lipopolysaccharide (LPS) and adenosine triphosphate (ATP). We assessed cell viability, pyroptosis, and the expression of triggering receptors expressed on myeloid cells 2 (TREM2), p-β-catenin, β-catenin, and pyroptosis-related factors. We also examined the interaction between tanshinone IIA and TREM2. Results: Co-stimulation with LPS and ATP significantly reduced NR8383 cell viability, increased pyroptosis, and upregulated pyroptosis-associated factors. Treatment with tanshinone IIA mitigated these effects. Tanshinone IIA was effectively bound to the TREM2 protein. Knockdown of TREM2 reduced its expression and the p-β-catenin and β-catenin levels, thereby reversing the protective effects of tanshinone IIA. Conversely, overexpression of TREM2 enhanced NR8383 cell viability, reduced pyroptosis, and suppressed pyroptosis-related factors following LPS and ATP co-stimulation. Furthermore, the NOD-like receptor family pyrin domain containing 3 (NLRP3) activation reversed the reduced pyroptosis induced by β-catenin overexpression. β-catenin knockdown reversed the protective effects of TREM2 overexpression, and activation of NLRP3 exacerbated pyroptosis in β-catenin knockdown cells. Conclusion: Tanshinone IIA inhibited NLRP3 activation and alleviated alveolar macrophage pyroptosis through the TREM2/β-catenin pathway.Keywords
Inflammatory lung diseases remain a significant threat to patients’ lives and health [1]. A key pathological feature of this disease is the activation of macrophages and the inflammatory response in the alveoli [2,3]. Dysfunctional macrophages in the lungs drive the pulmonary inflammatory response [4]. Lipopolysaccharide (LPS) can trigger an immune response [5], activating macrophages to secrete inflammatory mediators that maintain tissue homeostasis [6]. However, macrophage dysfunction and inflammation can result in increased pulmonary vascular wall thickness and smooth muscle cell proliferation, ultimately causing elevated pulmonary artery resistance and right ventricular failure [7]. Therefore, restoring normal macrophage function and reducing inflammation are critical in treating inflammatory lung disease.
Recent research has demonstrated that pyroptosis is crucial to the development and progression of inflammatory lung disease [8]. The production of pro-inflammatory cytokines and the activation of the intracellular inflammasome are two hallmarks of pyroptosis, which is a form of programmed cell death [9]. Among these inflammasomes, the NOD-like receptor family pyrin domain containing 3 (NLRP3) is particularly influential, as its activation induces inflammatory responses and macrophage pyroptosis [10]. NLRP3-mediated pyroptosis further damages vascular endothelial function, increasing susceptibility to vascular spasm and decreasing local blood flow, leading to pulmonary vascular diseases such as pulmonary arterial hypertension [11]. Thus, suppressing NLRP3 activation may reduce macrophage pyroptosis and alleviate inflammation.
The triggering receptor expressed on myeloid cells 2 (TREM2) is an immunomodulatory factor extensively expressed in immune cells, including macrophages and myeloid cells, and is essential for regulating inflammation and macrophage activity [12]. TREM2 signaling can phosphorylate β-catenin [13], which represents a key component of the Wnt signaling pathway crucial for immune defense, cell proliferation, and cell fate determination [14]. The activation status of NLRP3 is closely associated with TREM2 and β-catenin, influencing macrophage pyroptosis [15]. β-catenin can reduce NLRP3 expression, suppress inflammasome complex assembly, and mediate TREM2’s effects on NLRP3 inflammasome and macrophage pyroptosis [15]. However, the specific role of TREM2/β-catenin in inflammatory lung disease and its relationship with macrophage pyroptosis remains unclear.
Tanshinone IIA, one of the main components extracted from Salvia miltiorrhiza, contains several aromatic rings, aldehyde groups, and other functional structures [16]. It has been discovered to demonstrate widespread pharmacological activities and protective effects, including vasodilation, improved blood circulation, reduced blood viscosity, inhibition of platelet aggregation, and prevention of thrombosis [17,18]. Despite these benefits, tanshinone IIA may cause side effects such as diarrhea and itchy skin [19]. Recent studies have demonstrated that tanshinone IIA significantly attenuates cardiovascular disease progression due to its anti-inflammatory and antioxidant properties [20]. However, its efficacy and mechanisms in inflammatory lung disease are unclear.
This research was intended to examine the therapeutic potential of tanshinone IIA for NLRP3-mediated pyroptosis of alveolar macrophages. We investigated how LPS and adenosine triphosphate (ATP) induce cell pyroptosis and analyzed the role of TREM2 and the β-catenin pathway in this process. Our findings contribute to understanding the regulatory mechanisms of tanshinone IIA in inflammatory lung disease, which might help to find new therapeutic targets and develop effective therapeutic strategies to provide new clinical treatment options for patients.
The rat alveolar macrophage cell line NR8383 (Abiowell, AW-CNR441, Changsha, China) was cultured in F12K medium (Abiowell, AW-M004) containing 15% fetal bovine serum (FBS, Abiowell, AWC0219a), 2 mmol/L glutamine (Abiowell, AWC0035a), and 100 U/mL penicillin-streptomycin (Abiowell, AWH0529a) and grown in an incubator at 5% CO2 and 37°C. Additionally, mycoplasma contamination was not present in any of the cells used in this study.
LPS and ATP were engaged in regulating inflammatory responses [21]. Thus, we induced a pyroptosis model in NR8383 cells based on the methodology of Hu et al. [22]. The cells were classified into four groups: Control, LPS, ATP, and LPS + ATP. The LPS group was treated with 200 ng/mL LPS (MCE, HY-D1056, Jersey City, NJ, USA) for 48 h, the ATP group with 1 mmol/L ATP (MCE, HY-B2176) for 48 h, and the LPS + ATP group with both 200 ng/mL LPS and 1 mmol/L ATP for 48 h [23].
To assess how tanshinone IIA affects inflammatory NR8383 cells, we divided the cells into Control, LPS + ATP, Low, Medium, High, and Simvastatin groups. All groups, except the Control, were pretreated with 200 ng/mL LPS and 1 mmol/L ATP for 48 h. Following this pretreatment, the serum-free medium was substituted for the cell growth medium, and drugs (tanshinone IIA and Simvastatin) were added after 12 h. The cells were exposed to 0.6, 3.0, and 5.0 µM tanshinone IIA (T0154, Saint Paul, MN, USA) [24], representing Low, Medium, and High groups, respectively. The purity of tanshinone IIA was 98.5%. The Simvastatin group received 10 µM Simvastatin (MCE, HY-17502) [25], known for its anti-inflammatory effects. All treatments were incubated for 24 h.
To survey whether TREM2 is involved in the regulation of inflammatory NR8383 cell function by tanshinone IIA, the cells were classified into Control, LPS + ATP, Tanshinone IIA, Tanshinone IIA + si-NC, and Tanshinone IIA + si-TREM2 groups. Except for the Control group, all groups were pretreated with a combination of 200 ng/mL LPS and 1 mmol/L ATP for 48 h. In the Tanshinone IIA group, NR8383 cells were subjected to 3.0 µM tanshinone IIA for 24 h. In the Tanshinone IIA + si-NC and Tanshinone IIA + si-TREM2 group, NR8383 cells were transfected with si-NC (TTACGGCTTTCCGGAT) and small interfering RNA for TREM2 (si-TREM2, GCCAGCTGGCTGAGGGGCCATGCCAGCCGC), respectively. Then, the cells were first served with LPS and ATP for 48 h and then subjected to 3.0 µM tanshinone IIA for 24 h [24,26].
For the purpose of learning the effect of TREM2 overexpression on pyroptosis of inflammatory NR8383 cells, we grouped the cells into Control, LPS + ATP, oe-NC, and oe-TREM2 groups. Except for the control group, all groups were pretreated with a combination of 200 ng/mL LPS and 1 mmol/L ATP. Cells in the oe-NC and oe-TREM2 groups were transfected with oe-NC and an overexpressed TREM2 vector (oe-TREM2), respectively, for 48 h before LPS and ATP stimulation for another 48 h [24,26].
To determine whether β-catenin affects NR8383 pyroptosis by regulating NLRP3 activation, cells were divided into LPS + ATP, oe-NC, oe-β-catenin, and oe-β-catenin + Nigericin groups. All groups were treated with 200 ng/mL LPS and 1 mmol/L ATP for 48 h. The oe-β-catenin group was transfected with an overexpressed β-catenin vector (oe-β-catenin) for 48 h, ensued by LPS and ATP stimulation for 48 h. The oe-β-catenin + Nigericin group was transfected with oe-β-catenin for 48 h, pursued by LPS and ATP treatment for 48 h, and then treated with 6 µM Nigericin (Sigma-Aldrich, N7143-5MG, Shanghai, China) for 1 h.
To investigate the correlation between TREM2 and β-catenin in inflammatory NR8383 cells, the cells were divided into Control, oe-NC + si-NC, oe-TREM2 + si-NC, oe-TREM2 + si-β-catenin, oe-TREM2 + si-NC + Nigericin, and oe-TREM2 + si-β-catenin + Nigericin groups. All groups, except the Control, were pretreated with 200 ng/mL LPS and 1 mmol/L ATP. The oe-NC + si-NC group was co-transfected with oe-NC and si-NC for 48 h. The oe-TREM2 + si-NC group was co-transfected with oe-TREM2 and si-NC. The oe-TREM2 + si-β-catenin group was co-transfected with oe-TREM2 and si-β-catenin (GCTGATCTTGGACTTGATATT). The oe-TREM2 + si-NC + Nigericin group was co-transfected with oe-TREM2 and si-NC for 48 h, followed by 6 µM Nigericin for 1 h [27]. The oe-TREM2 + si-β-catenin + Nigericin group was co-transfected with oe-TREM2 and si-β-catenin for 48 h, followed by 6 µM Nigericin for 1 h.
In accordance with the manufacturer’s instructions, all transfections were accomplished with the Lipofectamine 2000 transfection reagent (Invitrogen, 11668-019, Carlsbad, CA, USA). The vectors used were obtained from HonorGene (Changsha, China).
Cell Counting Kit-8 (CCK-8, Abiowell, AWC0114a) was employed for assessing cell viability [28]. Briefly, the cells from the previously described groups were digested, counted, and inoculated into a 48-well plate at a density of 5 × 104 cells/well. Triplicate wells were set up. After cell culture and adhesion, CCK-8 (30 μL/well) reagent was added at the specified times post-treatment. Following a further 4 h incubation, the optical density (OD) at 450 nm was tested with a multifunctional microplate reader (Huisong, MB-530, Shenzhen, China).
The samples (1 × 105 cells/well density) were rinsed with phosphate-buffered saline (PBS, Abiowell, AWC0409) and collected by trypsin digestion (Abiowell, AWC0232). The samples were then resuspended in Caspase-1 probe FAM-YVAD-FMK working solution (AAT Bioquest, AAT-13483, Pleasanton, CA, USA) and mixed with propidium iodide (Abiowell, AWC0314a). The mixture was cultivated at 37°C in a direct-heat CO2 incubator (Mito Instruments, DH-160I, Shanghai, China) for 20 min in the dark. Within 1 h post-incubation, the samples were evaluated by flow cytometry (Beckman, A00-1-1102, Pasadena, CA, USA).
The proteins were isolated from the cell lysates of each group. Protein concentrations were examined using the BCA protein quantification kit (Abiowell, AWB0156). Proteins (20–30 μg) were mixed with 5× loading buffer (Abiowell, AWB0055), boiled for 5 min, and rapidly cooled on ice. The proteins were mixed with 5 × loading buffer, boiled for 5 min, and put into an ice box for quick cooling. The constant voltage of electrophoresis was 75 V for 130 min. Once the bromophenol blue reached the bottom of the gel, electrophoresis was stopped, and proteins were transferred to nitrocellulose (NC) membranes using a current of 300 mA. After transfer, the membranes were blocked with 5% skimmed milk at room temperature for 90 min. Additionally, abbreviations for antibodies are listed in Table 1. Next, membranes were cultivated with primary antibodies (Table 2) at 4°C overnight. On the subsequent day, membranes were fostered with secondary antibodies (Table 2) at room temperature for 90 min. An enhanced chemiluminescence (ECL) solution (Abiowell, AWB0005) was added and left for 1 min, followed by imaging with a chemiluminescent system (Clinx Science Instruments, ChemiScope6100, Shanghai, China).
Fluorescence intensity was evaluated using immunofluorescence. NR8383 cells were attached with 4% paraformaldehyde. Next, the samples were blocked with 0.3% Triton X-100 (Abiowell, AWT0107a) at 37°C, followed by 5% bovine serum albumin (BSA, Abiowell, AWT0206a) at 37°C. Primary antibody Caspase-1 (1:50, Proteintech, 22915-1-AP, Chicago, IL, USA) was incubated with the cells overnight at 4°C. The next day, cells were nurtured with goat anti-rabbit IgG (H + L) secondary antibody (1:200, Abiowell, AWS0005c) at room temperature for 1.5 h. Next, the nuclei were stained with DAPI working solution (Abiowell, AWI0429a) at 37°C for 10 min. The cells were then observed and imaged under a fluorescence microscope (Motic, BA410T, Xiamen, China). Fluorescence intensity was quantified by ImageJ software (National Institutes of Health, ImageJ 2.3.0, Bethesda, MD, USA).
Reverse transcription‑quantitative polymerase chain reaction (RT‑qPCR)
Total RNA from NR8383 cells was isolated using Trizol reagent (Thermo Fisher Scientific, 15596026, Waltham, MA, USA) following the manufacturer’s instructions. The RNA concentration used for RT-qPCR ranged from 250–350 ng/μL. The concentration of RNA was appraised by a NanoDrop spectrophotometer (Thermo Fisher Scientific, NanoDrop3300), and RNA integrity was surveyed by an Agilent Bioanalyzer (Agilent Technologies, Agilent 2100 Bioanalyzer, Santa Clara, CA, USA). It was verified that the RNA of the sample had high integrity with RNA integrity number (RIN) values of 8 or higher. Using the effective mRNA reverse transcription kit (Kangwei Century, CW2569, Beijing, China), total RNA was converted into cDNA. RT-qPCR was conducted using the QuantStudio1 system (ABI, QuantStudio1, Carlsbad, CA, USA) under the conditions: 95°C for 10 min (denaturation), pursued by 40 cycles of 95°C for 15 s and 60°C for 30 s (amplification). β-actin served as the internal reference gene. The 2−ΔΔCt method was utilized to quantify the mRNA levels of genes. Abbreviations for the genes are given in Table 1. The sequences of the specific primer sequences used are shown in Table 3.
The chemical structure of tanshinone IIA was predicted using PubChem (https://pubchem.ncbi.nlm.nih.gov/, accessed on 22 January, 2024) compound records. Molecular docking analysis of tanshinone IIA with the TREM2 protein was conducted using VINA 1.1.2 software (Scripps Research, VINA 1.1.2, San Diego, CA, USA), which utilized a semi-empirical free energy field to predict receptor-ligand binding energies. PyMOL 2.3 (Schrodinger, PyMOL 2.3, New York, NY, USA) was applied to visualize the ligands bound to the receptor-binding pocket and to forecast the receptor-ligand interactions and energies.
Drug affinity responsive target stability (DARTS)
First, TREM2 protein (0.5 μg/mL) was incubated with tanshinone IIA (5/20/40 μM) at room temperature for 1 h. Next, pronase (5 μg/mL, Solarbio, P8360, Beijing, China) was added and incubated for 15 min. The reaction was terminated by the addition of a supersampling buffer. After Coomassie Brilliant Blue (Beyotime, ST1119, Changsha, China) staining, sodium dodecyl sulfate polyacrylamide gel electrophoresis (SDS-PAGE) analysis was performed to show the binding of TREM2 and tanshinone IIA.
Statistical analysis of cellular experimental data was performed using GraphPad Prism 8 (8.0.2.263, San Diego, CA, USA). All experiments were conducted in six replicates. Measurement data were presented as mean ± standard deviation (SD). Data conforming to a normal distribution were compared between multiple groups using one-way analysis of variance (ANOVA) pursued by Tukey’s post hoc test or Bonferroni’s post hoc test. Statistical significance was determined as p < 0.05, with efforts made to avoid type I errors. The linear correlation between TREM2 and β-catenin and between TREM2 and p-β-catenin variables was evaluated using Pearson’s correlation test.
Combined stimulation of LPS and ATP promotes NR8383 cell pyroptosis
To explore the implications of LPS and ATP on NR8383 cells, we assessed NR8383 cell viability, pyroptosis, and protein expression of pyroptosis-related indicators following LPS and ATP treatments. The findings illustrated that the LPS and ATP groups all exhibited reduced NR8383 cell viability relative to the Control group. Additionally, the LPS + ATP group had lower NR8383 cell viability compared to the LPS or ATP groups (Fig. 1A). Flow cytometry revealed that pyroptosis was intensified in the LPS and ATP groups in contrast to the Control group. Moreover, NR8383 cell pyroptosis was elevated in the LPS + ATP group relative to the LPS or ATP groups (Fig. 1B). Moreover, the levels of IL-1β, Caspase-1, NLRP3, NLRC4, GSDMD-N, and ASC were assessed in NR8383 cells, and the findings demonstrated that after intervention with LPS or ATP alone, the levels of these factors were raised relative to the Control group, with the combination treatment of LPS and ATP being more effective than LPS or ATP alone (Fig. 1C). Additionally, after intervention with LPS or ATP alone, the level of Caspase-1 was enhanced as opposed to the Control group. Similarly, the combination intervention of LPS and ATP exacerbated the impact of LPS or ATP alone (Fig. 1D). These results indicated that combined stimulation of LPS and ATP promoted NR8383 cell pyroptosis.
Figure 1: Combined stimulation of LPS and ATP promotes NR8383 cell pyroptosis. (A) NR8383 cell viability was examined using CCK-8. (B) NR8383 cell pyroptosis was evaluated by flow cytometry. (C) IL-1β, Caspase-1, NLRP3, NLRC4, GSDMD-N, and ASC levels were measured by WB. (D) Caspase-1 expression was examined by immunofluorescence. *p < 0.05 vs. Control. #p < 0.05 vs. LPS. &p < 0.05 vs. ATP. n = 6.
Binding of tanshinone IIA and TREM2
TREM2 is known to attenuate NLRP3-mediated macrophage pyroptosis [15]. To further understand the role of tanshinone IIA in alleviating NR8383 cell pyroptosis, we analyzed its molecular structure using PubChem compound records (Fig. 2A) and performed molecular docking with TREM2 proteins. The binding energy was −7.5 kcal/mol, indicating a strong binding affinity of tanshinone IIA to the TREM2 protein. PyMOL analysis showed that tanshinone IIA binds stably to the TREM2 protein cavity and interacts with surrounding amino acids (Fig. 2B). Tanshinone IIA primarily interacts with TREM2 through hydrogen bonding and hydrophobic interactions (Fig. 2B). Specifically, tanshinone IIA forms a hydrogen bond with THR 88 and hydrophobic interactions with TRP 70, LEU 71, LEU 89, and PHE 74, enhancing its binding to the active site. Moreover, we also verified the binding of tanshinone IIA and TREM2 protein by applying DARTS in vitro experiments. Among them, the binding of 40 μM tanshinone IIA to TREM2 was particularly evident (Fig. 2C).
Figure 2: Binding of tanshinone IIA and TREM2. (A) Chemical structure of tanshinone IIA. (B) Molecular docking validation of tanshinone IIA binding to TREM2. (C) The binding of tanshinone IIA and TREM2 was validated by DARTS. *p < 0.05 vs. Pronase.
Tanshinone IIA alleviates NR8383 cell pyroptosis induced by combined stimulation of LPS and ATP
We further examined the effects of tanshinone IIA on NR8383 cell pyroptosis caused by combined LPS and ATP stimulation. Different concentrations of tanshinone IIA and Simvastatin reversed the diminished cell viability induced by LPS and ATP. Higher concentrations of tanshinone IIA exhibited stronger effects, with medium and high concentrations exhibiting significant improvements (Fig. 3A). Flow cytometry analysis indicated that NR8383 cell pyroptosis was augmented in the LPS + ATP group in contrast to the Control group. Treatment with different concentrations of tanshinone IIA reduced pyroptosis, with medium and high concentrations showing the most pronounced effects. Simvastatin also mitigated LPS- and ATP-induced pyroptosis (Fig. 3B). Western blot analysis revealed that tanshinone IIA at various concentrations reduced the elevated levels of IL-1β, Caspase-1, NLRP3, NLRC4, GSDMD-N, and ASC induced by LPS and ATP, with the medium and high concentrations of tanshinone IIA showing stronger effects. Simvastatin also decreased the expression of these pyroptosis-related proteins (Fig. 3C). Tanshinone IIA at 3.0 µM was selected for subsequent studies due to its similar efficacy to the 5.0 µM concentration. Control studies showed that ethanol alone did not cause cell death or protect against LPS and ATP-induced cell death (data not shown). These results confirmed that tanshinone IIA effectively alleviated NR8383 cell pyroptosis induced by combined LPS and ATP stimulation.
Figure 3: Tanshinone IIA alleviates NR8383 cell pyroptosis induced by combined stimulation of LPS and ATP. (A) NR8383 cell viability was examined using CCK-8. (B) NR8383 cell pyroptosis was tested by flow cytometry. (C) IL-1β, Caspase-1, NLRP3, NLRC4, GSDMD-N, and ASC levels were evaluated by WB. *p < 0.05 vs. Control. #p < 0.05 vs. LPS + ATP. n = 6.
Tanshinone IIA alleviates NR8383 cell pyroptosis induced by combined stimulation of LPS and ATP via TREM2/β-catenin/NLRP3
We investigated whether tanshinone IIA regulates NLRP3-mediated NR8383 cell pyroptosis via the TREM2/β-catenin pathway. β-catenin, a crucial downstream element of TREM2, can be phosphorylated by TREM2 at the Ser675 site [15]. The relative mRNA expression of TREM2 and CTNNB1 (β-catenin mRNA), as well as the protein expression of TREM2, p-β-catenin, and β-catenin were diminished in the LPS + ATP group relative to the Control group. Tanshinone IIA intervention elevated TREM2 level and β-catenin phosphorylation. Transfection with si-TREM2 diminished the mRNA and protein levels of TREM2 and decreased the protein levels of p-β-catenin and β-catenin without affecting β-catenin mRNA levels, compared to the Tanshinone IIA + si-NC group (Fig. 4A,B).
Figure 4: Tanshinone IIA alleviates NR8383 cell pyroptosis induced by combined stimulation of LPS and ATP via TREM2/β-catenin/NLRP3. (A) Relative mRNA expression of TREM2 and CTNNB1 was examined by RT‑qPCR. (B) The levels of TREM2, p-β-catenin, and β-catenin were assayed by WB. (C) Assessment of NR8383 cell viability using CCK-8 assay. (D) Flow cytometry analysis of NR8383 cell pyroptosis. (E) Measurement of IL-1β, Caspase-1, NLRP3, NLRC4, GSDMD-N, and ASC levels by WB. *p < 0.05 vs. Control. #p < 0.05 vs. LPS + ATP. &p < 0.05 vs. Tanshinone IIA + si-NC. n = 6.
Moreover, tanshinone IIA reversed the decreased NR8383 cell viability and increased pyroptosis caused by LPS and ATP. Knockdown of TREM2 reduced cell viability and increased pyroptosis relative to the Tanshinone IIA + si-NC group (Fig. 4C,D). Furthermore, TREM2 knockdown elevated the levels of IL-1β, Caspase-1, NLRP3, NLRC4, GSDMD-N, and ASC in contrast to the Tanshinone IIA + si-NC group (Fig. 4E). These findings indicated that tanshinone IIA alleviated NLRP3-mediated NR8383 cell pyroptosis through the TREM2/β-catenin pathway.
TREM2 overexpression inhibits NR8383 cell pyroptosis induced by combined stimulation of LPS and ATP
According to the results, the knockdown of TREM2 attenuated the protective effects of tanshinone IIA. To investigate the independent role of TREM2, NR8383 cells were transfected with an oe-TREM2 plasmid. Relative to the oe-NC group, the oe-TREM2 group exhibited elevated TREM2 levels, confirming successful plasmid transfection (Fig. 5A). TREM2 overexpression enhanced NR8383 cell viability, reduced cell pyroptosis, and suppressed levels of IL-1β, Caspase-1, NLRP3, NLRC4, GSDMD-N, and ASC in contrast to the oe-NC group (Fig. 5B–D). These findings clarified that TREM2 overexpression inhibited pyroptosis caused by concurrent LPS and ATP stimulation in NR8383 cells. Additionally, the impact of TREM2 overexpression on β-catenin and p-β-catenin levels was investigated. TREM2 overexpression reversed the reduction in β-catenin and p-β-catenin levels induced by LPS and ATP stimulation (Fig. 5E). A positive correlation between TREM2 and β-catenin and between TREM2 and p-β-catenin was verified by Pearson’s analysis (Fig. 5F). These results suggested that TREM2 mitigated NR8383 cell pyroptosis induced by simultaneous LPS and ATP stimulation by upregulating β-catenin expression.
Figure 5: TREM2 overexpression inhibits NR8383 cell pyroptosis induced by combined stimulation of LPS and ATP. (A) Verification of TREM2 overexpression by WB. (B) Assessment of NR8383 cell viability using CCK-8 assay. (C) Flow cytometry analysis of NR8383 cell pyroptosis. (D) Measurement of IL-1β, Caspase-1, NLRP3, NLRC4, GSDMD-N, and ASC levels by WB. (E) WB analysis of β-catenin and p-β-catenin levels. (F) Demonstration of the correlation between TREM2 and β-catenin and between TREM2 and p-β-catenin by Pearson’s correlation coefficient. *p < 0.05 vs. Control. #p < 0.05 vs. oe-NC. n = 6.
β-catenin affects NR8383 cell pyroptosis by regulating NLRP3 activation
To elucidate the role of β-catenin in NR8383 cell pyroptosis, we overexpressed β-catenin. The oe-β-catenin group showed elevated β-catenin and p-β-catenin levels with no notable change in TREM2 relative to the oe-NC group, confirming successful transfection and indicating that β-catenin overexpression did not influence TREM2 levels (Fig. 6A,B). Additionally, β-catenin overexpression upregulated the expression of its major target genes (CyclinD1, c-Myc, and TCF-1) (Fig. 6C). Moreover, β-catenin overexpression enhanced NR8383 cell viability and reduced cell pyroptosis compared to the oe-NC group. Conversely, activation of NLRP3 decreased NR8383 cell viability and increased cell pyroptosis relative to the oe-β-catenin group (Fig. 6D,E). Furthermore, NLRP3 activation reversed the reduced levels of IL-1β, Caspase-1, NLRP3, NLRC4, GSDMD-N, and ASC caused by β-catenin overexpression (Fig. 6F). These results indicated that β-catenin regulated NR8383 cell pyroptosis through modulation of NLRP3 activation.
Figure 6: β-catenin affects NR8383 cell pyroptosis by regulating NLRP3 activation. (A–B) Verification of β-catenin overexpression by RT-qPCR and WB. (C) Relative expression of CyclinD1, c-Myc, and TCF-1 evaluated by RT-qPCR. (D) Assessment of NR8383 cell viability using CCK-8 assay. (E) Flow cytometry evaluation of NR8383 cell pyroptosis. (F) Measurement of IL-1β, Caspase-1, NLRP3, NLRC4, GSDMD-N, and ASC levels by WB. *p < 0.05 vs. oe-NC. #p < 0.05 vs. oe-β-catenin. n = 6.
TREM2 inhibits NR8383 cell pyroptosis by regulating β-catenin protein
We further explored the regulatory mechanisms involving TREM2 and β-catenin. Initially, we examined the relative expression of CyclinD1, c-Myc, and TCF-1, key target genes in the β-catenin signaling pathway. Results showed that oe-TREM2 elevated the levels of CyclinD1, c-Myc, and TCF-1 as opposed to the oe-NC + si-NC group. Subsequent transfection with si-β-catenin markedly lessened the levels of these genes. Notably, there was no remarkable difference observed between the oe-TREM2 + si-NC group and the oe-TREM2 + si-NC + Nigericin group, nor between the oe-TREM2 + si-β-catenin group and the oe-TREM2 + si-β-catenin + Nigericin group, indicating that NLRP3 activation did not impact the levels of these genes (Fig. 7A).
Figure 7: TREM2 inhibits NR8383 cell pyroptosis by regulating β-catenin protein. (A) Relative expression of CyclinD1, c-Myc, and TCF-1 assessed by RT-qPCR. (B) Relative expression of TREM2 and CTNNB1 tested by RT-qPCR. (C) Protein expression of TREM2, p-β-catenin, and β-catenin examined by WB. (D) NR8383 cell viability was measured using CCK-8. (E) NR8383 cell pyroptosis was analyzed by flow cytometry. (F) Protein expression of IL-1β, Caspase-1, NLRP3, NLRC4, GSDMD-N, and ASC examined by WB. *p < 0.05 vs. oe-NC + si-NC. #p < 0.05 vs. oe-TREM2 + si-NC. &p < 0.05 vs. oe-TREM2 + si-NC + Nigericin. @p < 0.05 vs. oe-TREM2 + si-β-catenin. n = 6.
Additionally, TREM2 expression and the levels of p-β-catenin and β-catenin were elevated in the oe-TREM2 + si-NC group in contrast to the oe-NC + si-NC group, with no impact on β-catenin mRNA levels. Conversely, si-β-catenin transfection decreased both β-catenin mRNA and protein levels and β-catenin phosphorylation without affecting TREM2 expression. There was no remarkable difference observed with the addition of Nigericin relative to the oe-TREM2 + si-NC group, nor with the addition of Nigericin as opposed to the oe-TREM2 + si-β-catenin group, indicating that NLRP3 activation did not impact TREM2 levels or the expression of CTNNB1, p-β-catenin, and β-catenin (Fig. 7B,C). These results suggested that TREM2 regulated β-catenin at the protein level instead of the mRNA level, and both β-catenin and NLRP3 may serve as downstream targets of TREM2.
Knockdown of β-catenin or activation of NLRP3 reversed the enhanced NR8383 cell viability caused by TREM2 overexpression, indicating that β-catenin knockdown or NLRP3 activation inhibited the protective effect of TREM2 overexpression on NR8383 cell viability. Additionally, the knockdown of β-catenin exacerbated the suppression of activated NLRP3 on NR8383 cell viability (Fig. 7D). In contrast to the oe-TREM2 + si-NC group, either β-catenin knockdown or NLRP3 activation led to increased NR8383 cell pyroptosis. Furthermore, NR8383 cell pyroptosis was enhanced in the oe-TREM2 + si-β-catenin + Nigericin group relative to the oe-TREM2 + si-β-catenin group (Fig. 7E). Si-β-catenin transfection or Nigericin addition reversed the diminished levels of IL-1β, Caspase-1, NLRP3, NLRC4, GSDMD-N, and ASC caused by TREM2 overexpression. Knockdown of β-catenin exacerbated the elevated expression of these proteins induced by NLRP3 activation (Fig. 7F). These findings indicated that β-catenin knockdown reversed the protective function of TREM2 overexpression on NR8383 cell pyroptosis, while NLRP3 activation further enhanced TREM2/β-catenin-mediated NR8383 cell pyroptosis. Thus, TREM2 could inhibit NLRP3-mediated NR8383 cell pyroptosis by promoting β-catenin protein phosphorylation.
In recent years, understanding the complex mechanisms underlying inflammatory lung diseases has been a key focus of research. Clarifying the specific details of alveolar macrophage pyroptosis is crucial for the management and prevention of these conditions. Herein, our present study demonstrated that tanshinone IIA interacted with TREM2 protein, enhancing β-catenin phosphorylation, which inhibited NLRP3 expression and effectively reduced NR8383 cell pyroptosis.
LPS contributes significantly to infection by triggering an immune response that can lead to inflammation and various pathological effects [5]. In BV2 cells, a macrophage cell line isolated from mice hippocampal tissue, LPS has been shown to induce pyroptosis mediated by NLRP3 [29]. ATP, a fundamental intracellular energy molecule, is a primary energy source for cells and also participates in the mediation of pyroptosis [30]. Inflammatory processes frequently coincide with pyroptosis, while tanshinone IIA is noted for its potent anticancer and anti-inflammatory properties [31]. Therefore, this study investigated how simultaneous exposure to LPS and ATP induced pyroptosis in NR8383 cells and explored the potential therapeutic benefits of tanshinone IIA in this context.
Tanshinone IIA has been shown to reduce inflammatory mediators released from macrophages, such as cyclooxygenase (COX) and tumor necrosis factor-α (TNF-α), which attenuates the inflammation [32,33]. In LPS-mediated RAW264.7 macrophages, tanshinone IIA performs its anti-inflammatory action through miRNAs and the toll-like receptor 4-nuclear factor-κB (TLR4-NF-κB) pathway [34]. Additionally, tanshinone IIA has demonstrated efficacy in reducing inflammation in the pulmonary vasculature by inhibiting macrophage migration and the expression of inflammatory factors [35,36], suggesting its role in modulating pulmonary macrophage function and inflammatory responses. Pyroptosis could be regulated by key players like NLRP3 and NLRC4 [37,38]. NLRP3 and NLRC4 can be activated by LPS stimulation and affect Caspase-1 expression [39]. Caspase-1 also cleaves GSDMD into GSDMD-N, which forms pores in cell membranes, leading to cell lysis and pyroptosis [40]. Caspase-1, belonging to the caspase family, plays a crucial role in immune regulation [41]. IL-1β is pivotal in inflammatory processes [42]. GSDMD-N acts as an executor protein of inflammatory pyroptosis, its activation and cleavage are linked to Caspase-1 activity, inducing inflammatory cell death [40]. Additionally, research has shown that Didymin alleviates microglia cell pyroptosis and neuroinflammation through the Asc/Caspase-1/GSDMD pathway [43]. In this work, we observed that these pyroptosis-related indicators were key targets regulated by tanshinone IIA in NR8383 cells under conditions of LPS and ATP stimulation. These findings highlighted the potential of tanshinone IIA to effectively mitigate LPS- and ATP-induced pyroptosis in NR8383 cells.
In this study, tanshinone IIA was observed to form a stable hydrogen bond with THR88 of the TREM2 protein. The hydrophobic moiety of tanshinone IIA interacted hydrophobically with TRP70, LEU71, LEU89, and PHE74 residues of THR88, facilitating its binding to TREM2. TREM2 is an immunomodulatory protein expressed in macrophages and plays a role in neuroinflammatory responses and immune regulation [12]. β-catenin, an essential transcriptional regulator involved in cellular communication and adhesion, is closely linked to TREM2 [44]. Initiation of the β-catenin can inhibit the production of the inflammatory mediator NLRP3 [15]. In addition, activation of NLRP3 reversed the enhanced NR8383 cell viability and reduced cell pyroptosis caused by overexpression of β-catenin, suggesting that β-catenin modulated NR8383 cell pyroptosis by regulating NLRP3 activation. Additionally, the knockdown of β-catenin reversed the elevated NR8383 cell viability and diminished cell pyroptosis induced by overexpression of TREM2. Furthermore, further application of the NLRP3 activator Nigericin disrupted the beneficial effect of TREM2 overexpression on NR8383 cell pyroptosis. These findings indicated that TREM2 induced β-catenin phosphorylation, thereby effectively inhibiting NLRP3-mediated NR8383 cell pyroptosis. Dexamethasone upregulates macrophage piezo-type mechanosensitive ion channel component 1 (PIEZO1) via serum and glucocorticoid-regulated kinase 1 (SGK1), suppressing inflammation and enhancing reactive oxygen species (ROS) and apoptosis [45]. Aspirin inhibits macrophage inflammation by altering metabolic phenotypes in periodontitis [46]. Cyclosporine A inhibits T-cell function, thereby limiting macrophage accumulation [47]. Similarly, we observed that tanshinone IIA increased TREM2 protein activity, enhanced β-catenin phosphorylation, and suppressed NLRP3 expression in LPS- and ATP-stimulated macrophages (NR8383 cells). The suppression of tanshinone IIA on LPS- and ATP-induced NR8383 cell pyroptosis was attenuated upon TREM2 knockdown. These results demonstrated that tanshinone IIA protected against NLRP3-mediated NR8383 cell pyroptosis through the TREM2/β-catenin axis. Furthermore, tanshinone IIA showed promise in alleviating lung inflammatory diseases by attenuating pyroptosis in alveolar macrophages, suggesting its potential as a treatment for such conditions.
We also observed that TREM2 specifically regulated the protein level of β-catenin without impacting its mRNA expression. Several explanations could account for this observation: The 5′ untranslated region (5′-UTR) of TREM2 is subject to translational regulation [48], suggesting TREM2 may influence β-catenin expression by modulating protein synthesis rates or stability. The R47H variant of TREM2 is known to alter protein stability [49], implying that changes in β-catenin mRNA levels may not be significant, and TREM2 might instead modulate β-catenin protein levels through effects on its degradation pathway. TREM2 interacts with various proteins [15,50,51], which could either promote or inhibit the assembly of β-catenin complexes, thereby influencing its protein level. Such interactions may involve regulating β-catenin’s transcriptional activity and its cellular localization.
However, we must acknowledge several limitations of this study. Firstly, our experiments were conducted using NR8383 cells, a rat alveolar macrophage cell line. Therefore, further investigations using primary alveolar macrophages or in vivo studies are essential to validate our findings. Additionally, more research and experimental evidence are needed to elucidate the binding mechanism of tanshinone IIA with TREM2. Furthermore, exploring the effects of tanshinone IIA on other inflammatory signaling pathways and evaluating its efficacy in animal models and clinical settings will enhance our understanding of its therapeutic potential.
In conclusion, our study demonstrated that tanshinone IIA exerted a protective effect against NR8383 cell pyroptosis through the TREM2/β-catenin/NLRP3 pathway. These findings hold promise for developing novel therapeutic strategies for patients with inflammatory lung diseases. Tanshinone IIA may serve as a potential treatment for lung inflammatory diseases. Future research directions include evaluating the therapeutic effectiveness of tanshinone IIA in patients with inflammatory lung diseases and validating its effectiveness in diverse physiological contexts using animal models. Furthermore, investigating the detailed regulatory mechanisms of tanshinone IIA within the TREM2/β-catenin/NLRP3 pathway in macrophage pyroptosis will be crucial for advancing our understanding of its therapeutic potential.
Acknowledgement: None.
Funding Statement: This work was supported by the National Natural Science Foundation of China Youth Fund (Nos. 82305214 and 82004306); Excellent Youth Project of Hunan Provincial Department of Education (No. 22B0394); Health Research Project of Hunan Provincial Health Commission (No. W20243178); Hunan Provincial Administration of Traditional Chinese Medicine General Guidance Project (No. E2022018); and Hunan Natural Science Foundation Project (No. 2023JJ40401).
Author Contributions: Min Liu: conceptualization, data curation, formal analysis, funding acquisition, project administration, and writing of original draft; Xia Li: data curation, investigation, methodology, software, and writing—review & editing; Jun Liu: data curation; methodology; and writing—review & editing; Yu Liu: data curation, formal analysis, and writing—review & editing. All authors reviewed the results and approved the final version of the manuscript.
Availability of Data and Materials: The datasets generated during and/or analyzed during the current study are available from the corresponding author on reasonable request.
Ethics Approval: Not applicable.
Conflicts of Interest: The authors declare that they have no conflicts of interest to report regarding the present study.
References
1. Victoni T, Barreto E, Lagente V, Carvalho VF. Oxidative imbalance as a crucial factor in inflammatory lung diseases: could antioxidant treatment constitute a new therapeutic strategy? Oxid Med Cell Longev. 2021;2021:6646923. doi:10.1155/omcl.v2021.1. [Google Scholar] [CrossRef]
2. Ruopp NF, Cockrill BA. Diagnosis and treatment of pulmonary arterial hypertension: a review. JAMA. 2022;327(14):1379–91. doi:10.1001/jama.2022.4402. [Google Scholar] [PubMed] [CrossRef]
3. Luna-López R, Ruiz Martín A, Escribano Subías P. Pulmonary arterial hypertension. Med Clin. 2022;158(12):622–9. doi:10.1016/j.medcli.2022.01.003. [Google Scholar] [PubMed] [CrossRef]
4. Florentin J, Coppin E, Vasamsetti SB, Zhao J, Tai YY, Tang Y, et al. Inflammatory macrophage expansion in pulmonary hypertension depends upon mobilization of blood-borne monocytes. J Immunol. 2018;200(10):3612–25. doi:10.4049/jimmunol.1701287. [Google Scholar] [PubMed] [CrossRef]
5. Knirel YA, Anisimov AP, Kislichkina AA, Kondakova AN, Bystrova OV, Vagaiskaya AS, et al. Lipopolysaccharide of the Yersinia pseudotuberculosis Complex. Biomolecules. 2021;11(10):1410. doi:10.3390/biom11101410. [Google Scholar] [PubMed] [CrossRef]
6. Shapouri-Moghaddam A, Mohammadian S, Vazini H, Taghadosi M, Esmaeili SA, Mardani F, et al. Macrophage plasticity, polarization, and function in health and disease. J Cell Physiol. 2018;233(9):6425–40. doi:10.1002/jcp.26429. [Google Scholar] [PubMed] [CrossRef]
7. Willis GR, Fernandez-Gonzalez A, Reis M, Mitsialis SA, Kourembanas S. Macrophage immunomodulation: the gatekeeper for mesenchymal stem cell derived-exosomes in pulmonary arterial hypertension? Int J Mol Sci. 2018;19(9):2534. doi:10.3390/ijms19092534. [Google Scholar] [PubMed] [CrossRef]
8. Wu Y, Pan B, Zhang Z, Li X, Leng Y, Ji Y, et al. Caspase-4/11-mediated pulmonary artery endothelial cell pyroptosis contributes to pulmonary arterial hypertension. Hypertension. 2022;79(3):536–48. doi:10.1161/HYPERTENSIONAHA.121.17868. [Google Scholar] [PubMed] [CrossRef]
9. Yang F, Bettadapura SN, Smeltzer MS, Zhu H, Wang S. Pyroptosis and pyroptosis-inducing cancer drugs. Acta Pharmacol Sin. 2022;43(10):2462–73. doi:10.1038/s41401-022-00887-6. [Google Scholar] [PubMed] [CrossRef]
10. Seoane PI, Lee B, Hoyle C, Yu S, Lopez-Castejon G, Lowe M, et al. The NLRP3-inflammasome as a sensor of organelle dysfunction. J Cell Biol. 2020;219(12):3340. doi:10.1083/jcb.202006194. [Google Scholar] [PubMed] [CrossRef]
11. Foley A, Steinberg BE, Goldenberg NM. Inflammasome activation in pulmonary arterial hypertension. Front Med. 2021;8:826557. [Google Scholar]
12. Molgora M, Liu YA, Colonna M, Cella M. TREM2: a new player in the tumor microenvironment. Semin Immunol. 2023;67:101739. doi:10.1016/j.smim.2023.101739. [Google Scholar] [PubMed] [CrossRef]
13. Zheng Q, Han Y, Fan M, Gao X, Ma M, Xu J, et al. Potential role of TREM2 in high cholesterol‐induced cell injury and metabolic dysfunction in SH‐SY5Y cells. Exp Ther Med. 2023;25(5):205. doi:10.3892/etm. [Google Scholar] [CrossRef]
14. Miao Z, Zhao X, Liu X. Hypoxia induced β-catenin lactylation promotes the cell proliferation and stemness of colorectal cancer through the wnt signaling pathway. Exp Cell Res. 2023;422(1):113439. doi:10.1016/j.yexcr.2022.113439. [Google Scholar] [PubMed] [CrossRef]
15. Wang Y, Cao C, Zhu Y, Fan H, Liu Q, Liu Y, et al. TREM2/β-catenin attenuates NLRP3 inflammasome-mediated macrophage pyroptosis to promote bacterial clearance of pyogenic bacteria. Cell Death Dis. 2022;13(9):771. doi:10.1038/s41419-022-05193-x. [Google Scholar] [PubMed] [CrossRef]
16. Huang X, Deng H, Shen QK, Quan ZS. Tanshinone IIA: pharmacology, total synthesis, and progress in structure-modifications. Curr Med Chem. 2022;29(11):1959–89. doi:10.2174/0929867328666211108110025. [Google Scholar] [PubMed] [CrossRef]
17. Wan H, Li Y, Qin Y, An Y, Yan H, Liu X, et al. Polyphenol-mediated sandwich-like coating promotes endothelialization and vascular healing. Biomaterials. 2023;302:122346. doi:10.1016/j.biomaterials.2023.122346. [Google Scholar] [PubMed] [CrossRef]
18. Zhong L, Ding W, Zeng Q, He B, Zhang H, Wang L, et al. Corrigendum to sodium tanshinone IIA sulfonate attenuates erectile dysfunction in rats with hyperlipidemia. Oxid Med Cell Longev. 2020;2020:1062074. [Google Scholar] [PubMed]
19. Chen Z, Feng H, Peng C, Zhang Z, Yuan Q, Gao H, et al. Renoprotective effects of tanshinone IIA: a literature review. Molecules. 2023;28(4):1990. doi:10.3390/molecules28041990. [Google Scholar] [PubMed] [CrossRef]
20. Gao H, Sun W, Zhao J, Wu X, Lu JJ, Chen X, et al. Tanshinones and diethyl blechnics with anti-inflammatory and anti-cancer activities from Salvia miltiorrhiza Bunge. Sci Rep. 2016;6:33720. doi:10.1038/srep33720. [Google Scholar] [PubMed] [CrossRef]
21. Tang YS, Zhao YH, Zhong Y, Li XZ, Pu JX, Luo YC, et al. Neferine inhibits LPS-ATP-induced endothelial cell pyroptosis via regulation of ROS/NLRP3/Caspase-1 signaling pathway. Inflamm Res. 2019;68(9):727–38. doi:10.1007/s00011-019-01256-6. [Google Scholar] [PubMed] [CrossRef]
22. Hu Z, Murakami T, Suzuki K, Tamura H, Kuwahara-Arai K, Iba T, et al. Antimicrobial cathelicidin peptide LL-37 inhibits the LPS/ATP-induced pyroptosis of macrophages by dual mechanism. PLoS One. 2014;9(1):e85765. doi:10.1371/journal.pone.0085765. [Google Scholar] [PubMed] [CrossRef]
23. Deng Y, Guo SL, Wei B, Gao XC, Zhou YC, Li JQ. Activation of nicotinic acetylcholine α7 receptor attenuates progression of monocrotaline-induced pulmonary hypertension in rats by downregulating the NLRP3 inflammasome. Front Pharmacol. 2019;10:128. doi:10.3389/fphar.2019.00128. [Google Scholar] [PubMed] [CrossRef]
24. Li Y-I, Elmer G, LeBoeuf RC. Tanshinone IIA reduces macrophage death induced by hydrogen peroxide by upregulating glutathione peroxidase. Life Sci. 2008;83(15):557–62. doi:10.1016/j.lfs.2008.08.003. [Google Scholar] [PubMed] [CrossRef]
25. Zhou J, Li W, Xie Q, Hou Y, Zhan S, Yang X, et al. Effects of simvastatin on glucose metabolism in mouse MIN6 cells. J Diabetes Res. 2014;2014:376570. doi:10.1155/2014/376570. [Google Scholar] [PubMed] [CrossRef]
26. Zhao J, Li B, Ren Y, Liang T, Wang J, Zhai S, et al. Histone demethylase KDM4A plays an oncogenic role in nasopharyngeal carcinoma by promoting cell migration and invasion. Exp Mol Med. 2021;53(8):1207–17. doi:10.1038/s12276-021-00657-0. [Google Scholar] [PubMed] [CrossRef]
27. Hsu CG, Chávez CL, Zhang C, Sowden M, Yan C, Berk BC. The lipid peroxidation product 4-hydroxynonenal inhibits NLRP3 inflammasome activation and macrophage pyroptosis. Cell Death Differ. 2022;29(9):1790–803. doi:10.1038/s41418-022-00966-5. [Google Scholar] [PubMed] [CrossRef]
28. Zhang X, Wang CB, Duan LH, Long JJ, Xiao P, Wang YL, et al. Correlation research of serum substance P, CCK-8, and 5-HT values with depression levels in stroke survivors. Eur Rev Med Pharmacol Sci. 2023;27(4):1248–54. [Google Scholar] [PubMed]
29. Huang P, Zhang Z, Zhang P, Feng J, Xie J, Zheng Y, et al. TREM2 deficiency aggravates NLRP3 inflammasome activation and pyroptosis in MPTP-induced parkinson’s disease mice and LPS-induced BV2 cells. Mol Neurobiol. 2023;61(5):2590–605. [Google Scholar] [PubMed]
30. Zeng CY, Li CG, Shu JX, Xu LH, Ouyang DY, Mai FY, et al. ATP induces caspase-3/gasdermin E-mediated pyroptosis in NLRP3 pathway-blocked murine macrophages. Apoptosis. 2019;24(9–10):703–17. doi:10.1007/s10495-019-01551-x. [Google Scholar] [PubMed] [CrossRef]
31. Guan Z, Chen J, Li X, Dong N. Tanshinone IIA induces ferroptosis in gastric cancer cells through p53-mediated SLC7A11 down-regulation. Biosci Rep. 2020;40(8):87. doi:10.1042/BSR20201807. [Google Scholar] [PubMed] [CrossRef]
32. Ma X, Zhang L, Gao F, Jia W, Li C. Salvia miltiorrhiza and Tanshinone IIA reduce endothelial inflammation and atherosclerotic plaque formation through inhibiting COX-2. Biomed Pharmacother. 2023;167:115501. doi:10.1016/j.biopha.2023.115501. [Google Scholar] [PubMed] [CrossRef]
33. Yang L, Zhou G, Liu J, Song J, Zhang Z, Huang Q, et al. Tanshinone I and Tanshinone IIA/B attenuate LPS-induced mastitis via regulating the NF-κB. Biomed Pharmacother. 2021;137:111353. doi:10.1016/j.biopha.2021.111353. [Google Scholar] [PubMed] [CrossRef]
34. Fan G, Jiang X, Wu X, Fordjour PA, Miao L, Zhang H, et al. Anti-inflammatory activity of tanshinone IIA in LPS-stimulated RAW264.7 macrophages via miRNAs and TLR4-NF-κB pathway. Inflammation. 2016;39(1):375–84. doi:10.1007/s10753-015-0259-1. [Google Scholar] [PubMed] [CrossRef]
35. Guan R, Wang J, Li Z, Ding M, Li D, Xu G, et al. Sodium tanshinone IIA sulfonate decreases cigarette smoke-induced inflammation and oxidative stress via blocking the activation of MAPK/HIF-1α signaling pathway. Front Pharmacol. 2018;9:263. doi:10.3389/fphar.2018.00263. [Google Scholar] [PubMed] [CrossRef]
36. Zhang R, Liu Y, You J, Ge B. Tanshinone IIA inhibits ischemia-reperfusion-induced inflammation, ferroptosis and apoptosis through activation of the PI3K/Akt/mTOR pathway. Hum Exp Toxicol. 2023;42:9603271231180864. doi:10.1177/09603271231180864. [Google Scholar] [PubMed] [CrossRef]
37. Fu J, Wu H. Structural mechanisms of NLRP3 inflammasome assembly and activation. Annu Rev Immunol. 2023;41:301–16. doi:10.1146/annurev-immunol-081022-021207. [Google Scholar] [PubMed] [CrossRef]
38. Guan C, Huang X, Yue J, Xiang H, Shaheen S, Jiang Z, et al. SIRT3-mediated deacetylation of NLRC4 promotes inflammasome activation. Theranostics. 2021;11(8):3981–95. doi:10.7150/thno.55573. [Google Scholar] [PubMed] [CrossRef]
39. Hou X, Xu G, Wang Z, Zhan X, Li H, Li R, et al. Glaucocalyxin A alleviates LPS-mediated septic shock and inflammation via inhibiting NLRP3 inflammasome activation. Int Immunopharmacol. 2020;81:106271. doi:10.1016/j.intimp.2020.106271. [Google Scholar] [PubMed] [CrossRef]
40. Xing Y, Zhang F, Ji P, Wei M, Yin C, Yang A, et al. Efficient delivery of GSDMD-N mRNA by engineered extracellular vesicles induces pyroptosis for enhanced immunotherapy. Small. 2023;19(20):e2204031. doi:10.1002/smll.202204031. [Google Scholar] [PubMed] [CrossRef]
41. Jiang X, Ma C, Gao Y, Cui H, Zheng Y, Li J, et al. Tongxinluo attenuates atherosclerosis by inhibiting ROS/NLRP3/Caspase-1-mediated endothelial cell pyroptosis. J Ethnopharmacol. 2023;304:116011. doi:10.1016/j.jep.2022.116011. [Google Scholar] [PubMed] [CrossRef]
42. Han Y, Zhang YY, Pan YQ, Zheng XJ, Liao K, Mo HY, et al. IL-1β-associated NNT acetylation orchestrates iron-sulfur cluster maintenance and cancer immunotherapy resistance. Mol Cell. 2023;83(11):1887–902.e8. doi:10.1016/j.molcel.2023.05.011. [Google Scholar] [PubMed] [CrossRef]
43. Gu L, Sun M, Li R, Zhang X, Tao Y, Yuan Y, et al. Didymin suppresses microglia pyroptosis and neuroinflammation through the Asc/Caspase-1/GSDMD pathway following experimental intracerebral hemorrhage. Front Immunol. 2022;13:810582. doi:10.3389/fimmu.2022.810582. [Google Scholar] [PubMed] [CrossRef]
44. Liu J, Xiao Q, Xiao J, Niu C, Li Y, Zhang X, et al. Wnt/β-catenin signalling: function, biological mechanisms, and therapeutic opportunities. Signal Transduct Target Ther. 2022;7(1):3. doi:10.1038/s41392-021-00762-6. [Google Scholar] [PubMed] [CrossRef]
45. Liu H, Zhou L, Wang X, Zheng Q, Zhan F, Zhou L, et al. Dexamethasone upregulates macrophage PIEZO1 via SGK1, suppressing inflammation and increasing ROS and apoptosis. Biochem Pharmacol. 2024;222(12):116050. doi:10.1016/j.bcp.2024.116050. [Google Scholar] [PubMed] [CrossRef]
46. Shi Y, Zhang R, Da N, Wang Y, Yang J, Li B, et al. Aspirin loaded extracellular vesicles inhibit inflammation of macrophages via switching metabolic phenotype in periodontitis. Biochem Biophys Res Commun. 2023;667:25–33. doi:10.1016/j.bbrc.2023.05.024. [Google Scholar] [PubMed] [CrossRef]
47. Matsubara Y, Kiwan G, Liu J, Gonzalez L, Langford J, Gao M, et al. Inhibition of T-cells by cyclosporine a reduces macrophage accumulation to regulate venous adaptive remodeling and increase arteriovenous fistula maturation. Arterioscler Thromb Vasc Biol. 2021;41(3):e160–e74. doi:10.1161/ATVBAHA.120.315875. [Google Scholar] [PubMed] [CrossRef]
48. Yanaizu M, Adachi H, Araki M, Kontani K, Kino Y. Translational regulation and protein-coding capacity of the 5' untranslated region of human TREM2. Commun Biol. 2023;6(1):616. doi:10.1038/s42003-023-04998-6. [Google Scholar] [PubMed] [CrossRef]
49. Park JS, Ji IJ, Kim DH, An HJ, Yoon SY. The alzheimer’s disease-associated R47H variant of TREM2 has an altered glycosylation pattern and protein stability. Front Neurosci. 2016;10:618. [Google Scholar] [PubMed]
50. Park MD, Reyes-Torres I, LeBerichel J, Hamon P, LaMarche NM, Hegde S, et al. TREM2 macrophages drive NK cell paucity and dysfunction in lung cancer. Nat Immunol. 2023;24(5):792–801. doi:10.1038/s41590-023-01475-4. [Google Scholar] [PubMed] [CrossRef]
51. Xie M, Liu YU, Zhao S, Zhang L, Bosco DB, Pang YP, et al. TREM2 interacts with TDP-43 and mediates microglial neuroprotection against TDP-43-related neurodegeneration. Nat Neurosci. 2022;25(1):26–38. doi:10.1038/s41593-021-00975-6. [Google Scholar] [PubMed] [CrossRef]
Cite This Article
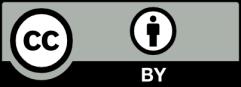
This work is licensed under a Creative Commons Attribution 4.0 International License , which permits unrestricted use, distribution, and reproduction in any medium, provided the original work is properly cited.