Open Access
REVIEW
Crossroads: Pathogenic role and therapeutic targets of neutrophil extracellular traps in rheumatoid arthritis
1 Department of Rheumatology, The First Affiliated Hospital of Anhui University of Chinese Medicine, Hefei, 230031, China
2 First Clinical Medical School, Anhui University of Chinese Medicine, Hefei, 230031, China
3 Institute of Rheumatology, Anhui Academy of Chinese Medicine, Hefei, 230031, China
* Corresponding Author: JIAN LIU. Email:
(This article belongs to the Special Issue: Advances in Biomarker Research: Unveiling the Pathways to Precision Medicine)
BIOCELL 2024, 48(1), 9-19. https://doi.org/10.32604/biocell.2023.045862
Received 10 September 2023; Accepted 23 November 2023; Issue published 30 January 2024
Abstract
Rheumatoid arthritis (RA) is a prevalent autoimmune disease whose main features include chronic synovial inflammation, bone destruction, and joint degeneration. Neutrophils are often considered to be the first responders to inflammation and are a key presence in the inflammatory milieu of RA. Neutrophil extracellular traps (NETs), a meshwork of DNA-histone complexes and proteins released by activated neutrophils, are widely involved in the pathophysiology of autoimmune diseases, especially RA, in addition to playing a key role in the neutrophil innate immune response. NETs have been found to be an important source of citrullinated autoantigen antibodies and inflammatory factor release, which can activate RA synovial fibroblasts (FLS) and cause joint damage. This article reviews the role of NETs in the pathophysiology of RA, demonstrating the application of multiple molecules with various therapies, with a view to informing the discovery and development of novel biomarkers and therapeutic targets for RA.Graphical Abstract
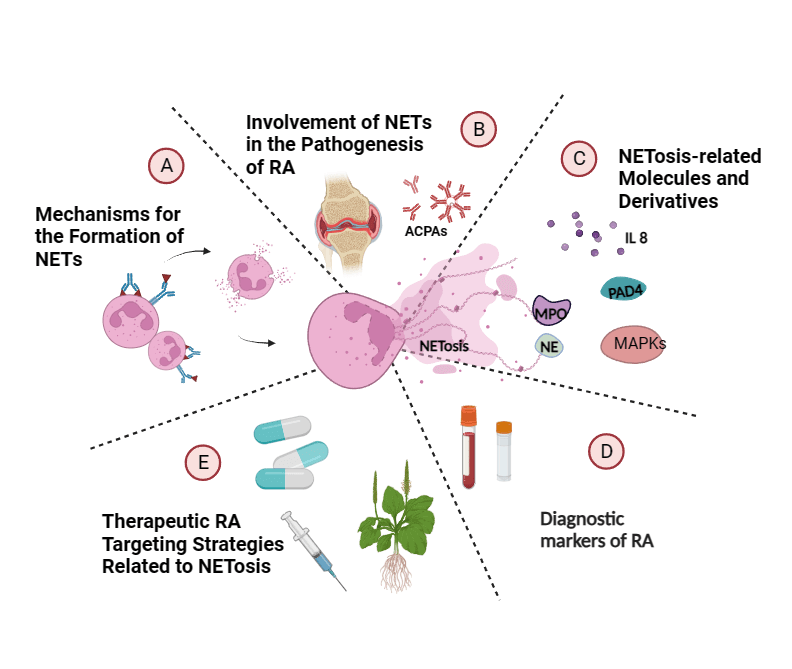
Keywords
Abbreviations
AA | Adjuvant arthritis |
ACPAs | Anti-citrullinated peptide antibodies |
AKT | Protein kinase B |
ANETA | Antibodies against NET |
Atg5 | Autophagy related protein 5 |
BAFF | B-cell activating factor |
Bax | Bcl-2 Assaciated X protein |
Bcl-2 | B-cell lymphoma 2 |
CarP | Carbamoylated proteins |
CCL-5 | C-C motif chemokine ligand 5 |
CIA | Collagen-induced arthritis |
CitH3 | Citrullinated histone H3 |
cNET | Carbamoylated NETs proteins |
CXCL1/2/8 | Chemokine (C-X-C Motif) Ligand 1/2/8 |
ERK | Extracellular regulated kinase |
FLS | Fibroblast-like synoviocytes |
GM-CSF | Granulocyte-macrophage colony stimulating factor |
GMSC | Gingival-derived mesenchymal stem cells |
IFN-γ | Interferon γ |
IL-6/17A/10/1β/4/8 | Interleukin 6/17A/10/1β/4/8 |
JNK | c-Jun N-terminal kinase |
LC3 | Microtubule-associated protein 1 light chain 3 |
LPS | Lipopolysaccharide |
Ly6G | Lymphocyte antigen 6G |
MAPK | Mitogen-activated protein kinase |
MHC | Major histocompatibility complex |
MPO | Myeloperoxidase |
NADPH | Nicotinamide adenine dinucleotide phosphate |
NE | Neutrophil elastase |
NETosis | Process of NET formation |
NETs | Neutrophil extracellular traps |
NF-κB | Nuclear factor-κB |
NOX | NADPH oxidase |
PAD4 | Protein-arginine deiminase type 4 |
PGE2 | Prostaglandin E2 |
PKC | Protein kinase C |
PMA | Phorbol 12-myristate 13-acetate |
PMN | Polymorphonuclear |
RA | Rheumatoid arthritis |
RF | Rheumatoid factor |
ROC | Receiver operating characteristic |
ROS | Reactive oxygen species |
SLE | Systemic lupus erythematosus |
TLR | Toll-like receptor |
TNF-α | Tumor necrosis factor α |
Rheumatoid arthritis (RA) is a systemic autoimmune disease dominated by multiple symmetrical arthritis [1,2], in which patients often experience symptoms of morning stiffness, swelling, and pain of the joints, which gradually progresses during recurrent episodes of prolonged disease to bone damage, joint deformity and functional disability [3,4]. In addition to joint damage, systemic inflammation can involve other organs, including the skin, eyes, blood vessels, heart and lungs in 40% of RA patients [5]. The global prevalence of RA is reported to be about 1%, with female patients being two to three times more likely to suffer than male patients, and the disability rate is also higher and increasing year by year, which seriously affects patients’ quality of life and social participation [6,7].
Although the exact etiology of RA is still not fully understood, most studies suggest that it may be closely related to environmental and genetic factors [8]. Previous studies have shown that autoimmune responses and inflammation are important pathophysiological bases for the development of RA [4]. Therefore, abnormal infiltration and activation of synovial membranes by different immune cells and an imbalance between pro- and anti-inflammatory cellular immune responses are crucial for the onset and progression of RA [9]. Neutrophils, as the most abundant circulating form of human leukocytes, are key effector cells in the innate immune system [10] as well as being considered the first line of defence during injury and infection. Neutrophils have been reported to be the most abundant cell type in the synovial fluid of RA patients [11]. Increasing evidence suggests that neutrophils are closely associated with RA synovial inflammation and cartilage damage, and may also be involved in the development of RA through mechanisms such as the release of immune mediators, respiratory burst, and apoptosis [12,13].
Neutrophil extracellular traps (NETs) are a specific defense mechanism of neutrophils, a large network of depolymerized DNA fibers and derived nuclear, cytoplasmic, and granule proteins released by that trap and kill pathogens [14]. Brinkmann et al. [15] first described and formally named the process NETosis, defining a new paradigm for antimicrobial innate responses. In addition to their role as host defense mechanisms, a large body of literature has demonstrated that NETs may also lead to toxic effects in the host. For example, a recent study reported the pro-tumourigenic activity of NETs in terms of their involvement in cancer immunoediting, progression, and metastatic spread [16]. The ability of NETs to induce autoimmunity has been widely suggested in a wide variety of autoimmune diseases such as systemic lupus erythematosus, rheumatoid arthritis, antineutrophil cytoplasmic antibody-associated vasculitis, antiphospholipid syndrome, and type 1 diabete [17,18]. Thus, NETs often play a complex double-edged role in autoimmune diseases.
In this review, we first describe the mechanism of formation and the key pathogenic roles of NETs in RA (Fig. 1), then we show some key derived molecules, respectively, and accumulate evidence that NETosis is a potential biomarker for diagnosis, subtype identification, and risk detection. Finally, we discuss the targeting mechanisms of NETosis by various therapies, including conventional and biologic agents and traditional Chinese medicine, suggesting that NETosis has the potential to be a revolutionary precision medicine target for personalized treatment and subsequent drug development in RA.
Figure 1: The process of neutrophil extracellular traps (NETs) formation (NETosis) is involved in the pathogenesis and progression of RA in four main ways. (1) NETosis upregulates the production of inflammatory cytokines, amplifies joint inflammation, activates synoviocytes, and presents autoantigens to T cells, secretes BAFF to promote B cell proliferation, thus linking innate immunity with the modulation of adaptive immune responses; (2) components of NETs may act as a source of antibodies to guanosine-primed autoantigens; (3) NETs promote the production of anti-ammonocarbamylated protein (CarP) antibodies and upregulate RANKL production by FLS, which mediates osteoclast formation; (4) NETs are associated with periodontitis and smoking, two major susceptibility factors for RA. Abbreviations: ACPAs: anti-citrullinated peptide antibodies; BAFF: B-cell activating factor; CarPs: carbamoylated proteins; CitH3: citrullinated histone H3; FcR: Fc receptors; FLS: fibroblast-like synoviocytes; IFN-γ: interferon γ; IgG: immunoglobulin G; IL-6/8: interleukin 6/8; LPS: lipopolysaccharide; MHC : major histocompatibility complex; MPO: myeloperoxidase; NADPH: nicotinamide adenine dinucleotide phosphate; NE: neutrophil elastase; NETs: neutrophil extracellular traps; NOX: NADPH oxidase; PAD4: protein-arginine deiminase type 4; PMA: phorbol 12-myristate 13-acetate; ROS: reactive oxygen species; TLRs: toll-like receptors; TNF-α: tumor necrosis factor α.
Mechanisms for the Formation of NETs
In recent years, two different major pathways for the formation of NETs have been identified. The most well-known pathway is the traditional suicidal NETosis, which is a gradual process. Upon exposure of neutrophils to various stimuli such as fopperol 12-myristate 13-acetate (PMA) and lipopolysaccharide (LPS) [19,20], triggered by innate immune receptors in combination with downstream intracellular mediators [21], calcium is released from the endoplasmic reticulum of the neutrophils, which activates nicotinamide adenine dinucleotide phosphate (NADPH) oxidase (NOX) via the protein kinase C (PKC) signaling pathway. Peroxisomes and mitochondria generate reactive oxygen species (ROS), which in turn activates myeloperoxidase (MPO) and mediates translocation of activated neutrophil elastase (NE) to the nucleus, collectively driving protein hydrolysis and cellular degradation [22]. At the same time, activated protein-arginine deiminase type 4 (PAD4) induces citrullination involved in the formation of citrullinated histone H3 (CitH3), which triggers chromatin decondensation [23]. Finally, this mixture of chromatin and granulin is formed and released into the extracellular space, where neutrophils undergo lysis and release NETs. The key to this process is the production of ROS by NOX during the “respiratory burst” of neutrophils, which is also known as the NOX-dependent pathway. The other pathway is the NOX-independent pathway, known as essential NETosis or non-lytic NETosis, where neutrophils release NETs without disrupting the nuclear or plasma membranes, are not accompanied by neutrophil death, and retain some of their regular cellular functions, such as cell phagocytosis and migration [24]. This NOX-independent NETosis is largely dependent on mitochondrial ROS (mROS) production, and after stimulation with LPS or C5a (protein fragments released from cleavage of the complement component C5), mitochondria in neutrophils act as ROS generators, with an influx of calcium carriers into the extracellular calcium, and inducing the production of mROS [25].
Involvement of NETosis in the Pathogenesis of RA
Neutrophils can participate in immunoinflammation of RA via the process of extracellular reticular traps (NETosis)
In the early stages of RA, large numbers of neutrophils pre-activated by immune complexes are recruited into synovial tissues and joints, and infiltrating neutrophils exhibit an ‘activated’ phenotype, including increased production of cytokines, chemokines, and ROS, delayed apoptosis and activation of NETs [26]. NETs has been reported to be detected in the synovial fluid of diseased joints as well as rheumatoid nodules in RA patients, and neutrophils in RA patients release more and faster NETs than in healthy subjects [27]. Excessive increase of NETs in synovial and peripheral blood neutrophils stimulates the induction of inflammatory factors such as tumor necrosis factor (TNF)-α, interleukin (IL)-6, IL-8, IL-17A, and interferon (IFN)-γ, further promoting the expansion of the inflammatory response and the formation of an “inflammatory storm” [28]. A large number of studies have confirmed the existence of an “inflammatory storm” in RA patients, which is an immune-inflammatory response characterized by a high release of pro-inflammatory cytokines and a decrease in anti-inflammatory cytokines [29]. Pro-inflammatory cytokines such as IL-17A, TNF-α, and IL-8 are in turn strong inducers of NETosis in neutrophils [30], thus creating a vicious circle. Fibroblast-like synoviocytes (FLS) are known to be the main effector cells involved in the process of joint destruction [31]. FLS exposed to NETs exhibit pro-inflammatory, hyperproliferative and more than invasive properties, leading to pannus formation in chronic RA [32]. In addition, FLS promotes major histocompatibility complex (MHC) class II upregulation through the internalization of NETs and shares epitopes to present modified self-antigens to CD4 T cells [33]. Tillack et al. [34] first described that NETs mediate T cell proliferation and pro-inflammatory cytokine secretion by lowering its activation threshold. NETs also stimulate neutrophils to secrete IL-8 and B-cell activating factor (BAFF), promoting a vicious cycle between B cells and neutrophils [35]. In conclusion, NETs, as a specific structure and form of neutrophils, activate synoviocytes, upregulate pro-inflammatory cytokine production, amplify joint inflammation, and connect innate immunity with the modulation of adaptive immune responses involved in the inflammatory process of RA.
NETs as a source of antibodies to citrullinated autoantigens
Autoantibodies, including rheumatoid factor (RF) and anti-citrullinated peptide antibodies (ACPAs), are diagnostic markers of RA according to the 2010 American College of Rheumatology/European League Against Rheumatic Diseases mandated classification criteria for RA [36]. It has been claimed that ACPAs are present in the serum of more than two-thirds of RA patients, several years before the onset of clinical symptoms, compared to the classical and well-known RA-specific antibody RF [37,38], which are widely used for diagnosis and prognosis prediction. It has been demonstrated that histones and other NETs-associated proteins can be citrullinated by PAD enzymes (especially PAD4), providing a source of citrullinated proteins for the immune system and facilitating the production of ACPAs [39]. In turn, ACPAs can perpetuate inflammation by stimulating the formation of NETs and promoting inflammation in RA [40], thus creating a vicious cycle. As observed by Okamoto [41], NETs residues are associated with localized ACPA production and levels as well as inflammatory factors such as IL-1β and IL-6. It has been documented that NETs are positively correlated with ACPA in the sputum of susceptible individuals and first-degree relatives of RA patients, which may be associated with the onset or early stages of the disease [42]. In addition, Felty syndrome is a severe form of RA, and Pratesi et al. [43] further proposed that citrullinated H4 from activated neutrophils and NETs is the target of the antibody ACPA in Felty syndrome, all of which further elucidates the role of NETs in RA.
NETs mediate osteoclast formation
Anti-carbamoylated protein (CarP) antibodies have been reported to be significantly associated with bone destruction, joint erosion, and disease activity in RA patients, and are emerging as a biomarker for RA [44,45]. NETs release carbamoylated proteins (CarP), which leads to the production of antibodies to CarP and antibodies to carbamoylated NETs proteins (cNET) production, which promotes osteoclast formation and activation [46]. At the same time, NETs are internalized by FLS and upregulate FLS production of RANKL, which promotes osteoclast formation by CD14 monocytes [47]. In addition, NE in NETs can directly degrade cartilage components and expanded FLS and macrophages can further contribute to joint damage [48].
NETs are associated with susceptibility factors for RA
Periodontitis and smoking have been documented as susceptibility factors for RA [49,50]. It has been reported that expression of PADase homologs by Porphyromonas gingivalis induces the production of citrullinated antigens and enhances NETosis in patients with periodontitis, which triggers pathophysiology associated with RA [51]. Kaneko et al. [52] found that compared to control individuals, RA patients with periodontitis had significantly higher serum levels of CarP and NETs, which correlated with disease severity. Oliveira et al. [53] were even more direct in pointing out that enhanced localized formation of NETs in periodontitis may be an early event in RA, and that periodontal treatment also leads to circulating significant changes in NETs levels. Several studies have confirmed the strong association between cigarette smoking and increased risk of RA, especially in ACPA-positive patients [54,55]. Cigarette smoking can induce ACPA in the lungs by nicotine-inducing NETs and citrulline formation in a PAD4-dependent manner [56]. Lee et al. [57] demonstrated that nicotine is a potent inducer of NETosis, which may accelerate arthritis progression on a murine model of collagen-induced arthritis. Proteomic analysis of bronchial biopsies and synovial biopsies also identified the same citrullinated protein targets in the lungs of RA patients and RA joints, suggesting that the lungs are a possible origin of ACPA [58]. Demoruelle et al. [59] found that in individuals at risk of developing RA individuals with high levels of NETs in sputum were strongly associated with antibody reactivity such as ACPA, further supporting the link between NETs and the development of ACPA in the lungs.
NETosis-Related Molecules and Derivatives
IL-8, also known as CXCL8, is a CXC-type pro-inflammatory chemokine that plays an important role in neutrophil activation and recruitment to sites of inflammation. It activates and induces neutrophils to undergo migration to the site of inflammation while promoting their mass infiltration, thus facilitating the formation of NETs at their focal sites [60]. The data of Shu et al. [61] confirmed that the formation of NETs is induced by IL-8 and proposed that the process is associated with the mitogen-activated protein kinase and nicotinamide adenine dinucleotide phosphate pathways. Shang et al. [62] found that exosomes stimulate the expression of IL-8 expression which in turn promotes the formation of tumor-associated NETs. Abrams et al. [63] developed a new assay for NETs and found that IL-8 levels are closely related to their formation, and that inhibition of IL-8 therapy significantly attenuates NETosis and thereby reduces organ damage and mortality, as demonstrated in a mouse model of sepsis. In conclusion, numerous studies have confirmed that IL-8 is a strong inducer of NETosis.
Myeloperoxidase (MPO) and neutrophil elastase (NE)
MPO and NE are well-defined markers of neutrophil infiltration [64]. MPO is one of the most abundant proteins in neutrophils, accounting for up to 5% of the cell’s dry weight, and is normally stored in nitrogenophilic granules and released in response to stimulation of neutrophils. Metzler et al. [65] demonstrated that the process of NETs formation requires high local concentrations of MPO products, while pharmacological inhibition of MPO delays and reduces the formation of NETs. It has been reported that abundant MPO is detected in the inflamed synovium of RA patients [66], which leads to further neutrophil recruitment, amplification of inflammation, and FLS proliferation [67]. In addition, MPO also interacts with vascular endothelial cells interacting to increase endothelial permeability, which is also a key process during inflammation [68]. NE, a protein hydrolase stored in the neutrophilic granules of neutrophils, derives its name from its ability to degrade the extracellular matrix protein elastin, as well as being an important source of extracellular killing of bacteria and degradation of virulence factors by NETs [69]. Studies have shown that NE can induce cell proliferation and activate a variety of cytokine and chemokine signaling pathways [70]. Conversely, inhibition of NE signaling inhibits the formation of NETs, reduces Nets-mediated vascular damage, and mitigates the production of inflammatory cytokines [71]. Muley et al. [72] also found that NE can promote knee injury in mice through PAR2-dependent activation of the p44/42 MAPK pathway.
Peptidyl arginine deiminase 4 (PAD 4)
The PAD gene encodes a PAD protein that converts arginine residues to citrulline. Interestingly, many pathological and genetic studies have identified the gene encoding the PAD4 enzyme (PADI4) as a susceptibility gene for RA, which has recently been identified as an important risk factor for people at high risk of RA, especially in North American populations [73]. Turunen et al. [74] found that PAD4 was abundant in the synovial tissue of RA and involved in the citrullination of fibrin, which was closely related to tissue inflammation. The PAD4 enzyme catalyzes the deimination of arginine residues to produce the main antigenic target of ACPA in RA, considered a key enzyme in the pathogenesis of RA [75]. PAD4-mediated citrullination has also been found to be involved in monoclonal ACPA-induced tenosynovitis, as well as a key mechanism of pain and bone loss [76]. Jonsson et al. [77] found that the ability of serum to activate PAD4 was associated with early RA patients’ ACPA and RF positivity and disease flares. Gómez-Bañuelos et al. [78] identified associations between various subtypes of anti-PAD4 and clinical outcomes in RA including imaging joint damage, rheumatoid nodules and interstitial lung disease. Similarly, anti-PAD4 antibodies were found to recognize RA patients with higher levels of radiographic damage and bone erosion and lower levels of anti-PAD4 antibodies were associated with lung involvement in RA patients in a cohort by Palterer et al. [79]. This is supported by another finding that PAD4 antibodies are present in sputum and saliva of some RA patients [80], while a meta-analysis by Zhang et al. found that RA patients carrying anti-PAD4 antibodies had a higher likelihood of DAS28, ESR, swollen joint count (SJC), as well as of developing interstitial lung disease and pulmonary fibrosis [81]. In conclusion, the above evidence suggests that the PAD4 enzyme and anti-PAD4 antibodies are involved in three important features of RA: citrullination, lung involvement, and bone destruction, and thus could be potentially useful diagnostic biomarkers of RA and important therapeutic target candidates.
Mitogen-activated protein kinases (MAPKs)
A series of protein kinases in the MAPK signaling pathway, including c-Jun N-terminal kinase (JNK), extracellular regulated kinase (ERK) and p38 kinase, are known to promote the early production of inflammatory cytokines [82]. Currently, it has been documented that MAPKs are the upstream signaling pathways of NETs in a variety of diseases. Dömer et al. [83] first determined that Nets-induced neutrophil activation occurs through pathways involving phosphorylation of protein kinase B (Akt), ERK1/2, and p38. Ji et al. [84] found that anggranin prevents neutrophil recruitment and NETosis by inhibiting the activation of nuclear factor (NF)-κB and MAPK signaling pathways in LPS-induced acute lung injury. Zhou et al. [85] confirmed that cannabinoid receptors play a key role in neutrophil chemotaxis and NETosis in aseptic liver inflammation, which is related to the ROS/p38 MAPK signaling pathway. Jati et al. [86] found in rats with gouty arthritis induced by monosodium urate that piperine regulates the immunosuppression of NLRP3 inflammasome through MAPK/NF-κB, reducing inflammatory symptoms and improving gouty caused by NETosis. In conclusion, we believe that the MAPK family is one of the key regulatory pathways of NETs expression.
Application in the Diagnosis and Treatment of RA
Some scholars have proposed that neutrophil activation and NETs derivatives in the plasma of RA patients have become promising diagnostic markers for monitoring RA. NETs derivatives including plasma cell free nucleosomes, MPO, NE and cathepsin G were closely related to the severity of RA. Pérez-Sánchez et al. [87] evaluated 40 patients with RA and 75 healthy individuals and found the elevated levels of NETs degradation products, such as circulating free DNA, free nucleosomes, NE, and MPO complexes in patients with RA, which may have diagnostic potential for disease activity and atherosclerosis as well as for the assessment of therapeutic effectiveness in RA. Sur Chowdhury et al. [88] measured Nets-derived free nucleosomes in RA serum by receiver operating characteristic (ROC) curve, showing diagnostic value with area under ROC > 97%, sensitivity of 91%, and specificity of 92%. de Bont et al. [89] first reported that antibodies against NET (ANETA) were detectable in baseline RA patients (22%–69%) and that serum ANETA levels in RF-positive RA patients were significantly higher than in negative RA patients. This evidence suggests that quantitative testing of NETs and their derivatives may be a useful complementary tool for identifying individuals at risk and monitoring patients with RA.
Therapeutic RA Targeting Strategies Related to NETosis
Elimination and inhibition of NETosis
As previously stated, dysregulated NETosis is closely implicated in the pathogenesis of RA. Therefore, targeting specific steps or products of NETosi might be a viable strategy for developing drugs and therapies for RA (Table 1). DNase I was the earliest described direct degrader of NETs [90]. Studies have shown that DNase-1 cuts and fragments DNA and destroys the skeleton of NETs [91]. Kolaczkowska et al. [92] also demonstrated that DNase not only effectively removed DNA carried by NETs, but also significantly inhibited the proteolytic activity of NE. At present, DNase I targeted destructive NETs have been used in the treatment of a variety of diseases, such as systemic lupus erythematosus [93], ischemia-reperfusion [94], malignant tumors [95], etc. Wang et al. [96] developed a DNase-functionalized hydrogel that demonstrated significant efficacy in a mouse model of CIA by continuously degrading NETs with new drug targets to reduce inflammatory responses in RA. In addition, some drugs designed to indirectly inhibit the formation of NETs may also be used to treat and relieve RA. Wan et al. [97] reported that dexamethasone can significantly reduce Staphylococcus aureus-induced NETosis by modulating toll-like receptors (TLRs), primarily TLR2 and TLR4. Kaundal et al. [98] conducted a study on 103 RA patients and found that MTX treatment was associated with the reduction of ROS production and CD177 expression, indirectly inhibiting NETosis, which may be one of the mechanisms of MTX treatment of RA. Kawaguchi et al. [99] demonstrated that injection of the PAD inhibitor chloramidine (CI-amidine) reduced protein citrullination and IL-6 in a mouse model of glucose-6-phosphate isomerase-induced arthritis (pGIA). A novel oral selective inhibitor of PAD4, JBI-589, prevents the formation of NETs and PAD4-dependent citcitline, reducing the severity of arthritis in mouse models [100]. Ruiz-Limón et al. [101] reported that after 6 months of treatment with the IL-6 R inhibitor tocilizumab, MPO and NE in RA patients decreased along with suppressed NETosis. Ascomycin and cyclosporin A can reduce IL-8-induced NETs by inhibiting the calcineurin pathway, and have been widely used in the treatment of rheumatoid arthritis, asthma, etc., [102,103]. Prostaglandin E2 (PGE2) is the most abundant prostaglandin in the human body and has been shown to have anti-inflammatory effects. Zhao et al. [104] reported that an emerging infusion therapy for gingival-derived mesenchymal stem cells (GMSCS) in recent years can improve inflammatory arthritis by inhibiting NETosis through the PGE2-PKA-ERK signaling pathway. From this perspective, pharmacological interventions or patient management are currently being developed to target inhibition ofNETosis at several levels: inhibiting the activation of inducible cytokines such as IL-8, targeting the intracellular processes of NETosis, and facilitating the removal or neutralization of NETosis-derived products such as MPO and NE.
NETosis participates in Chinese medicine treatment
In recent years, TCM has attracted more and more attention due to its advantages such as safety and fewer adverse reactions [105]. Using herbs and their extracts to treat RA has been practiced in Asian countries for thousands of years, whose efficacy has been confirmed by clinical applications and experimental studies [106]. Emodin accelerates apoptosis and inhibits autophagy and NETosis by reducing IL-6, IFN-γ, and TNF-α in adjuvant arthritis in mice [107]. Andrographolide inhibits autophagy-dependent NETosis by accelerating neutrophil apoptosis, while significantly reducing the phosphorylation of p38 MAPK and ERK1/2 [108]. Triptolide (TP) has shown potential as a treatment for RA by reducing neutrophil recruitment and down- regulation of TNF-α and IL-6 expression, and TP can also inhibit autophagy and NETosis in neutrophils [109]. Polydatin (PD) significantly inhibited the formation of NETs in myelo-derived neutrophils in RA patients as well as reduced the deposition of NETs in the ankle of CIA mice [110]. Compound Simiaoyong’an Decoction effectively inhibited arthritis in CIA mice by promoting neutrophil apoptosis, reducing ROS production and NETosis [111]. In conclusion, the above studies provide references and ideas for exploring new drug-target strategies for RA (Table 2).
In conclusion, in this paper, we discussed the multiple mechanisms of NETs in the pathogenesis of RA. Neutrophils participate in the immunoinflammation of RA in the form of NETosis. NETs may also be an important source of autoantigens, mediate the formation of osteoclasts, and are closely related to RA susceptibility factors. These may provide more insights into the occurrence and development of RA. In addition, the presence of NETs and their related components may provide potential biomarkers and indicators, and further studies of inhibition of NETosis processes and disruption of interactions between various stimuli will help identify new therapeutic targets and strategies. Nevertheless, experimental studies are still needed here to evaluate the efficacy and value of various NETosis-derived product assays as biomarkers for predicting RA and to assist in assessing drug efficacy. Given the differences in immune system and physiologic functions between mouse models and humans, more clinical studies are needed in the future to confirm the efficacy of various drugs or therapies that inhibit aberrant NETosis or related molecules.
Acknowledgement: We take thankful pleasure in acknowledging the unsparing assistance of all participants.
Funding Statement: This work was supported by grants from the National Traditional Chinese Medicine Inheritance and Innovation Project Fund (Development and Reform Office [2022] 366), National Key Discipline of Traditional Chinese Medicine (Traditional Chinese Medicine [2023] No. 85), the Ministry of Science and Technology National Key Research and Development Program Chinese Medicine Modernization Research Key Project (2018YFC1705204), National Nature Fund Program (82074373, 82274490, 82205090), and Anhui Provincial Laboratory of Applied Basis and Development of Internal Medicine of Modern Traditional Chinese Medicine (2016080503B041).
Author Contributions: The authors confirm contribution to the paper as follows: study conception and design: Yang Li and Jian Liu; data collection: Yuedi Hu, Chengzhi Cong; analysis and interpretation of results: Qiao Zhou, Yiming Chen; draft manuscript preparation: Yang Li; final approval of the manuscript: Jian Liu. All authors reviewed the results and approved the final version of the manuscript.
Availability of Data and Materials: The datasets generated during the current study are available from the corresponding author on reasonable request.
Ethics Approval: Not applicable.
Conflicts of Interest: The authors declare that they have no conflicts of interest to report regarding the present study.
References
1. Gravallese EM, Firestein GS. Rheumatoid arthritis—common origins, divergent mechanisms. New Engl J Med. 2023;388(6):529–42. [Google Scholar] [PubMed]
2. Wen J, Liu J, Wan L, Wang F. Long noncoding RNA/circular RNA regulates competitive endogenous RNA networks in rheumatoid arthritis: molecular mechanisms and traditional Chinese medicine therapeutic significances. Ann Med. 2023;55(1):973–89. [Google Scholar] [PubMed]
3. Sun Y, Liu J, Xin L, Wen J, Zhou Q, Chen X, et al. Traditional Chinese medicine is associated with reduced risk of readmission in rheumatoid arthritis patients with anemia: a retrospective cohort study. Evid Based Complement Alternat Med. 2022;2022:4553985. [Google Scholar] [PubMed]
4. Blagov AV, Grechko AV, Nikiforov NG, Zhuravlev AD, Sadykhov NK, Orekhov AN. Effects of metabolic disorders in immune cells and synoviocytes on the development of rheumatoid arthritis. Metabolites. 2022;12(7):634. [Google Scholar] [PubMed]
5. Coutant F, Miossec P. Evolving concepts of the pathogenesis of rheumatoid arthritis with focus on the early and late stages. Curr Opin Rheumatol. 2020;32(1):57–63. [Google Scholar] [PubMed]
6. Myasoedova E, Davis J, Matteson EL, Crowson CS. Is the epidemiology of rheumatoid arthritis changing? Results from a population-based incidence study, 1985–2014. Ann Rheum Dis. 2020;79(4):440–4. [Google Scholar] [PubMed]
7. Shi G, Liao X, Lin Z, Liu W, Luo X, Zhan H, et al. Estimation of the global prevalence, incidence, years lived with disability of rheumatoid arthritis in 2019 and forecasted incidence in 2040: results from the global burden of disease study 2019. Clin Rheumatol. 2023;42(9):2297–309. [Google Scholar] [PubMed]
8. Liu Y, Jia X, Yang Z, Liu R. Association of caspase-1 gene polymorphisms with rheumatoid arthritis risk in a Chinese Han population. Cytokine. 2023;162(6):156101. [Google Scholar] [PubMed]
9. Alivernini S, Firestein GS, McInnes IB. The pathogenesis of rheumatoid arthritis. Immunity. 2022;55(12):2255–70. [Google Scholar] [PubMed]
10. Irwandi RA, Chiesa ST, Hajishengallis G, Papayannopoulos V, Deanfield JE, D’Aiuto F. The roles of neutrophils linking periodontitis and atherosclerotic cardiovascular diseases. Front Immunol. 2022;13:915081. [Google Scholar] [PubMed]
11. Wright HL, Lyon M, Chapman EA, Moots RJ, Edwards SW. Rheumatoid arthritis synovial fluid neutrophils drive inflammation through production of chemokines, reactive oxygen species, and neutrophil extracellular traps. Front Immunol. 2020;11:584116. [Google Scholar] [PubMed]
12. Bach M, Moon J, Moore R, Pan T, Nelson JL, Lood C. A neutrophil activation biomarker panel in prognosis and monitoring of patients with rheumatoid arthritis. Arthritis Rheumatol. 2020;72(1):47–56. [Google Scholar] [PubMed]
13. Fajgenbaum DC, June CH. Cytokine storm. N Engl J Med. 2020;383(23):2255–73. [Google Scholar] [PubMed]
14. Guo Y, Zeng H, Gao C. The role of neutrophil extracellular traps in central nervous system diseases and prospects for clinical application. Oxid Med Cell Longev. 2021;2021:9931742. [Google Scholar] [PubMed]
15. Brinkmann V, Reichard U, Goosmann C, Fauler B, Uhlemann Y, Weiss DS, et al. Neutrophil extracellular traps kill bacteria. Science. 2004;303(5663):1532–5. [Google Scholar] [PubMed]
16. Masucci MT, Minopoli M, Del Vecchio S, Carriero MV. The emerging role of neutrophil extracellular traps (NETs) in tumor progression and metastasis. Front Immunol. 2020;11:1749. [Google Scholar] [PubMed]
17. Sadeghi M, Dehnavi S, Jamialahmadi T, Johnston TP, Sahebkar A. Neutrophil extracellular trap: a key player in the pathogenesis of autoimmune diseases. Int Immunopharmacol. 2023;116(1):109843. [Google Scholar] [PubMed]
18. Wigerblad G, Kaplan MJ. Neutrophil extracellular traps in systemic autoimmune and autoinflammatory diseases. Nat Rev Immunol. 2023;23(5):274–88. [Google Scholar] [PubMed]
19. Damascena HL, Silveira WAA, Castro MS, Fontes W. Neutrophil activated by the famous and potent PMA (phorbol myristate acetate). Cells. 2022;11(18):2889. [Google Scholar] [PubMed]
20. Chen JL, Tong Y, Zhu Q, Gao LQ, Sun Y. Neutrophil extracellular traps induced by Porphyromonas gingivalis lipopolysaccharide modulate inflammatory responses via a Ca2+-dependent pathway. Arch Oral Biol. 2022;141(6):105467. [Google Scholar] [PubMed]
21. Erpenbeck L, Gruhn AL, Kudryasheva G, Günay G, Meyer D, Busse J, et al. Effect of adhesion and substrate elasticity on neutrophil extracellular trap formation. Front Immunol. 2019;10:2320. [Google Scholar] [PubMed]
22. Song W, Ye J, Pan N, Tan C, Herrmann M. Neutrophil extracellular traps tied to rheumatoid arthritis: points to ponder. Front Immunol. 2020;11:578129. [Google Scholar] [PubMed]
23. Thiam HR, Wong SL, Qiu R, Kittisopikul M, Vahabikashi A, Goldman AE, et al. NETosis proceeds by cytoskeleton and endomembrane disassembly and PAD4-mediated chromatin decondensation and nuclear envelope rupture. Proc Natl Acad Sci USA. 2020;117(13):7326–37. [Google Scholar] [PubMed]
24. Bonaventura A, Liberale L, Carbone F, Vecchié A, Diaz-Cañestro C, Camici GG, et al. The pathophysiological role of neutrophil extracellular traps in inflammatory diseases. Thromb Haemostasis. 2018;118(1):6–27. [Google Scholar]
25. Douda DN, Khan MA, Grasemann H, Palaniyar N. SK3 channel and mitochondrial ROS mediate NADPH oxidase-independent NETosis induced by calcium influx. Proc Natl Acad Sci USA. 2015;112(9):2817–22. [Google Scholar] [PubMed]
26. Zhang L, Yuan Y, Xu Q, Jiang Z, Chu CQ. Contribution of neutrophils in the pathogenesis of rheumatoid arthritis. J Biomed Res. 2020;34(2):86–93. [Google Scholar]
27. Wang W, Peng W, Ning X. Increased levels of neutrophil extracellular trap remnants in the serum of patients with rheumatoid arthritis. Int J Rheum Dis. 2018;21(2):415–21. [Google Scholar] [PubMed]
28. Zeng MY, Miralda I, Armstrong CL, Uriarte SM, Bagaitkar J. The roles of NADPH oxidase in modulating neutrophil effector responses. Mol Oral Microbiol. 2019;34(2):27–38. [Google Scholar] [PubMed]
29. Navrátilová A, Andrés Cerezo L, Hulejova H, Bečvář V, Tomčík M, Komarc M, et al. IL-40: a new B cell-associated cytokine up-regulated in rheumatoid arthritis decreases following the rituximab therapy and correlates with disease activity, autoantibodies, and netosis. Front Immunol. 2021;12:745523. [Google Scholar]
30. Borges L, Pithon-Curi TC, Curi R, Hatanaka E. COVID-19 and neutrophils: the relationship between hyperinflammation and neutrophil extracellular traps. Mediat Inflamm. 2020;2020:8829674. [Google Scholar]
31. Masoumi M, Bashiri H, Khorramdelazad H, Barzaman K, Hashemi N, Sereshki HA, et al. Destructive roles of fibroblast-like synoviocytes in chronic inflammation and joint damage in rheumatoid arthritis. Inflamm. 2021;44(2):466–79. [Google Scholar]
32. Pan W, Xin Q, Xu J, He J, Chen Z, Hu X, et al. IgD enhances the release of neutrophil extracellular traps (NETs) via FcδR in rheumatoid arthritis patients. Int Immunopharmacol. 2023;114:109484. [Google Scholar] [PubMed]
33. Carmona-Rivera C, Carlucci PM, Moore E, Lingampalli N, Uchtenhagen H, James E, et al. Synovial fibroblast-neutrophil interactions promote pathogenic adaptive immunity in rheumatoid arthritis. Sci Immunol. 2017;2(10):eaag3358. [Google Scholar] [PubMed]
34. Tillack K, Breiden P, Martin R, Sospedra M. T lymphocyte priming by neutrophil extracellular traps links innate and adaptive immune responses. J Immunol. 2012;188(7):3150–9. [Google Scholar] [PubMed]
35. Karmakar U, Vermeren S. Crosstalk between B cells and neutrophils in rheumatoid arthritis. Immunol. 2021;164(4):689–700. [Google Scholar]
36. Aletaha D, Neogi T, Silman AJ, Funovits J, Felson DT, Bingham COIII, et al. 2010 rheumatoid arthritis classification criteria: an American College of Rheumatology/European League Against Rheumatism collaborative initiative. Arthritis Rheum. 2010;62(9):2569–81. [Google Scholar] [PubMed]
37. Iyengar KP, Vaish A, Nune A. Anti-cyclic citrullinated peptide antibody (ACPA) and Rheumatoid arthritis: clinical relevance. J Clin Orthopaed Trauma. 2022;24(9285):101729. [Google Scholar]
38. Petrovská N, Prajzlerová K, Vencovský J, Šenolt L, Filková M. The pre-clinical phase of rheumatoid arthritis: from risk factors to prevention of arthritis. Autoimmun Rev. 2021;20(5):102797. [Google Scholar]
39. Okamato Y, Ghosh T, Okamoto T, Schuyler RP, Seifert J, Charry LL, et al. Subjects at-risk for future development of rheumatoid arthritis demonstrate a PAD4-and TLR-dependent enhanced histone H3 citrullination and proinflammatory cytokine production in CD14hi monocytes. J Autoimmun. 2021;117(5):102581. [Google Scholar] [PubMed]
40. Wu S, Peng W, Liang X, Wang W. Anti-citrullinated protein antibodies are associated with neutrophil extracellular trap formation in rheumatoid arthritis. J Clin Lab Anal. 2021;35(3):e23662. [Google Scholar] [PubMed]
41. Okamoto Y, Devoe S, Seto N, Minarchick V, Wilson T, Rothfuss HM, et al. Association of sputum neutrophil extracellular trap subsets with IgA anti-citrullinated protein antibodies in subjects at risk for rheumatoid arthritis. Arthritis Rheumatol. 2022;74(1):38–48. [Google Scholar] [PubMed]
42. Demoruelle MK, Harrall KK, Ho L, Purmalek MM, Seto NL, Rothfuss HM, et al. Anti-citrullinated protein antibodies are associated with neutrophil extracellular traps in the sputum in relatives of rheumatoid arthritis patients. Arthritis Rheumatol. 2017;69(6):1165–75. [Google Scholar] [PubMed]
43. Pratesi F, Dioni I, Tommasi C, Alcaro MC, Paolini I, Barbetti F, et al. Antibodies from patients with rheumatoid arthritis target citrullinated histone 4 contained in neutrophils extracellular traps. Ann Rheum Dis. 2014;73(7):1414–22. [Google Scholar] [PubMed]
44. Elsawy NA, Mohamed RA, Ghazala RA, Abdelshafy MA, Elnemr R. Anti-carbamylated protein antibodies in premenopausal rheumatoid arthritis women: relation to disease activity and bone loss. Rheumatol. 2021;60(3):1419–28. [Google Scholar]
45. Wang L, Hua L, Hong X, Chen F, Du H. Association of serum anti-carbamylated protein antibodies with disease activity and bone loss in rheumatoid arthritis. Clin Chim Acta. 2023;546(184):117371. [Google Scholar] [PubMed]
46. O’Neil LJ, Oliveira CB, Wang X, Navarrete M, Barrera-Vargas A, Merayo-Chalico J, et al. Neutrophil extracellular trap-associated carbamylation and histones trigger osteoclast formation in rheumatoid arthritis. Ann Rheum Dis. 2023;82(5):630–8. [Google Scholar] [PubMed]
47. O’Neil LJ, Barrera-Vargas A, Sandoval-Heglund D, Merayo-Chalico J, Aguirre-Aguilar E, Aponte AM, et al. Neutrophil-mediated carbamylation promotes articular damage in rheumatoid arthritis. Sci Adv. 2020;6(44):eabd2688. [Google Scholar] [PubMed]
48. Carmona-Rivera C, Carlucci PM, Goel RR, James E, Brooks SR, Rims C, et al. Neutrophil extracellular traps mediate articular cartilage damage and enhance cartilage component immunogenicity in rheumatoid arthritis. JCI Insight. 2020;5(13):691. [Google Scholar]
49. Berthelot JM, Bandiaky ON, Le Goff B, Amador G, Chaux AG, Soueidan A, et al. Another look at the contribution of oral microbiota to the pathogenesis of rheumatoid arthritis: a narrative review. Microorganisms. 2021;10(1):59. [Google Scholar] [PubMed]
50. Qian Y, Zhang L, Wu DJ, Xie Z, Wen C, Mao Y. Genetic predisposition to smoking is associated with risk of rheumatoid arthritis: a Mendelian randomization study. Arthritis Res Ther. 2020;22(1):1–5. [Google Scholar]
51. Ahmadi P, Mahmoudi M, Kheder RK, Faraj TA, Mollazadeh S, Abdulabbas HS, et al. Impacts of Porphyromonas gingivalis periodontitis on rheumatoid arthritis autoimmunity. Int Immunopharmacol. 2023;118:109936. [Google Scholar] [PubMed]
52. Kaneko C, Kobayashi T, Ito S, Sugita N, Murasawa A, Nakazono K, et al. Circulating levels of carbamylated protein and neutrophil extracellular traps are associated with periodontitis severity in patients with rheumatoid arthritis: a pilot case-control study. PLoS One. 2018;13(2):e0192365. [Google Scholar] [PubMed]
53. Oliveira SR, de Arruda JAA, Schneider AH, Carvalho VF, Machado CC, Corrêa JD, et al. Are neutrophil extracellular traps the link for the cross-talk between periodontitis and rheumatoid arthritis physiopathology? Rheumatol. 2022;61(1):174–84. [Google Scholar]
54. Hedström AK, Stawiarz L, Klareskog L, Alfredsson L. Smoking and susceptibility to rheumatoid arthritis in a Swedish population-based case-control study. Eur J Epidemiol. 2018;33(4):415–23. [Google Scholar]
55. Wouters F, Maurits MP, van Boheemen L, Verstappen M, Mankia K, Matthijssen XME, et al. Determining in which pre-arthritis stage HLA-shared epitope alleles and smoking exert their effect on the development of rheumatoid arthritis. Ann Rheum Dis. 2022;81(1):48–55. [Google Scholar] [PubMed]
56. Hosseinzadeh A, Thompson PR, Segal BH, Urban CF. Nicotine induces neutrophil extracellular traps. J Leucocyte Biol. 2016;100(5):1105–12. [Google Scholar] [PubMed]
57. Lee KH, Kronbichler A, Park DD, Park Y, Moon H, Kim H, et al. Neutrophil extracellular traps (NETs) in autoimmune diseases: a comprehensive review. Autoimmun Rev. 2017;16(11):1160–73. [Google Scholar] [PubMed]
58. Ytterberg AJ, Joshua V, Reynisdottir G, Tarasova NK, Rutishauser D, Ossipova E, et al. Shared immunological targets in the lungs and joints of patients with rheumatoid arthritis: identification and validation. Ann Rheum Dis. 2015;74(9):1772–7. [Google Scholar] [PubMed]
59. Demoruelle MK, Bowers E, Lahey LJ, Sokolove J, Purmalek M, Seto NL, et al. Antibody responses to citrullinated and noncitrullinated antigens in the sputum of subjects with rheumatoid arthritis and subjects at risk for development of rheumatoid arthritis. Arthritis Rheumatol. 2018;70(4):516–27. [Google Scholar] [PubMed]
60. Gonzalez-Aparicio M, Alfaro C. Influence of interleukin-8 and neutrophil extracellular trap (NET) formation in the tumor microenvironment: is there a pathogenic role? J Immunol Res. 2019;2019(8):6252138. [Google Scholar] [PubMed]
61. Shu Q, Zhang N, Liu Y, Wang X, Chen J, Xie H, et al. IL-8 triggers neutrophil extracellular trap formation through an nicotinamide adenine dinucleotide phosphate oxidase- and mitogen-activated protein kinase pathway-dependent mechanism in uveitis. Invest Ophthalmol Vis Sci. 2023;64(13):19. [Google Scholar] [PubMed]
62. Shang A, Gu C, Zhou C, Yang Y, Chen C, Zeng B, et al. Exosomal KRAS mutation promotes the formation of tumor-associated neutrophil extracellular traps and causes deterioration of colorectal cancer by inducing IL-8 expression. Cell Commun Signal. 2020;18(1):52. [Google Scholar] [PubMed]
63. Abrams S, Morton B, Alhamdi Y, Alsabani M, Cheng Z, Lane S, et al. A novel assay of neutrophil extracellular trap (NET) formation identifies anti-IL-8 therapies to reduce disseminated intravascular coagulation and mortality in the intensive care unit. Clin Med. 2020;20(Suppl 2):s114–5. [Google Scholar]
64. Cecchi I, Arias de la Rosa I, Menegatti E, Roccatello D, Collantes-Estevez E, Lopez-Pedrera C, et al. Neutrophils: novel key players in rheumatoid arthritis. Current and future therapeutic targets. Autoimmun Rev. 2018;17(11):1138–49. [Google Scholar] [PubMed]
65. Metzler KD, Fuchs TA, Nauseef WM, Reumaux D, Roesler J, Schulze I, et al. Myeloperoxidase is required for neutrophil extracellular trap formation: implications for innate immunity. Blood J Am Soc Hematol. 2011;117(3):953–9. [Google Scholar]
66. Fernandes RMSN, NPd Silva, Sato EI. Increased myeloperoxidase plasma levels in rheumatoid arthritis. Rheumatol Int. 2012;32(6):1605–9. [Google Scholar] [PubMed]
67. Odobasic D, Yang Y, Muljadi RC, O’Sullivan KM, Kao W, Smith M, et al. Endogenous myeloperoxidase is a mediator of joint inflammation and damage in experimental arthritis. Arthritis Rheumatol. 2014;66(4):907–17. [Google Scholar] [PubMed]
68. Etwebi Z, Landesberg G, Preston K, Eguchi S, Scalia R. Mechanistic role of the calcium-dependent protease calpain in the endothelial dysfunction induced by MPO (myeloperoxidase). Hypertension. 2018;71(4):761–70. [Google Scholar] [PubMed]
69. Crocetti L, Quinn MT, Schepetkin IA, Giovannoni MP. A patenting perspective on human neutrophil elastase (HNE) inhibitors (2014–2018) and their therapeutic applications. Expert Opin Ther Pat. 2019;29(7):555–78. [Google Scholar] [PubMed]
70. Zhao P, Lieu T, Barlow N, Sostegni S, Haerteis S, Korbmacher C, et al. Neutrophil elastase activates protease-activated receptor-2 (PAR2) and transient receptor potential vanilloid 4 (TRPV4) to cause inflammation and pain. J Biol Chem. 2015;290(22):13875–87. [Google Scholar] [PubMed]
71. Okeke EB, Louttit C, Fry C, Najafabadi AH, Han K, Nemzek J, et al. Inhibition of neutrophil elastase prevents neutrophil extracellular trap formation and rescues mice from endotoxic shock. Biomaterials. 2020;238(80):119836. [Google Scholar] [PubMed]
72. Muley MM, Reid AR, Botz B, Boelcskei K, Helyes Z, McDougall JJ. Neutrophil elastase induces inflammation and pain in mouse knee joints via activation of proteinase-activated receptor-2. Br J Pharmacol. 2016;173(4):766–77. [Google Scholar] [PubMed]
73. Mergaert AM, Bawadekar M, Nguyen TQ, Massarenti L, Holmes CL, Rebernick R, et al. Reduced anti-histone antibodies and increased risk of rheumatoid arthritis associated with a single nucleotide polymorphism in PADI4 in North Americans. Int J Mol Sci. 2019;20(12):3093. [Google Scholar] [PubMed]
74. Turunen S, Huhtakangas J, Nousiainen T, Valkealahti M, Melkko J, Risteli J, et al. Rheumatoid arthritis antigens homocitrulline and citrulline are generated by local myeloperoxidase and peptidyl arginine deiminases 2, 3 and 4 in rheumatoid nodule and synovial tissue. Arthritis Res Ther. 2016;18(1):239. [Google Scholar] [PubMed]
75. Xu M, Du R, Xing W, Chen X, Wan J, Wang S, et al. Platelets derived citrullinated proteins and microparticles are potential autoantibodies ACPA targets in RA patients. Front Immunol. 2023;14:1084283. [Google Scholar] [PubMed]
76. Krishnamurthy A, Circiumaru A, Sun J, Kisten Y, Damberg P, Sakuraba K, et al. Combination of two monoclonal anti-citrullinated protein antibodies induced tenosynovitis, pain, and bone loss in mice in a peptidyl arginine deiminase-4-dependent manner. Arthritis Rheumatol. 2023;75(2):164–70. [Google Scholar] [PubMed]
77. Jonsson MK, Kantyka T, Falkowski K, Aliko A, Aga AB, Lillegraven S, et al. Peptidylarginine deiminase 4 (PAD4) activity in early rheumatoid arthritis. Scand J Rheumatol. 2020;49(2):87–95. [Google Scholar] [PubMed]
78. Gómez-Bañuelos E, Shi J, Wang H, Danila MI, Bridges SL, Giles JT, et al. Heavy chain constant region usage in antibodies to peptidylarginine deiminase 4 as a marker of disease subsets in rheumatoid arthritis. Arthritis Rheumatol. 2022;74(11):1746–54. [Google Scholar]
79. Palterer B, Vitiello G, Del Carria M, D’Onofrio B, Martinez-Prat L, Mahler M, et al. Anti-protein arginine deiminase antibodies are distinctly associated with joint and lung involvement in rheumatoid arthritis. Rheumatol. 2023;62(7):2410–7. [Google Scholar]
80. Demoruelle MK, Wang H, Davis RL, Visser A, Hoang J, Norris JM, et al. Anti-peptidylarginine deiminase-4 antibodies at mucosal sites can activate peptidylarginine deiminase-4 enzyme activity in rheumatoid arthritis. Arthritis Res Ther. 2021;23(1):163. [Google Scholar] [PubMed]
81. Zhang G, Xu J, Du D, Liu Y, Dai L, Zhao Y. Diagnostic values, association with disease activity and possible risk factors of anti-PAD4 in rheumatoid arthritis: a meta-analysis. Rheumatol. 2023:kead545. [Google Scholar]
82. Ronkina N, Gaestel M. MAPK-activated protein kinases: servant or partner? Annu Rev Biochem. 2022;91(1):505–40. [Google Scholar] [PubMed]
83. Dömer D, Walther T, Möller S, Behnen M, Laskay T. Neutrophil extracellular traps activate proinflammatory functions of human neutrophils. Front Immunol. 2021;12:636954. [Google Scholar]
84. Ji W, Zhang X, Sang C, Wang H, Zhou K, Zhang Y, et al. Punicalin attenuates LPS-induced acute lung injury by inhibiting inflammatory cytokine production and MAPK/NF-κB signaling in mice. Heliyon. 2023;9(4):e15434. [Google Scholar] [PubMed]
85. Zhou X, Yang L, Fan X, Zhao X, Chang N, Yang L, et al. Neutrophil chemotaxis and NETosis in murine chronic liver injury via cannabinoid receptor 1/Gαi/o/ROS/p38 MAPK signaling pathway. Cells. 2020;9(2):373. [Google Scholar] [PubMed]
86. Jati GAK, Assihhah N, Wati AA, Salasia SIO. Immunosuppression by piperine as a regulator of the NLRP3 inflammasome through MAPK/NF-κB in monosodium urate-induced rat gouty arthritis. Vet World. 2022;15(2):288–98. [Google Scholar] [PubMed]
87. Pérez-Sánchez C, Ruiz-Limón P, Aguirre M, Jiménez-Gómez Y, Arias-de la Rosa I, Ábalos-Aguilera M, et al. Diagnostic potential of NETosis-derived products for disease activity, atherosclerosis and therapeutic effectiveness in rheumatoid arthritis patients. J Autoimmun. 2017;82(5):31–40. [Google Scholar]
88. Sur Chowdhury C, Giaglis S, Walker UA, Buser A, Hahn S, Hasler P. Enhanced neutrophil extracellular trap generation in rheumatoid arthritis: analysis of underlying signal transduction pathways and potential diagnostic utility. Arthritis Res Ther. 2014;16(3):1–14. [Google Scholar]
89. de Bont CM, Stokman ME, Faas P, Thurlings RM, Boelens WC, Wright HL, et al. Autoantibodies to neutrophil extracellular traps represent a potential serological biomarker in rheumatoid arthritis. J Autoimmun. 2020;113(2010):102484. [Google Scholar] [PubMed]
90. Kessenbrock K, Krumbholz M, Schönermarck U, Back W, Gross WL, Werb Z, et al. Netting neutrophils in autoimmune small-vessel vasculitis. Nat Med. 2009;15(6):623–5. [Google Scholar] [PubMed]
91. Demkow U. Molecular mechanisms of neutrophil extracellular trap (NETs) degradation. Int J Mol Sci. 2023;24(5):4896. [Google Scholar] [PubMed]
92. Kolaczkowska E, Jenne CN, Surewaard BG, Thanabalasuriar A, Lee WY, Sanz MJ, et al. Molecular mechanisms of NET formation and degradation revealed by intravital imaging in the liver vasculature. Nat Commun. 2015;6(1):6673. [Google Scholar] [PubMed]
93. Angeletti A, Volpi S, Bruschi M, Lugani F, Vaglio A, Prunotto M, et al. Neutrophil extracellular traps-DNase balance and autoimmunity. Cells. 2021;10(10):2667. [Google Scholar] [PubMed]
94. Wang CL, Wang Y, Jiang QL, Zeng Y, Yao QP, Liu X, et al. DNase I and sivelestat ameliorate experimental hindlimb ischemia-reperfusion injury by eliminating neutrophil extracellular traps. J Inflamm Res. 2023;16:707–21. [Google Scholar] [PubMed]
95. Zhang H, Wang Y, Onuma A, He J, Wang H, Xia Y, et al. Neutrophils extracellular traps inhibition improves PD-1 blockade immunotherapy in colorectal cancer. Cancers. 2021;13(21):5333. [Google Scholar] [PubMed]
96. Wang N, Ma J, Song W, Zhao C. An injectable hydrogel to disrupt neutrophil extracellular traps for treating rheumatoid arthritis. Drug Deliv. 2023;30(1):2173332. [Google Scholar] [PubMed]
97. Wan T, Zhao Y, Fan F, Hu R, Jin X. Dexamethasone inhibits S. aureus-induced neutrophil extracellular pathogen-killing mechanism, possibly through toll-like receptor regulation. Front Immunol. 2017;8:60. [Google Scholar] [PubMed]
98. Kaundal U, Khullar A, Leishangthem B, Jain S, Dhooria A, Saikia B, et al. The effect of methotrexate on neutrophil reactive oxygen species and CD177 expression in rheumatoid arthritis. Clin Exp Rheumatol. 2021;39(3):479–86. [Google Scholar] [PubMed]
99. Kawaguchi H, Matsumoto I, Osada A, Kurata I, Ebe H, Tanaka Y, et al. Peptidyl arginine deiminase inhibition suppresses arthritis via decreased protein citrullination in joints and serum with the downregulation of interleukin-6. Mod Rheumatol. 2019;29(6):964–9. [Google Scholar] [PubMed]
100. Gajendran C, Fukui S, Sadhu NM, Zainuddin M, Rajagopal S, Gosu R, et al. Alleviation of arthritis through prevention of neutrophil extracellular traps by an orally available inhibitor of protein arginine deiminase 4. Sci Rep. 2023;13(1):3189. [Google Scholar] [PubMed]
101. Ruiz-Limon P, Ortega R, de la Rosa IA, Del Carmen Abalos-Aguilera M, Perez-Sanchez C, Jimenez-Gomez Y, et al. Tocilizumab improves the proatherothrombotic profile of rheumatoid arthritis patients modulating endothelial dysfunction, NETosis, and inflammation. Transl Res. 2017;183:87–103. [Google Scholar] [PubMed]
102. Gupta AK, Giaglis S, Hasler P, Hahn S. Efficient neutrophil extracellular trap induction requires mobilization of both intracellular and extracellular calcium pools and is modulated by cyclosporine A. PLoS One. 2014;9(5):e97088. [Google Scholar] [PubMed]
103. Mutua V, Gershwin LJ. A review of neutrophil extracellular traps (NETs) in disease: potential anti-NETs therapeutics. Clin Rev Allergy Immunol. 2021;61(2):194–211. [Google Scholar] [PubMed]
104. Zhao J, Liu Y, Shi X, Dang J, Liu Y, Li S, et al. Infusion of GMSCs relieves autoimmune arthritis by suppressing the externalization of neutrophil extracellular traps via PGE2-PKA-ERK axis. J Adv Res. 2023. doi:https://doi.org/10.1016/j.jare.2023.05.001. [Google Scholar] [PubMed] [CrossRef]
105. Liu X, Wang Z, Qian H, Tao W, Zhang Y, Hu C, et al. Natural medicines of targeted rheumatoid arthritis and its action mechanism. Front Immunol. 2022;13:945129. [Google Scholar] [PubMed]
106. Li TP, Zhang AH, Miao JH, Sun H, Yan GL, Wu FF, et al. Applications and potential mechanisms of herbal medicines for rheumatoid arthritis treatment: a systematic review. RSC Adv. 2019;9(45):26381–92. [Google Scholar] [PubMed]
107. Zhu M, Yuan K, Lu Q, Zhu Q, Zhang S, Li X, et al. Emodin ameliorates rheumatoid arthritis by promoting neutrophil apoptosis and inhibiting neutrophil extracellular trap formation. Mol Immunol. 2019;112:188–97. [Google Scholar] [PubMed]
108. Li X, Yuan K, Zhu Q, Lu Q, Jiang H, Zhu M, et al. Andrographolide ameliorates rheumatoid arthritis by regulating the apoptosis-NETosis balance of neutrophils. Int J Mol Sci. 2019;20(20):5035. [Google Scholar] [PubMed]
109. Huang G, Yuan K, Zhu Q, Zhang S, Lu Q, Zhu M, et al. Triptolide inhibits the inflammatory activities of neutrophils to ameliorate chronic arthritis. Mol Immunol. 2018;101:210–20. [Google Scholar] [PubMed]
110. Yang F, Luo X, Luo G, Zhai Z, Zhuang J, He J, et al. Inhibition of NET formation by polydatin protects against collagen-induced arthritis. Int Immunopharmacol. 2019;77:105919. [Google Scholar] [PubMed]
111. Jie SS, Sun HJ, Liu JX, Gao Y, Bai D, Zhu LL, et al. Simiao Yong’an decoction ameliorates murine collagen-induced arthritis by modulating neutrophil activities: an in vitro and in vivo study. J Ethnopharmacol. 2023;305(7):116119. [Google Scholar] [PubMed]
112. Lu Q, Jiang H, Zhu Q, Xu J, Cai Y, Huo G, et al. Tetrandrine ameliorates rheumatoid arthritis in mice by alleviating neutrophil activities. Evid Based Complement Alternat Med. 2022;2022(6):1–13. [Google Scholar]
113. Jiang H, Lu Q, Xu J, Huo G, Cai Y, Geng S, et al. Sinomenine ameliorates adjuvant-induced arthritis by inhibiting the autophagy/NETosis/inflammation axis. Sci Rep. 2023;13(1):3933. [Google Scholar] [PubMed]
114. Yuan K, Zhu Q, Lu Q, Jiang H, Zhu M, Li X, et al. Quercetin alleviates rheumatoid arthritis by inhibiting neutrophil inflammatory activities. J Nutr Biochem. 2020;84(10055):108454. [Google Scholar] [PubMed]
Cite This Article
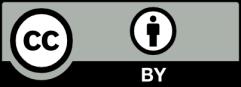