Open Access
ARTICLE
Fang-Xia-Dihuang decoction inhibits breast cancer progression induced by psychological stress via down-regulation of PI3K/AKT and JAK2/STAT3 pathways: An in vivo and a network pharmacology assessment
1 School of Graduate, Beijing University of Chinese Medicine, Beijing, 100029, China
2 Department of Breast, Beijing University of Chinese Medicine Third Affiliated Hospital, Beijing, 100029, China
3 Department of Pharmacy, Fangshan Hospital of Beijing University of Chinese Medicine, Beijing, 102488, China
4 Department of Breast, The First Hospital of Hunan University of Chinese Medicine, Changsha, 410007, China
5 Department of Chest and Breast, The Xiamen Hospital of Beijing Universality of Chinese Medicine, Xiamen, 361001, China
* Corresponding Authors: HONGQIAO FAN. Email: ; XIAOHUA PEI. Email:
# Lingyan Lv and Jing Zhao contributed equally to this work
BIOCELL 2023, 47(9), 1977-1994. https://doi.org/10.32604/biocell.2023.030742
Received 20 April 2023; Accepted 19 June 2023; Issue published 28 September 2023
Abstract
Background: The development and prognosis of breast cancer are intricately linked to psychological stress. In addition, depression is the most common psychological comorbidity among breast cancer survivors, and reportedly, Fang-Xia-Dihuang decoction (FXDH) can effectively manage depression in such patients. However, its pharmacological and molecular mechanisms remain obscure. Methods: Public databases were used for obtaining active components and related targets. Main active components were further verified by ultra-high-performance liquid chromatography-high-resolution mass spectrometry (UPLC-HRMS). Protein–protein interaction and enrichment analyses were taken to predict potential hub targets and related pathways. Molecule docking was used to understand the interactions between main compounds and hub targets. In addition, an animal model of breast cancer combined with depression was established to evaluate the intervention effect of FXDH and verify the pathways screened by network pharmacology. Results: 174 active components of FXDH and 163 intersection targets of FXDH, breast cancer, and depression were identified. Quercetin, methyl ferulate, luteolin, ferulaldehyde, wogonin, and diincarvilone were identified as the principal active components of FXDH. Protein–protein interaction and KEGG enrichment analyses revealed that the phosphoinositide-3-kinase–protein kinase B (PI3K/AKT) and Janus kinase/signal transducer and activator of transcription (JAK2/STAT3) signaling pathways played a crucial role in mediating the efficacy of FXDH for inhibiting breast cancer progression induced by depression. In addition, in vivo experiments revealed that FXDH ameliorated depression-like behavior in mice and inhibited excessive tumor growth in mice with breast cancer and depression. FXDH treatment downregulated the expression of epinephrine, PI3K, AKT, STAT3, and JAK2 compared with the control treatment (p < 0.05). Molecular docking verified the relationship between the six primary components of FXDH and the three most important targets, including phosphatidylinositol-4,5-bisphosphate 3-kinase catalytic subunit alpha (PIK3CA), AKT, and STAT3. Conclusion: This study provides a scientific basis to support the clinical application of FXDH for improving depression-like behavior and inhibiting breast cancer progression promoted by chronic stress. The therapeutic effects FXDH may be closely related to the PI3K/AKT and JAK2/STAT3 pathways. This finding helps better understand the regulatory mechanisms underlying the efficacy of FXDH.Keywords
Supplementary Material
Supplementary Material FileBreast cancer has surpassed lung cancer as the most prevalent malignant tumor worldwide and has become the leading cause of cancer death in women (Siegel et al., 2023). Reportedly, up to 32% of breast cancer survivors experience depression (Pilevarzadeh et al., 2019), and numerous studies have found that psychological stress can notably affect the development and progression of tumors (Cormanique et al., 2015; Kruk et al., 2019). For example, a meta-analysis of 163,363 individuals found that patients’ self-reported psychological pain scores were associated with cancer-related mortality (Batty et al., 2017). Moreover, another large meta-analysis of 282,203 patients with breast cancer has demonstrated that depression and anxiety are independent predictors of breast cancer recurrence and survival (Wang et al., 2020). The mechanism by which psychological stress promotes cancer progression is believed to be a complex process involving multiple pathways and targets, such as disorder of the neuroendocrine system, excessive secretion of stress hormones and receptor activation, disturbance of the immune system, alteration of the tumor microenvironment, and induction of tumor angiogenesis. All these processes promote the proliferation, invasion, and colonization of cancer cells (Thaker et al., 2006; Yang et al., 2019; Liu et al., 2022; Silva et al., 2022).
In China and a few other Asian countries, traditional Chinese medicine (TCM) has been widely used in the adjuvant breast cancer treatment (Jaradat et al., 2016; Hung et al., 2023). Specifically, TCM has been used to reduce inflammatory exudates after breast cancer surgery, prevent the cardiotoxicity of anthracycline drugs, and improve endocrine therapy-related hot flashes and sleep disorders (Xue et al., 2011; Zhu et al., 2018; Cao et al., 2022). According to our previous research, the decoction of the TCM compound Fangji Huangqi may inhibit the proliferation and metastasis of triple-negative breast cancer (Guo et al., 2022). Other TCM compounds have also been demonstrated to alleviate mental and neurological symptoms, such as depression. Xiaoyaosan, for example, may ameliorate depression-like behavior in animals by regulating factors such as the intestinal flora, inflammatory pathway, and hypothalamic Apelin-APJ system (Yan et al., 2018; Zhu et al., 2019; Jiao et al., 2021a; Yang et al., 2022). A study has found that Sinisan inhibits lung metastasis induced by chronic psychological stress by interfering with the GRP78/LRP5 stem cell signaling (Zheng et al., 2021).
The composition of FXDH is an improvement on the Fangji-Dihuang decoction recorded in the Golden Chamber, a classic TCM text. However, in clinical practice, the original formula has been used to primarily treat emotional disorders, particularly mental disorders caused by liver and kidney Yin deficiency, phlegm, and blood stagnation (Wang et al., 2018b; Li et al., 2022a). Accordingly, we augmented the potency of the original formula by adding herbs such as Prunella vulgaris, Gardenia jasminoides, and Pinellia ternata to alleviate depression and dispersing stagnation. In our clinical practice, this formula was found to have a therapeutic effect in patients with breast cancer with comorbid depression (BCCD). The composition of FXDH is presented in Table 1.
According to TCM, psychological emotion and the physical body are one unit and influence one another. FXDH primarily regulates abnormal mental emotions and improves the “soil environment” disorder caused by negative psychological stress. This way, FXDH inhibits the growth and spread of the “bad seeds,” named cancer. However, the potential pharmacological and molecular mechanisms underlying the activity of FXDH remain unknown.
Network pharmacology offers the advantage of integrating multi-level and multi-disciplinary biological information to reveal the correlation among complex diseases, syndromes, and drugs (e.g., TCM molecules)(Li and Zhang, 2013). This is consistent with the “holistic” nature of TCM. Molecular docking is a computer-based technology that allows the study of structural design. Docking of small-molecule drugs or compounds on the three-dimensional structures of related biological macromolecular targets allows the screening and identification of potential drug targets. This additionally helps elucidate the mechanisms underlying actions of the drug, according to the degree of binding tightness (Huang et al., 2018). The comprehensive application of these two virtual screening methods can improve the efficiency, cost-effectiveness, and pertinence of the TCM screening process (Jiao et al., 2021b). Currently, many studies have assessed the anti-breast cancer effects of TCM molecules using network pharmacology and molecular docking. TCM compounds or extracts may exert anti-tumor effects in patients with breast cancer via different mechanisms, including induction of apoptosis, anti-tumor angiogenesis, and improvement of drug resistance. These effects were primarily demonstrated for the active ingredients such as quercetin, luteolin, and tangerine (Pandi et al., 2022). However, existing virtual screening studies mainly focused on breast cancer, and few studies have assessed the effect of TCM compounds on breast cancer and comorbid depression. Accordingly, in the present study, network pharmacology and reverse molecular docking were employed to predict the bioactive compounds, target proteins, and signaling pathways of FXDH in the treatment of BCCD. The compounds screened using network pharmacology were quantitatively verified by UPLC-HRMS. In addition, a mice model of BCCD was established to observe the efficacy of FXDH and the mechanism underlying its effect. The study workflow is depicted in Fig. 1.
Figure 1: The workflow of the study.
Potential active constituents and targets of Fang-Xia-Dihuang
Using the online databases Traditional Chinese Medicine Systems Pharmacology Database and Analysis Platform(TCMSP, https://tcmspw.com/tcmsp.php) (Ru et al., 2014), HERB (http://herb.ac.cn/) (Fang et al., 2021), and Encyclopedia of Traditional Chinese Medicine (ETCM, http://www.tcmip.cn/ETCM/) (Xu et al., 2019) via a literature search, the active constituents of each herb in FXDH were evaluated. The molecular structures of the active constituents were obtained from the PubChem database (https://pubchem.ncbi.nlm.nih.gov/) (Dashti et al., 2019); these were then saved in 3Dsdf format and then imported into the SwissADME platform (http://www.swissadme.ch/) (Daina et al., 2017) for screening. The screening criteria for potential active compounds were as follows: gastrointestinal absorption was “high” and drug-likeness (Lipinski, Ghose, Veber, Egan, and Muegge) had at least two “Yes” values. The compounds that did not meet these criteria were reviewed in the scientific literature and those with noticeable pharmacological effects in BCCD were retained. Using the Swiss Target Prediction database (http://www.swisstargetprediction.ch/) (Daina et al., 2019), target proteins for the included compounds were identified. A probability of >0 was set as the condition for screening.
Screening candidate targets of breast cancer and depression
Possible targets for breast cancer and depression were obtained from GeneCards (https://www.genecards.org/) (Safran et al., 2010), DisGeNet (https://www.disgenet.org/home/) (Pinero et al., 2017), OMIM (https://www.omim.org/) (Amberger and Hamosh, 2017), and DrugBank (https://www.drugbank.ca/) (Wishart et al., 2018). The DrugBank database is based on evidence from clinical trials, whereas the other databases are based on literature. The four databases complement each other to allow for increased precision and search. For example, the terms “breast cancer,” “breast carcinoma,” and “mammary cancer” were used to search breast cancer-related genes, whereas “depression” and “depressive disorder” were used to identify depression-related genes. After combining the genes from the four databases and eliminating duplicates, breast cancer- and depression-related target datasets were generated. Genes were uniformly named using gene symbols from the UniProt protein database (https://www.uniprot.org/) (Soudy et al., 2020).
Construction of protein–protein interaction (PPI) network and screening of hub targets
Overlapping genes (OGEs) among FXDH, breast cancer, and depression targets were visualized using the E Venn tool (https://www.ehbio.com/test/venn/#/). The obtained OGEs were then inputted into the STRING database (https://string-db.org/) (Szklarczyk et al., 2019) with a threshold of confidence scores ≥0.9 and species restricted to Homo sapiens to generate the PPI network. Topological network analysis was conducted using Cytoscape 3.9.1 (Otasek et al., 2019) to visualize the PPI network. Hub targets were identified using a minimum criterion of having a node degree value of at least twice the median value.
Gene Ontology (GO) and Kyoto Encyclopedia of Genes and Genomes (KEGG) analyses
The ClusterProfiler package (Yu et al., 2012) from the Bioconductor R3.6.2 project was used for GO and KEGG analyses. The genes identified using GO analysis were classified according to their role in either biological process (BP), molecular function (MF), or cellular component (CC). The results of GO and KEGG analyses were screened based on p-value, with a screening threshold of p < 0.05. The top thirty genes were then selected for visualization using bubble diagrams. Using the Cytoscape software, the mechanism and targets of FXDH in BCCD were clarified, along with the component–target–pathway network.
The 3D conformations of the hub target proteins were acquired from the RCSB PDB database (https://www.rcsb.org/) (Goodsell et al., 2020), whereas structures of the active compounds were obtained from PubChem. The Surflex-dock module of SYBYL-X 2.1.1 (Jain, 2007) was used for molecular docking with an FXDH core compound ligand and a hub target protein receptor. This matching method is based on the similarity of structure and shape and is highly accurate, has a high positive rate, and has a rapid matching speed. In general, the higher the Totalscore value, the lower the free binding energy between the receptor and ligand, indicating greater stability. A Total score of ≥5.0 indicates good binding ability between the ligand and the target protein, whereas a Totalscore of ≥7.0 indicates robust docking activity.
Ultra-high-performance liquid chromatography–high-resolution mass spectrometry analysis of Fang-Xia-Dihuang
FXDH decoction was provided by the Pharmacy Department of the Xiamen Hospital of Traditional Chinese Medicine (Xiamen, China). Briefly, 420 g of decoction was weighed according to the ratio mentioned in Table 1. This was then mixed with distilled water at a ratio of 1:12 and then extracted at 90°C for 1.5 h. The filtrate obtained after three extractions was mixed and concentrated at 60°C in an oven, followed by vacuum drying. One gram of the dry powder was obtained from approximately 2.44 g of decoction.
The main chemical constituents of FXDH extract were assessed using a Vanquish UHPLC system (Thermo Scientific, Waltham, MA) equipped with an Accuity UPLC HSS-T3 column (100 × 2.1 mm; particle size: 1.8 μm size; Waters, Shanghai, China) at a temperature of 35°C. Mobile phase A was water + 0.1% formic acid, and mobile phase B was acetonitrile + 0.1% formic acid (LC-MS grade solvents, Fisher chemical). The samples were separated at a flow rate of 0.3 mL/min using the following gradient: 5% B for 1 min, 98% B for 16 min, 5% B for 0.2 min, and 5% B for 2.8 min. The UHPLC system was coupled with the Q-Exactive HFX mass spectrometer (Thermo Fisher Scientific, Bremen, Germany). Mass spectra were obtained using data-dependent acquisition mode under both positive and negative electrospray ionization settings, with a mass range of 90–1,300 m/z. The capillary and probe heater were set at 320°C and 370°C, respectively. This procedure was conducted for quality assurance, at the Shanghai Applied Protein Technology Co., Ltd. (Shanghai, China).
Experimental animals and treatment
Female Balb/c mice (6–8 weeks old) were purchased from Beijing HFK Bioscience Co., Ltd. (Beijing, China; license: SCXK (Beijing) 2020-0004) and raised under specific pathogen-free conditions at the Animal Laboratory Center of Xiamen University (license: SYXK (MIN) 2018-0010). All experimental procedures were conducted in strict adherence to the guidelines provided by the Welfare and Ethics Committee of Laboratory Animals of Xiamen University (XMULAC20200055).
After 1 week of adaptive feeding, Balb/c mice were randomly divided into six groups: the blank group (BG, 0.9% saline), the 4T1 breast cancer transplantation group (4T1, 0.9% saline), the corticosterone group (CORT, 0.9% saline), the 4T1 tumor transplantation + corticosterone group (4T1+CORT, 0.9% saline), the FXDH group (FXDH, 13.65 g/(kg · d), the dosage of crude drug is same as the clinical equivalent dosages), and the capecitabine chemotherapy group (CAP, 390 mg/(kg · d), same as the clinical equivalent dosages). As depicted in Fig. 2, mice were injected subcutaneously with 4T1 cells on day 0 and received daily corticosterone injections for 28 days (Zhao et al., 2008). Then, on day 7, FXDH or CAP was administered intragastrically until 21 days. Every week, the body weight of mice was recorded. Every 3 days, tumor size was measured using a vernier caliper, and tumor volume was calculated using the following equation:
Figure 2: Establishment of a mouse model of breast cancer with comorbid depression and timepoints indicating drug intervention and behavior test.
Volume (mm3) = 0.5 × [length (mm)] × [width (mm)]2
After 21 days of drug treatment, animals were sacrificed. Subsequently, the tumor tissues were resected, weighed, and photographed; in addition, spleen tissues were collected to detect epinephrine levels.
Sucrose preference test and open field test
Depression-like behavior in mice was assessed using the sucrose preference test (SPT) and the open field test (OFT). For SPT, the animals were housed in solitary confinement and were provided free access to water and food. During the 48 h of drinking training, two bottles each of 2% sucrose water and pure water were provided. The amount of water consumed from the two bottles over 8 h was measured (the bottles’ positions were switched after 24 h). Sucrose preference was determined using the following equation:
For OFT, mice were individually placed in a 40 × 40 × 40 cm box without a ceiling for 5 min. During this time, the mice were monitored to record the total moving distance, displacement speed, central area moving distance, and the number of central area entries using the TopScanLite 2.00 software (Clever Sys, USA).
Fresh tumor and hippocampal samples were fixed for 24 h using 4% paraformaldehyde. After dehydration with gradient concentration of alcohol, the tissues were fixed in paraffin wax and then sliced into 4-mm thick sections. The sections were then dewaxed with xylene and ethanol and were subsequently stained with hematoxylin and eosin (H&E), according to the instructions for H&E staining kit (Biyuntian, China). Finally, the sections were sealed with neutral resin. Light microscopy was used to visualize tissue histology.
RNA extraction and quantitative reverse transcription polymerase chain reaction (RT-qPCR)
Total RNA was extracted from tumor tissues using TRIzol reagent (Invitrogen, USA) and reverse transcribed to cDNA using the PrimeScript™ RT reagent Kit with gDNA Eraser (TaKaRa, Japan), per the manufacturer’s instructions. Subsequently, RT-qPCR was performed using TB Green™ Premix Ex Taq™ (TaKaRa, Japan) and the Real-time PCR Detection System (Roche-LightCycler 480, USA). The relative abundance of mRNA was determined utilizing GAPDH as the internal control, and the expression was determined using the 2−ΔΔCt method. The specific primers used (Sangon Biotech, Shanghai, China) are listed in Table 2.
Enzyme-linked immunosorbent assay (ELISA)
The expression of epinephrine in the spleen was determined using an ELISA kit (Senbeijia Biotechnology, Nanjing, China), as per the instructions provided by the manufacturer. Then, using a tissuelyser (MB-48L-1, Zhejiang, China), 50 mg of spleen tissue was accurately weighed and homogenized with 450 μL phosphate buffer (0.01 mol/L). The homogenized mixture was then centrifuged at 3,500 rpm for 15 min at 4°C to obtain supernatant. The supernatant was removed and stored in 2 mL centrifuge tubes at −80°C until further analysis.
Using the bicinchoninic acid method, 100 mg tumor tissues were lysed with a potent RIPA buffer containing a complete protease inhibitor cocktail (Siding Biotechnology, Shanghai, China). 100 mg of lysed tissues were electrophoresed on sodium dodecyl sulfate-polyacrylamide gel to separate proteins. Equal amounts of proteins (approximately 30 µg) from each sample were separated on sodium dodecyl sulfate-polyacrylamide gel and transferred to the PVDF membrane (Merk Millipore, United States). The PVDF membranes were incubated overnight at 4°C with primary antibodies including anti-JAK2 (1:1000, ab108596, Abcam, USA), anti-PIK3 (1:1000, 20584-1-AP, Proteintech, USA), and anti-AKT (1:10000, ab179463, Abcam, USA). Subsequently, they were incubated with the secondary antibody against horseradish peroxidase-conjugated rabbit IgG (1:20000, FDR007, Fude Biological Technology, Hangzhou, China) at room temperature for 1 h. Using enhanced chemiluminescence reagents (Siding Biotechnology, Shanghai, China), immunoreactive proteins were detected and analyzed with Image J.
All statistical analyses were conducted using SPSS 26.0, and GraphPad Prism 8.0 was utilized to generate the graphs. Two-way or one-way analysis of variance was used to determine p-values, followed by a post hoc analysis with the least significant difference in multiple comparison tests. The statistical significance threshold was set at p < 0.05.
Construction of the herb-compound-common target network
The TCMSP and ETCM databases and related literature searches yielded 174 potential active components of FXDH after eliminating redundancy. Details of these compounds are provided in Suppl. Table S1. Then, the Canonical SMILES format was imported into Swiss Target Prediction, and after removing duplicate genes, 676 prediction targets were obtained (Suppl. Table S2). Using the UniProt database for deduplication and correction, 4299 BC targets and 1649 depression targets were identified from the DisGeNET, GeneCards, OMIM, and DrugBank databases. Next, FXDH-related pharmacological targets, BC-related disease targets, and depression-related disease targets were mapped and intersected to yield 163 common targets (Suppl. Fig. S1 and Suppl. Table S2). The herb–compound-common target network was constructed using 362 nodes and 1,707 edges (Fig. 3). In descending order of “degrees,” the top six active compounds were identified as quercetin, methyl ferulate, luteolin, ferulaldehyde, wogonin, and diincarvilone A. The degrees represent the number of connected edges of nodes, and the greater the degree, the greater the regulatory function of nodes within the network.
Figure 3: The herb–compound-common target network. Circular nodes represent the common targets, square nodes represent the potential active compounds, and triangles represent the herbs from Fang-Xia-Dihuang decoction (FXDH). The gray lines denote the interaction between the nodes.
Protein-protein interaction network construction and hub targets acquisition
The 163 common targets were uploaded to the String11.5 database, and a PPI network comprising 156 nodes and 1269 edges was generated (Fig. 4A). The median node degree was 16. In total, 19 hub targets were screened out according to the rule in method (Suppl. Fig. S2): AKT1, STAT3, EGFR, TNF, IL-6, TP53, VEGFR, IL-1B, ALB, MAPK1, EGF, HRAS, EP300, GAPDH, FN1, PIK3R1, ESR1, PIK3CA, and STAT1. A PPI network of these 19 hub targets was additionally constructed (Fig. 4B).
Figure 4: Construction of protein-protein interaction (PPI) network and acquisition of hub targets. (A) PPI network of the potential, Fang-Xia-Dihuang decoction (FXDH) targets for breast cancer with comorbid depression. Each node represents a target protein; the redder the color, the more significant. (B) PPI network of the interactions between the hub targets.
Gene ontology analysis and kyoto encyclopedia of genes and genomes pathway enrichment analysis
GO and KEGG pathway enrichment analyses were performed to classify the potential functions of 163 initial common targets of disease–compounds. In total, 2651 GO bio-function entries are associated with the interaction between FXDH anti-BC and depression. Among them, 2391 BPs are primarily associated with protein kinase activity, metabolic process, and signal response. In addition, 77 CCs are primarily associated with lumen, membrane, cytoplasm, caveola, alpha granules, and neuronal cell body; 183 MFs are associated with signaling receptor activator activity, receptor–ligand activity, binding functions, cytokine activity, and oxidoreductase activity (Suppl. Table S3). Fig. 5A depicts the top 10 terms enriched in each category. The top 10 BPs were: regulation of protein serine/threonine kinase activity, response to extracellular stimulus, response to nutrient levels, reactive oxygen species metabolism, response to the drug, response to lipopolysaccharide, activation of protein kinase activity, response to molecules of bacterial origin, regulation of reactive oxygen species metabolism, and positive regulation of reactive oxygen species metabolism. The top 10 CCs were: membrane raft, membrane microdomain, neuronal cell body, external side of the plasma membrane, vesicle lumen, endoplasmic reticulum lumen, plasma membrane raft, caveola, platelet alpha granule, and dendrite cytoplasm. The top 10 MFs identified were: signaling receptor activator activity, receptor–ligand activity, receptor binding, cytokine activity, oxidoreductase activity acting on paired donors with incorporation or reduction of molecular oxygen, heme binding, tetrapyrrole binding, protease binding, growth factor receptor binding, and insulin receptor substrate binding.
Figure 5: Gene Ontology (GO) and Kyoto Encyclopedia of Genes and Genomes (KEGG) functional analyses. (A) The top ten entries of biological processes (BP), cellular components (CC), and molecular functions (MF) in GO analysis. (B) The top 30 significantly enriched pathways in KEGG enrichment analysis. The bubble size indicates the extent of gene enrichment in the pathway; the more genes, the larger the bubble. The bubble color denotes the p-value; the higher the p-value, the redder the color.
A total of 171 signaling pathways were identified via KEGG pathway enrichment analysis, and the top 30 pathways are shown according to their p-values in Fig. 5B and Suppl. Table S2. A literature search revealed that phosphoinositide-3-kinase–protein kinase B (PI3K/AKT), HIF-1, FoxO, JAK/STAT, chemical carcinogenesis receptor activation, endocrine resistance, and PD1/PDL1-related pathways may play an important role in BCCD. In addition, the target gene–signaling pathway network (Fig. 6) validated that the intervention effect of FXDH on BCCD might be mediated by the correlation between multiple targets and pathways. In particular, the PI3K-AKT signaling pathway had 36 intersection targets and may play a major role in depression-related breast cancer progression.
Figure 6: Target gene–signaling pathway network of Fang-Xia-Dihuang decoction (FXDH) for breast cancer with comorbid depression. Inverted triangular nodes represent the pathways; the circles represent the genes. The larger size of a node, the greater the number of genes enriched in the pathway or the more pathways the gene is involved in.
Major components of Fang-Xia-Dihuang decoction as assessed by ultra-high-performance liquid chromatography–high-resolution mass spectrometry analysis
The compounds selected by network pharmacology were validated using qualitative UPLC-HRMS of the FXDH extract. Figs. 7A and 7B illustrate the positive- and negative-ion base peak chromatograms of FXDH. The abundant peaks observed in the base peak chromatograms were analyzed based on their peak shape and were confirmed by secondary spectra. As a result, 32 active components of FXDH were identified, the majority of which included aporphines, benzopyran, morphinans, proberberine alkaloids, flavonoids, phenols, cinnamic acids, glycerophospholipids, saccharolipids, carboxylic acids, and fatty acyls (Suppl. Table S4). Based on the network pharmacology results, quercetin, methyl ferulate, luteolin, ferulaldehyde, wogonin, and diincarvilone A were considered for molecular docking. The chemical structures of these six core components are depicted in Fig. 7C.
Figure 7: The major components of Fang-Xia-Dihuang decoction (FXDH) were identified by ultra-high-performance liquid chromatography–high-resolution mass spectrometry analysis. (A) Base peak chromatograms in positive-ion mode. (B) Base peak chromatograms in negative-ion mode. Overall, 32 compounds were identified, which have been detailed in Suppl. Table S4. (C) The chemical structures of the top six active components in FXDH.
To understand the interactions between compounds and target genes, the six major components of FXDH identified by UPLC-HRMS were used as ligands for molecular docking. According to the literature and degree value, six core target proteins (IL-6, AKT1, EGFR, ESR1, PIK3, and STAT3) were identified from the PPI network as receptors for molecular docking. Fig. 8A displays the total score for every docking activity. In total, 22 of the 36 results exhibited exceptional docking activity (Totalscore ≥ 5.0), with 5 exhibiting strong matching activity (Totalscore ≥ 7.0). As shown in Fig. 8A, luteolin had the strongest binding affinity for the core targets, whereas AKT and STAT3 had the highest average binding levels with the six components. Fig. 8B depicts the 3D structures of the seven receptor–ligand interactions with the highest scores.
Figure 8: Molecular docking results. (A) Heat map for the total scores of the docked key components within the hub targets. A redder color indicates a stronger combination between the receptor and the ligand. (B) The 3D structure of the top seven ligand–protein complexes. A rod-like structure represents a ligand, whereas a floating band refers to a protein skeleton, with different peptide chains indicating different colors.
Fang-Xia-Dihuang decoction ameliorated depression‑like behavior in mice with breast cancer with comorbid depression
The plasma levels of corticosterone, a typical glucocorticoid in rodents, are significantly elevated following exposure to stress (Wu et al., 2022). In addition, multiple corticosterone injections have been shown to induce depression-like behaviors in mice (Ding et al., 2018). Accordingly, we exposed treated orthotopic 4T1 tumor-bearing mice to corticosterone for 28 consecutive days to establish a mice model of breast cancer complicated with comorbid depression. Compared with the blank group, 4T1, CORT, and 4T1+CORT groups had lower weight, total and central area distance, and sugar preference. As depicted in Fig. 9, the 4T1+CORT group had lower weight, movement ability, exploration behavior, and surface water preference, indicating a higher level of depression, which was mitigated by FXDH intervention. It is well-known that sympathetic nerve fibers innervate various peripheral tissues, particularly the spleen. Therefore, several studies have assessed the levels of catecholamine to evaluate the sympatho-adrenal-medullary activity in stressed mice (Cao et al., 2002; Thaker et al., 2006). Accordingly, the present study found that the CORT group had a higher splenic EPI level than the other groups. Moreover, FXDH could effectively alleviate this effect.
Figure 9: Fang-Xia-Dihuang decoction (FXDH) ameliorated depression-like behavior in mice with breast cancer and depression. (A) The curve of weight change in mice. (B) The percentage of sucrose water preference on days 0 and 28. (C) The splenic epinephrine (EPI) level in mice was detected by ELISA. (D–G) Results of the open field test: (D) total moving distance; € frequency of central area entry; (F) central area movement distance; and (G) representative movement trajectory of the mice. The data are presented as means ± standard deviation (SD) (n = 5), *p < 0.05, **p < 0.01, ***p < 0.001, ns, not significant.
Fang-Xia-Dihuang decoction was inhibited BC progression and accelerated by corticosterone through the downregulation of the hub target expression
Sustained corticosterone stress may rapidly promote tumor progression, as evidenced by a significant increase in tumor volume and weight. This effect was mitigated by both FXDH and CAP (Figs. 10A–10C). H&E staining demonstrated that treatment with FXDH or CAP inhibited the proliferative effects of corticosterone, including tight arrangements of tumor cells, a high nuclear/cytoplasmic ratio, and increased karyokinesis as well as the development of large patchy necrosis in tumor tissue (Fig. 10D). Based on the KEGG pathway enrichment analysis and published literature, the regulatory effect of FXDH on BCCD in mice might be related to the PIK3CA-AKT and STAT-JAK signaling pathways. RT-qPCR revealed that in tumor tissues, the expression of PIK3, AKT, STAT3, and JAK2 mRNAs was upregulated after chronic stress. Moreover, FXDH treatment could downregulate the expression of these genes (Fig. 10E). In addition, the protein-level expression of PIK3, AKT, and JAK2 exhibited the same pattern, confirming the crucial role of hub targets in treating BCCD by FXDH (Fig. 10F).
Figure 10: Fang-Xia-Dihuang decoction (FXDH) inhibited breast cancer progression accelerated by corticosterone and regulated the hub target expressions. (A) The tumor growth curve was monitored every 3 days from day 7 to day 28. (B) Tumor weight and (C) photographs of excised 4T1 solid tumor recorded after the mice were sacrificed on day 29. (D) HE staining of tumor tissue sections. (E) mRNA expressions of PIK3, AKT, STAT3, and JAK2, as detected by RT-qPCR and using GAPDH as the internal control gene. (F) Western blotting of the protein levels of PIK3, AKT, and JAK2, with β-actin as the internal control. The data are presented as means ± standard deviation (SD) (A–E, n = 5; F, n = 3), *p < 0.05, **p < 0.01, ***p < 0.001, ns, not significant.
Recently, disease models have transformed from the traditional biomedical models to the biopsychosocial model; as a result, the role of psychological factors in the etiology and prognosis of tumors has received increasing attention. Depression affects the quality of life of breast cancer survivors (Avis et al., 2020) as well as affects their treatment adherence, adverse reactions, drug resistance, and survival rate (Mausbach et al., 2015; Antoni et al., 2017; Tian et al., 2021; Lv et al., 2022). Consequently, treating the psychological symptoms of patients with breast cancer along with administering conventional anti-tumor therapy is a crucial component of providing more effective, comprehensive anti-tumor treatment (Greenlee et al., 2017). Acupuncture, Tai Chi, and decoctions of natural compounds have been used to effectively and extensively alleviate chronic psychological stress in patients with cancer (Irwin et al., 2017; Li et al., 2019; Gosain et al., 2020). Specifically, clinical studies have found that FXDH is effective in patients with BCCD. In line with this, the present study observed the beneficial effect of FXDH in a mouse model of BCCD and revealed its constituents and the potential mechanism underlying FXDH activity.
According to network pharmacology and UPLC-HRMS results, quercetin, methyl ferulate, luteolin, ferulaldehyde, wogonin, and diincarvilone A, may be the primary active ingredients of FXDH that significantly contribute to the treatment of BCCD. Quercetin is a flavonoid found in numerous plants, such as P. vulgaris, G. jasminoides, and licorice, as the ingredients for FXDH. Moreover, quercetin can cross the blood–brain barrier and thus demonstrate a good neuroprotective effect via anti-inflammatory and anti-oxidative mechanisms. In addition, it can inhibit pyroptosis, stimulate the immune system, and alleviate depression caused by breast cancer (Luo et al., 2021; Zhu et al., 2022c). Furthermore, as direct anti-tumor effects, quercetin can induce apoptosis in breast cancer cells as well as reverse chemotherapy resistance (Prieto-Vila et al., 2020; Mohammed et al., 2021; Zhu et al., 2022c). Luteolin, present in P. vulgaris of FXDH, is a flavonoid with various biological properties, including anti-oxidative, anti-cancer, anti-inflammatory, and neuroprotective effects. It may additionally protect against BCCD by regulating the miR-124-3p/TNF-α/TRAF6 pathway (Dirscherl et al., 2010; Wu et al., 2021; Zhu et al., 2022b). Wogonin, a flavonoid, is present in Saposhnikovia divaricata component of FXDH. Studies demonstrated that wogonin has a practical anti-breast cancer effect and may inhibit monoamine oxidase A, a potential active compound for the treatment of depression (Lee et al., 2017; Yang et al., 2020). In FXDH, Rehmannia radix is the source of methyl ferulate, ferulaldehyde, and diincarvilone A. These compounds are non-toxic and non-irritating; may additionally regulate the crucial PI3K/AKT signaling pathway; have anti-inflammatory and anti-oxidative effects (Phuong et al., 2014; Lee et al., 2017; Cheng et al., 2022; Zhang et al., 2022b). Currently, available literature primarily focuses on the antidepressant and anti-tumor effects of a single compound, and the combined effects of the aforementioned compounds have not been reported. Therefore, our future research will focus on combining two or more of the six aforementioned active compounds to complement each other’s benefits and contribute to treating BCCD.
In the present study network pharmacology was used and identified IL-6, AKT1, EGFR, ESR1, PIK3, and STAT3 as the core targets of FXDH for treating BCCD. The results of GO analysis suggested that these targets were mainly involved in biological processes such as metabolism with protein kinase activity, metabolic process, and signal response, and their molecular functions may be mainly related to signaling receptor activator activity, cytokine activity, and oxidoreductase activity. EGFR, a transmembrane tyrosine kinase receptor, is an upstream target of the PI3K/Akt/mTOR pathway and is involved in regulating cell proliferation and survival (Wee and Wang, 2017; He et al., 2018). Notably, KEGG pathway analysis and molecular docking identified the PI3K/AKT signaling pathway as one of the two primary signaling pathways underlying the effect of FXDH. Reportedly, the PI3K-AKT signaling pathway is widely upregulated in breast cancer (Gray, 2016; Pascual and Turner, 2019). Furthermore, mutations in genes from this pathway, including PIK3CA, PIK3R1, PTEN, and AKT, were prevalent in breast cancer patients. Specifically, PIK3CA was mutated in approximately 36% of breast cancer and up to 44% of Chinese individuals (Liu et al., 2011; Gray, 2016; Chen et al., 2018). Our network pharmacological results also demonstrate the importance of PIK3CA in BCCD. Moreover, studies have confirmed that the PI3K-AKT signaling pathway regulates the development of breast cancer (Miricescu et al., 2020). As a result, its abnormal overexpression often promotes excessive cell proliferation and anti-apoptosis and is related to chemotherapy, targeted therapy, and endocrine drug resistance (Zhu et al., 2022a). Chronic stress regulates the neuroendocrine system to activate the PI3K/AKT pathway, promote β-catenin expression and nuclear localization, and increase the activity of the SLUG promoter. This subsequently promotes epithelial–mesenchymal transformation and the invasion of cancer cells (Bu et al., 2020). Thus, PI3K inhibitors such as alpelisib, taselisib, and inavolisib, as well as AKT inhibitors such as capivasertib and ipatasertib are increasingly being utilized in clinical settings for breast cancer treatment. Quercetin, one of the active components of FXDH, inhibits the EGFR/PI3K/Akt pathway to induce apoptosis in breast cancer cell lines (MCF-7 and MDA-MB-231) (Balakrishnan et al., 2017). The JAK/STAT3 signaling pathway is another core pathway predicted by network pharmacology. The present study identified IL-6 as a core target and is additionally a primary upstream regulator of the JAK/STAT3 pathway. IL-6 is positively associated with depressive symptoms and tumor progression in patients with metastatic breast cancer (Jehn et al., 2012; Chen et al., 2023). Moreover, IL-6 binds to IL-6 receptors and glycoprotein 130 (gp130) receptors to form signal transduction hexamer receptor complexes that recruit and activate JAKs (Manore et al., 2022). Non-receptor tyrosine kinase JAK2 is a signaling hub that receives extracellular signals related to processes such as cytokines and growth factors and activation of STAT3 (Harrison et al., 1995; Loh et al., 2019). This activation of the oncogenic transcription factor STAT3 is responsible for multiple biological processes, such as tumor proliferation, growth, anti-apoptosis, and angiogenesis, which affect breast cancer recurrence and metastasis (Lo et al., 2007; Wang et al., 2017; Jiang et al., 2021). Moreover, STAT3 has been identified as a potential target for triple-negative breast cancer due to its overexpression in this cancer type (Balko et al., 2016; Qin et al., 2019). Reportedly, the activation of the JAK2/STAT3 pathway drives cancer cell proliferation and aggressiveness while inhibiting apoptosis. STAT3, in turn, further enhances IL-6 signaling, promotes a malignant inflammatory cycle, activates surrounding microenvironmental cells (e.g., myeloid suppressor cells), and induces immunosuppression. All these changes contribute to increased growth and metastasis of breast cancer (Wang et al., 2018a; An et al., 2021; Manore et al., 2022). ESR1, located on chromosome 6q25, is an important mediator of hormone response in estrogen-sensitive tissues (Carausu et al., 2019; Dustin et al., 2019). The somatic base missense mutations of ESR1 have been identified in approximately 1/3 of endocrine-resistant metastatic breast cancer tissues (Li et al., 2022b). It is worth noting that ESR1 is also a key factor in depression, especially in women (Zhang et al., 2022a). Genetic variations in the estrogen receptor may alter estrogen signals, thus affecting women’s susceptibility to depression (Ryan and Ancelin, 2012). However, at present, research on the role of ESR1 in depression and breast cancer progression is insufficient to conduct a correlation analysis. Moreover, several studies are required to better explain the crosstalk between depression and breast cancer progression.
In the present study, molecular docking helped identify ligand–protein complexes with good docking activity, and most of these complexes (73.3%) comprised PIK3CA, AKT, and STAT3 as receptors. To further verify network pharmacology results, in vivo experiments were conducted. We observed that corticosterone-induced stress could increase tumor growth rate and volume, in line with previous findings (Wu et al., 2022). In addition, these rapidly growing tumors had high PI3K, AKT, JAK2, and STAT3 expression. However, FXDH inhibited the overactivation of the PI3K/AKT and JAK2/STAT3 pathways. This finding supports the growing evidence indicating that TCM molecules can inhibit tumor growth by downregulating the PI3K/AKT and JAK2/STAT pathways (Zhang et al., 2019; Xu et al., 2020; Qiu et al., 2022). Remarkably, FXDH had a regulatory effect on depression-like behavior in mice. It could enhance the sucrose preference and motor ability of depressed mice in an open field and improve their central exploration behavior. To understand the antidepressant properties of FXDH, we must first consider catecholamine hormones. Reportedly, the stress hormone adrenaline is associated with mental illness (Peacock et al., 2017) and with tumor development after being delivered to the tumor microenvironment via circulating blood (Qin et al., 2015). When chronic stress induces negative emotions, the sympathetic nervous system continuously activates and releases catecholamines such as epinephrine and norepinephrine. These hormones bind to adrenergic receptors (ARs), especially β2-Ars, on the surface of tumor cells through circulating blood (Krizanova et al., 2016). The activation of β2-ARs may induce lactate dehydrogenase A to produce lactic acid, alter the pH level in the body, and stabilize MYC protein and ubiquitin. In addition, β2-ARs may activate the PI3K/AKT signal pathway, increase the activity of the SLUG promoter, promote the activity of breast cancer stem cells, and increase the probability of tumor progression (Cui et al., 2019; Yan et al., 2023). In addition, epinephrine is considered an anti-apoptotic stimulant of human breast cancer cells in vitro, which inactivates the pro-apoptotic protein BAD in a PKA-dependent manner by phosphorylation (Conceicao et al., 2021). In the present study, we observed an increase in epinephrine levels and a counteracting effect of FXDH in tumor-bearing depressed mice. Thus, FXDH may play a role in attenuating breast cancer progression by down-regulating abnormally elevated epinephrine levels in depressed states. However, the precise mechanism by which FXDH regulates the epinephrine level in mice remains unknown, as does its relationship to the PI3K/AKT or JAK2/STAT signaling pathways. Thus, to determine the molecular mechanism underlying FXDH in BCCD, we intend to analyze the expression of related proteins in both tumor and hippocampal tissues from mice in further studies.
A few limitations of the study need to be acknowledged. An in vitro experiment could not be performed to further verify the network pharmacology results, owing to the limitations of our laboratory condition. This can be an aim of future study. In addition, molecular docking experiments revealed four core targets; however, we could only validate the three targets, AKT, STAT3, and PIK3CA, but not ESR1. Reportedly, ESR1 may be closely involved in drug resistance to endocrine therapy for breast cancer. However, we used the 4T1 breast cancer cells, a type of hormone receptor-negative breast cancer cells. Thus, it is best to use MCF-7 and T47D cell lines with positive expression of hormone receptors in the future to observe the changes of the ESR1 target in BCCD and evaluate the efficacy of FXDH on the ESR1.
In summary, network pharmacology, molecular docking, and in vivo experiments revealed that FXDH might inhibit tumor growth caused by corticosterone-induced stress by regulating the PI3K/AKT and JAK2/STAT signaling pathways. Moreover, FXDH may reduce the level of adrenergic hormone secretion in response to stress to improve depression-like behavior in mice. Despite some limitations, these findings may contribute to the treatment of patients with BCCD. However, future clinical and experimental research is required to determine the precise therapeutic effect and mechanism of FXDH in breast cancer.
Acknowledgement: Not applicable.
Funding Statement: This study was supported by the Xiamen High-Level Health Talents Introduction and Training Project (Xiaweidang 2021-124) and the National Natural Science Foundation of China (No. 81774319).
Author Contributions: XP and HF contributed to the conception and design of the study. LL and JZ performed most of the experimental work and statistical analysis, and wrote the original draft. LX and XW provided various experimental assistance, such as specimen collection and statistical analysis. YF and CW critically reviewed the manuscript. All authors have read and approved the manuscript.
Availability of Data and Materials: All study findings are provided in the article and its supplementary materials; further inquiries can be directed to the corresponding author.
Ethics Approval: The animal experiment was reviewed and approved by the Ethics Committee of Laboratory Animals of Xiamen University (XMULAC202000550).
Conflicts of Interest: The authors declare that they have no conflicts of interest to report regarding the present study.
Supplementary Materials: The supplementary materials are available online at https://doi.org/10.32604/biocell.2023.030742.
References
Amberger JS, Hamosh A (2017). Searching online mendelian inheritance in man (OMIMA knowledgebase of human genes and genetic phenotypes. Current Protocols in Bioinformatics 58: 1.2.1–1.2.12. https://doi.org/10.1002/cpbi.27 [Google Scholar] [CrossRef]
An J, Feng L, Ren J, Li Y, Li G et al. (2021). Chronic stress promotes breast carcinoma metastasis by accumulating myeloid-derived suppressor cells through activating β-adrenergic signaling. Oncoimmunology 10: 2004659. https://doi.org/10.1080/2162402X.2021.2004659 [Google Scholar] [CrossRef]
Antoni MH, Jacobs JM, Bouchard LC, Lechner SC, Jutagir DR, Gudenkauf LM, Blomberg BB, Gluck S, Carver CS (2017). Post-surgical depressive symptoms and long-term survival in non-metastatic breast cancer patients at 11-year follow-up. General Hospital Psychiatry 44: 16–21. https://doi.org/10.1016/j.genhosppsych.2016.10.002 [Google Scholar] [CrossRef]
Avis NE, Levine B, Goyal N, Crawford SL, Hess R, Colvin A, Bromberger JT, Greendale GA (2020). Health-related quality of life among breast cancer survivors and noncancer controls over 10 years: Pink SWAN. Cancer 126: 2296–2304. https://doi.org/10.1002/cncr.32757 [Google Scholar] [CrossRef]
Balakrishnan S, Mukherjee S, Das S, Bhat FA, Raja Singh P, Patra CR, Arunakaran J (2017). Gold nanoparticles-conjugated quercetin induces apoptosis via inhibition of EGFR/PI3K/Akt-mediated pathway in breast cancer cell lines (MCF-7 and MDA-MB-231). Cell Biochemistry and Function 35: 217–231. https://doi.org/10.1002/cbf.3266 [Google Scholar] [CrossRef]
Balko JM, Schwarz LJ, Luo N, Estrada MV, Giltnane JM et al. (2016). Triple-negative breast cancers with amplification of JAK2 at the 9p24 locus demonstrate JAK2-specific dependence. Science Translational Medicine 8: 334–353. https://doi.org/10.1126/scitranslmed.aad3001 [Google Scholar] [CrossRef]
Batty GD, Russ TC, Stamatakis E, Kivimaki M (2017). Psychological distress in relation to site specific cancer mortality: Pooling of unpublished data from 16 prospective cohort studies. British Medical Journal 356: j108. https://doi.org/10.1136/bmj.j108 [Google Scholar] [CrossRef]
Bu S, Wang Q, Sun J, Li X, Gu T, Lai D (2020). Melatonin suppresses chronic restraint stress-mediated metastasis of epithelial ovarian cancer via NE/AKT/β-catenin/SLUG axis. Cell Death and Disease 11: 644. https://doi.org/10.1038/s41419-020-02906-y [Google Scholar] [CrossRef]
Cao L, Filipov NM, Lawrence DA (2002). Sympathetic nervous system plays a major role in acute cold/restraint stress inhibition of host resistance to Listeria monocytogenes. Journal of Neuroimmunology 125: 94–102. https://doi.org/10.1016/S0165-5728(02)00039-5 [Google Scholar] [CrossRef]
Cao S, Xue J, Chen L, Hao Y, Lu M, Feng M, Wang H, Zhou J, Yao C (2022). Effects of the Chinese herbal medicine Hong Huang decoction, on myocardial injury in breast cancer patients who underwent anthracycline-based chemotherapy. Frontiers in Cardiovascular Medicine 9: 921753. https://doi.org/10.3389/fcvm.2022.921753 [Google Scholar] [CrossRef]
Carausu M, Bidard FC, Callens C, Melaabi S, Jeannot E, Pierga JY, Cabel L (2019). ESR1 mutations: A new biomarker in breast cancer. Expert Review of Molecular Diagnostics 19: 599–611. https://doi.org/10.1080/14737159.2019.1631799 [Google Scholar] [CrossRef]
Chen J, Li J, Qiao H, Hu R, Li C (2023). Disruption of IDO signaling pathway alleviates chronic unpredictable mild stress-induced depression-like behaviors and tumor progression in mice with breast cancer. Cytokine 162: 156115. https://doi.org/10.1016/j.cyto.2022.156115 [Google Scholar] [CrossRef]
Chen L, Yang L, Yao L, Kuang XY, Zuo WJ et al. (2018). Characterization of PIK3CA and PIK3R1 somatic mutations in Chinese breast cancer patients. Nature Communications 9: 1357. https://doi.org/10.1038/s41467-018-03867-9 [Google Scholar] [CrossRef]
Cheng X, Han ZX, Su ZJ, Zhang FL, Li BP, Jiang ZR, Tang L, Yang JS (2022). Network pharmacology-based exploration on the intervention of Qinghao Biejia decoction on the inflammation-carcinoma transformation process of chronic liver disease via MAPK and PI3k/AKT pathway. BioMed Research International 2022: 9202128. https://doi.org/10.1155/2022/9202128 [Google Scholar] [CrossRef]
Conceicao F, Sousa DM, Paredes J, Lamghari M (2021). Sympathetic activity in breast cancer and metastasis: Partners in crime. Bone Research 9: 9. https://doi.org/10.1038/s41413-021-00137-1 [Google Scholar] [CrossRef]
Cormanique TF, Almeida LE, Rech CA, Rech D, Herrera AC, Panis C (2015). Chronic psychological stress and its impact on the development of aggressive breast cancer. Einstein (Sao Paulo) 13: 352–356. https://doi.org/10.1590/S1679-45082015AO3344 [Google Scholar] [CrossRef]
Cui B, Luo Y, Tian P, Peng F, Lu J et al. (2019). Stress-induced epinephrine enhances lactate dehydrogenase A and promotes breast cancer stem-like cells. Journal of Clinical Investigation 129: 1030–1046. https://doi.org/10.1172/JCI121685 [Google Scholar] [CrossRef]
Daina A, Michielin O, Zoete V (2017). SwissADME: A free web tool to evaluate pharmacokinetics, drug-likeness and medicinal chemistry friendliness of small molecules. Scientific Reports 7: 42717. https://doi.org/10.1038/srep42717 [Google Scholar] [CrossRef]
Daina A, Michielin O, Zoete V (2019). SwissTargetPrediction: Updated data and new features for efficient prediction of protein targets of small molecules. Nucleic Acids Research 47: W357–W364. https://doi.org/10.1093/nar/gkz382 [Google Scholar] [CrossRef]
Dashti H, Wedell JR, Westler WM, Markley JL, Eghbalnia HR (2019). Automated evaluation of consistency within the PubChem compound database. Scientific Data 6: 190023. https://doi.org/10.1038/sdata.2019.23 [Google Scholar] [CrossRef]
Ding H, Cui XY, Cui SY, Ye H, Hu X, Zhao HL, Liu YT, Zhang YH (2018). Depression-like behaviors induced by chronic corticosterone exposure via drinking water: Time-course analysis. Neuroscience Letters 687: 202–206. https://doi.org/10.1016/j.neulet.2018.09.059 [Google Scholar] [CrossRef]
Dirscherl K, Karlstetter M, Ebert S, Kraus D, Hlawatsch J, Walczak Y, Moehle C, Fuchshofer R, Langmann T (2010). Luteolin triggers global changes in the microglial transcriptome leading to a unique anti-inflammatory and neuroprotective phenotype. Journal of Neuroinflammation 7: 3. https://doi.org/10.1186/1742-2094-7-3 [Google Scholar] [CrossRef]
Dustin D, Gu G, Fuqua SAW (2019). ESR1 mutations in breast cancer. Cancer 125: 3714–3728. https://doi.org/10.1002/cncr.32345 [Google Scholar] [CrossRef]
Fang S, Dong L, Liu L, Guo J, Zhao L et al. (2021). HERB: A high-throughput experiment- and reference-guided database of traditional Chinese medicine. Nucleic Acids Research 49: D1197–D1206. https://doi.org/10.1093/nar/gkaa1063 [Google Scholar] [CrossRef]
Goodsell DS, Zardecki C, Di Costanzo L, Duarte JM, Hudson BP et al. (2020). RCSB protein data bank: Enabling biomedical research and drug discovery. Protein Science 29: 52–65. https://doi.org/10.1002/pro.3730 [Google Scholar] [CrossRef]
Gosain R, Gage-Bouchard E, Ambrosone C, Repasky E, Gandhi S (2020). Stress reduction strategies in breast cancer: Review of pharmacologic and non-pharmacologic based strategies. Seminars in Immunopathology 42: 719–734. https://doi.org/10.1007/s00281-020-00815-y [Google Scholar] [CrossRef]
Gray JW (2016). PI3 kinase pathway mutations in human cancers. JAMA Oncology 2: 1543–1544. https://doi.org/10.1001/jamaoncol.2016.0875 [Google Scholar] [CrossRef]
Greenlee H, DuPont-Reyes MJ, Balneaves LG, Carlson LE, Cohen MR et al. (2017). Clinical practice guidelines on the evidence-based use of integrative therapies during and after breast cancer treatment. CA: A Cancer Journal for Clinicians 67: 194–232. https://doi.org/10.3322/caac.21397 [Google Scholar] [CrossRef]
Guo Q, Pei XH, Chu AJ, Guo YB, Fan YY, Wang CH, Zhang SJ, Sun SQ, Liu YF, Wang X (2022). The mechanism of action of Fangji Huangqi Decoction on epithelial-mesenchymal transition in breast cancer using high-throughput next-generation sequencing and network pharmacology. Journal of Ethnopharmacology 284: 114793. https://doi.org/10.1016/j.jep.2021.114793 [Google Scholar] [CrossRef]
Harrison DA, Binari R, Nahreini TS, Gilman M, Perrimon N (1995). Activation of a drosophila janus kinase (JAK) causes hematopoietic neoplasia and developmental defects. The EMBO Journal 14: 2857–2865. https://doi.org/10.1002/j.1460-2075.1995.tb07285.x [Google Scholar] [CrossRef]
He L, Liu X, Yang J, Li W, Liu S et al. (2018). Imbalance of the reciprocally inhibitory loop between the ubiquitin-specific protease USP43 and EGFR/PI3K/AKT drives breast carcinogenesis. Cell Research 28: 934–951. https://doi.org/10.1038/s41422-018-0079-6 [Google Scholar] [CrossRef]
Huang H, Zhang G, Zhou Y, Lin C, Chen S, Lin Y, Mai S, Huang Z (2018). Reverse screening methods to search for the protein targets of chemopreventive compounds. Frontiers in Chemistry 6: 138. https://doi.org/10.3389/fchem.2018.00138 [Google Scholar] [CrossRef]
Hung YL, Leung SS, Chiu SP, Li PY, Kan AC et al. (2023). Perceptions about traditional Chinese medicine use among Chinese breast cancer survivors: A qualitative study. Cancer Medicine 12: 1997–2007. https://doi.org/10.1002/cam4.5046 [Google Scholar] [CrossRef]
Irwin MR, Olmstead R, Carrillo C, Sadeghi N, Nicassio P, Ganz PA, Bower JE (2017). Tai Chi Chih compared with cognitive behavioral therapy for the treatment of insomnia in survivors of breast cancer: A randomized, partially blinded, noninferiority trial. Journal of Clinical Oncology 35: 2656–2665. https://doi.org/10.1200/JCO.2016.71.0285 [Google Scholar] [CrossRef]
Jain AN (2007). Surflex-Dock 2.1: Robust performance from ligand energetic modeling, ring flexibility, and knowledge-based search. Journal of Computer-Aided Molecular Design 21: 281–306. https://doi.org/10.1007/s10822-007-9114-2 [Google Scholar] [CrossRef]
Jaradat NA, Shawahna R, Eid AM, Al-Ramahi R, Asma MK, Zaid AN (2016). Herbal remedies use by breast cancer patients in the West Bank of Palestine. Journal of Ethnopharmacology 178: 1–8. https://doi.org/10.1016/j.jep.2015.11.050 [Google Scholar] [CrossRef]
Jehn CF, Flath B, Strux A, Krebs M, Possinger K, Pezzutto A, Luftner D (2012). Influence of age, performance status, cancer activity, and IL-6 on anxiety and depression in patients with metastatic breast cancer. Breast Cancer Research and Treatment 136: 789–794. https://doi.org/10.1007/s10549-012-2311-2 [Google Scholar] [CrossRef]
Jiang D, Xu J, Liu S, Nasser MI, Wei W, Mao T, Liu X, Zou X, Li J, Li X (2021). Rosmanol induces breast cancer cells apoptosis by regulating PI3K/AKT and STAT3/JAK2 signaling pathways. Oncology Letters 22: 631. https://doi.org/10.3892/ol.2021.12892 [Google Scholar] [CrossRef]
Jiao X, Jin X, Ma Y, Yang Y, Li J, Liang L, Liu R, Li Z (2021b). A comprehensive application: Molecular docking and network pharmacology for the prediction of bioactive constituents and elucidation of mechanisms of action in component-based Chinese medicine. Computational Biology and Chemistry 90: 107402. https://doi.org/10.1016/j.compbiolchem.2020.107402 [Google Scholar] [CrossRef]
Jiao H, Yang H, Yan Z, Chen J, Xu M et al. (2021a). Traditional Chinese formula xiaoyaosan alleviates depressive-like behavior in CUMS Mice by regulating PEBP1-GPX4-mediated ferroptosis in the hippocampus. Neuropsychiatric Disease and Treatment 17: 1001–1019. https://doi.org/10.2147/NDT.S302443 [Google Scholar] [CrossRef]
Krizanova O, Babula P, Pacak K (2016). Stress, catecholaminergic system and cancer. Stress 19: 419–428. https://doi.org/10.1080/10253890.2016.1203415 [Google Scholar] [CrossRef]
Kruk J, Aboul-Enein BH, Bernstein J, Gronostaj M (2019). Psychological stress and cellular aging in cancer: A meta-analysis. Oxidative Medicine and Cellular Longevity 2019: 1270397. https://doi.org/10.1155/2019/1270397 [Google Scholar] [CrossRef]
Lee HW, Ryu HW, Kang MG, Park D, Lee H, Shin HM, Oh SR, Kim H (2017). Potent inhibition of monoamine oxidase A by decursin from Angelica gigas Nakai and by wogonin from Scutellaria baicalensis Georgi. International Journal of Biological Macromolecules 97: 598–605. https://doi.org/10.1016/j.ijbiomac.2017.01.080 [Google Scholar] [CrossRef]
Li M, Chen Z, Liu Z, Zhang N, Liu J et al. (2019). Twelve Chinese herbal preparations for the treatment of depression or depressive symptoms in cancer patients: A systematic review and meta-analysis of randomized controlled trials. BMC Complementary and Alternative Medicine 19: 28. https://doi.org/10.1186/s12906-019-2441-8 [Google Scholar] [CrossRef]
Li YM, Ma CC, Zhan QX (2022a). Randomized controlled trial study of modified Fangji Dihuang decoction in treating insomnia after endocrine therapy for breast cancer. World Clinical Drugs 43: 436–441. https://doi.org/10.13683/j.wph.2022.04.013 [Google Scholar] [CrossRef]
Li Z, Wu Y, Yates ME, Tasdemir N, Bahreini A et al. (2022b). Hotspot ESR1 mutations are multimodal and contextual modulators of breast cancer metastasis. Cancer Research 82: 1321–1339. https://doi.org/10.1158/0008-5472.CAN-21-2576 [Google Scholar] [CrossRef]
Li S, Zhang B (2013). Traditional Chinese medicine network pharmacology: Theory, methodology and application. Chinese Journal of Natural Medicine 11: 110–120. https://doi.org/10.1016/S1875-5364(13)60037-0 [Google Scholar] [CrossRef]
Liu P, Cheng H, Santiago S, Raeder M, Zhang F et al. (2011). Oncogenic PIK3CA-driven mammary tumors frequently recur via PI3K pathway-dependent and PI3K pathway-independent mechanisms. Nature Medicine 17: 1116–1120. https://doi.org/10.1038/nm.2402 [Google Scholar] [CrossRef]
Liu HM, Ma LL, Li C, Cao B, Jiang Y, Han L, Xu R, Lin J, Zhang D (2022). The molecular mechanism of chronic stress affecting the occurrence and development of breast cancer and potential drug therapy. Translational Oncology 15: 101281. https://doi.org/10.1016/j.tranon.2021.101281 [Google Scholar] [CrossRef]
Lo HW, Hsu SC, Xia W, Cao X, Shih JY, Wei Y, Abbruzzese JL, Hortobagyi GN, Hung MC (2007). Epidermal growth factor receptor cooperates with signal transducer and activator of transcription 3 to induce epithelial-mesenchymal transition in cancer cells via up-regulation of TWIST gene expression. Cancer Research 67: 9066–9076. https://doi.org/10.1158/0008-5472.CAN-07-0575 [Google Scholar] [CrossRef]
Loh CY, Arya A, Naema AF, Wong WF, Sethi G, Looi CY (2019). Signal transducer and activator of transcription (STATs) proteins in cancer and inflammation: Functions and therapeutic implication. Frontiers in Oncology 9: 48. https://doi.org/10.3389/fonc.2019.00048 [Google Scholar] [CrossRef]
Luo T, Zhang Y, Liu X, Liang Q, Zhu L et al. (2021). The central nervous system can directly regulate breast cancer progression and blockage by quercetin. Annals of Translational Medicine 9: 999. https://doi.org/10.21037/atm-21-2558 [Google Scholar] [CrossRef]
Lv D, Lan B, Zhang L, Sun X, Yang M, Ma F (2022). Association between depression and anxiety status of breast cancer patients before adjuvant chemotherapy and chemotherapy‐induced adverse events. Cancer Medicine 12: 4794–4800. https://doi.org/10.1002/cam4.5283 [Google Scholar] [CrossRef]
Manore SG, Doheny DL, Wong GL, Lo HW (2022). IL-6/JAK/STAT3 signaling in breast cancer metastasis: Biology and treatment. Frontiers in Oncology 12: 866014. https://doi.org/10.3389/fonc.2022.866014 [Google Scholar] [CrossRef]
Mausbach BT, Schwab RB, Irwin SA (2015). Depression as a predictor of adherence to adjuvant endocrine therapy (AET) in women with breast cancer: A systematic review and meta-analysis. Breast Cancer Research and Treatment 152: 239–246. https://doi.org/10.1007/s10549-015-3471-7 [Google Scholar] [CrossRef]
Miricescu D, Totan A, Stanescu SII, Badoiu SC, Stefani C, Greabu M (2020). PI3K/AKT/mTOR signaling pathway in breast cancer: From molecular landscape to clinical aspects. International Journal of Molecular Sciences 22: 173. https://doi.org/10.3390/ijms22010173 [Google Scholar] [CrossRef]
Mohammed HA, Sulaiman GM, Anwar SS, Tawfeeq AT, Khan RA, Mohammed SAA, Al-Omar MS, Alsharidah M, Rugaie OA, Al-Amiery AA (2021). Quercetin against MCF7 and CAL51 breast cancer cell lines: Apoptosis, gene expression and cytotoxicity of nano-quercetin. Nanomedicine 16: 1937–1961. https://doi.org/10.2217/nnm-2021-0070 [Google Scholar] [CrossRef]
Otasek D, Morris JH, Boucas J, Pico AR, Demchak B (2019). Cytoscape automation: Empowering workflow-based network analysis. Genome Biology 20: 185. https://doi.org/10.1186/s13059-019-1758-4 [Google Scholar] [CrossRef]
Pandi S, Kulanthaivel L, Subbaraj GK, Rajaram S, Subramanian S (2022). Screening of potential breast cancer inhibitors through molecular docking and molecular dynamics simulation. BioMed Research International 2022: 3338549. https://doi.org/10.1155/2022/3338549 [Google Scholar] [CrossRef]
Pascual J, Turner NC (2019). Targeting the PI3-kinase pathway in triple-negative breast cancer. Annals of Oncology 30: 1051–1060. https://doi.org/10.1093/annonc/mdz133 [Google Scholar] [CrossRef]
Peacock BN, Scheiderer DJ, Kellermann GH (2017). Biomolecular aspects of depression: A retrospective analysis. Comprehensive Psychiatry 73: 168–180. https://doi.org/10.1016/j.comppsych.2016.11.002 [Google Scholar] [CrossRef]
Phuong NT, Cuong TT, Quang DN (2014). Anti-inflammatory activity of methyl ferulate isolated from Stemona tuberosa Lour. Asian Pacific Journal of Tropical Medicine 7: S327–331. https://doi.org/10.1016/S1995-7645(14)60254-6 [Google Scholar] [CrossRef]
Pilevarzadeh M, Amirshahi M, Afsargharehbagh R, Rafiemanesh H, Hashemi SM, Balouchi A (2019). Global prevalence of depression among breast cancer patients: A systematic review and meta-analysis. Breast Cancer Research and Treatment 176: 519–533. https://doi.org/10.1007/s10549-019-05271-3 [Google Scholar] [CrossRef]
Pinero J, Bravo A, Queralt-Rosinach N, Gutierrez-Sacristan A, Deu-Pons J, Centeno E, Garcia-Garcia J, Sanz F, Furlong LI (2017). DisGeNET: A comprehensive platform integrating information on human disease-associated genes and variants. Nucleic Acids Research 45: D833–D839. https://doi.org/10.1093/nar/gkw943 [Google Scholar] [CrossRef]
Prieto-Vila M, Shimomura I, Kogure A, Usuba W, Takahashi RU, Ochiya T, Yamamoto Y (2020). Quercetin inhibits Lef1 and resensitizes docetaxel-resistant breast cancer cells. Molecules 25: 2576. https://doi.org/10.3390/molecules25112576 [Google Scholar] [CrossRef]
Qin JF, Jin FJ, Li N, Guan HT, Lan L, Ni H, Wang Y (2015). Adrenergic receptor β2 activation by stress promotes breast cancer progression through macrophages M2 polarization in tumor microenvironment. BMB Reports 48: 295–300. https://doi.org/10.5483/BMBRep.2015.48.5.008 [Google Scholar] [CrossRef]
Qin JJ, Yan L, Zhang J, Zhang WD (2019). STAT3 as a potential therapeutic target in triple negative breast cancer: A systematic review. Journal of Experimental and Clinical Cancer Research 38: 195. https://doi.org/10.1186/s13046-019-1206-z [Google Scholar] [CrossRef]
Qiu J, Zhang Z, Hu A, Zhao P, Wei X, Song H, Yang J, Li Y (2022). Integrating UPLC-HR-MS/MS, network pharmacology, and experimental validation to uncover the mechanisms of Jin’gan Capsules against breast cancer. ACS Omega 7: 28003–28015. https://doi.org/10.1021/acsomega.2c01921 [Google Scholar] [CrossRef]
Ru J, Li P, Wang J, Zhou W, Li B et al. (2014). TCMSP: A database of systems pharmacology for drug discovery from herbal medicines. Journal of Cheminformatics 6: 13. https://doi.org/10.1186/1758-2946-6-13 [Google Scholar] [CrossRef]
Ryan J, Ancelin ML (2012). Polymorphisms of estrogen receptors and risk of depression: Therapeutic implications. Drugs 72: 1725–1738. https://doi.org/10.2165/11635960-000000000-00000 [Google Scholar] [CrossRef]
Safran M, Dalah I, Alexander J, Rosen N, Iny Stein T et al. (2010). GeneCards Version 3: The human gene integrator. Database 2010: baq020. https://doi.org/10.1093/database/baq020 [Google Scholar] [CrossRef]
Siegel RL, Miller KD, Wagle NS, Jemal A (2023). Cancer statistics, 2023. CA: A Cancer Journal for Clinicians 73: 17–48. https://doi.org/10.3322/caac.21763 [Google Scholar] [CrossRef]
Silva D, Quintas C, Goncalves J, Fresco P (2022). Contribution of adrenergic mechanisms for the stress-induced breast cancer carcinogenesis. Journal of Cellular Physiology 237: 2107–2127. https://doi.org/10.1002/jcp.30707 [Google Scholar] [CrossRef]
Soudy M, Anwar AM, Ahmed EA, Osama A, Ezzeldin S, Mahgoub S, Magdeldin S (2020). UniprotR: Retrieving and visualizing protein sequence and functional information from universal protein resource (UniProt knowledgebase). Journal of Proteomics 213: 103613. https://doi.org/10.1016/j.jprot.2019.103613 [Google Scholar] [CrossRef]
Szklarczyk D, Gable AL, Lyon D, Junge A, Wyder S et al. (2019). STRING v11: Protein-protein association networks with increased coverage, supporting functional discovery in genome-wide experimental datasets. Nucleic Acids Research 47: D607–D613. https://doi.org/10.1093/nar/gky1131 [Google Scholar] [CrossRef]
Thaker PH, Han LY, Kamat AA, Arevalo JM, Takahashi R et al. (2006). Chronic stress promotes tumor growth and angiogenesis in a mouse model of ovarian carcinoma. Nature Medicine 12: 939–944. https://doi.org/10.1038/nm1447 [Google Scholar] [CrossRef]
Tian W, Liu Y, Cao C, Zeng Y, Pan Y, Liu X, Peng Y, Wu F (2021). Chronic stress: Impacts on tumor microenvironment and implications for anti-cancer treatments. Frontiers in Cell and Developmental Biology 9: 777018. https://doi.org/10.3389/fcell.2021.777018 [Google Scholar] [CrossRef]
Wang S, Liang K, Hu Q, Li P, Song J et al. (2017). JAK2-binding long noncoding RNA promotes breast cancer brain metastasis. Journal of Clinical Investigation 127: 4498–4515. https://doi.org/10.1172/JCI91553 [Google Scholar] [CrossRef]
Wang X, Wang N, Zhong L, Wang S, Zheng Y, Yang B, Zhang J, Lin Y, Wang Z (2020). Prognostic value of depression and anxiety on breast cancer recurrence and mortality: A systematic review and meta-analysis of 282,203 patients. Molecular Psychiatry 25: 3186–3197. https://doi.org/10.1038/s41380-020-00865-6 [Google Scholar] [CrossRef]
Wang XQ, Yue LF, Li YQ, Han YM, Zhai ZG (2018b). Clinical observation of Fang Ji Di Huang Decoction in the treatment of perimenopausal liver kidney yin deficiency generalized anxiety disorder. Chinese Journal of Basic Medicine in Traditional Chinese Medicine 24: 624–626. https://doi.org/10.19945/j.cnki.issn.1006-3250.2018.05.020 [Google Scholar] [CrossRef]
Wang L, Zhan X, Shen X, Li M, Yang J, Yu W, Chen H, Jin B, Mao Z (2018a). P16 promotes the growth and mobility potential of breast cancer both in vitro and in vivo: The key role of the activation of IL-6/JAK2/STAT3 signaling. Molecular and Cellular Biochemistry 446: 137–148. https://doi.org/10.1007/s11010-018-3281-4 [Google Scholar] [CrossRef]
Wee P, Wang Z (2017). Epidermal growth factor receptor cell proliferation signaling pathways. Cancers 9: 52. https://doi.org/10.3390/cancers9050052 [Google Scholar] [CrossRef]
Wishart DS, Feunang YD, Guo AC, Lo EJ, Marcu A et al. (2018). DrugBank 5.0: A major update to the DrugBank database for 2018. Nucleic Acids Research 46: D1074–D1082. https://doi.org/10.1093/nar/gkx1037 [Google Scholar] [CrossRef]
Wu HT, Lin J, Liu YE, Chen HF, Hsu KW, Lin SH, Peng KY, Lin KJ, Hsieh CC, Chen DR (2021). Luteolin suppresses androgen receptor-positive triple-negative breast cancer cell proliferation and metastasis by epigenetic regulation of MMP9 expression via the AKT/mTOR signaling pathway. Phytomedicine 81: 153437. https://doi.org/10.1016/j.phymed.2020.153437 [Google Scholar] [CrossRef]
Wu Y, Luo X, Zhou Q, Gong H, Gao H et al. (2022). The disbalance of LRP1 and SIRPalpha by psychological stress dampens the clearance of tumor cells by macrophages. Acta Pharmaceutica Sinica B 12: 197–209. https://doi.org/10.1016/j.apsb.2021.06.002 [Google Scholar] [CrossRef]
Xu HY, Zhang YQ, Liu ZM, Chen T, Lv CY et al. (2019). ETCM: An encyclopaedia of traditional Chinese medicine. Nucleic Acids Research 47: D976–D982. https://doi.org/10.1093/nar/gky987 [Google Scholar] [CrossRef]
Xu X, Zhang J, Zhang Z, Wang M, Liu Y, Li X (2020). Systems pharmacology in combination with proteomics reveals underlying mechanisms of Xihuang pill against triple-negative breast cancer. Bioengineered 11: 1170–1188. https://doi.org/10.1080/21655979.2020.1834726 [Google Scholar] [CrossRef]
Xue D, Sun H, Li PP (2011). Long-term Chinese herbs decoction administration for management of hot flashes associated with endocrine therapy in breast cancer patients. Chinese Journal of Cancer Research 23: 74–78. https://doi.org/10.1007/s11670-011-0074-7 [Google Scholar] [CrossRef]
Yan J, Chen Y, Luo M, Hu X, Li H, Liu Q, Zou Z (2023). Chronic stress in solid tumor development: From mechanisms to interventions. Journal of Biomedical Science 30: 8. https://doi.org/10.1186/s12929-023-00903-9 [Google Scholar] [CrossRef]
Yan Z, Jiao H, Ding X, Ma Q, Li X et al. (2018). Xiaoyaosan improves depressive-like behaviors in mice through regulating apelin-APJ system in hypothalamus. Molecules 23: 1073. https://doi.org/10.3390/molecules23051073 [Google Scholar] [CrossRef]
Yang D, Guo Q, Liang Y, Zhao Y, Tian X, Ye Y, Tian J, Wu T, Lu N (2020). Wogonin induces cellular senescence in breast cancer via suppressing TXNRD2 expression. Archives of Toxicology 94: 3433–3447. https://doi.org/10.1007/s00204-020-02842-y [Google Scholar] [CrossRef]
Yang H, Xia L, Chen J, Zhang S, Martin V et al. (2019). Stress-glucocorticoid-TSC22D3 axis compromises therapy-induced antitumor immunity. Nature Medicine 25: 1428–1441. https://doi.org/10.1038/s41591-019-0566-4 [Google Scholar] [CrossRef]
Yang FR, Zhu XX, Kong MW, Zou XJ, Ma QY, Li XJ, Chen JX (2022). Xiaoyaosan exerts antidepressant-like effect by regulating autophagy involves the expression of GLUT4 in the Mice hypothalamic neurons. Frontiers in Pharmacology 13: 873646. https://doi.org/10.3389/fphar.2022.873646 [Google Scholar] [CrossRef]
Yu G, Wang LG, Han Y, He QY (2012). clusterProfiler: An R package for comparing biological themes among gene clusters. OMICS: A Journal of Integrative Biology 16: 284–287. https://doi.org/10.1089/omi.2011.0118 [Google Scholar] [CrossRef]
Zhang Y, Lu J, Zhong YJ, Yang CF, Chen L et al. (2022b). Methyl ferulic acid ameliorates alcohol-induced hepatic insulin resistance via miR-378b-mediated activation of PI3K-AKT pathway. Biomedicine and Pharmacotherapy 145: 112462. https://doi.org/10.1016/j.biopha.2021.112462 [Google Scholar] [CrossRef]
Zhang T, Wei W, Chang S, Liu N, Li H (2022a). Integrated network pharmacology and comprehensive bioinformatics identifying the mechanisms and molecular targets of yizhiqingxin formula for treatment of comorbidity with Alzheimer’s disease and depression. Frontiers in Pharmacology 13: 853375. https://doi.org/10.3389/fphar.2022.853375 [Google Scholar] [CrossRef]
Zhang XQ, Yao C, Bian WH, Chen X, Xue JX, Zhu ZY, Ying Y, Xu YL, Wang C (2019). Effects of astragaloside IV on treatment of breast cancer cells execute possibly through regulation of Nrf2 via PI3K/AKT/mTOR signaling pathway. Food Science and Nutrition 7: 3403–3413. https://doi.org/10.1002/fsn3.1154 [Google Scholar] [CrossRef]
Zhao Y, Ma R, Shen J, Su H, Xing D, Du L (2008). A mouse model of depression induced by repeated corticosterone injections. European Journal of Pharmacology 581: 113–120. https://doi.org/10.1016/j.ejphar.2007.12.005 [Google Scholar] [CrossRef]
Zheng Y, Zhang J, Huang W, Zhong LLD, Wang N et al. (2021). Sini san inhibits chronic psychological stress-induced breast cancer stemness by suppressing cortisol-mediated GRP78 activation. Frontiers in Pharmacology 12: 714163. https://doi.org/10.3389/fphar.2021.714163 [Google Scholar] [CrossRef]
Zhu HZ, Liang YD, Ma QY, Hao WZ, Li XJ, Wu MS, Deng LJ, Li YM, Chen JX (2019). Xiaoyaosan improves depressive-like behavior in rats with chronic immobilization stress through modulation of the gut microbiota. Biomedicine and Pharmacotherapy 112: 108621. https://doi.org/10.1016/j.biopha.2019.108621 [Google Scholar] [CrossRef]
Zhu Q, Meng P, Han Y, Yang H, Yang Q, Liu Z, Wang Y, Long M (2022b). Luteolin induced hippocampal neuronal pyroptosis inhibition by regulation of miR-124-3p/TNF-alpha/TRAF6 axis in mice affected by breast-cancer-related depression. Evidence-Based Complementary Alternative Medicine 2022: 2715325. https://doi.org/10.1155/2022/2715325 [Google Scholar] [CrossRef]
Zhu K, Wu Y, He P, Fan Y, Zhong X, Zheng H, Luo T (2022a). PI3K/AKT/mTOR-targeted therapy for breast cancer. Cells 11: 2508. https://doi.org/10.3390/cells11162508 [Google Scholar] [CrossRef]
Zhu ZY, Xue JX, Yu LX, Bian WH, Zhang YF, Sohn KC, Shin IH, Yao C (2018). Reducing postsurgical exudate in breast cancer patients by using San Huang decoction to ameliorate inflammatory status: A prospective clinical trial. Current Oncology 25: e507–e515. https://doi.org/10.3747/co.25.4108 [Google Scholar] [CrossRef]
Zhu Q, Yang L, Yang H, Han Y, Chen Y, He Y (2022c). Quercetin alleviates the progression of breast cancer-related depression via inhibiting the pyroptosis and promoting the immune response. Mediators of Inflammation 2022: 8011988. https://doi.org/10.1155/2022/8011988 [Google Scholar] [CrossRef]
Cite This Article
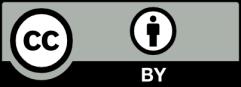
This work is licensed under a Creative Commons Attribution 4.0 International License , which permits unrestricted use, distribution, and reproduction in any medium, provided the original work is properly cited.