Open Access
ARTICLE
The therapeutic mechanism of dexamethasone in lung injury induced by hydrogen sulfide
1 Department of Emergency Medicine, Changshu Hospital Affiliated to Soochow University, The First People’s Hospital of Changshu, Changshu, 215500, China
2 Department of Emergency Medicine, The First Affiliated Hospital of Nanjing Medical University, Nanjing, 210029, China
3 The Department of Oncology, The Affiliated Cancer Hospital of Nanjing Medical University, Jiangsu Cancer Hospital and Jiangsu Institute of Cancer Research, Nanjing, 210000, China
4 Key Lab of Modern Toxicology, Ministry of Education, Department of Toxicology, School of Public Health, Nanjing Medical University, Nanjing, 211166, China
* Corresponding Authors: HONGWEI YE. Email: ; BO CHEN. Email:
# Contributed equally to this work
BIOCELL 2023, 47(9), 2027-2035. https://doi.org/10.32604/biocell.2023.029277
Received 13 February 2023; Accepted 01 June 2023; Issue published 28 September 2023
Abstract
Background: The lung is one of the primary target organs of hydrogen sulfide (H2S), as exposure to H2S can cause acute lung injury (ALI) and pulmonary edema. Dexamethasone (Dex) exerts a protective effect on ALI caused by exposure to toxic gases and is commonly used in the clinic; however, the underlying mechanisms remain elusive, and the dose is unclear. Methods: In vivo experiments: divided C57BL6 mice into 6 groups at random, 12 in each group. The mice were exposed to H2S for 3 h and 5 or 50 mg/kg Dex pretreated before exposure, sacrificed 12 h later. The morphological changes of HE staining and the ultrastructural changes of lungs under transmission electron microscopy were evaluated. The wet/dry ratio of lung tissue was measured. Bronchial alveolar lavage fluid (BALF) protein content and lung permeability index were detected. The expression of AQP5 protein was measured by immunohistochemistry and Western Blot (WB). In vitro experiments: divided human lung adenocarcinoma cell line A549 into 4 groups. 1 μmol/L dexamethasone was added to pre-incubation. The WB analyzed the protein of p-ERK1/2, p-JNK, and p-p38 in MAPK pathway after 1 h of NaHS exposure; six hours after NaHS exposure, the AQP5 protein was measured by WB. Results: Dex treatment could significantly attenuate the H2S-induced destruction to the alveolar wall, increase the wet-to-dry weight ratio and decrease pulmonary permeability index, with high-dose dexamethasone seemingly functioning better. Additionally, our previous studies showed that aquaporin 5 (AQP 5), a critical protein that regulates water flux, decreased both in a mouse and cell model following the exposure to H2S. This study indicates that tThe decrease in AQP 5 can be alleviated by Dex treatment. Additionally, the mitogen activated protein kinase (MAPK) pathway may be involved in the protective effects of Dex in ALI caused by exposure to H2S since H2S-induced MAPK activation could be inhibited by Dex. Conclusion: The present results indicate that AQP 5 may be considered a therapeutic target for Dex in H2S or other hazardous gases-induced ALI.Keywords
Glucocorticoids (GCs), such as dexamethasone, are used for the early treatment of acute pulmonary edema to suppress inflammation, endotoxin, allergy, shock, and exert immunosuppressive effects (Marik et al., 2011; Hemmerling et al., 2017; Thompson, 2003). Therefore, GC is commonly used to treat clinical acute lung injury (ALI), including injury after exposure to acute toxic gases.
Hydrogen sulfide (H2S), an acidic, colorless, and highly toxic gas with a characteristic odor of rotten eggs, is widely used in industries such as pulp and paper manufacturing, petroleum refineries, sewage treatment, and synthetic fiber. Exposure to high-concentration H2S poses a serious hazard to human health. Exposure to moderate levels (50–100 ppm) of H2S may cause respiratory irritation and olfactory fatigue, and chronic exposure to 250–500 ppm of H2S can lead to acute lung injury (ALI) (Dorman et al., 2000). Previous studies have revealed that treatment with dexamethasone (Dex) significantly ameliorates H2S or other hazardous gases-induced ALI (Wang et al., 2014; Al-Harbi et al., 2016). However, the underlying mechanism is very complex and difficult to be fully elucidated, which leaves its usage and dosage an unsettled issue. Therefore, one of the objectives of this study is to explore the protective effects of different doses of dexamethasone on hydrogen sulfide-induced acute lung injury to guide clinical dexamethasone application.
Aquaporin 5 (AQP 5), one of the most important aquaporins in type I pulmonary alveolar epithelial cells, serves as the main way for the transport of osmotically driven water in the lung (Verkman, 2002). Decreased AQP 5 expression causes the homeostatic disruption of water transport, leading to ALI. In severe acute pancreatitis-induced ALI, Xu et al. (2017) found that AQP 5 expression significantly decreased, resulting in lung edemas in the rat lung. Meanwhile, in LPS-induced septic rat lungs, the AQP 5 protein and mRNA levels were markedly reduced (Tao et al., 2016). In addition, our previous study demonstrated that H2S exposure can cause a marked decrease in AQP 5 expression in both mouse lungs and lung cell models (Xu et al., 2017). Therefore, it can be hypothesized that AQP 5 may be a significant therapeutic target of Dex in acute lung injury (ALI) stimulated by H2S. Therefore, another purpose of this study is to investigate the effect of Dex on addressing the reduction of AQP 5 expression induced by H2S, and to explore the possible mechanism of its therapeutic effect, based on the in vivo and in vitro studies.
The C57BL6 male mice used in the experiment were provided by The Animal Experimental Center of Nanjing Medical University, weighing 18–22 g. They were maintained under specific pathogen-free conditions with a constant temperature of 18°C–22°C, relative humidity of 30%–50%, and a 12/12 h light/dark illumination cycle. Clean water and standard food were provided. All experiments involving animal samples were carried out according to the guidelines of the Case and Use of Laboratory Animals of the National Institutes of Health, and all procedures have been approved by the Institutional Animal Care and Use Committee of Nanjing Medical University (permit no. 20110521).
Experimental groups and treatment
The H2S exposure mouse model was set up according to methods described by Dorman et al. (2000) and in our previous study (Xu et al., 2017). We divided 72 male C57BL6 mice into six groups in random; (I) the control group (normal saline); (II) H2S exposure group; (III) Dex (5 mg/kg) + H2S group; (IV) Dex (50 mg/kg) + H2S group; (V) Dex (5 mg/kg) group; and (VI) Dex (50 mg/kg) group. A total of 36 mice were exposed to 300 ppm H2S gas for 3 h. The mice were placed inside a cylindrical glass chamber (SpecialtyGlass, Willow Grove, PA, USA), and two mass flow controllers with Kalrez seals and a microprocessor control unit were used to control the flow rates of compressed air and H2S to achieve the desired H2S concentration in the exposure chamber. Following the exposure to H2S (300 ppm) for 3 h, the mice were returned to normal air and sacrificed 12 h later. For the Dex-treated group (36 mice), Dex was administrated 1 h prior to the H2S exposure. The mice were injected subcutaneously with 5 and 50 mg/kg Dex (Joh et al., 2012; Wigenstam et al., 2015).
The rabbit anti-mouse antibodies for extracellular regulated protein kinases1/2 (ERK1/2) (1:1000, catalog no. #4695), phosphorylated extracellular regulated protein kinases1/2 (p-ERK1/2) (1:1000, catalog no. #4370), c-Jun N-terminal kinase (JNK) (1:1000, catalog no. #9252), phosphorylated c-Jun N-terminal kinase (p-JNK) (1:1000, catalog no. #4668), p38 mitogen activated protein kinase (p38 MAPK) (1:1000, catalog no. #8690) and phosphorylated p38 mitogen activated protein kinase (p-p38 MAPK) (1:1000, catalog no. #9211) were obtained from Cell Signaling Technology, Danvers, MA, USA. Goat anti-AQP 5 antibody (1:200) was obtained from Santa Cruz Biotechnology, Robles, CA, USA (catalog no. sc-9890).
The human lung cell line A549 was obtained from the American Type Culture Collection, Rockefeller, Maryland, America and was grown in Roswell Park Memorial Institute-1640 medium (Sigma-Aldrich, St. Louis, USA) supplemented with 2 ml glutamine and 10% FBS. Cells were incubated at 37°C with 5% CO2. Trypsin-EDTA solution was used for trypsinization and sub-culturing of the cells.
Treatment of A549 cells with dexamethasone and NaHS
The control (ctrl) Dex group and the H2S + Dex group were pre-treated with 1 μmol Dex for 24 h in a 37°C incubator, then the ctrl and H2S groups were subjected to medium exchange. For the H2S exposure, the H2S and H2S + Dex groups were incubated with a final concentration of 500 μM NaHS in the medium, and the dishes were sealed and kept for 30 min in a 37°C separate incubator to avoid affecting other cells and then unsealed with the NaHS removed. After 1 and 6 h, the cells were collected to measure the expression of MAPK pathway proteins (p38, ERK1/2, and JNK) and the AQP 5 protein.
The right lower lobe of the lungs from each mouse was removed following 12 h exposure to H2S and fixation with 4% paraformaldehyde for 24 h. Then, they were embedded in paraffin, cut into 5 μm thin continuous sections, and stained by hematoxylin and eosin (H&E) for 5 min at room temperature for microscopic analysis. Then, to estimate lung injury, a semi-quantitative scoring system was used to score the lungs, which was used in our previous studies (Wang et al., 2014). Images were collected by an Olympus BX41 microscope and handled by Image-Pro Plus 4.5 software (Media Cybernetics, Rockville, MD, USA).
Detection of the wet-to-dry weight ratio (W/D) of the lung
The weight ratio of W/D of mice’s lungs was determined after the 12-h exposure to H2S (Xu et al., 2017). The left lungs of mice were resected to measure the proportion of wet to dry weight. The W/D weight proportion was acquired by wet weight divided by dry weight, with the dry weight obtained by first heating their left lungs at 80°C for 48 h in an oven.
Determination of the pulmonary permeability and bronchoalveolar lavage fluid (BALF) protein content
Four mice were selected from each group, and BALF was collected from each mouse after they were centrifuged for 10 min (4°C, 800 × g). The protein concentration in the blood serum and BALF supernatant were determined with a spectrophotometer (Beckman Coulter, Inc., Brea, CA, USA) at a wavelength of 564 nm based on the Pierce BCA Protein assay (Thermo Fisher Scientific, Inc., Waltham, USA). The pulmonary permeability index (PPI) was defined as the proportion of total proteins (TP) in BALF to TP in serum. The lung tissue was not used for other purposes after bronchoalveolar lavage.
Tissue sections of 4 µm-thick from the lower lobe of the right lung in mice were prepared, and placed into methanol with 0.3% hydrogen peroxide to restrain endogenous peroxidases in them for 30 min. Then, the sections were soaked in 0.05% protease XIV at 37°C for 5 min to complete antigen retrieval. Then they were incubated overnight at 4°C with anti-normal goat IgG (1:500) or anti-AQP 5 antibodies (1:50) being the control. After that, the donkey anti-goat secondary antibody was incubated on the slides at room temperature for 45 min followed by the same procedure with a complex of streptavidin-biotin peroxidase at room temperature for 45 min (Vectastain ABC kit; Vector Laboratories, Newark, CA, USA). The reaction was visualized with diaminobenzidine for 10 s. Lastly, the slides were re-stained with hematoxylin and observed using a 40X objective (Olympus BX41).
Cell lysates were prepared with RIPA buffer (200 μL, Sigma-Aldrich Merck KGaA, St. Louis, MO, USA), Phosphatase Inhibitor (Roche 1 tablet plus 10 mL RIPA in proportion), and Protease Inhibitor Cocktail (500:1, Sigma-Aldrich Merck KGaA) per dish cells (25 T), lysed on the ice for 10 min. Then, they were scraped off and collected into the enzyme-inactivated EP tube. Then we centrifuged the lysates for 15 min at 4°C (12,000 × g). Then the concentrations of protein in the supernatants collected were measured using Pierce BCA protein assay reagent. Samples containing 40 μg protein were electrophoresed on 10% sodium dodecyl sulfate-polyacrylamide gels and then blotted onto polyvinylidene fluoride membranes of 0.22-mM (EMD Millipore, Burlington, MA, USA). The membranes were in 5% non-fat milk to block and then incubated with the polyclonal AQP 5 antibody (1:200), rabbit antibody p-ERK1/2, ERK1/2, p-p38, p38, p-JNK, and JNK at 4°C overnight. Subsequently, TBST was employed to wash the membranes three times at room temperature, then a 1:5000 dilution of secondary AffiniPure donkey anti-goat antibody IgG (Jackson ImmunoResearch Laboratories, West Grove, PA, USA) was applied to incubate the membranes for 2 h. Membranes were washed again six times with TBST, and the chemiluminescent horseradish peroxidase substrate (EMD Millipore) was applied. The densities of bands of each sample were standardized to that of β-actin (1:10000, CMCTAG, Dover, DE, USA).
Mean ± standard deviation (SD) of the mean was calculated with SPSS software (version 19.0; SPSS, Inc., Chicago, IL, USA). The least significant difference multiple comparison test or Dunnett’s test was used for pairwise comparisons. The statistical significances between each group were calculated by One-way ANOVA. If p-value < 0.05, the results have statistical significance. All experiments were conducted with three or more independent repetitions.
Dex attenuates H2S-induced lung injury
Mice were first pre-treated with 5 and 50 mg/kg doses of Dex for 1 h and then were exposed to H2S for 12 h. Then their lung morphological changes were observed. As displayed by H&E staining (Fig. 1), H2S exposure caused ALI, characterized by inflammatory cells infiltration, alveolar and interstitial hemorrhage, alveolar collapse, and widespread alveolar septal thickening (Fig. 1D). Notably, treatment with both high and low doses of Dex significantly attenuated H2S-induced lung injury (Figs. 1E and 1F), while treatment with Dex in control groups without exposure to H2S caused no obvious changes in the lung tissue (Figs. 1A–1C). The histological scores of the mouse lung tissue are presented in Fig. 1G.
Figure 1: Effect of H2S on the lung tissue with different doses of Dexamethasone (Dex). (A) The control group. (B) The control + Dex (5 mg/kg) group. (C) The control + Dex (50 mg/kg) group. (D) H2S exposure group. (E) Dex (5 mg/kg) + H2S group. (F) H2S + Dex (50 mg/kg) group. (G) Histological scores for all groups of mice. The arrowheads indicate interlobular septal thickening. The & indicates alveolar hemorrhage and interstitial hemorrhage. The * indicates alveolar collapse and atelectasis. Scale bar, 100 μm. #p < 0.05 vs. H2S. *p < 0.05 vs. control.
Dex improves the permeability of lung tissue in H2S-poisoned mice
As PPI, W/D ratios, and the BALF protein content are important indicators of lung permeability in vivo (Huang and Li, 2016). We examined these parameters 12 h after H2S exposure. As displayed in Fig. 2, H2S exposure increased the W/D ratios of the lung tissue, indicating the occurrence of pulmonary edema after H2S exposure. Meanwhile, Dex treatment substantially reduced the lung tissue W/D ratios. Besides, the BALF protein content and PPI were also examined to further support the hypothesis of H2S-inducing ALI. As presented in Figs. 3A and 3B, H2S exposure significantly increased BALF protein content and PPI, and pre-treatment with Dex (5 and 50 mg/kg) markedly inhibited these processes, which confirmed Dex was effective against acute lung injury induced by H2S.
Figure 2: Influence of H2S on the lung W/D ratio with different doses of dexamethasone (Dex). The lung W/D ratio of dexamethasone-pretreated mice after 12 h exposure to H2S. #p < 0.05 vs. H2S. *p < 0.05 vs. control.
Figure 3: Impact of H2S on lung permeability with different doses of dexamethasone (Dex). (A) The protein content in bronchoalveolar lavage fluid (BALF) of Dex-pretreated mice after the 12 h exposure of H2S. (B) The pulmonary permeability index of Dex-pretreated mice after the 12 h exposure to H2S. #p < 0.05 vs. H2S. *p < 0.05 vs. control.
Dexamethasone upregulates aquaporin 5 expression in mice lung tissues
H2S exposure leads to pulmonary edema and AQP 5 plays a pivotal role in water transport; therefore, the research aims at exploring the potential effects of H2S and Dex on AQP 5 expression. As presented in Fig. 4, after 12-h H2S exposure, the protein expression of AQP 5 significantly declined, while Dex substantially reversed the H2S-induced downregulation of the expression of AQP 5, and Dex apparently increased the AQP 5 protein expression in lung tissue exposed to 50 mg/kg H2S. Immunohistochemical analysis testified that in the H2S exposure group, the AQP 5 protein expression dramatically decreased in the lung tissue after exposure to H2S for 12 h and exhibited the lowest mean optical density (MOD) value, which was consistent with the W/B results. Meanwhile, the Dex application significantly increased the MOD value (Fig. 5), suggesting the direct regulatory effect of Dex on AQP 5.
Figure 4: Influence of H2S on the aquaporin 5 (AQP 5) in mice lungs with different doses of dexamethasone (Dex) with mice pre-treated with 5 and 50 mg/kg Dex. (A) Immunoblots of AQP 5 in lung tissues after contact with H2S for 12 h. (B) The densities of the blot of the AQP 5 idio-bands were quantified in each group (28 KD) of lung tissues. The results are relative expressions with β-actin as the internal reference after comparison with the controls. Values are represented as the mean ± S.D for three times of independent experiments. #p < 0.05 vs. H2S. *p < 0.05 vs. control.
Figure 5: Influence of H2S on the aquaporin 5 (AQP 5) in mice lungs with different doses of dexamethasone (Dex). AQP 5 expression in the alveolus is indicated by brown parts. (A) Control group. (B) Control and Dex (5 mg/kg) group. (C) Control and Dex (50 mg/kg) group. (D) H2S exposure group. (E) H2S exposure and Dex (5 mg/kg) group. (F) H2S exposure and Dex (50 mg/kg) group. (G) The mean optical density of the six groups. The scale bar represents 20 μm. #p < 0.05 vs. H2S. *p < 0.05 vs. control.
Effects of dexamethasone on AQP 5 in A549 cells
To further explore the regulation of Dex on AQP 5 expression, the human lung cell line A549 was used. As presented in Figs. 6A and 6B, after the exposure to H2S for 6 h, the AQP 5 expression in the A549 cells significantly decreased. However, following Dex pre-treatment, the decreased expression level of AQP 5 in A549 cells mediated by NaHS was markedly attenuated. The results indicate that AQP 5 may be a potential target protein for Dex.
Figure 6: Influence of NaHS on the aquaporin 5 (AQP 5) protein in A549 cells prepared with dexamethasone (Dex). (A) Immunoblots of APQ 5 in different treated A549 cells after 6 h. (B) Quantification of the densities of the blot for the AQP 5 idio-bands in A549 cell of each group (28 KD). The results were relative expressions with β-actin as the internal reference after contrasted with the control. Values were denoted as the mean ± S.D for three independent experiments. #p < 0.05 vs. H2S. *p < 0.05 vs. control.
Effects of dexamethasone on the mitogen activated protein kinase signaling pathway
Since the MAPK signaling pathway plays an indispensable role in the regulation of AQP 5, this study examines whether H2S exposure activates the MAPK signaling pathway and whether Dex treatment ameliorates this process. After the A549 cells were incubated for 1 h with NaHS, the phosphorylation of JNK, p38 MAPK, and ERK1/2 were assayed. As presented in Fig. 7, the phosphorylation of JNK, p38 MAPK, and ERK1/2 increased, indicating that NaHS treatment activated the pathways of JNK, p38 MAPK, and ERK1/2. While pre-treatment with Dex inhibited the activation of p38 MAPK and ERK1/2 mediated by H2S, the phosphorylation showed no significant difference in JNK when compared with the H2S exposure group.
Figure 7: Effect of NaHS for 1 h on the mitogen activated protein kinase (MAPK)-associated proteins in A549 cells prepared with dexamethasone (Dex). Measurement and quantification of phosphorylated extracellular regulated protein kinases1/2 (p-ERK1/2), phosphorylated c-Jun N-terminal kinase (p-JNK), and p-p38 protein expression are shown. The phosphorylated levels were relative expressions with T-ERK1/2, T-JNK, T-p38, and β-actin as the internal reference after compared with the controls. Values are represented as the mean ± S.D for the mean of three independent experiments. #p < 0.05 vs. H2S. *p < 0.05 vs. control.
The majority of H2S-associated deaths reported proved to be related to industrial exposure (Sams et al., 2013), such as in heavily polluted environments, such as sewers, manure pits, oil, and acid gas industries (Arnold et al., 1985). The investigation showed that 1364 cases of acute occupational poisoning were caused by H2S from 1993 to 2016, second only to that by CO poisoning (Sun et al., 2018). At the same time, there is no specific detoxification drug for poisoning by H2S gas, as the knowledge about it is still insufficient. Therefore, ALI caused by exposure to H2S is considered a leading cause of mortality. Moreover, our previous study has demonstrated that H2S-exposed lung specimens exhibited significant morphological changes (Wang et al., 2014), such as inflammatory cell infiltration. Given the anti-inflammatory and anti-allergic effects, among others, DEX has been used in other pulmonary diseases and is considered a potential therapeutic drug for ALI caused by exposure to H2S (Zhao et al., 2022; Xiao et al., 2020; Geng et al., 2018). However, whether Dex can really exert protective effects against it remains unknown.
There are two primary mechanisms of water transport in the alveoli, with one accompanied by the active transport of Na+ and the other through AQPs on the alveolar epithelium (Marik et al., 2011). Our previous study demonstrated that Dex treatment markedly increased the α-epithelial Na+ channel (α-ENaC), which was accountable for the clearance of alveolar fluid. In rats and mice, AQP 5 mainly exists in the apical membrane of alveolar type I and type II cells in the lung, which is involved in water transport between the vasculature and alveolar directly (Hasan et al., 2014). Our previous study showed that H2S exposure could inhibit the expression of AQP5 in lung tissue. In vitro, the expression of AQP5 was significantly reduced, and the MAPK family was fully activated after NaHS exposure. Blocking the expression of p-ERK1/2 and p-p38 could partially reverse the inhibition of AQP5 expression (Xu et al., 2017). Therefore, we believe that ERK and p38MAPK signaling pathways are involved in the regulation of AQP5.
Previous studies analyzed the impact of Dex on AQP 5 expression in the lung pathological state. The expression of AQP 5 was suppressed in the mouse model of asthma induced by ovalbumin and Dex; ambroxol and terbutaline proved to increase the lowered expression, reducing edema and respiratory tract secretions. Similarly, emodin and Dex can lessen pulmonary edema by enhancing the lowered expression of AQP 5 in the rat model of ALI induced by acute pancreatitis (Xu et al., 2016; Dong et al., 2012). In this case, we wondered whether Dex could also increase the expression of AQP5 in the lung tissue after H2S inhalation poisoning when the expression of AQP5 decreased, to reduce pulmonary edema. To investigate the potential role of Dex on AQP 5, in vivo and in vitro experiments were conducted to show that the expression of AQP 5 increased after Dex application in this study. The immunohistochemistry results suggested that the groups of Dex (5 mg/kg) + H2S and Dex (50 mg/kg) + H2S exhibited a higher MOD compared with the H2S exposure group. Additionally, in the WB analysis, a high dose of Dex exerted more pronounced effects on AQP 5 regulation. These results were consistent with the ratio to lung W/D, LPI, and lung histology score of the pulmonary edema mediated by Dex, implying that Dex, especially high-dose Dex, improves transmembrane transport of lung water, which in turn relieves acute pulmonary edema. These results, taken together, suggest that apart from the α-ENaC, AQP 5 may be a potential target protein for Dex, at least in the case of ALI caused by exposure to H2S. These results were also partially in line with the results of an OVA-induced mouse pulmonary edema model, in which Dex also exerted protective effects through the upregulation of AQP 5 (Dong et al., 2008).
Relevant research has revealed that the MAPK signaling, especially p38 MAPK, and ERK1/2, are associated with alveolar fluid clearance and water transport. For example, following H2S exposure, the ERK1/2 pathway was activated, and inhibition of ERK 1/2 significantly upregulated α-ENaC expression (Jiang et al., 2014). The relation among the expression of ERK1/2, p38 MAPK, and AQP 5 was verified in our previous study in NaHS-treated cells, as idio-inhibitors of ERK1/2 and p38 MAPK significantly restored the expression of AQP 5, showing that both ERK1/2 and p38 MAPK were involved in the regulation of AQP 5 after exposure to NaHS. We found that ERK1/2 and p38 MAPK were markedly activated in 1 h, and this time point was chosen according to our previous study, in which 1 h of NaHS exposure had a more significant influence than any other groups (Xu et al., 2017). Meanwhile, Dex treatment attenuated the activation of ERK1/2 and p38 MAPK induced by H2S and augmented AQP 5 expression. These results indicate that Dex attenuates the NaHS-induced decrease of AQP 5 via ERK1/2 and p38 MAPK signaling.
In conclusion, the research outlines the molecular mechanism of the therapeutic actions of Dex on ALI induced by H2S. The pathways of p38 MAPK and ERK1/2 signaling are shown to participate in AQP 5 regulation mediated by Dex (Fig. 8). However, the potential mechanism of Dex on ALI caused by exposure to H2S remains elusive, and the associations between the p38 MAPK and ERK1/2 signaling pathways and AQP 5 expression needs further investigation. Collectively, these findings reveal a previously unrecognized preventive effect mediated by Dex, and AQP 5 may be a potential molecular target for ALI caused by exposure to toxic gases.
Figure 8: Schematic representation showing abnormal expression of the aquaporin 5 (AQP 5) induced by H2S. In this model, the partial therapeutic effect of dexamethasone (Dex) may be due to an inhibition of the activation of p38 mitogen activated protein kinase (p38 MAPK) and extracellular regulated protein kinases1/2 (ERK1/2) signaling pathways, which in turn partially reverses the inhibited expression of AQP 5 protein. Therefore, the transmembrane transport of water recovers, which eventually alleviates acute lung injury (ALI) and pulmonary edema.
Acknowledgement: None.
Funding Statement: This work was supported by the Science and Technology Plan Project of Jiangsu Province (Grant No. BL2014088), the Program of Changshu Science and Technology Bureau (Grant No. CS201813), Suzhou Medical and Health Science and Technology Innovation Project (Grant No. SKJY2021007), and Suzhou Gusu Health Talent Project (Grant No. GSWS2022101).
Author Contributions: CX, BC, and JZ drew up the study direction and designed the study; CX and XP established the animal models and performed the experiments; CY and LJ collected and organized the data; HY and JW analyzed the data; CX, CY, XP, and BC prepared the manuscript. All authors read and approved the final manuscript.
Availability of Data and Materials: The datasets generated during and/or analyzed during the current study are available from the corresponding author on reasonable request.
Ethics Approval: This research was approved by the Ethics Committee of the First People’s Hospital of Changshu (Changshu, China, Approval No. 2017003).
Conflicts of Interest: The authors declare that they have no conflicts of interest to report regarding the present study.
References
Al-Harbi NO, Imam F, Al-Harbi MM, Ansari MA, Zoheir KM, Korashy HM, Sayed-Ahmed MM, Attia SM, Shabanah OA, Ahmad SF (2016). Dexamethasone attenuates LPS-induced acute lung injury through inhibition of NF-κB, COX-2, and pro-inflammatory mediators. Immunological Investigations 45: 349–369. https://doi.org/10.3109/08820139.2016.1157814 [Google Scholar] [PubMed] [CrossRef]
Arnold IM, Dufresne RM, Alleyne BC, Stuart PJ (1985). Health implication of occupational exposures to hydrogen sulfide. Journal of Occupational Medicine 27: 373–376. https://doi.org/10.1097/00043764-198505000-00018 [Google Scholar] [PubMed] [CrossRef]
Dong C, Lu J, Wang G, Li B, Xiao K, Chen Z, Bai C (2008). Effects of dexamethasone on alveolar fluid clearance in mouse asthma models. Pharmaceutical Care and Research 8: 24–27. [Google Scholar]
Dong C, Wang G, Li B, Xiao K, Ma Z, Huang H, Wang X, Bai C (2012). Anti-asthmatic agents alleviate pulmonary edema by upregulating AQP1 and AQP5 expression in the lungs of mice with OVA-induced asthma. Respiratory Physiology & Neurobiology 181: 21–28. https://doi.org/10.1016/j.resp.2011.12.008 [Google Scholar] [PubMed] [CrossRef]
Dorman DC, Brenneman KA, Struve MF, Miller KL, James RA, Marshall MW, Foster PM (2000). Fertility and developmental neurotoxicity effects of inhaled hydrogen sulfide in Sprague-Dawley rats. Neurotoxicology and Teratology 22: 71–84. https://doi.org/10.1016/S0892-0362(99)00055-0 [Google Scholar] [PubMed] [CrossRef]
Geng P, Ma T, Xing J, Jiang L, Sun H, Zhu B, Zhang H, Xiao H, Wang J, Zhang J (2018). Dexamethasone ameliorates H2S-induced acute lung injury by increasing claudin-5 expression via the PI3K pathway. Human & Experimental Toxicology 37: 626–635. https://doi.org/10.1177/0960327117721961 [Google Scholar] [PubMed] [CrossRef]
Hasan B, Li FS, Siyit A, Tuyghun E, Luo JH, Upur H, Ablimit A (2014). Expression of aquaporins in the lungs of mice with acute injury caused by LPS treatment. Respiratory Physiology & Neurobiology 200: 40–45. https://doi.org/10.1016/j.resp.2014.05.008 [Google Scholar] [PubMed] [CrossRef]
Hemmerling M, Nilsson S, Edman K, Eirefelt S, Russell W et al. (2017). Selective nonsteroidal glucocorticoid receptor modulators for the inhaled treatment of pulmonary diseases. Journal of Medicinal Chemistry 60: 8591–8605. https://doi.org/10.1021/acs.jmedchem.7b01215 [Google Scholar] [PubMed] [CrossRef]
Huang R, Li M (2016). Protective effect of Astragaloside IV against sepsis-induced acute lung injury in rats. Saudi Pharmaceutical Journal 24: 341–347. https://doi.org/10.1016/j.jsps.2016.04.014 [Google Scholar] [PubMed] [CrossRef]
Jiang L, Wang J, Su C, Qian W, Chen J, Zhu B, Zhang H, Xiao H, Zhang J (2014). α-ENaC, a therapeutic target of dexamethasone on hydrogen sulfide induced acute pulmonary edema. Environmental Toxicology and Pharmacology 38: 616–624. https://doi.org/10.1016/j.etap.2014.08.012 [Google Scholar] [PubMed] [CrossRef]
Joh EH, Gu W, Kim DH (2012). Echinocystic acid ameliorates lung inflammation in mice and alveolar macrophages by inhibiting the binding of LPS to TLR4 in NF-κB and MAPK pathways. Biochemical Pharmacology 84: 331–340. https://doi.org/10.1016/j.bcp.2012.04.020 [Google Scholar] [PubMed] [CrossRef]
Marik PE, Meduri GU, Rocco PR, Annane D (2011). Glucocorticoid treatment in acute lung injury and acute respiratory distress syndrome. Critical Care Clinics 27: 589–607. https://doi.org/10.1016/j.ccc.2011.05.007 [Google Scholar] [PubMed] [CrossRef]
Sams RN, Carver HW 2nd, Catanese C, Gilson T (2013). Suicide with hydrogen sulfide. The American Journal of Forensic Medicine and Pathology 34: 81–82. https://doi.org/10.1097/PAF.0b013e3182886d35 [Google Scholar] [PubMed] [CrossRef]
Sun G, Zhou C, Yang X, Yang B, Chen X (2018). Analysis on current situation of occupational poisoning in China from 1993 to 2016 and prevention and control countermeasures. Journal of Safety Science and Technology 14: 187–192. [Google Scholar]
Tao B, Liu L, Wang N, Wang W, Jiang J, Zhang J (2016). Effects of hydrogen-rich saline on aquaporin 1, 5 in septic rat lungs. The Journal of Surgical Research 202: 291–298. https://doi.org/10.1016/j.jss.2016.01.009 [Google Scholar] [PubMed] [CrossRef]
Thompson BT (2003). Glucocorticoids and acute lung injury. Critical Care Medicine 31: S253–S257. https://doi.org/10.1097/01.CCM.0000057900.19201.55 [Google Scholar] [PubMed] [CrossRef]
Verkman AS (2002). Physiological importance of aquaporin water channels. Annals of Medicine 34: 192–200. https://doi.org/10.1080/ann.34.3.192.200 [Google Scholar] [CrossRef]
Wang J, Zhang H, Su C, Chen J, Zhu B, Zhang H, Xiao H, Zhang J (2014). Dexamethasone ameliorates H2S-induced acute lung injury by alleviating matrix metalloproteinase-2 and -9 expression. PLoS Clinical Trials 9: e94701. https://doi.org/10.1371/journal.pone.0094701 [Google Scholar] [PubMed] [CrossRef]
Wigenstam E, Koch B, Bucht A, Jonasson S (2015). N-acetyl cysteine improves the effects of corticosteroids in a mouse model of chlorine-induced acute lung injury. Toxicology 328: 40–47. https://doi.org/10.1016/j.tox.2014.12.008 [Google Scholar] [PubMed] [CrossRef]
Xiao J, Lin F, Pan L, Dai H, Jing R, Lin J, Liang F (2020). Dexamethasone on alleviating lung ischemia/reperfusion injury in rats by regulating PI3K/AKT pathway. Zhonghua Wei Zhong Bing Ji Jiu Yi Xue 32: 188–193. https://doi.org/10.3760/cma.j.cn121430-20190723-00035 [Google Scholar] [PubMed] [CrossRef]
Xu J, Huang B, Wang Y, Tong C, Xie P, Fan R, Gao Z (2016). Emodin ameliorates acute lung injury induced by severe acute pancreatitis through the up-regulated expressions of AQP1 and AQP5 in lung. Clinical and Experimental Pharmacology & Physiology 43: 1071–1079. https://doi.org/10.1111/1440-1681.12627 [Google Scholar] [PubMed] [CrossRef]
Xu C, Jiang L, Zou Y, Xing J, Sun H, Zhu B, Zhang H, Wang J, Zhang J (2017). Involvement of water channel aquaporin 5 in H2S-induced pulmonary edema. Environmental toxicology and pharmacology 49: 202–211. https://doi.org/10.1016/j.etap.2016.12.013 [Google Scholar] [PubMed] [CrossRef]
Zhao Y, Zhao L, Zhao J, Lu J, Tian W, Hu J, Su B, Fu L, Guo R (2022). Dexamethasone up-regulates TNFAIP3 to attenuate inflammatory response with smoke inhalation-induced acute lung injury based on the GEO database. Journal of Chinese Pharmaceutical Sciences 9: 689–697. [Google Scholar]
Cite This Article
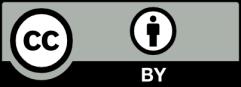
This work is licensed under a Creative Commons Attribution 4.0 International License , which permits unrestricted use, distribution, and reproduction in any medium, provided the original work is properly cited.