Open Access
ARTICLE
The potency of N, N'-diphenyl-1,4-phenylenediamine and adipose-derived stem cell co-administration in alleviating hepatorenal dysfunction complications associated with type 1 diabetes mellitus in rats
1 Department of Chemistry, College of Science, King Faisal University, Al-Ahsa, Saudi Arabia
2 Chemistry Department, Faculty of Science, Sohag University, Sohag, Egypt
3 Department of Biology, College of Science, University of Jeddah, Jeddah, Saudi Arabia
4 Department of Biotechnology, College of Science, Taif University, Taif, Saudi Arabia
5 Fellow of Biochemistry, Genetic Unit, Children Hospital, Faculty of Medicine, Mansoura University, Mansoura, Egypt
6 Chemistry Department (Biochemistry Branch), Faculty of Science, Suez University, Suez, Egypt
7 Department of Clinical Laboratories Sciences, College of Applied Medical Sciences, Shaqra University, Riyadh, Saudi Arabia
8 Biology Department, Faculty of Science, Mansoura University, Mansoura, Egypt
9 Department of Chemistry, Organic Chemistry Division, College of Science, Mansoura University, Mansoura, Egypt
10 Zoology Department, Faculty of Science, Arish University, North Sinai, Egypt
* Corresponding Authors: HANY M. ABD EL-LATEEF. Email: ; SHADY G. EL-SAWAH. Email:
(This article belongs to the Special Issue: Cell-Based Regenerative Therapies)
BIOCELL 2023, 47(8), 1885-1895. https://doi.org/10.32604/biocell.2023.030680
Received 18 April 2023; Accepted 01 June 2023; Issue published 28 August 2023
Abstract
Background: The increasing occurrence of diabetes mellitus (DM) noted worldwide has considerably elicited concern in the recent past. DM is associated with elevated vascular complications, morbidity, mortality, and poor quality of life. In this context, mesenchymal stem cells (MSCs) have shown significant therapeutic potentialities in managing and curing type 1 DM owing to their self-renewable, immunosuppressive, and differentiation capacities. We investigated the potential action of N, N′-diphenyl-1,4-phenylenediamine (DPPD), a well-known synthetic antioxidant to enhance the therapeutic ability of the adipose-derived stem cells (AD-MSCs) in alleviating kidney and liver complications in diabetic rats. Methods: Over the four weeks of experiments, albino male rats (n = 36) were split into six test groups: control, DPPD (250 mg/kg, i.p.), STZ-diabetic (D), D+DPPD, D+AD-MSCs (1 × 10 cell/rat, i.v.), and D+AD-MSCs+DPPD treated groups. Results: Significant declines in the renal and hepatic oxidative stress markers (MDA, ROS, and AGEs) were observed coupled with a significant elevation in many antioxidant marker levels (GSH, SOD, CAT, GPx, HO-1, and TAC) in the diabetic rats treated with either DPPD or AD-MSCs or their co-administered injection compared to the diabetic untreated rats. This was suggested to be the leading cause of amelioration of the kidney functions (as measured by urea, uric acid, and creatine levels) and liver functions (as evidenced by the levels of AST, ALT, ALP, bilirubin, total proteins, albumin, and globulins). Conclusion: DPPD and AD-MSCs co-administration showed superior results in terms of the enhancement of the relative hepato-renal function, indicating the beneficial role of DPPD supplementation in increasing the therapeutic potential of AD-MSCs.Graphical Abstract
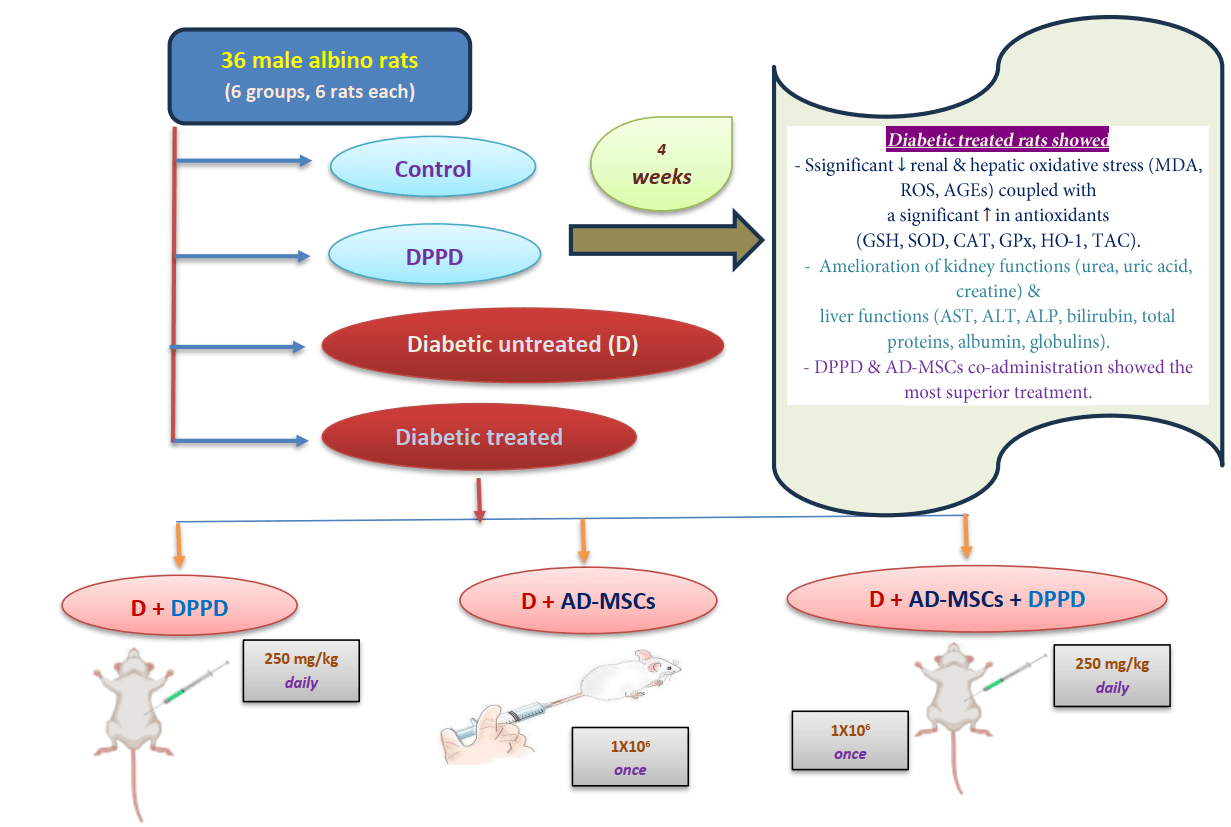
Keywords
Supplementary Material
Supplementary Material FileList of Abbreviations
AD-MSCs | Adipose-derived mesenchymal stem cells |
AGEs | Advanced glycation end products |
BUN | Blood urea nitrogen |
CAT | Catalase |
CD | Cluster of differentiation |
CP | Cisplatin |
D | Diabetic |
DM | Diabetes mellitus |
DMEM | Dulbecco’s modified Eagle’s medium |
DN | Diabetic nephropathy |
DPPD | N N′-diphenyl-1, 4-phenylenediamine |
FBG | Fasting blood glucose |
FBS | Fetal bovine serum |
GSH-Px | Glutathione peroxidase |
GSH | Glutathione |
GST | Glutathione-S-transferase |
H2O2 | Hydrogen peroxide |
HO-1 | Heme-oxygenase 1 |
IDF | International Diabetes Federation |
I.P. | Intraperitoneal |
ISCT | International Society for Cellular Therapy |
LPO | Lipid peroxides |
MDA | Malondialdehyde |
MSCs | Mesenchymal stem cells |
OS | Oxidative stress |
PBS | Phosphate-buffered saline |
ROS | Reactive oxygen species |
SEM | Standard error of mean |
SOD | Superoxide dismutase |
SPSS | Statistical Package for Social Scientists |
STZ | Streptozotocin |
T1DM | Type 1 diabetes mellitus |
TAC | Total antioxidant capacity |
TB | Total bilirubin |
TP | Total proteins |
XO | Xanthine oxidase |
Diabetes mellitus (DM) is a chronic metabolic and systemic disease the incidence of which is increasing globally at an alarming rate. Its current prevalence among 20–79-year-old adults is 9.3%, and is expected to rise by 10.2% by 2030 and 10.9% by 2045 (Liu et al., 2022). Insulin deficiency and chronic hyperglycemia are the hallmarks in young patients with type 1 diabetes mellitus (T1DM). This is due to the autoimmunity in the pancreatic islets resulting in β cell dysfunction and death. Further, T1DM counts for approximately 5% to 10% of diabetic patients (Mobasseri et al., 2020). In 2021, 8.4 million patients were diagnosed with TIDM worldwide of which 1.5 million were below 20 years of age, 5.4 million were between 20–59 years old, and 1.6 million were over 60 years old (Gregory et al., 2022). Moreover, it is an independent risk factor for many body complications and diseases, including cancer, blindness, stroke, lower limb amputation, and chronic kidney and coronary heart diseases. Additionally, it accounts for 11.3% of global deaths, placing a heavy burden on worldwide health (Erendor et al., 2021).
The current treatments have significant limitations and efficient pharmacological therapeutics for diabetes are not available till now. This is mainly due to the complicated, unclear etiopathogenesis and pathophysiologic mechanisms of the malady. Therefore, new approaches are required to optimize diabetes therapy. On these lines, stem cell therapy has shown significant promise for many disorders, including T1DM (Chen et al., 2020; Liu et al., 2022). Stem cell therapy has highlighted a promising medicinal outcome in the last decade concerning the 3Rs (Replacement, Regeneration, and Repair) for repairing and replacing damaged organs (van Pham et al., 2016; Dang et al., 2017). Therefore, stem cell administration could be an excellent platform for DM treatment because of the ability to slow down the disease progression besides alleviating its long-term tissue complications. This could be attributed to the high self-renewing potential, low immunogenicity, easy sourcing, and multi-differentiating capacity of stem cells (Liu et al., 2022). Their primary therapeutic mechanism of these cells relies on their differentiation ability to replace damaged cells and tissues. They can repair and even regenerate damaged tissues and organs by increasing angiogenesis, regulating the remodeling of the extracellular matrix, and promoting wound closure acceleration. Moreover, in one study, MSCs could activate the neighboring cells of the microenvironment, enhancing the process of tissue repair via secreting various stimulant factors (Dang et al., 2017). Of note, adipose tissue is considered one of the richest of sources of MSCs. Hence, adipose-derived stem cells (AD-MSCs) are most often used for regenerative therapies due to the ease of obtaining and culturing them, wide source availability, and little suffering associated (Wang et al., 2020).
A significant relationship between diabetes and liver damage has been confirmed by many numerous previous research articles (Sirasanagandla et al., 2013; Xie et al., 2014; Muruganathan et al., 2017). In DM, hepatic glucose release dysregulation contributes significantly to these pathophysiological conditions. Chronic or acute liver disease can also aggravate insulin resistance with marked disturbances in the insulin physiological effects on hepatocytes. Further, insulin resistance was considered an independent risk factor for liver injury development in one report (Schattenberg and Schuchmann, 2009). Hepatic injury induced by diabetes is associated with several biochemical abnormalities related to the release of specific intracellular enzymes into circulation like AST, ALT, ALP, γ-GT, and LDH. The measurements of their levels are considered as useful quantitative markers for the hepatocellular damage extent (Sirasanagandla et al., 2013). Muruganathan et al. (2017) showed that subchronic exposure of rats to STZ increased the activities of AST and ALT by more than 4 and 6 times, respectively than their normal values. Moreover, Xie et al. (2014) stated that in STZ diabetic rats there was a marked increase in serum AST, ALT, and γ-GT levels due to damage occurring in the liver tissue as a result of hyperglycemia. Furthermore, injection of MSCs was found to initiate the regeneration of endogenous hepatocytes given their characteristic differentiation and self-renewal abilities that resulted in significant liver function amelioration (Kumar et al., 2011).
The kidneys have a major role in glucose homeostasis by regulating glucose utilization, gluconeogenesis, and glucose reabsorption. The kidneys consume nearly 10% of the total glucose utilized by the body, where nearly 20%–25% of glucose liberated into the general circulation occurs through gluconeogenesis from the kidneys (Zoidis and Papamikos, 2016). In fact, the kidneys remove metabolic wastes such as ions, creatinine, and urea and optimally maintain the chemical composition of body fluids. According to a report, the metabolite concentration increase in diabetic untreated rats may suggest renal damage associated with uncontrolled DM (Hamza et al., 2016). Of note, hyperglycemia could result in diabetic nephropathy (DN) and lead to severe kidney dysfunction (Rogal et al., 2019). Many studies also reported a marked incline in blood urea nitrogen (BUN), serum uric acid, and creatinine in STZ-diabetic rats compared with control (Hamza et al., 2016; Jiang et al., 2016). Interestingly, MSCs were suggested as an attractive candidate for renal repair given that nephrons are of mesenchymal origin (Hamza et al., 2016). In this line, histopathological analysis in many reports demonstrated that treatment of diabetic animals with MSCs restored the organizational structure of the kidney, which indicated a significant renal functional improvement. This was evidenced in the marked lowering of serum creatinine, BUN, and uric acid levels (Davey et al., 2014; Zhou et al., 2016; El-Kholy et al., 2018).
Unfortunately, several studies proposed that abnormal insulin activity and the hyperglycemic environment negatively affected MSCs (Suzuki and Shults, 2019; El-Sawah et al., 2021b). This might occur due to the weakening of their differentiation potential into β-cells and losing their proliferative potential, increasing their senescence and apoptosis (Kornienko et al., 2019). While low levels of reactive oxygen species (ROS) are needed physiologically for the self-renewal ability maintenance of stem cells, increased ROS production can initiate damage to mitochondrial DNA, produce multiple chromosomal aberrations, and cause stem cell differentiation defection (Domingues et al., 2019). Of note, a significant oxidative stress (OS) reduction has been reported following antioxidant-upregulated MSC delivery, resulting in a significant alleviation in systemic body inflammation (Skubis-Sikora et al., 2020). However, there are limited research reports on the GSH/GSSG ratio. Further, MSCs have been suggested as strong candidates for OS reduction, which was believed to be the leading cause of most diabetic complications. This is by delivering intracellular antioxidants intraperitoneally to the reserve of the adipose tissue and other adipose tissue-rich viscera (e.g., kidney and liver) (Domingues et al., 2019; Skubis-Sikora et al., 2020).
Many reports have highlighted antioxidant-MSC co-administration over the past years. In this context, stem cell activities might be enhanced by mitigating OS through the neutralization of free radicals coupled with increased antioxidant activities. Further, stem cell survival can be improved by preserving their genomic stability, enhancing their adhesion to culture media, and expanding their differentiation and potency. Furthermore, such a strategy increased neurogenesis in neurodegenerative disorders and ameliorated diabetic hepato-renal dysfunction, improving infarcted myocardial tissue regeneration in a report. Additionally, different antioxidants (e.g., melatonin and tempol) were investigated in maintaining the viability and differentiation capacities of stem cells (Orciani et al., 2015; Shaban et al., 2017; Kornienko et al., 2019).
As far as we know, our work is the first-time approach to use N, N′-diphenyl-1,4-phenylenediamine (DPPD) as a synthetic antioxidant in amplifying the therapeutic capacity of AD-MSCs in DM management. Given its color and stability, DPPD is the most frequently potent antioxidant used in oils, and rubber, especially in tire industries. In the medical field, DPPD is used as an intracellular antioxidant that can (1) affect the efficiency of the microsomal enzyme system, which is involved in the metabolism of the foreign compounds, (2) increase the lipid-soluble antioxidant pool, (3) inhibit lipid peroxidation and nephrotoxicity, (4) inhibit collagen deposition, and (5) prevent histopathological damage. Notably, the antioxidant capability of DPPD was attributed to its hydrogen donation capability to different radical species, resulting in an obvious reduction in the generation of ROS in tissues, which lowered tissue cell apoptosis (Kawai et al., 2009; Omar et al., 2012; Nabil et al., 2020). Given these findings, our work explores the ameliorating effects of DPPD antioxidant supplementation to the injected AD-MSCs on enhancing renal and hepatic complications in diabetic rats.
Streptozotocin (STZ, ≥99.5%), DPPD (Product number 292265, ≥99.8%), and culture media components for AD-MSC culture were obtained from Sigma Aldrich (USA). All assessed parameters including renal and hepatic antioxidants [heme-oxygenase 1 (HO-1), GSH, total antioxidant capacity (TAC), CAT, SOD, and GST], OS markers [MDA, H2O2, AGEs, and xanthine oxidase (XO)], total serum proteins, albumin, globulins, liver function markers [γ-GT, ALT, AST, ALP and total bilirubin (TB)], kidney function markers (creatinine, uric acid, and urea) were performed using commercial Biodiagnostic Co., Egypt kits according to the suppliers’ instructions. The cell G0/G1 assessment (%) was performed using a FACS caliber flow cytometer (Becton Dickinson, Sunnyvale, CA, USA) (Dean and Jett, 1974).
Isolation, preparation and characterization of adipose-derived mesenchymal stem cells
The techniques entailed were carried out as described earlier by Chen et al. (2020). Briefly, allograft AD-MSCs were lipoaspirated from subcutaneous adipose tissues of 6–8 weeks-old male Wistar rats, digested by collagenase solution (0.075%), then centrifuged for 15 min at 1000 × g. Cells were collected as a pellet and placed in Dulbecco’s Modified Eagle Medium (DMEM) and incubated overnight at room temperature. The cells were used after undergoing 3–5 passages. Finally, the stemness of the cultured cells was confirmed with flow cytometric analysis of select positive and negative surface markers using antibody kits (Sigma Aldrich Co., USA) in a FACS Caliber flow cytometer (Becton Dickinson, USA) (Wang et al., 2020). The fluorescein isothiocyanate (FITC) fluorochrome was directly linked to the primary antibody.
Experimental animal maintenance and grouping
The experiments were conducted on 36 albino male rats (Wistar) weighing approximately 100–120 g. The animals were acclimatized for 2 weeks and kept in polypropylene cages under standard laboratory conditions (12 h light/12 dark cycle, 25 ± 2°C temperature and a relative humidity of 45 ± 5%) where access to tap water and food pellets was ad libitum. STZ was used to induce diabetes, which was confirmed three days following induction by measuring blood glucose samples obtained from the tail vein. Only rats with fasting blood glucose (FBG) above 250 mg/kg were considered diabetic (Kodidela et al., 2020). We divided six groups of rats (6 rats in each group) and treated them as follows: Control group (intraperitoneal (i.p.) single dose of sodium citrate buffer, pH 4.5, once), DPPD group (i.p. single dose of DPPD, 250 mg/kg, daily), Diabetic (D) untreated group (i.p STZ single dose, 45 mg/kg, once), Diabetic treated groups with either DPPD or AD-MSCs (1 × 106 cell/rat, i.v., once) or both. The treatment continued for four weeks after confirming the diabetes induction. The study followed the instructions of the Animal Ethics Committee of the Faculty of Science, Suez Canal University, Ismailia, Egypt (REC 112/2022).
Sample collection and processing
Rats were anesthetized using pentobarbital and sacrificed. The collection of blood samples was carried out through cardiac puncture. The serum samples were carefully separated by centrifugation for 15 min at 1000 × g and stored at −20°C for biochemical estimations. The kidney and liver specimens were collected, weighed, and homogenized in cold distilled water into a 10% (w/v) homogenate, and preserved at −20°C for subsequent biochemical analysis.
For data evaluation, we applied analysis of variance (ANOVA) followed by post hoc Tukey multiple range tests, using Statistical Package for the Social Sciences (SPSS/21 software version) for Windows. Data were represented as the Mean ± standard error (SE), where n = 6. p ≤ 0.05 was set as the minimum significance level between different groups.
The phenotype of AD-MSCs was confirmed via flow cytometric characterization in accordance with the minimum criteria of the International Society for Cellular Therapy (ISCT). The AD-MSCs showed positive (high) expressions of CD73, CD90, and CD105 surface markers (Figs. 1A–1C, respectively) while exhibiting a negative expression of CD11b, CD34, and CD45 surface markers (Figs. 2A–2C, respectively).
Figure 1: Flow cytometric characterization of positive cell surface markers (CD 73: 95.1%, CD 90: 97.6%, CD 105: 96.2%) of adipose-derived mesenchymal stem cells (AD-MSCs).
Figure 2: Flow cytometric characterization of negative cell surface markers (CD 11b: 1.81%, CD 34: 2.1%, CD 45: 4.8%) of adipose-derived mesenchymal stem cells (AD-MSCs).
Furthermore, we observed a marked elevation in glucose and HbA1c levels coupled with a significant decline in serum insulin levels in diabetic rats compared to the control group (Suppl. Table S1). All measured parameters of the three treated diabetic groups showed an obvious improvement compared to diabetic untreated rats. Of note, among all these treated groups, the AD-MSCs+DPPD combination documented the highest hypoglycemic potentiality, as described in a previous report (Shaaban et al., 2023).
Further, significant elevations in both renal and hepatic OS markers (MDA, H2O2, AGEs, and XO) levels were observed along with a substantial decrease in antioxidant levels (GSH, SOD, CAT, GST, TAC, and HO-1) and the G0/G1 (%) in diabetic rats compared with the control rats (Figs. 3A–3C, 4A–4C, Suppl. Tables S2 and S3). Further, treatment of diabetic rats with either AD-MSCs or DPPD or both exhibited a marked decline in the oxidative status, accompanied by a marked increase in antioxidant levels and viable cell number compared to diabetic untreated rats. The greatest antioxidant potential was recorded in the diabetic rats treated with the AD-MSCs+DPPD combination.
Figure 3: Analysis of the renal oxidative stress (A), antioxidant markers levels (B), and viable cells (G0/G1 in %) (C). Values are expressed as ± SEM (n = 6). a,b&c are significant differences (p ≤ 0.05) compared to control, diabetic untreated, and DPPD-diabetic treated groups, respectively. MDA: Malondialdehyde, XO: Xanthine oxidase, GSH: Glutathione reductase, HO-1: Heme-Oxygenase 1, G0/G1: Growth phases, H2O2: Hydrogen peroxide, SOD: Superoxide dismutase, GST: Glutathione-s-transferase, TAC: Total antioxidant capacity, CAT: Catalase, AGEs: Advanced glycation end products.
Figure 4: Analysis of hepatic oxidative stress (A), antioxidant markers levels (B), and viable cells (G0/G1 in %) (C). Values are expressed as ± SEM (n = 6). a,b&c are significant differences (p ≤ 0.05) compared to control, diabetic untreated, and DPPD-diabetic treated groups, respectively. MDA: Malondialdehyde, XO: Xanthine oxidase, GSH: Glutathione reductase, HO-1: Heme-Oxygenase 1, G0/G1: Growth phases, H2O2: Hydrogen peroxide, SOD: Superoxide dismutase, GST: Glutathione-s-transferase, TAC: Total antioxidant capacity, CAT: Catalase, AGEs: Advanced glycation end products.
We also found that diabetic rats exhibited an evident elevation in serum kidney markers (uric acid, urea, and creatinine levels) and liver function markers (AST, ALT, ALP, γ-GT, and total bilirubin levels), as shown in Figs. 5 and 6, Suppl. Tables S4 and S5. On the other hand, a significant decline in the protein profile (total proteins, albumin, and globulins) was detected in diabetic rats compared to the control. Further, diabetic rats injected with either DPPD or AD-MSCs or their combination showed a marked improvement in these parameters compared to diabetic untreated rats. Interestingly, the co-administration of AD-MSCs and DPPD showed the most significant enhancement in hepato-renal functions.
Figure 5: Levels of serum creatinine, uric acid, total bilirubin, total proteins, albumin, and globulins. Values expressed as ± SEM (n = 6). a,b&c are significant differences (p ≤ 0.05) compared to control, diabetic untreated, and DPPD-diabetic treated groups, respectively.
Figure 6: Levels of serum urea, AST, ALT, γ-GT, and ALP. Values expressed as ± SEM (n = 6). a,b&c are significant differences (p ≤ 0.05) compared to control, diabetic untreated, and DPPD-diabetic treated groups, respectively. AST: Aspartate transferase, ALT: Alanine transferase, γ-GT: Gamma-glutamyltransferase, ALP: Alkaline phosphatase.
Evidence suggests that MSCs are very sensitive to ROS and OS, which could negatively influence their development and impair their abilities of self-renewal, proliferation, differentiation, and direct reprogramming in target cells. Additionally, antioxidant co-administration could stimulate the proliferation of MSCs (Orciani et al., 2015).
Oxidative stress and antioxidants
Many studies have shown that injection of MSCs coupled with antioxidants administration might be helpful in supporting therapeutic strategies of cell reprogramming. This could be by minimizing OS and enhancing the cell potency, survival, or differentiation to open a new use of antioxidants in biomedicine (Shaban et al., 2017; Suzuki and Shults, 2019). According to a report, the proliferation of AD-MSCs was augmented due to antioxidant co-administration. This was confirmed by the significant rise in the number of cells in the S phase. Furthermore, the functions of the antioxidants were suggested to (1) initiate the secretion of multiple growth factors, including insulin-like growth factor (IGF-1) and hepatocyte growth factor (HGF), (2) increase the expression of many endogenous antioxidants during adipogenesis, like GSH, CAT, SOD, and HO-1, (3) increase the expression of various stemness genes (CDC2, CDK2 and CDK4) and (5) enhance the differentiation potential of AD-MSCs (Sun et al., 2013).
On these lines, Wang et al. (2016) found that pretreatment of AD-MSCs with either 0.2 mM of L-Ascorbic acid 2-phosphate (AAP, an oxidation-resistant derivative of ascorbic acid) or 3 mM of N-acetyl-L-cysteine (NAC, a prodrug/precursor of the biological antioxidant glutathione) for 20 h suppressed AGEs-induced apoptosis. This was through a microRNA-dependent mechanism, which is a key modulator of intracellular apoptotic signaling. This finding was consistent with an earlier study by Li et al. (2015), who reported a synergistic protective effect of NAC and AAP against apoptosis and necroptosis of human MSCs treated with H2O2.
Additionally, the ability of either stem cells or the DPPD antioxidant in inhibiting lipid peroxidation and their potential role as free radical scavengers was documented in other reports. For example, MSCs injection significantly decreased the levels of increased ROS, XO, and MDA levels. Further, the mRNA expression of various antioxidants was increased such as GSH, GSH-Px-1, GSH-Px-3 and GSH-Px-4, HO-1, CAT, SOD-1, and SOD-3, and TAC in the pancreas, liver, kidney, and heart tissues of STZ-diabetic rats compared to diabetic untreated rats (Abdel Fattah et al., 2020; Aminzadeh et al., 2020; El-Sawah et al., 2021a, 2021b).
Further, Ahmed et al. (2011) indicated that DPPD is a potent antioxidant against testicular oxidative damage induced by cisplatin (CP) as evidenced by the significant decline in the levels of total peroxides, lipid peroxides (LPO), and O2.− in testes of rats treated with DPPD. This was accompanied by a substantial increase in the activity of some the antioxidant enzymes like GSH, GST, CAT, and SOD. Of note, administering DPPD before the CP injection enhanced the testicular histology and decreased the number of apoptotic cells. Similar results were also recorded in the plasma, testes, liver, and kidney in other studies (Omar et al., 2011; Omar et al., 2012). According to Nabil et al. (2020), DPPD also ameliorated the OS status induced by HgCl2 in rat kidney and liver tissues as evidenced by the decreased MDA level coupled with a significant elevation in antioxidant levels (GSH, CAT, and SOD). In our work, the profiles of the renal and hepatic antioxidants were strongly suggested to be the main reason for the amelioration of kidney and liver dysfunctions.
Several renal cell types are considered insulin sensitive and elevated blood glucose levels can result in severe kidney dysfunction called diabetic nephropathy (DN) (Rogal et al., 2019). Interestingly, Zahran et al. (2016) found that cisplatin-induced nephrotoxicity was greatly ameliorated following a single treatment of MSCs or DPPD. The treatment exhibited a remarkable anti-fibrotic effect as seen by the decreased levels of MDA, creatinine, and BUN and histopathological examination. Furthermore, MSCs were able to document homing and repair the injured kidney through (1) anti-inflammatory cytokine secretion like TGFβ1 to limit apoptosis and dampen the inflammatory response, (2) differentiation into tubular epithelial cells, (3) lipid peroxidation reduction, and (4) marked increase in antioxidant levels. In addition, many reports suggest that the antioxidant potential of DPPD could inhibit renal interstitial fibrosis and nephrotoxicity induced by either cisplatin (Kawai et al., 2009) or HgCl2 (Zahran et al., 2014; Nabil et al., 2020). This was by targeting the collagen deposition and the hepatorenal fibrosis progression, either directly through renal fibrogenesis or indirectly via decreasing ROS production. This was evidenced by the significant lowering in serum levels of uric acid, urea, and creatinine indicating the promising role of DPPD in alleviating renal dysfunction. On the other hand, El-Sawah et al. (2022) reported a significant renal protective effect of AD-MSCs in STZ-diabetic rats, evidenced by the marked decline in many serum renal functions (uric acid, urea, and creatinine).
This study also strongly confirmed the known and significant relationship between DM and liver damage. Further, we also noted the capacity of AD-MSC and DPPD co-administration to restore normal hepatic functions. Further, AD-MSCs transplantation could mitigate hyperglycemia in diabetic rats (Liao et al., 2017; El-Sawah et al., 2022) and mice (Ma et al., 2014) in addition to restoring the normal function of the diseased liver and protecting against liver fibrosis. This was confirmed by the significant reduction in the serum levels of TB, ALT, AST, and ALP along with significantly reduced histological liver tissue lesions. Recently, El-Sawah et al. (2022) reported a significant hepato-protective effect of AD-MSCs in STZ-diabetic rats, evidenced by the marked decline in many serum liver functions (ALT, AST, ALP, and bilirubin). This suggests AD-MSC-based treatment as a promising therapeutic protocol for diabetic patients with liver fibrosis. Further, Nabil et al. (2020) reported that serum ALT, AST, and ALP levels were significantly decreased in HgCl2-injected rats treated with DPPD, indicating the enhancing role of DPPD in alleviating the hepatic dysfunction due to HgCl2 administration.
The co-administration of AD-MSCs and DPPD showed a significant hepato-renal protective role in T1DM, evidenced by the restoration of appropriate protein metabolism balance and the enhanced liver and kidney functions of diabetic rats. This could be attributed to their capacity to reduce OS and increase both renal and hepatic antioxidant contents. These results were reflected by the substantial decline in the renal and hepatic OS status coupled with the marked increase in the antioxidant levels of the diabetic rats treated with either DPPD or AD-MSCs or their co-administered injection compared to the diabetic untreated rats. These aspects were suggested to be the predominant cause of the amelioration of the kidney and liver function dysfunction. Further, the co-administration of DPPD and AD-MSCs showed relatively superior effects in enhancing hepatorenal functions. This indicates the beneficial role of DPPD supplementation in increasing the therapeutic potential of AD-MSCs. Our data may create a new innovative solution to mitigate diabetic complications, especially hepatorenal issues.
Acknowledgement: None.
Funding Statement: The authors acknowledge the Deanship of Scientific Research, Vice Presidency for Graduate Studies and Scientific Research at King Faisal University, Saudi Arabia, for financial support under the annual funding track (Grant 3730).
Author Contributions: The authors confirm their contribution to the paper as follows: SGE, SHQ, HMA, SAA, IJ, and EF conceived and designed the study. Experiments and lab work were done by SGE, SS, MKD, RM, and ES; data tabulating and acquisition, searching for literature and preparing the first draft of the manuscript were performed by SGE, MKD, RM and ES. All authors reviewed the results and approved the final version of the manuscript.
Availability of Data and Materials: All data generated or analyzed during this study are included in this published article (and its supplementary information files).
Ethics Approval: The study followed the instructions of the Animal Ethics Committee of the Faculty of Science, Suez Canal University, Ismailia, Egypt (REC 112/2022).
Conflicts of Interest: The authors declare that they have no conflicts of interest to report regarding the present study.
Supplementary Materials: The supplementary materials are available online at DOI: 10.32604/biocell.2023.030680.
References
Abdel Fattah S, Waly H, Abou El-Enein B, Kamel A, Labib H (2020). Mesenchymal stem cells versus curcumin in enhancing the alterations in the cerebellar cortex of streptozotocin-induced diabetic albino rats. The role of GFAP, PLC and α-synuclein. Journal of Chemical Neuroanatomy 109: 101842. https://doi.org/10.1016/j.jchemneu.2020.101842 [Google Scholar] [PubMed] [CrossRef]
Ahmed EA, Omar HM, Abd Elghaffar SK, Ragb SMM, Nasser AY (2011). The antioxidant activity of vitamin C, DPPD and L-cysteine against cisplatin-induced testicular oxidative damage in rats. Food Chemical Toxicology 49: 1115–1121. https://doi.org/10.1016/j.fct.2011.02.002 [Google Scholar] [PubMed] [CrossRef]
Aminzadeh A, Maroof NT, Mehrabani M, Juybari KP, Sharifi AM (2020). Investigating the alterations of oxidative stress status, antioxidant defense mechanisms, MAP kinase and mitochondrial apoptotic pathway in adipose-derived mesenchymal stem cells from STZ diabetic rats. Cell 22: 38–48. https://doi.org/10.22074/cellj.2020.6958 [Google Scholar] [PubMed] [CrossRef]
Chen TS, Lai PF, Kuo CH, Day CH, Chen RJ et al. (2020). Resveratrol enhances therapeutic effect on pancreatic regeneration in diabetes mellitus rats receiving autologous transplantation of adipose-derived stem cells. Chinese Journal of Physiology 63: 122–127. https://doi.org/10.4103/CJP.CJP_3_20 [Google Scholar] [PubMed] [CrossRef]
Dang L, Phan N, Truong K (2017). Mesenchymal stem cells for diabetes mellitus treatment: New advances. Biomedical Research and Therapy 4: 1062–1081. https://doi.org/10.15419/bmrat.v4i1.144 [Google Scholar] [CrossRef]
Davey GC, Patil SP, O’Loughlin A, O’Brien T (2014). Mesenchymal stem cell-based treatment for microvascular and secondary complications of Diabetes mellitus. Frontiers in Endocrinology 5: 1–16. https://doi.org/10.3389/fendo.2014.00086 [Google Scholar] [PubMed] [CrossRef]
Dean PN, Jett JH (1974). Mathematical analysis of DNA distributions derived from flow microfluorometry. Journal of Cell Biology 60: 523–524. https://doi.org/10.1083/jcb.60.2.523 [Google Scholar] [PubMed] [CrossRef]
Domingues CC, Kundu N, Kropotova Y, Ahmadi N, Sen S (2019). Antioxidant-upregulated mesenchymal stem cells reduce inflammation and improve fatty liver disease in diet-induced obesity. Stem Cell Research & Therapy 10: 280. https://doi.org/10.1186/s13287-019-1393-8 [Google Scholar] [PubMed] [CrossRef]
El-Kholy WM, Hussein RH, Khalil DY (2018). Assessment of the potential ameliorating effects of BM-MSCs or insulin on the altered metabolic status of pancreas, liver and kidney in STZ-diabetic rats. International Journal of Advanced Research 6: 18–34. https://doi.org/10.21474/IJAR01/7487 [Google Scholar] [CrossRef]
El-Sawah SG, Althobaiti F, Aldhahrani A, Fayad E, Amen RM, Shabana E, El-Hallous EI, Rashwan HM (2021b). Investigation of the antioxidant defensive role of both AD-MSCs and BM-MSCs in modulating the alteration in the oxidative stress status in various STZ-diabetic rats’ tissues. BIOCELL 9: 1561–1568. https://doi.org/10.32604/biocell.2021.016869 [Google Scholar] [CrossRef]
El-Sawah SG, Althobaiti F, Aldhahranil A, Fayad E, Abdel-Dayem MA, Amen RM, Shabana E, El-Hallous EI, Rashwan HM (2022). AD-MSCs and BM-MSCs ameliorating effects on the metabolic and hepato-renal abnormalities in diabetic rats. Saudi Journal of Biological Sciences 29: 1053–1060. https://doi.org/10.1016/j.sjbs.2021.09.067 [Google Scholar] [PubMed] [CrossRef]
El-Sawah SG, Althobaiti F, Rashwan HM, Aldhahranil A, Abdel-Dayem MA, Fayad E, Amen RM, Shabana E, El-Hallous EI (2021a). Anti-inflammatory and antioxidant potential capacities of AD-MSCs and BM-MSCs in suppressing pancreatic β-cells auto-immunity and apoptosis in rats with T1DM induced model. BIOCELL 11. https://doi.org/10.32604/biocell.2021 [Google Scholar] [CrossRef]
Erendor F, EmreEksi E, Sahin EO, Balci MK, Griffith TS, Sanlioglu S (2021). Lentivirus mediated pancreatic beta-cell-specific insulin gene therapy for STZ-induced diabetes. Molecular Therapy 29: 149–161. https://doi.org/10.1016/j.ymthe.2020.10.025 [Google Scholar] [PubMed] [CrossRef]
Gregory GA, Robinson TIG, Linklater SE, Wang F, Colagiuri S et al. (2022). Global incidence, prevalence, and mortality of type 1 diabetes in 2021 with projection to 2040: A modelling study. The Lancet Diabetes & Endocrinology 10: 741–760. https://doi.org/10.1016/S2213-8587(22)00218-2 [Google Scholar] [PubMed] [CrossRef]
Hamza AH, Al-Bishri WM, Damiati LA, Ahmed HH (2016). Mesenchymal stem cells: A future experimental exploration for recession of diabetic nephropathy. Renal Failure 39: 67–76. https://doi.org/10.1080/0886022X.2016.1244080 [Google Scholar] [PubMed] [CrossRef]
Jiang Z, Liu Y, Niu X, Yin J, Hu B, Guo S, Fan Y, Wang Y (2016). Exosomes secreted by human urine-derived stem cells could prevent kidney complications from type I diabetes in rats. Stem Cell Research and Therapy 7: 24–37. https://doi.org/10.1186/s13287-016-0287-2 [Google Scholar] [PubMed] [CrossRef]
Kawai Y, Satoh T, Hibi D, Ohno Y, Kohda Y, Miura K, Gemba M (2009). The effect of antioxidant on development of fibrosis by cisplatin in rats. Journal of Pharmacological Sciences 111: 433–439. https://doi.org/10.1254/jphs.09185FP [Google Scholar] [PubMed] [CrossRef]
Kodidela S, BegumShaik F, Chinta V, AliMohammad S, Pasala C, Mittameedi S, Maddu N, Wudayagiri R, Nallanchakravarthula V (2020). Possible ameliorative role of green tea on chronic alcohol mediated renal toxicity of STZ-induced diabetic rats. Clinical Nutrition Experimental 34: 1–25. https://doi.org/10.1016/j.yclnex.2020.09.001 [Google Scholar] [CrossRef]
Kornienko JS, Smirnova IS, Pugovkina NA, Ivanova JS, Shilina MA, et al. (2019). High doses of synthetic antioxidants induce premature senescence in cultivated mesenchymal stem cells. Scientific Reports 9: 1296. https://doi.org/10.1038/s41598-018-37972-y [Google Scholar] [PubMed] [CrossRef]
Kumar A, Pati NT, Sarin SK (2011). Use of stem cells for liver diseases-current scenario. Journal of Clinical Experimental Hepatology 1: 17–26. https://doi.org/10.1016/S0973-6883(11)60114-X [Google Scholar] [PubMed] [CrossRef]
Li CJ, Sun LY, Pang CY (2015). Synergistic protection of N-acetylcysteine and ascorbic acid 2-phosphate on human mesenchymal stem cells against mitoptosis, necroptosis and apoptosis. Scientific Reports 24: 98–109. https://doi.org/10.1038/srep09819 [Google Scholar] [PubMed] [CrossRef]
Liao N, Zheng Y, Xie H, Zhao B, Zeng Y, Liu X, Liu J (2017). Adipose tissue-derived stem cells ameliorate hyperglycemia, insulin resistance and liver fibrosis in the type 2 diabetic rats. Stem Cell Research & Therapy 8: 10949. https://doi.org/10.1186/s13287-017-0743-7 [Google Scholar] [PubMed] [CrossRef]
Liu J, Zhang Y, Tian Y, Huang W, Tong N, Fu X (2022). Integrative biology of extracellular vesicles in diabetes mellitus and diabetic complications. Theranostics 12: 1342–1372. https://doi.org/10.7150/thno.65778 [Google Scholar] [PubMed] [CrossRef]
Ma S, Xie N, Li W, Yuan B, Shi Y, Wang Y (2014). Immunobiology of mesenchymal stem cells. Cell Death and Differentiation 21: 216–225. https://doi.org/10.1038/cdd.2013.158 [Google Scholar] [PubMed] [CrossRef]
Mobasseri M, Shirmohammadi M, Amiri T, Vahed N, Hosseini Fard H, Ghojazadeh M (2020). Prevalence and incidence of type 1 diabetes in the world: A systematic review and meta-analysis. Health Promotion Perspective 10: 98–115. https://doi.org/10.34172/hpp.2020.18 [Google Scholar] [PubMed] [CrossRef]
Muruganathan U, Srinivasan S, Vinothkumar V (2017). Antidiabetogenic efficiency of menthol, improves glucose homeostasis and attenuates pancreatic β-cell apoptosis in streptozotocin-nicotinamide induced experimental rats through ameliorating glucose metabolic enzymes. Biomedicine and Pharmacotherapy 92: 229–239. https://doi.org/10.1016/j.biopha.2017.05.068 [Google Scholar] [PubMed] [CrossRef]
Nabil A, Elshemy MM, Asem M, Gomaa HF (2020). Protective effect of DPPD on mercury chloride-induced hepatorenal toxicity in rats. Journal of Toxicology 2020: 1–7. https://doi.org/10.1155/2020/4127284 [Google Scholar] [PubMed] [CrossRef]
Omar HM, Ahmed E, Sarry A (2011) The antioxidant activity of vitamin C, DPPD, and L-cysteine against cisplatin-induced testicular oxidative stress in rats. Food and Cosmetics Toxicology 49: 1115–1121. [Google Scholar]
Omar HM, Ahmed E, Sarry A, Mohammed S, Nasser AY (2012). Hepatoprotective effects of vitamin C, DPPD and L-cysteine against cisplatin-induced oxidative stress in male rats. Journal of Biology and Earth Science 2: B28–B36. [Google Scholar]
Orciani M, Davis S, Appolloni G, Lazzarini R, Mattioli-Belmonte M, Ricciuti RA, Boscaro M, di Primio R, Arnaldi G (2015). Isolation and characterization of progenitor mesenchymal cells in human pituitary tumors. Cancer Gene Therapy 22: 9–16. https://doi.org/10.1038/cgt.2014.63 [Google Scholar] [PubMed] [CrossRef]
Rogal J, Zbinden A, Schenke-Layland K, Loskill B (2019). Stem-cell based organ-on-a-chip models for diabetes research. Advanced Drug Delivery Reviews 140: 101–128. https://doi.org/10.1016/j.addr.2018.10.010 [Google Scholar] [PubMed] [CrossRef]
Schattenberg JM, Schuchmann M (2009). Diabetes and apoptosis: Liver. Apoptosis 14: 1459–1469. https://doi.org/10.1007/s10495-009-0366-2 [Google Scholar] [PubMed] [CrossRef]
Shaaban S, El-Shamy H, Gouda M, Darwish MK, Abd El-Lateef HM, Khalaf MM, El-Hallous EI, Radwan KH, Rashwan HM, El-Sawah SG (2023). N,N-diphenyl-1,4-phenylenediamine antioxidant’s potential role in enhancing the pancreatic antioxidant, immunomodulatory, and anti-apoptotic therapeutic capabilities of adipose-derived stem cells in type I diabetic rats. Antioxidants 12: 58. https://doi.org/10.3390/antiox12010058 [Google Scholar] [PubMed] [CrossRef]
Shaban S, Wanees M, El-Husseny A, Abushouk AI, Salem AMA, Mamdouh M, Abdel-Daim MM (2017). Effects of antioxidant supplements on the survival and differentiation of stem cells. Oxidative Medicine and Cellular Longevity 2017: 1–16. https://doi.org/10.1155/2017/5032102 [Google Scholar] [PubMed] [CrossRef]
Sirasanagandla S, Babu Kasetti RB, Shaik AN, Natava R, Surtineni VP, Cirradur SR, Chippada A (2013). Antihyperglycemic and antihyperlipidemic activities of 2-(4-[(2-hydroxybenzyl) amino]-phenyl amino-methyl)-phenol in STZ induced diabetic rats. European Journal of Medicinal Chemistry 66: 400–406. https://doi.org/10.1016/j.ejmech.2013.05.014 [Google Scholar] [PubMed] [CrossRef]
Skubis-Sikora A, Sikora B, Witkowska A, Mazurek U, Gola J (2020). Osteogenesis of adipose-derived stem cells from patients with glucose metabolism disorders. Molecular Medicine 26: 67. https://doi.org/10.1186/s10020-020-00192-0 [Google Scholar] [PubMed] [CrossRef]
Sun LY, Pang CY, Li DK, Liao CH, Huang WC et al. (2013). Antioxidants cause rapid expansion of human adipose-derived mesenchymal stem cells via CDK and CDK inhibitor regulation. Journal of Biomedical Science 20: 53–60. https://doi.org/10.1186/1423-0127-20-53 [Google Scholar] [PubMed] [CrossRef]
Suzuki YJ, Shults NV (2019). Antioxidant regulation of cell reprogramming. Antioxidants 8: 323. https://doi.org/10.3390/antiox8080323 [Google Scholar] [PubMed] [CrossRef]
van Pham P, Vu NB, Phan NK (2016). Umbilical cord-derived stem cells (ModulatistTM) show strong immunomodulation capacity compared to adipose tissue-derived or bone marrow-derived mesenchymal stem cells. Biomedical Research and Therapy 3: 687–696. https://doi.org/10.7603/s40730-016-0029-1 [Google Scholar] [CrossRef]
Wang Z, Li H, Guo R, Wang Q, Zhang D (2016). Antioxidants inhibit advanced glycosylation end-product-induced apoptosis by downregulation of miR-223 in human adipose tissue-derived stem cells. Scientific Reports 6: 1–11. https://doi.org/10.1038/srep23021 [Google Scholar] [PubMed] [CrossRef]
Wang L, Shi S, Bai R, Wang Y, Guo Z, Li D (2020). Biological properties of bone marrow stem cells and adipose-derived stem cells derived from T2DM rats: A comparative study. Cell & Bioscience 10: 102. https://doi.org/10.1186/s13578-020-00465-5 [Google Scholar] [PubMed] [CrossRef]
Xie M, Chena D, Zhanga F, Willskyb GR, Cransc DC, Ding W (2014). Effects of vanadium (III, IV, V)-chlorodipicolinate on glycolysis and antioxidant status in the liver of STZ-induced diabetic rats. Journal of Inorganic Biochemistry 136: 47–56. https://doi.org/10.1016/j.jinorgbio.2014.03.011 [Google Scholar] [PubMed] [CrossRef]
Zahran F, El Ghareb M, Nabil A (2014). Bone marrow derived mesenchymal stem cells as a therapy for renal injury. International Journal of Advance Research 4: 11–16. [Google Scholar]
Zahran F, Nabil A, Karef A, Lotfy A, Mahmoud K, Hozayen WG, Sobh M (2016). Effect of antioxidants and mesenchymal stem cells on cisplatin induced renal fibrosis in rats. Stem Cell Research & Therapy 1: 150–158. https://doi.org/10.15406/jsrt.2016.01.00026 [Google Scholar] [CrossRef]
Zhou J, Zhang Z, Qian G (2016). Mesenchymal stem cells to treat diabetic neuropathy: A long and strenuous way from bench to the clinic. Cell Death Discovery 2: 16055. https://doi.org/10.1038/cddiscovery.2016.55 [Google Scholar] [PubMed] [CrossRef]
Zoidis E, Papamikos V (2016). Glucose: Metabolism and regulation. Encyclopedia of Food and Health 6: 233–238. https://doi.org/10.1016/B978-0-12-384947-2.00351-2 [Google Scholar] [CrossRef]
Supplementary Materials
TABLE S1. Levels of glucose and insulin
TABLE S2. Renal oxidative stress, antioxidant markers levels & viable cells (G0/G1)%
TABLE S3. Hepatic oxidative stress, antioxidant markers levels & viable cells (G0/G1)%
TABLE S4. Serum creatinine, urea, and uric acid levels
TABLE S5. Serum AST, ALT, ALP, γ-GT, total bilirubin, total proteins, albumin & globulins levels
Cite This Article
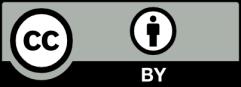