Open Access
VIEWPOINT
Expression profiles of circulating tRNA-derived small RNAs and their potential role in diabetes
1 Department of Ophthalmology, Shanghai Children’s Medical Center, School of Medicine, Shanghai Jiao Tong University, Shanghai, 200127, China
2 Department of Ophthalmology, Shanghai General Hospital, School of Medicine, Shanghai Jiao Tong University, Shanghai, 200080, China
3 Department of Endocrinology, Shanghai Ninth People’s Hospital, School of Medicine, Shanghai Jiao Tong University, Shanghai, 200011, China
4 Department of Cardiology, Shanghai Sixth People’s Hospital, School of Medicine, Shanghai Jiao Tong University, Shanghai, 200233, China
5 Department of Medical Oncology, Shanghai Pulmonary Hospital, School of Medicine, Tongji University, Shanghai, 200433, China
* Corresponding Author: WENCHENG ZHAO. Email:
# Contributed equally to this work
BIOCELL 2023, 47(7), 1645-1650. https://doi.org/10.32604/biocell.2023.029493
Received 22 February 2023; Accepted 20 March 2023; Issue published 21 June 2023
Abstract
Background: This work aimed to reveal the expression profiles of tRNA-derived small RNAs (tsRNAs) in diabetes. Methods: Thirty-five diabetes patients and thirty-three controls were enrolled. The serum samples of 4 diabetes patients and 4 controls were subjected to tRF and tiRNA polymerase chain reaction (PCR) Array analysis. Then quantitative PCR (qPCR) validation was performed on all the samples. Bioinformatics analyses were conducted to explore their functions. Results: We found 115 tsRNAs that significantly differed between the two groups. 3′tiR-080-ProTGG(mt) was selected for further qPCR validation in all participants, and it was significantly decreased in diabetes patients compared with controls. Bioinformatics analysis indicated that 3′tiR-080-ProTGG(mt) may play regulatory roles via the cyclic adenosine monophosphate (cAMP) signaling pathway in the pathogenesis and progression of diabetes. Conclusion: Hence, we report that circulating tsRNAs are dysregulated that could be involved in the pathogenesis of diabetes.Keywords
Diabetes mellitus has emerged as a major public health concern, both in developed and developing countries. It is estimated that 8.8% or 415 million people suffer from diabetes worldwide, and the number is expected to rise to 10.4% (642 million) by 2040 (Koye et al., 2018; Li et al., 2022). Diabetes is characterized by metabolic disorders related to hyperglycemia (American Diabetes Association, 2013). The chronic hyperglycemia of diabetes is associated with long-term damage, dysfunction, and failure of different organs. Long-term complications of diabetes include nephropathy leading to renal failure, retinopathy with vision loss, peripheral neuropathy with potential foot ulcers, and autonomic neuropathy causing gastrointestinal and cardiovascular symptoms. These are life-threatening, disabling, and costly (Papatheodorou et al., 2018; Zheng et al., 2018; Cole and Florez, 2020; Jin and Ma, 2021; Yan et al., 2021). Although tremendous progress has been made in the diagnosis and treatment of diabetes, the exact mechanism remains unclear and the current therapies still need improvement.
For decades, researchers have predominantly focused on non-coding RNAs such as long non-coding RNAs (lncRNAs), micro RNA (miRNAs), and piwi-interacting RNAs (piRNAs). However, few studies focused on transfer RNA (tRNA), which has long been recognized as an amino acids carrier to the ribosome. In recent years, next-generation sequencing revealed that the cleaved tRNA fragments are not randomly degraded products (Zhu et al., 2018), but they were involved in various important cellular processes. Therefore, these fragments were recognized as a novel category of non-coding RNAs, which has been named as tRNA-derived small RNAs (tsRNAs). tRNA fragments (tRFs) (14–30 nucleotides) and tRNA halves (30–45 nucleotides) are the two major types of tsRNA. They play important roles in the cellular stress response, epigenetic regulation, mRNA stability repression, translational inhibition, protein biosynthesis, and carcinogenesis (Maute et al., 2013; Babski et al., 2014; Shao et al., 2017; Guzzi et al., 2018; Zhu et al., 2018).
Despite the emerging interest in tRFs and tRNA halves, their biogenesis and functional mechanisms remain largely unknown. In this study, we aimed to reveal the expression profiles of tsRNAs in diabetes. We found that tRFs and tRNA halves were significantly different between diabetes patients and controls by Human tRF&tiRNA PCR Array, and we validated the results by qPCR. Finally, we investigated the role of tRFs and tRNA in the pathogenesis of diabetes based on bioinformatics analysis.
Patients with a diagnosis of diabetes and healthy volunteers as controls were enrolled in this study at the Shanghai General Hospital of Shanghai Jiao Tong University School of Medicine. Informed consent was obtained from all participants. The study protocol has been approved by the ethics committee of Shanghai Jiao Tong University School of Medicine. The inclusion criteria of the control group were: healthy volunteers aged 30–85 years, regardless of gender, having no diabetes. The inclusion criteria for the diabetes group were: patients aged 30–85 years, regardless of gender, who have been diagnosed with diabetes according to the American Diabetes Association (ADA) diagnostic criteria. The exclusion criteria were as follows: patients with malignant tumors; with hypertension or other systematic diseases; with active inflammation and infectious diseases; severe liver and kidney dysfunction; long-term use of glucocorticoids, antipsychotics, and other drugs that affect blood glucose.
Serum samples were thawed and then homogenized by centrifuging at 3000 g for 5 min to pellet debris and insoluble components. The supernatants were transferred to a new tube and RNA was isolated by TRIZOL-LS (Ambion, Life Technology, Carlsbad, CA, USA) according to the manufacturer’s instructions. Then, the rtStar™ tRF&tiRNA Pretreatment Kit (Arraystar Inc., Rockville, USA) was used for RNA sample pretreatment and rtStar™ First-Strand cDNA Synthesis Kit (3′ and 5′ adaptor) (Arraystar Inc.) was used for cDNA synthesis. Briefly, the pretreatment procedures included 3′-terminal deacylation, 3′-cP removal and 5′-P addition, and demethylation. The cDNA synthesis protocol included 3′adaptor ligation, hybridization of reverse transcription primer, 5′adaptor ligation, and the process of reverse transcription.
The cDNA obtained was mixed with Arraystar SYBR® Green qPCR Master Mix (ROX+) (Arraystar Inc.), and loaded on the nrStar™ Human tRF&tiRNA PCR Array (Arraystar Inc.). The PCR cycles were carried out on an ABI PRISM 7900 HT sequence detection system (Applied Biosystems, Waltham, Massachusetts, USA). Then the mixtures were incubated in a 384-well plate at 95°C for 10 min, following 40 cycles of 95°C for 10 s and 60°C for 1 min. All data were analyzed by the 2−ΔΔCt method and were normalized against the U6 control. The Ct values were averaged from three separate cDNA runs.
Validations by real-time polymerase chain reaction
Total RNA was isolated using TRIZOL-LS (Ambion) and rtStar™ tRF&tiRNA Pretreatment Kit (Arraystar Inc.). The RNA sample pretreatment was done using the rtStar™ First-Strand cDNA Synthesis Kit (3′ and 5′ adaptor) (Arraystar Inc.) was used for cDNA synthesis both according to the manufacturer’s instructions. Real time PCR assays were performed using Arraystar SYBR® Green Real-Time qPCR Master Mix (Arraystar Inc.) according to the manufacturer’s protocol. Data for relative quantification was normalized to U6 expression levels. The expression levels of tRF and tiRNA were calculated using the 2−ΔΔCt method for relative quantification. All the primers used in this work are listed as follows: 3′tiR-080-ProTGG(mt):
5′ CTACAGTCCGACGATCTTGGG3′ and 5′ GTGTGCTCTTCCGATCTGGT3′; U6: 5′GCTTCGGCAGCACATATACTAAAAT3′ and 5′CGCTTCACGAATTTGCGTGTCAT3′.
Gene ontology (GO) and kyoto encyclopedia of genes and genomes (KEGG) pathway analyses
The cellular component, biological process, and molecular function of target tRFs and tiRNAs and tiRNAs were explored through Gene Ontology (GO) (http://www.geneontology.org/). Further, the potential signaling pathways were investigated in the Kyoto Encyclopedia of Genes and Genomes (KEGG) database (http://www.genome.jp/kegg/).
Statistical analysis was conducted with SPSS software (version 19.0). Statistical significance was assessed using Student’s t-test, One-Way analysis of variance (ANOVA), and Mann–Whitney U tests. p < 0.05 was defined as statistically significant.
A total of 68 participants were included in this study, including 33 healthy controls and 35 diabetic patients. All subjects were aged from 37 to 88 years, the control group subjects were aged from 47 to 88 years, and the diabetic patients were aged from 37 to 87. In the control group, 16 were male, and 17 were female; in the diabetes group, 22 were male, and 13 were female. The detailed demographic and clinical data are presented in Table 1.
Altered expression of tRFs and tiRNAs in diabetic patients
In order to obtain an overview of the tRFs/tiRNAs profile in diabetes, we analyzed the serum sample of diabetic patients and controls using the Human tRF&tiRNA PCR Array. We found that 115 tRFs/tiRNAs showed differential expression in the control group and diabetes group. Of this, 112 tRFs/tiRNAs were downregulated in the diabetes group and 3 tRFs/tiRNAs were upregulated. The expression variations of tRFs/tiRNAs between the two groups are shown in Fig. 1.
Figure 1: Expression profiles of tRNA fragments (tRF) & tRNA-derived small RNAs (tsRNAs) in diabetic patients vs. controls. (A) Cluster analysis of tRFs/tiRNAs in diabetic patients (D1–D4) vs. controls (N1–N4). The green color represented high expression and the red color represented low expression. (B) Volcano plot showing differential expression between diabetic patients and controls. The green dots indicated significantly downregulated tsRNAs and the grey dots indicated the tsRNAs that were not differentially expressed.
To validate tRF&tiRNA PCR Array data, qPCR was carried out to confirm the expression changes of 3′tiR-080-ProTGG(mt). The expression levels of 3′tiR-080-ProTGG(mt) in diabetic patients were downregulated compared with the control group (Fig. 2).
Figure 2: Comparison of 3′tiR-080-ProTGG(mt) levels in diabetes group and controls. *p < 0.05 (n = 3).
Target genes of scanned tRFs/tiRNAs
To investigate the function of 3′tiR-080-ProTGG(mt) in diabetes, we identified the associated target genes based on TargetScan and Miranda algorithms. The potential target genes have been shown in Fig. 3A.
Figure 3: Target gene prediction (A) and gene ontology enrichment analyses (B) for 3′tiR-080-ProTGG(mt).
GO enrichment and KEGG pathway analysis
GO enrichment and KEGG pathway analyses were done to investigate the potential mechanisms and functions of the altered tRFs/tiRNAs. The GO analysis revealed that the 3′tiR-080-ProTGG(mt) enriched in various processes, such as oxoacid metabolic process, carboxylic acid metabolic process, organic acid metabolic process, regulation of immune response through mast cell activation and degranulation, regulation of phosphatidylinositol 3-kinase signaling, and antibiotic biosynthetic process. GO cellular component analysis revealed that 3′tiR-080-ProTGG(mt) was most enriched in intracellular membrane-bounded organelle, plasma membrane bounded cell projection part, synapse, extracellular matrix component, etc. Molecular function analysis indicated that 3′tiR-080-ProTGG(mt) plays a crucial role in chloride channel regulatory activity, thiolester hydrolase activity, signal transducer activity, downstream receptor, anion binding, carbohydrate derive binding (Fig. 3B). Finally, the top 10 significant pathways of 3′tiR-080-ProTGG(mt) were shown in Fig. 4.
Figure 4: Predicted signaling pathways of 3′tiR-080-ProTGG(mt). The horizontal axis indicated the enrichment score (−log10 transformed p-value) and gene number of each cluster. The vertical axis showed annotated functions of target genes.
Moreover, KEGG pathway analysis revealed that 3′tiR-080-ProTGG(mt) may be involved in thermogenesis, the Rap1 signaling pathway, the cAMP signaling pathway, and the Fc epsilon R1 signaling pathway. Rap 1 signaling regulates defective angiogenesis, cell adhesion, migration, survival, and proliferation, which we thought would be involved in microvascular dysfunctions of diabetic patients. cAMP signaling is relevant to secretion, cell proliferation, apoptosis, fatty acid degradation, and regulation of vascular functions (Fig. 5).
Figure 5: Mapping of the cAMP signal pathway. Eleven tsRNA targeted genes are highlighted in orange.
Diabetes and its complications pose serious challenges to our healthcare system. In recent years, considerable progress has been made in exploring new therapeutic and screening methods, however, the exact mechanism remains unclear, and more effective treatments are needed to combat diabetes and its complications.
As sequencing technology develops, more tRNA-derived ncRNAs are being identified to be involved in a variety of pathological processes (Goodarzi et al., 2015; Schorn et al., 2017; Huang et al., 2018; Grafanaki et al., 2019; Liang et al., 2022). In particular, altered tRNA modifications and tRNA fragmentation have been reported to have a major impact on the heritability of diabetes (Arroyo et al., 2021). In the current study, we found that tRNA-derived ncRNAs are dysregulated in the serum of diabetic patients compared with controls. In the diabetes group, the expression of tRFs/tiRNAs was generally downregulated. This result suggests that tRNA-derived ncRNAs may play important roles in diabetes. We then validated the expression of 3′tiR-080-ProTGG(mt) by qPCR, which was downregulated with statistical significance, and the results were consistent with the tRF&tiRNA PCR Array data.
GO analysis showed that 3′tiR-080-ProTGG(mt) is abundantly widespread in intracellular membrane-bounded organelle, plasma membrane bounded cell projection part, synapse, extracellular matrix component, etc. All these integral components are crucial for signaling and cell communication.
Meanwhile, the biological process and molecular function analysis indicated that 3′tiR-080-ProTGG(mt) is enriched in various crucial processes, such as oxoacid metabolic process, regulation of immune system, regulation of phosphatidylinositol 3-kinase signaling, signal transducer activity, downstream receptor binding, etc. It has been reported that the dysregulated immune system is a possible contributing factor associated with diabetes. Researchers have found that insulin could regulate various aspects of immune cells, such as metabolic reprogramming, cell survival, and differentiation via PI3K signaling (van Niekerk et al., 2021). Taken together, we speculate that 3′tiR-080-ProTGG(mt) may play roles in regulating the immune system via PI3K signaling and dysregulated tRF/tiRNA may be involved in diabetes progression. Further studies would focus on the validation of its potential regulatory function in diabetes.
The cAMP pathway is one of the top ten pathways identified by KEGG analysis. It has been reported in diabetes, cAMP could activate PKA, and regulates glucose homeostasis, insulin secretion, gluconeogenesis, glucose uptake, and neural control of glucose homeostasis (Yang and Yang, 2016; Aslam and Ladilov, 2022). Therefore, we focused on the cAMP pathway for detailed analysis. The results illustrated in Fig. 5 provide further support for the significant role of tRFs/tiRNAs in diabetes. However, other pathways such as the Fc epsilon R1 signaling pathway and Rap 1 signaling pathway should not be ignored for their role in diabetes.
To our knowledge, this is the first study to report altered expression profiles of tRFs/tiRNAs in diabetes, and that tRFs/tiRNAs are generally downregulated in diabetes. In particular, 3′ tiR-080-ProTGG(mt) has been validated to be downregulated in diabetes and it may act as a regulatory molecule interacting with many signaling pathways.
Acknowledgement: The authors thank the patients and volunteers that participated in the study and Dr. Dan Wang for her critical discussion of the study.
Funding Statement: This study was funded by the Project of the Shanghai Municipal Health Commission (Grant No. 20194Y0268) and Project of Science and Technology Commission of Shanghai Municipality (Grant No. 20Z11900400).
Author Contributions: J Jin, X Li and T Qiu performed the experiments and drafted the manuscript, L Song and Y Cui analyzed the data, G Zhang and S Li collected samples. W Zhao designed the study and revised the article. All authors read and approved the final manuscript.
Availability of Data and Materials: The datasets generated during and/or analyzed during the current study are available from the corresponding author on reasonable request.
Ethics Approval: The authors state that the study protocol has obtained institutional review board approval and all the research was conformed to the provisions of the Declaration of Helsinki. Informed consent was obtained from all participants.
Conflicts of Interest: The authors declare that they have no conflicts of interest to report regarding the present study.
References
American Diabetes Association (2013). Diagnosis and classification of diabetes mellitus. Diabetes Care 36: S67–S74. https://doi.org/10.2337/dc13-S067 [Google Scholar] [PubMed] [CrossRef]
Arroyo MN, Green JA, Cnop M, Igoillo-Esteve M (2021). tRNA biology in the pathogenesis of diabetes: Role of genetic and environmental factors. International Journal of Molecular Sciences 22: 496. [Google Scholar] [PubMed]
Aslam M, Ladilov Y (2022). Emerging role of cAMP/AMPK signaling. Cells 11: 308. [Google Scholar] [PubMed]
Babski J, Maier LK, Heyer R, Jaschinski K, Prasse D, Jäger D, Randau L, Schmitz RA, Marchfelder A, Soppa J (2014). Small regulatory RNAs in Archaea. RNA Biology 11: 484–493. [Google Scholar] [PubMed]
Cole JB, Florez JC (2020). Genetics of diabetes mellitus and diabetes complications. Nature Reviews Nephrology 16: 377–390. [Google Scholar] [PubMed]
Goodarzi H, Liu X, Nguyen HCB, Zhang S, Fish L, Tavazoie SL (2015). Endogenous tRNA-derived fragments suppress breast cancer progression via YBX1 displacement. Cell 161: 790–802. [Google Scholar] [PubMed]
Grafanaki K, Anastasakis D, Kyriakopoulos G, Skeparnias I, Georgiou S, Stathopoulos C (2019). Translation regulation in skin cancer from a tRNA point of view. Epigenomics 11: 215–245. [Google Scholar] [PubMed]
Guzzi N, Cieśla M, Ngoc PCT, Lang S, Arora S et al. (2018). Pseudouridylation of tRNA-derived fragments steers translational control in stem cells. Cell 173: 1204–1216. [Google Scholar] [PubMed]
Huang SQ, Sun B, Xiong ZP, Shu Y, Zhou HH, Zhang W, Xiong J, Li Q (2018). The dysregulation of tRNAs and tRNA derivatives in cancer. Journal of Experimental & Clinical Cancer Research 37: 101. [Google Scholar]
Jin Q, Ma RCW (2021). Metabolomics in diabetes and diabetic complications: insights from epidemiological studies. Cells 10: 2832. [Google Scholar] [PubMed]
Koye DN, Magliano DJ, Nelson RG, Pavkov ME (2018). The global epidemiology of diabetes and kidney disease. Advances in Chronic Kidney Disease 25: 121–132. [Google Scholar] [PubMed]
Li Y, Hao C, Chen W, Meng Q (2022). Analysis of specific lipid metabolites in cord blood of patients with gestational diabetes mellitus. BIOCELL 46: 1565–1573. https://doi.org/10.32604/biocell.2022.018347 [Google Scholar] [CrossRef]
Liang W, Luo Q, Zhang Z, Yang K, Yang A, Chi Q, Hu H (2022). An integrated bioinformatics analysis and experimental study identified key biomarkers CD300A or CXCL1, pathways and immune infiltration in diabetic nephropathy mice. BIOCELL 46: 1989–2002. https://doi.org/10.32604/biocell.2022.019300 [Google Scholar] [CrossRef]
Maute RL, Schneider C, Sumazin P, Holmes A, Califano A, Basso K, Dalla-Favera R (2013). tRNA-derived microRNA modulates proliferation and the DNA damage response and is down-regulated in B cell lymphoma. Proceedings of the National Academy of Sciences of the United States of America 110: 1404–1409. [Google Scholar] [PubMed]
Papatheodorou K, Banach M, Bekiari E, Rizzo M, Edmonds M (2018). Complications of diabetes 2017. Journal of Diabetes Research 2018: 3086167. [Google Scholar] [PubMed]
Schorn AJ, Gutbrod MJ, LeBlanc C, Martienssen R (2017). LTR-retrotransposon control by tRNA-derived small RNAs. Cell 170: 61–71. [Google Scholar] [PubMed]
Shao Y, Sun Q, Liu X, Wang P, Wu R, Ma Z (2017). tRF-Leu-CAG promotes cell proliferation and cell cycle in non-small cell lung cancer. Chemical Biology & Drug Design 90: 730–738. [Google Scholar]
van Niekerk G, van der Merwe M, Engelbrecht AM (2021). Diabetes and susceptibility to infections: Implication for COVID-19. Immunology 164: 467–475. [Google Scholar] [PubMed]
Yan Z, Cui X, Huang M, Luo Z, Zhou Q et al. (2021). Integrated analysis of tRNA-derived small RNAs reveals new therapeutic genes of hyperbaric oxygen in diabetic foot ulcers. Epigenomics 13: 1817–1829. [Google Scholar] [PubMed]
Yang H, Yang L (2016). Targeting cAMP/PKA pathway for glycemic control and type 2 diabetes therapy. Journal of Molecular Endocrinology 57: R93–R108. [Google Scholar] [PubMed]
Zheng Y, Ley SH, Hu FB (2018). Global aetiology and epidemiology of type 2 diabetes mellitus and its complications. Nature Reviews Endocrinology 14: 88–98. [Google Scholar] [PubMed]
Zhu L, Liu X, Pu W, Peng Y (2018). tRNA-derived small noncoding RNAs in human disease. Cancer Letters 419: 1–7. [Google Scholar] [PubMed]
Cite This Article
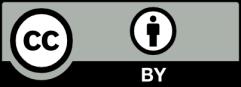
This work is licensed under a Creative Commons Attribution 4.0 International License , which permits unrestricted use, distribution, and reproduction in any medium, provided the original work is properly cited.