Open Access
REVIEW
Molecular regulation mechanism of oocyte maturation in beef cattle
Ningxia Key Laboratory of Ruminant Molecular and Cellular Breeding, College of Agriculture, Ningxia University, Yinchuan, 750021, China
* Corresponding Authors: ZHUOMA LUORENG. Email: ; XINGPING WANG. Email:
(This article belongs to the Special Issue: Cellular and Molecular Toxicology in Reproductive and Developmental Biology)
BIOCELL 2023, 47(7), 1509-1518. https://doi.org/10.32604/biocell.2023.028646
Received 03 January 2023; Accepted 20 March 2023; Issue published 21 June 2023
Abstract
Bovine oocytes are one of the indispensable cells in cattle reproduction and have become a research hot spot in cattle reproduction in recent years. The maturation process of oocytes is mainly regulated by enzymes, hormones, cytokines, and other molecules. The factors affecting cattle oocyte maturation have been previously studied to clarify the molecular mechanisms of cattle oocyte maturation. In this review article, phospholipid protein-3-kinase/protein kinase B, mitogen-activated protein kinase/extracellular signal-regulated kinase, Janus kinase/signal transducer and activator of transcription, epidermal growth factor receptor/extracellular signal-regulated kinase, and other signaling pathways related to oocyte maturation are discussed. In addition, the molecular mechanisms of some coding genes (JY-1, FGF-10, CDC20, etc.) and non-coding genes (miRNA, lncRNA, and circRNA) regulating oocyte maturation have been reviewed to provide new ideas for high reproductive performance molecular breeding of high-quality cattle.Keywords
Oocytes are mainly of primary, secondary, and mature types, which are important for the formation of new mammalian organisms (Walker and Biase, 2020). In females, oogonia proliferate and differentiate to form primary oocytes. After the first meiosis, primary oocytes form secondary oocytes and polar bodies; these secondary oocytes form oocytes and second polar bodies after the second meiosis. Finally, both polar bodies die, and the remaining cells become mature oocytes (Duncan et al., 2020). However, most oocytes stagnate in the metaphase of the first meiosis before entering puberty, and only a few oocytes can resume meiosis and reach maturity. In the stagnant stage of meiosis, the chromatin in the germinal vesicle (GV) is regulated at various levels. Morphologically, the chromosomes lose their individuality and form a loose chromatin mass. The decondensed configuration of chromatin then undergoes profound rearrangements during the final stages of oocyte growth. Functionally, the discrete stages of chromatin condensation are characterized by different levels of transcriptional activity, DNA methylation, and covalent histone modifications. These changes are crucial to confer the oocyte with meiotic and developmental competencies (Luciano et al., 2014). When mammalian oocytes (including cow oocytes) are removed from follicles, they have the ability to spontaneously resume meiosis, which is believed to be the result of the lack of an unidentified follicle inhibitor in domestic animals (Sirard et al., 2006). The maturation of oocytes is influenced by the functional activities of surrounding hematocele or granulation cells. These cells promote the development and maturation of oocytes by regulating various hormones, proteins, metabolites, and regulatory factors (Petro et al., 2012). As the basis of embryonic engineering, the maturation of oocytes is of great significance for the development of in vitro fertilization, transgenesis, embryo cloning, and related biotechnology. The maturation of cattle oocytes also plays a vital role in cattle reproduction (Walter et al., 2020; Gennari et al., 2021). Further understanding of the molecular regulation mechanism of cattle oocyte maturation can accelerate the breeding of improved cattle, aid in tapping the reproductive potential of the most productive female cattle, and provide new means and methods for cattle breeding with high reproductive performance. It further enriches the content of cattle molecular breeding and lays a foundation for developing cattle developmental biology. Thus, this article aimed to provide new ideas for improving the high reproductive performance of high-quality cattle by elucidating the action mechanism affecting the signaling pathway of oocyte maturation and molecular mechanisms of some genes regulating cattle oocyte maturation.
The development of oocytes starts in the fetal ovary, and the final growth and maturation occur in adulthood (Hunt and Hassold, 2008). Cattle oocyte maturation (nuclear maturation and cytoplasmic maturation) refers to the process that cattle oocytes acquire the ability to fertilize after growth and development changes. Cattle excrete secondary oocytes in the middle of the second meiosis (Pan and Li, 2019). Then the sperm enters the zona pellucida, the oocytes are activated and release the second polar body, and the cattle oocytes are fully mature.
As the first meiosis progresses half-way, the oocyte stops dividing, and the chromatin in the nucleus is highly sparse. In addition, its morphological structure is a vesicular GV. In the presence of gonadotropins, meiosis resumes, the nuclear membrane ruptures, ribosomal RNA (rRNA) synthesis ceases, the nucleolus disappears, the nucleus densifies, and the germinal vesicle breaks down (GVBD). The first polar body is expelled and stalls at mid-second meiosis (MII) (Conti and Franciosi, 2018). Of these, the expulsion of the first polar body is the most important characteristic of oocyte nuclear maturation.
Cytoplasmic maturation of oocytes
In oocyte nucleus maturation, the cytoplasm also experiences maturation changes. Oocyte cytoplasmic maturation refers to a process in which the oocyte is prepared for fertilization by dynamic changes in the distribution of organelles (e.g., mitochondria and Golgi apparatus). Cytoplasmic maturation is characterized by molecular and structural changes involving organelles, cytoskeletal reorganization, messenger RNA (mRNA), and protein storage, among which mitochondria changes can be used as a basis of cytoplasmic maturation (Kurowska et al., 2020). In the cytoplasm of most immature eggs, mitochondria show a peripheral distribution with small clusters of mitochondria, which increases in number during oocyte maturation and migrates from the cytoplasmic cortex to the cytoplasmic interior to provide energy for oocyte maturation (Roth, 2018). The migration of mitochondria to high-energy consumption regions is crucial for oocyte maturation.
Major Signaling Pathways Related to Cattle Oocyte Maturation
Similar to other mammals, the maturation of cattle oocytes is a complex and dynamic process (Wen et al., 2020), and some genes and signaling pathways are also involved in its maturation process. To date, several mechanisms related to the regulations of oocyte maturation in animals have been elucidated, involving phospholipid protein-3-kinase/protein kinase B (PI3K/Akt) signaling pathway (Tomek and Smiljakovic, 2005), mitogen-activated protein kinase/extracellular signal-regulated kinase (MAPK/ERK) signaling pathway (Frodin and Gammeltoft, 1999; Conti et al., 2012), Janus kinase/signal transducer and activator of transcription (JAK/STAT) signaling pathway (Meng et al., 2015), and epidermal growth factor receptor/extracellular signal-regulated kinase (EGFR/ERK) signaling pathway (Richani and Gilchrist, 2018; Pocar et al., 2020). Genes and proteins related to oocyte maturation promote or inhibit oocyte maturation through the regulation of the above signaling pathways.
The phospholipid protein-3-kinase/protein kinase B signaling pathway
PI3K/AKT signaling pathway plays a crucial role in cell proliferation (Wang et al., 2019), apoptosis (Zhang et al., 2020), DNA repair (Chen et al., 2008), and protein synthesis (Bibollet-Bahena and Almazan, 2009; Andrade et al., 2017). In animal reproduction, the PI3K/Akt signaling pathway is associated with ovarian functions in mice and other mammals, such as primordial follicle recruitment, granulation proliferation, voxel lutein survival, and oocyte maturation (Adhikari and Liu, 2009; Makker et al., 2014). Activation of the PI3K/AKT signaling pathway requires the involvement of the SRC homology domain-containing protein tyrosine phosphatase 2 (SHP2), while a high expression of PI3K/AKT promotes oocyte maturation (Vigneron et al., 2004; Zhang et al., 2016). Epidermal growth factor (EGF) and cell factor can activate SHP2. The activated SHP2 triggers the PI3K/AKT signaling cascade, allowing PI3K to produce the second messenger phosphatidylinositol 3,4,5-triphosphate (PIP3), which binds to phosphoinositide-dependent dependent kinase-1 (PDK1), prompting PDK1 to phosphorylate threonine 308 (Thr308) of Akt protein and causing Akt to be activated at the GVBD and MI (mid-meiotic) stages of oocytes (Fig. 1). Activated Akt is activated in the MI phase, stimulating the transition from MI (mid-meiotic division I) to MII and promoting oocyte maturation (Tomek and Smiljakovic, 2005). Phosphatase and tensin homolog deleted on chromosome ten (PTEN) can convert PIP3 into phosphatidylinositol diphosphate (PIP2) (McLaughlin et al., 2014), thereby negatively regulating the PI3K-AKT signaling pathway and inhibiting oocyte maturation. As the major signaling pathway affecting oocyte maturation in cattle, the PI3K/AKT pathway promotes oocyte maturation mainly by facilitating the transition from oocyte MI to MII. However, the interaction mechanism between the PI3K/AKT pathway and other signaling pathways is still unclear.
Figure 1: The phospholipid protein-3-kinase/protein kinase B (PI3K/AKT) signaling pathway.
The mitogen-activated protein kinase/extracellular signal-regulated kinase
MAPK/ERK signaling pathway is a protein signaling cascade pathway for oocyte maturation in mammals, such as cattle, sheep, pigs, horses, and mice (Fan and Sun, 2004). Although spontaneous GVBD of mammalian oocytes does not require MAPK activity, artificially increased MAPK activity also accelerates GVBD (Fan and Sun, 2004). In bovine oocytes, MAPK is activated by the injection of Moloney sarcoma oncogene (MOS) mRNA, accelerating the resumption of oocyte meiosis. The activation state of MAPK is determined by the balance between the upstream mitogen-activated extracellular signal-regulated kinase activity, the MAPK cascade activity, and the phosphatase activity responsible for the direct dephosphorylation of MAPK (Kumano et al., 2001).
During oocyte maturation, ribosomal S6 protein kinase (p90RSK) activates MAPK. The activated MAPK binds to myelin transcription factor 1 protein and inactivates the C-terminal phosphorylation of MAPK, thereby promoting the phosphorylation of threonine 14 (Thr14) and tyrosine 15 (Tyr15) of cyclin-dependent kinase-1 (CDK1). It leads to the activation of the maturation-promoting factor (MPF) and enables oocytes to resume meiosis and complete the G2/M phase transformation (Frodin and Gammeltoft, 1999), promoting the maturation of oocytes.
In the granulosa cells of the preovulatory follicle, luteinizing hormone (LH) binds to its parietal granulosa cell receptor LHGC, producing cyclic adenosine monophosphate (cAMP) and activating protein kinase A (PKA). The activated PKA triggers the release of the EGF-related peptides amphiregulin (AREG) and epiregulin (EREG) release and binds to EGF receptors located on mural granulosa cells and cumulus cells (Conti et al., 2012), activating ERK1/2 signaling molecules. High expression of ERK1/2 induces activation of CCAAT, enhancer-binding proteins α/β, transcriptional cofactor CITED4, and genes essential for oocyte maturation, ovulation, and luteinization and inhibits processes regulated by the follicle-stimulating hormone (FSH) pathway (e.g., estrogen synthesis, granulosa cell proliferation, etc.) (Fan et al., 2008; Fan et al., 2010). In addition, ERK1/2 activated by EGF family paracrine factors in cumulus cells phosphorylates connexin 37 (CX37), which reduces the transmission of cAMP and cyclic guanosine monophosphate (cGMP) molecules between cumulus cells and oocytes. In oocytes, cAMP inhibits the resumption of meiosis, and cGMP inhibits phosphodiesterase 3A (PDE3A), which is responsible for the degradation of cAMP. The reduction of cAMP and cGMP in oocytes prevents the inhibition of PKA on CDK1, and the activation of CDK1 allows oocytes to break the GV phase block and enter the division phase (Su et al., 2002; Sela-Abramovich et al., 2005; Fan et al., 2011), promoting oocyte growth and maturation.
The MAPK/ERK signaling pathway plays a vital role in promoting follicle development and oocyte growth maturation (Fig. 2). Although the MAPK/ERK signaling pathway was studied, its specific mechanism during follicle development and oocyte maturation in cattle still needs to be further elucidated.
Figure 2: The role of mitogen-activated protein kinase/extracellular signal-regulated kinase (MAPK/ERK) signaling pathway in animal oocyte maturation.
The Janus kinase/signal transducer and activator of the transcription signaling pathway
The JAK/STAT3 signaling pathway has three main components: receptors, JAKs, signaling sensors, and STATs (Brooks et al., 2014). JAK family is composed of non-receptor tyrosine protein kinases (TYKs), comprising four family members, JAK1, JAK2, JAK3, and TYK2. When cytokines bind to their receptors, JAK tyrosine kinases are activated and transmit regulatory signals (Hu et al., 2021). The STAT family consists of STAT1, STAT2, STAT3, STAT4, STAT5a, STAT5b, and STAT6. In organisms, STAT3 acts as a signal sensor and an activator of transcription and is activated into DNA-binding proteins through tyrosine phosphorylation (Zhong et al., 1994; Hu et al., 2021).
After participating in cytokine receptors, such as leukemia inhibitory factor (LIF), members of the JAK family are phosphorylated, leading to the phosphorylation of downstream STAT proteins. Phosphorylated STAT protein promotes cytoplasmic STAT dimerization and translocates to the nucleus to regulate the transcription of the prolactin gene (PRL) (Martínez-Alarcóna et al., 2022). PRL promotes oocyte maturation through its receptor-mediated calcium regulation mechanism (Meng et al., 2015) (Fig. 3).
Figure 3: The role of Janus kinase/signal transducer and activator of transcription (JAK/STAT) signaling pathway in oocytes maturation.
The Epidermal growth factor receptor/extracellular signal-regulated kinase signaling pathway
EGFR (also known as HER1) is a member of the epidermal growth factor receptor (HER) family, which includes HER1, HER2, HER3, and HER4, and plays an important regulatory role in the regulation of cell physiology. EGFR can be activated by EGF-like peptides (e.g., heparin-binding EGF-like growth factor, transforming growth factor alpha [TGF-α], AREG, and EREG) and converted from monomers to dimers. Stimulation of the EGFR pathway initiates a phosphorylation cascade that activates ERK1/2. Activating ERK1/2 can mediate the translocation of aromatic hydrocarbon receptor (AHR) to the nucleus and form a heterodimer with the AhR nuclear translocator (ARNT) when binding to ligands. Then, the AhR/ARNT complex binds to a specific DNA sequence in the promoter of the target gene cytochrome P450 family 1 subfamily A member 1 (CYP1A1) and triggers its expression. CYP1A1 affects oocyte maturation by promoting the resumption of oocyte meiosis (Pocar et al., 2020) (Fig. 4). In addition, EGFR can induce the expansion of granulation sugar and hydrops cells in follicles by transmitting LH signals to oocytes (Richani and Gilchrist, 2018) and promote the maturation of the oocyte cytoplasm.
Figure 4: The role of epidermal growth factor receptor/extracellular signal-regulated kinase (EGFR/ERK) signaling pathway in oocytes maturation.
Signaling pathways, such as tumor necrosis factor, Wnt/β-catenin, TGF-β, and cAMP, are related to the maturation of cattle oocytes. TGF-β/SMAD signal transduction is caused by the TGF-β and TβRII trigger, TβRII in turn, recruits and phosphorylates TβRI. After TβRII is activated, TβRI phosphorylates R-Smad (Smad 2 or 3), binds it with a common Smad (Smad 4), forms complexes, that translocate to the nucleus, where they regulate the transcription of various target genes together with transcription factors (Hata and Chen, 2016). FSH and LH can regulate the level of natriuretic peptide precursor in mural granulosa cells through TGF-β to regulate the meiotic process of oocytes and affect the maturation of oocytes (Feng et al., 1988; Yang et al., 2019). In addition, TGF-β Superfamily members (such as bone morphogenetic protein 15 [BMP15] and growth and differentiation factor 9 [GDF9]) or related growth factor binding proteins (such as (trans,trans)-1-fluoro-2,5-bis(3-hydroxycarbonyl-4-hydroxy)styrylbenzene or FSB) have been shown to regulate bovine oocyte maturation (Zhang et al., 2015).
Molecular Regulation of Coding Genes during Maturation of Cattle Oocyte
The maturation of oocytes depends on the regulation of many factors. In addition to the environment, oocyte maturation is regulated by many genes (JY-1, FGF-10, CDC20, etc.). Studies suggest that oocyte-expressed JY-1 is required for at least two important aspects of oocyte maturation: nuclear maturation and cumulus expansion (Lee et al., 2014). Pocar et al. (2004) found that CYP1A1 promotes oocyte maturation by activating the AHR signaling pathway and proved the physiological role of AHR in meiotic recovery. Yang et al. (2021) analyzed the porcine GV and MII oocytes by single-cell RNA sequencing (RNA-seq) technology and found 1807 (602 up-regulated and 1205 down-regulated) differential mRNAs, which are mostly enriched in biological and signal pathways related to the meiosis of oocytes.
The 19 coding genes reviewed in the table below regulate oocyte maturation in cattle by up-regulating or down-regulating the expression of genes in related signaling pathways (Table 1). These studies provide a theoretical basis for further exploring and revealing the basic laws of cattle reproduction. However, in the process of oocyte maturation, there are many differentially expressed genes. Further studies are needed to explore how some genes play roles through key genes and related signaling pathways.
Regulation of Non-Coding Genes on Cattle Oocyte Maturation
Non-coding genes achieve their biological functions through interactions with their target genes (Bhatt and Ferrell, 1999). Studies have shown that microRNA (miRNA) (Uhde et al., 2017), long non-coding RNA (lncRNA) (Li et al., 2021), and circular RNA (circRNA) (Fu et al., 2018) have regulatory effects on the maturation of cattle oocytes.
Regulation of miRNA on the maturation of cattle oocytes
MiRNAs are a class of regulatory RNAs, which are short-stranded non-coding RNAs of 21–25 nucleotides in length (Turchinovich et al., 2011). In mammalian cells, the seed region of miRNAs can bind complementarily to sequences on the three prime untranslated regions (3′UTR) of target genes, inhibiting the expression and translation of target genes (Turchinovich et al., 2011). MiRNA levels change during oocyte maturation and ovarian follicle development in cattle, implying that miRNAs may have a regulatory role (Uhde et al., 2017; Salas-Huetos et al., 2019).
In the physiological process of oocyte growth and maturation, multiple miRNAs act on PI3K/Akt, JAK/STAT, and ERK/MAPK signaling pathways to promote or inhibit cattle oocyte maturation by binding to target genes, such as WEE1 homolog (Schizosaccharomyces cerevisiae) (WEE1A), MYC proto-oncogene (MYC), SMAD family member 5 (SMAD5), SMAD2, and BMP type II receptor (BMPR2), respectively (Table 2).
Regulation of lncRNA on the maturation of cattle oocytes
LncRNAs are non-coding RNAs over 200 nucleotides in length that regulate post-transcriptional base editing and translational control of mRNAs and exhibit significant biological roles by affecting the potential regulatory functions of proximity genes (Choi et al., 2019). LncRNAs can participate in post-transcriptional gene regulation through processes, such as RNA maturation, transport, protein synthesis, and silencing of transcribed genes through chromosomal regulation (Whitehead et al., 2009; Geisler and Coller, 2013). However, the molecular mechanism of lncRNA regulation of ovarian oocyte maturation in cattle has been less studied. Li et al. (2021) have identified 1761 differentially expressed lncRNAs by RNA sequencing of bovine GV and mature MII oocytes and found that the above lncRNAs may participate in the maturation of cattle oocytes from GV to MII by regulating key signal pathways. Particularly, lncRNA MSTRG17927, which is significantly decreased in MII stage oocytes, is involved in oocyte maturation through PI3K signaling (Wang et al., 2020). In addition, MSTRG19140 is involved in cattle oocyte meiotic resumption, progesterone-mediated oocyte maturation, and cell cycle regulation (Li et al., 2021). Notably, the MSTRG.283534.2, MSTRG.222844.7, MSTRG.77987.3, MSTRG.123289.5, and MSTRG.18894 are associated with the oocyte maturation of sheep (Shabbir et al., 2021).
To summarize, the function of lncRNA in livestock oocyte maturation has been partially studied, but the functional study in cattle oocytes has just started. Further exploration of lncRNA expression in cattle oocytes and the mechanism of action on oocyte maturation are the urgent problems to be solved in the present study.
Regulation of circRNA on the maturation of cattle oocytes
In recent years, circRNA has attracted attention as a new member of the non-coding RNA family. CircRNA is a new endogenous non-coding RNA, which can regulate gene expression and various biological processes by acting as a miRNA sponge (Xie et al., 2020). Hu et al. (2018) found that circRNAs might regulate ovarian follicle development in ewes by binding miRNAs that regulate the expression of target genes. For example, circ0008219 can regulate follicle growth in ewes by regulating miR-34c-5p, miR-483, and miR-1468-3p to prevent follicular occlusion from leading to granulocyte apoptosis (Hu et al., 2018). Cao et al. (2019) screened 7067 and 637 circRNAs in cumulus cells and oocytes, respectively, through in-depth sequencing and bioinformatics analysis, and found that some circRNA host genes were significantly enriched in various signal pathways related to cumulus cell function and oocyte maturation. Fu et al. (2018) constructed four complementary DNA (cDNA) libraries of bovine cumulus cells, identified 1706 circRNAs, and screened out differential circRNA expression. Through functional annotation and enrichment analysis of host genes, differential circRNA participated in biological processes, such as movement, reproduction, biological adhesion, growth, and so on.
CircARMC4 is an up-regulated circRNA in porcine oocytes required for meiotic maturation and early embryonic development. Inhibition of circARMC4 expression significantly reduced the expulsion of the first polar body of porcine oocytes, leading to a significant decrease in the maturation rate of porcine oocytes, which is the first demonstration of the regulatory role of circRNA on oocyte maturation (Cao et al., 2019). CircRNAs may also regulate oocyte growth and maturation by regulating signaling pathways related to oocyte maturation, but their specific mechanisms of action are not yet clear. In conclusion, circRNA in the maturation of animal oocytes was preliminarily studied. However, studies on the maturation of cattle oocytes should be explored in the future.
As an indispensable cell in cattle reproduction, the rapid maturation of cattle oocytes is conducive to breeding high-quality cattle with high reproductive performance. In recent years, in-depth research on the signaling pathways, functional genes, and molecular markers affecting and regulating oocyte maturation in cattle has been conducted. However, the research on the application of lncRNA and circRNA in the maturation of cattle oocytes is in its initial stage. Furthermore, the molecular regulatory network participation remains to be clarified. Therefore, it is still necessary to excavate more genes related to cattle oocyte maturation in the future and clarify its mechanism through gene function research technology to provide new ideas for the molecular breeding of high reproductive performance of high-quality cattle.
Funding Statement: This study was supported by grants from the Key Research and Development Plan Project of Ningxia Hui Autonomous Region (2021BEF02029), the Key Research and Development Plan Project (Talent Introduction Project) of Ningxia Hui Autonomous Region (2020BEB04006), and the Introducing Talent Research Startup Project of Ningxia University (030900002254).
Author Contributions: BWB designed the research and drafted the manuscript. YXL, FF, and ZXJ for helping to draw the images. JPW, ZMLR, and XPW for modifying the language. All authors reviewed the results and approved the final version of the manuscript.
Ethics Approval: Not applicable.
Conflicts of Interest: The authors declare that they have no conflicts of interest to report regarding the present study.
References
Adhikari D, Liu K (2009). Molecular mechanisms underlying the activation of mammalian primordial follicles. Endocrine Reviews 30: 438–464. https://doi.org/10.1210/er.2008-0048 [Google Scholar] [PubMed] [CrossRef]
Andrade GM, Da Silveira JC, Perrini C, Del Collado M, Gebremedhn S, Tesfaye D, Meirelles FV, Perecin F, Zhang M (2017). The role of the PI3K-Akt signaling pathway in the developmental competence of bovine oocytes. PLoS One 12: e185045. https://doi.org/10.1371/journal.pone.0185045 [Google Scholar] [PubMed] [CrossRef]
Barros RG, Lima PF, Soares ACS, Sanches L, Price CA, Buratini J (2019). Fibroblast growth factor 2 regulates cumulus differentiation under the control of the oocyte. Journal of Assisted Reproduction and Genetics 36: 905–913. https://doi.org/10.1007/s10815-019-01436-7 [Google Scholar] [PubMed] [CrossRef]
Bhatt RR, FerrellJr JE (1999). The protein kinase p90 rsk as an essential mediator of cytostatic factor activity. Science 286: 1362–1365. https://doi.org/10.1126/science.286.5443.1362 [Google Scholar] [PubMed] [CrossRef]
Bibollet-Bahena O, Almazan G (2009). IGF-1-stimulated protein synthesis in oligodendrocyte progenitors requires PI3K/mTOR/Akt and MEK/ERK pathways. Journal of Neurochemistry 109: 1440–1451. https://doi.org/10.1111/j.1471-4159.2009.06071.x [Google Scholar] [PubMed] [CrossRef]
Brooks AJ, Dai W, Mara O, Abankwa ML, Chhabra D et al. (2014). Mechanism of activation of protein kinase JAK2 by the growth hormone receptor. Science 344: 1249783. https://doi.org/10.1126/science.1249783 [Google Scholar] [PubMed] [CrossRef]
Cao Z, Gao D, Xu T, Zhang L, Tong X et al. (2019). Circular RNA profiling in the oocyte and cumulus cells reveals that circARMC4 is essential for porcine oocyte maturation. Aging 11: 8015–8034. https://doi.org/10.18632/aging.102315 [Google Scholar] [PubMed] [CrossRef]
Chen Y, Liu M, Chang Y, Wu C, Hu C, Chen J (2008). Epstein-Barr virus latent membrane protein 1 represses DNA repair through the PI3K/Akt/FOXO3a pathway in human epithelial cells. Journal of Virology 82: 8124–8137. https://doi.org/10.1128/JVI.00430-08 [Google Scholar] [PubMed] [CrossRef]
Choi J, Shin D, Lee H, Oh J (2019). Comparison of long noncoding RNA between muscles and adipose tissues in Hanwoo cattle. Animal Cells and Systems 23: 50–58. https://doi.org/10.1080/19768354.2018.1512522 [Google Scholar] [PubMed] [CrossRef]
Chouzouris T, Dovolou E, Krania F, Pappas IS, Dafopoulos K, Messinis IE, Anifandis G, Amiridis GS (2017). Effects of ghrelin on activation of Akt1 and ERK1/2 pathways during in vitro maturation of bovine oocytes. Zygote 25: 183–189. https://doi.org/10.1017/S096719941700003X [Google Scholar] [PubMed] [CrossRef]
Conti M, Franciosi F (2018). Acquisition of oocyte competence to develop as an embryo: Integrated nuclear and cytoplasmic events. Human Reproduction Update 24: 245–266. https://doi.org/10.1093/humupd/dmx040 [Google Scholar] [PubMed] [CrossRef]
Conti M, Hsieh M, Musa Zamah A, Oh JS (2012). Novel signaling mechanisms in the ovary during oocyte maturation and ovulation. Molecular and Cellular Endocrinology 356: 65–73. https://doi.org/10.1016/j.mce.2011.11.002 [Google Scholar] [PubMed] [CrossRef]
Dehghan Z, Mohammadi-Yeganeh S, Salehi M (2020). MiRNA-155 regulates cumulus cells function, oocyte maturation, and blastocyst formation. Biology of Reproduction 103: 548–559. https://doi.org/10.1093/biolre/ioaa098 [Google Scholar] [PubMed] [CrossRef]
Duncan FE, Schindler K, Schultz RM, Blengini CS, Stein P, Stricker SA, Wessel GM, Williams CJ (2020). Unscrambling the oocyte and the egg: Clarifying terminology of the female gamete in mammals. Molecular Human Reproduction 26: 797–800. https://doi.org/10.1093/molehr/gaaa066 [Google Scholar] [PubMed] [CrossRef]
Dye ZT, Rutledge LV, Penuela S, Dyce PW (2020). Pannexin 1 inhibition delays maturation and improves development of Bos taurus oocytes. Journal of Ovarian Research 13: 98. https://doi.org/10.1186/s13048-020-00704-w [Google Scholar] [PubMed] [CrossRef]
Fan H, Liu Z, Johnson PF, Richards JS (2011). CCAAT/Enhancer-Binding proteins (C/EBP)-α and -β are essential for ovulation, luteinization, and the expression of key target genes. Molecular Endocrinology 25: 253–268. https://doi.org/10.1210/me.2010-0318 [Google Scholar] [PubMed] [CrossRef]
Fan H, O’Connor A, Shitanaka M, Shimada M, Liu Z, Richards JS (2010). β-catenin (CTNNB1) promotes preovulatory follicular development but represses LH-Mediated ovulation and luteinization. Molecular Endocrinology 24: 1529–1542. https://doi.org/10.1210/me.2010-0141 [Google Scholar] [PubMed] [CrossRef]
Fan H, Shimada M, Liu Z, Cahill N, Noma N, Wu Y, Gossen J, Richards JS (2008). Selective expression of KrasG12D in granulosa cells of the mouse ovary causes defects in follicle development and ovulation. Development 135: 2127–2137. https://doi.org/10.1242/dev.020560 [Google Scholar] [PubMed] [CrossRef]
Fan H, Sun Q (2004). Involvement of mitogen-activated protein kinase cascade during oocyte maturation and fertilization in mammals1. Biology of Reproduction 70: 535–547. https://doi.org/10.1095/biolreprod.103.022830 [Google Scholar] [PubMed] [CrossRef]
Feng P, Catt KJ, Knecht M (1988). Transforming growth factor-beta stimulates meiotic maturation of the rat oocyte. Endocrinology 122: 181–186. https://doi.org/10.1210/endo-122-1-181 [Google Scholar] [PubMed] [CrossRef]
Frodin M, Gammeltoft S (1999). Role and regulation of 90 kDa ribosomal S6 kinase (RSK) in signal transduction. Molecular and Cellular Endocrinology 151: 65–77. https://doi.org/10.1016/S0303-7207(99)00061-1 [Google Scholar] [PubMed] [CrossRef]
Fu Y, Jiang H, Liu J, Sun X, Zhang Z, Li S, Gao Y, Yuan B, Zhang J (2018). Genome-wide analysis of circular RNAs in bovine cumulus cells treated with BMP15 and GDF9. Scientific Reports 8: 7944. https://doi.org/10.1038/s41598-018-26157-2 [Google Scholar] [PubMed] [CrossRef]
Geisler S, Coller J (2013). RNA in unexpected places: Long non-coding RNA functions in diverse cellular contexts. Nature Review Molecular Cell Biology 14: 699–712. https://doi.org/10.1038/nrm3679 [Google Scholar] [PubMed] [CrossRef]
Gennari VC, Credendio EM, Fernandes A, Vila RA, Libardi MFC, Silveira RE, Barbosa LR (2021). Folic acid supplementation during oocytes maturation influences in vitro production and gene expression of bovine embryos. Zygote 29: 342–349. https://doi.org/10.1017/S0967199421000022 [Google Scholar] [PubMed] [CrossRef]
Hata A, Chen YG (2016). TGF-β signaling from receptors to smads. Cold Spring Harbor Perspectives in Biology 8: a022061. https://doi.org/10.1101/cshperspect.a022061 [Google Scholar] [PubMed] [CrossRef]
Hu X, Li J, Fu M, Zhao X, Wang W (2021). The JAK/STAT signaling pathway: From bench to clinic. Signal Transduct Target Ther 6: 402. https://doi.org/10.1038/s41392-021-00791-1 [Google Scholar] [PubMed] [CrossRef]
Hu T, Xiong Q, Zhang F, Zhang N, Liu Y, Suo X, Li X, Yang QP, Chen M (2018). Circular RNA profiling reveals chi_circ_0008219 function as microRNA sponges in pre-ovulatory ovarian follicles of goats (Capra hircus). Genomics 110: 257–266. https://doi.org/10.1016/j.ygeno.2017.10.005 [Google Scholar] [PubMed] [CrossRef]
Hunt PA, Hassold TJ (2008). Human female meiosis: What makes a good egg go bad? Trends in Genetics 24: 86–93. https://doi.org/10.1016/j.tig.2007.11.010 [Google Scholar] [PubMed] [CrossRef]
Hussein HE, Johnson WC, Taus NS, Suarez CE, Scoles GA, Ueti MW (2019). Silencing expression of the Rhipicephalus microplus vitellogenin receptor gene blocks Babesia bovis transmission and interferes with oocyte maturation. Parasit Vectors 12: 7. https://doi.org/10.1186/s13071-018-3270-1 [Google Scholar] [PubMed] [CrossRef]
Idrees M, Xu L, Song S, Joo M, Lee K, Muhammad T, El Sheikh M, Sidrat T, Kong I (2019). PTPN11 (SHP2) is indispensable for growth factors and cytokine signal transduction during bovine oocyte maturation and blastocyst development. Cells 8: 1272. https://doi.org/10.3390/cells8101272 [Google Scholar] [PubMed] [CrossRef]
Jamnongjit M, Gill A, Hammes SR (2005). Epidermal growth factor receptor signaling is required for normal ovarian steroidogenesis and oocyte maturation. Proceedings of the National Academy Sciences 102: 16257–16262. https://doi.org/10.1073/pnas.0508521102 [Google Scholar] [PubMed] [CrossRef]
Kumano M, Carroll DJ, Denu JM, Foltz KR (2001). Calcium-Mediated inactivation of the MAP kinase pathway in sea urchin eggs at fertilization. Developmental Biology 236: 244–257. https://doi.org/10.1006/dbio.2001.0328 [Google Scholar] [PubMed] [CrossRef]
Kurowska P, Mlyczyńska E, Estienne A, Barbe A, Rajska I, Soból K, Poniedziałek-Kempny K, Dupont J, Rak A (2020). Expression and impact of vaspin on in vitro oocyte maturation through MAP3/1 and PRKAA1 signalling pathways. International Journal of Molecular Sciences 21: 9342. https://doi.org/10.3390/ijms21249342 [Google Scholar] [PubMed] [CrossRef]
Lee K, Wee G, Zhang K, Folger JK, Knott JG, Smith GW (2014). Functional role of the bovine Oocyte-Specific protein JY-1 in meiotic maturation, cumulus expansion, and subsequent embryonic development1. Biology of Reproduction 90: 1–7. https://doi.org/10.1095/biolreprod.113.115071 [Google Scholar] [PubMed] [CrossRef]
Li MH, Niu MH, Feng YQ, Zhang SE, Tang SW, Wang JJ, Cao HG, Shen W (2021). Establishment of lncRNA-mRNA network in bovine oocyte between germinal vesicle and metaphase II stage. Gene 791: 145716. https://doi.org/10.1016/j.gene.2021.145716 [Google Scholar] [PubMed] [CrossRef]
Liang S, Zhao M, Guo J, Choi J, Kim N, Cui X (2016). Polo-like kinase 4 regulates spindle and actin assembly in meiosis and influence of early embryonic development in bovine oocytes. Theriogenology 85: 754–761. https://doi.org/10.1016/j.theriogenology.2015.10.021 [Google Scholar] [PubMed] [CrossRef]
Luciano AM, Franciosi F, Dieci C, Lodde V (2014). Changes in large-scale chromatin structure and function during oogenesis: A journey in company with follicular cells. Animal Reproduction Science 149: 3–10. https://doi.org/10.1016/j.anireprosci.2014.06.026 [Google Scholar] [PubMed] [CrossRef]
Makker A, Goel MM, Mahdi AA (2014). PI3K/PTEN/Akt and TSC/mTOR signaling pathways, ovarian dysfunction, and infertility: An update. Journal of Molecular Endocrinology 53: R103–R118. https://doi.org/10.1530/JME-14-0220 [Google Scholar] [PubMed] [CrossRef]
Marei WF, Abayasekara DRE, Wathes DC, Fouladi-Nashta AA (2014). Role of PTGS2-generated PGE2 during gonadotrophin-induced bovine oocyte maturation and cumulus cell expansion. Reproduction Biomedicine Online 28: 388–400. https://doi.org/10.1016/j.rbmo.2013.11.005 [Google Scholar] [PubMed] [CrossRef]
Martínez-Alarcóna O, García-Lópeza G, José Raúl Guerra-Morab CAM, Díaza NEDW (2022). Prolactin from pluripotency to central nervous system development. Neuroendocrinology 112: 201–214. https://doi.org/10.1159/000516939 [Google Scholar] [PubMed] [CrossRef]
McLaughlin M, Kinnell HL, Anderson RA, Telfer EE (2014). Inhibition of phosphatase and tensin homologue (PTEN) in human ovary in vitro results in increased activation of primordial follicles but compromises development of growing follicles. Molecular Human Reproduction 20: 736–744. https://doi.org/10.1093/molehr/gau037 [Google Scholar] [PubMed] [CrossRef]
Meng F, Forrester-Gauntlett B, Turner P, Henderson H, Oback B (2015). Signal inhibition reveals JAK/STAT3 pathway as critical for bovine inner cell mass development. Biology of Reproduction 93: 1–9. https://doi.org/10.1095/biolreprod.115.134254 [Google Scholar] [PubMed] [CrossRef]
Miles JR, McDaneld TG, Wiedmann RT, Cushman RA, Echternkamp SE, Vallet JL, Smith TPL (2012). MicroRNA expression profile in bovine cumulus-oocyte complexes: Possible role of let-7 and miR-106a in the development of bovine oocytes. Animal Reproduction Science 130: 16–26. https://doi.org/10.1016/j.anireprosci.2011.12.021 [Google Scholar] [PubMed] [CrossRef]
Pan B, Li J (2019). The art of oocyte meiotic arrest regulation. Reproductive Biology and Endocrinology 17: 8. https://doi.org/10.1186/s12958-018-0445-8 [Google Scholar] [PubMed] [CrossRef]
Petro EML, Leroy JLMR, Covaci A, Fransen E, De Neubourg D, Dirtu AC, de Pauw I, Bols PEJ (2012). Endocrine-disrupting chemicals in human follicular fluid impair in vitro oocyte developmental competence. Human Reproduction 27: 1025–1033. https://doi.org/10.1093/humrep/der448 [Google Scholar] [PubMed] [CrossRef]
Pocar P, Augustin R, Fischer B (2004). Constitutive expression of CYP1A1 in bovine cumulus Oocyte-Complexes in vitro: Mechanisms and biological implications. Endocrinology 145: 1594–1601. https://doi.org/10.1210/en.2003-1254 [Google Scholar] [PubMed] [CrossRef]
Pocar P, Berrini A, di Giancamillo A, Fischer B, Borromeo V (2020). Regulation of the aryl hydrocarbon receptor activity in bovine cumulus-oocyte complexes during in vitro maturation: The role of EGFR and post-EGFR ERK1/2 signaling cascade. Theriogenology 156: 59–69. https://doi.org/10.1016/j.theriogenology.2020.06.037 [Google Scholar] [PubMed] [CrossRef]
Richani D, Gilchrist RB (2018). The epidermal growth factor network: Role in oocyte growth, maturation and developmental competence. Human Reproduction Update 24: 1–14. https://doi.org/10.1093/humupd/dmx029 [Google Scholar] [PubMed] [CrossRef]
Roche J RCRM (2017). Apelin (APLN) regulates progesterone secretion and oocyte maturation. Reproduction 153: 589–603. https://doi.org/10.1530/REP-16-0677 [Google Scholar] [PubMed] [CrossRef]
Roth Z (2018). Symposium review: Reduction in oocyte developmental competence by stress is associated with alterations in mitochondrial function. Journal of Dairy Science 101: 3642–3654. https://doi.org/10.3168/jds.2017-13389 [Google Scholar] [PubMed] [CrossRef]
Sabry R, Apps C, Reiter-Saunders JA, Saleh AC, Balachandran S, St. John EJ, Favetta LA (2021). BPA and BPS affect connexin 37 in bovine cumulus cells. Genes 12: 321. https://doi.org/10.3390/genes12020321 [Google Scholar] [PubMed] [CrossRef]
Salas-Huetos A, James ER, Aston KI, Jenkins TG, Carrell DT, Yeste M (2019). The expression of miRNAs in human ovaries, oocytes, extracellular vesicles, and early embryos: A systematic review. Cell 8: 1564. https://doi.org/10.3390/cells8121564 [Google Scholar] [PubMed] [CrossRef]
Sela-Abramovich S, Chorev E, Galiani D, Dekel N (2005). Mitogen-activated protein kinase mediates luteinizing hormone-induced breakdown of communication and oocyte maturation in rat ovarian follicles. Endocrinology 146: 1236–1244. https://doi.org/10.1210/en.2004-1006 [Google Scholar] [PubMed] [CrossRef]
Shabbir S, Boruah P, Xie L, Kulyar MF, Nawaz M, Yousuf S, Liu T, Jabeen F, Miao X (2021). Genome-wide transcriptome profiling uncovers differential miRNAs and lncRNAs in ovaries of Hu sheep at different developmental stages. Scientific Reports 11: 5865. https://doi.org/10.1038/s41598-021-85245-y [Google Scholar] [PubMed] [CrossRef]
Sinha PB, Tesfaye D, Rings F, Hossien M, Hoelker M, Held E, Neuhoff C, Tholen E, Schellander K, Salilew-Wondim D (2017). MicroRNA-130b is involved in bovine granulosa and cumulus cells function, oocyte maturation and blastocyst formation. Journal of Ovarian Research 10: 37. https://doi.org/10.1186/s13048-017-0336-1 [Google Scholar] [PubMed] [CrossRef]
Sirard MA, Richard F, Blondin P, Robert C (2006). Contribution of the oocyte to embryo quality. Theriogenology 65: 126–136. https://doi.org/10.1016/j.theriogenology.2005.09.020 [Google Scholar] [PubMed] [CrossRef]
Su YQ, Wigglesworth K, Pendola FL, O'Brien MJ, Eppig JJ (2002). Mitogen-activated protein kinase activity in cumulus cells is essential for gonadotropin-induced oocyte meiotic resumption and cumulus expansion in the mouse. Endocrinology 143: 2221–2232. https://doi.org/10.1210/endo.143.6.8845 [Google Scholar] [PubMed] [CrossRef]
Tian X, Wang F, He C, Zhang L, Tan D, Reiter RJ, Xu J, Ji P, Liu G (2014). Beneficial effects of melatonin on bovine oocytes maturation: A mechanistic approach. Journal of Pineal Research 57: 239–247. https://doi.org/10.1111/jpi.12163 [Google Scholar] [PubMed] [CrossRef]
Tomek W, Smiljakovic T (2005). Activation of Akt (protein kinase B) stimulates metaphase I to metaphase II transition in bovine oocytes. Reproduction 130: 423–430. https://doi.org/10.1530/rep.1.00754 [Google Scholar] [PubMed] [CrossRef]
Turchinovich A, Weiz L, Langheinz A, Burwinkel B (2011). Characterization of extracellular circulating microRNA. Nucleic Acids Research 39: 7223–7233. https://doi.org/10.1093/nar/gkr254 [Google Scholar] [PubMed] [CrossRef]
Uhde K, van Tol H, Stout T, Roelen B (2017). MicroRNA expression in bovine cumulus cells in relation to oocyte quality. Non-Coding RNA 3: 12. https://doi.org/10.3390/ncrna3010012 [Google Scholar] [PubMed] [CrossRef]
Uzbekova S, Arlot-Bonnemains Y, Dupont J, Dalbiès-Tran R, Papillier P et al. (2008). Spatio-Temporal expression patterns of aurora kinases a, b, and c and cytoplasmic Polyadenylation-Element-Binding protein in bovine oocytes during meiotic maturation1. Biology of Reproduction 78: 218–233. https://doi.org/10.1095/biolreprod.107.061036 [Google Scholar] [PubMed] [CrossRef]
Vendrell-Flotats M, García-Martínez T, Martínez-Rodero I, López-Béjar M, LaMarre J, Yeste M, Mogas T (2020). In vitro maturation in the presence of Leukemia Inhibitory Factor modulates gene and miRNA expression in bovine oocytes and embryos. Scientific Reports 10: 17777. https://doi.org/10.1038/s41598-020-74961-6 [Google Scholar] [PubMed] [CrossRef]
Vigneron CL, Perreau C, Dupont JL, Uzbekova S, Prigent C, Mermillod P (2004). Several signaling pathways are involved in the control of cattle oocyte maturation. Molecular Reproduction and Development 69: 466–474. https://doi.org/10.1002/(ISSN)1098-2795 [Google Scholar] [CrossRef]
Walker BN, Biase FH (2020). The blueprint of RNA storages relative to oocyte developmental competence in cattle (Bos taurus). Biology of Reproduction 102: 784–794. https://doi.org/10.1093/biolre/ioaa015 [Google Scholar] [PubMed] [CrossRef]
Walter J, Monthoux C, Fortes C, Grossmann J, Roschitzki B, Meili T, Riond B, Hofmann-Lehmann R, Naegeli H, Bleul U (2020). The bovine cumulus proteome is influenced by maturation condition and maturational competence of the oocyte. Scientific Reports 10: 9880. https://doi.org/10.1038/s41598-020-66822-z [Google Scholar] [PubMed] [CrossRef]
Wang J, Niu M, Zhang T, Shen W, Cao H (2020). Genome-Wide network of lncRNA-mRNA during ovine oocyte development from germinal vesicle to metaphase II in vitro. Frontiers in Physiology 11: 1019. https://doi.org/10.3389/fphys.2020.01019 [Google Scholar] [PubMed] [CrossRef]
Wang Q, Zhang Q, Zhang Y, Zhao X (2019). Yak OXGR1 promotes fibroblast proliferation via the PI3K/AKT pathways. Journal of Cellular Biochemistry 120: 6729–6740. https://doi.org/10.1002/jcb.27970 [Google Scholar] [PubMed] [CrossRef]
Wen J, Wang G, Yuan H, Zhang J, Xie H, Gong S, Han X, Tan J (2020). Effects of glucose metabolism pathways on nuclear and cytoplasmic maturation of pig oocytes. Scientific Reports 10: 2782. https://doi.org/10.1038/s41598-020-59709-6 [Google Scholar] [PubMed] [CrossRef]
Whitehead J, Pandey GK, Kanduri C (2009). Regulation of the mammalian epigenome by long noncoding RNAs. Biochimica et Biophysica Acta 1790: 936–947. https://doi.org/10.1016/j.bbagen.2008.10.007 [Google Scholar] [PubMed] [CrossRef]
Xie S, Li M, Chen Y, Liu Y, Ma L, Sun X, Sun Y, Gao R, Huang T (2020). Identification of circular RNAs in the ovarian follicles of Meishan and Duroc sows during the follicular phase. Journal of Ovarian Research 13: 104. https://doi.org/10.1186/s13048-020-00709-5 [Google Scholar] [PubMed] [CrossRef]
Xu D, Wu L, Jiang X, Yang L, Cheng J, Chen H, Hua R, Geng G, Yang L, Li Q (2019). SIRT2 inhibition results in meiotic arrest, mitochondrial dysfunction, and disturbance of redox homeostasis during bovine oocyte maturation. International Journal of Molecular Sciences 20: 1365. https://doi.org/10.3390/ijms20061365 [Google Scholar] [PubMed] [CrossRef]
Yang WL, Li J, An P, Lei AM (2014). CDC20 downregulation impairs spindle morphology and causes reduced first polar body emission during bovine oocyte maturation. Theriogenology 81: 535–544. https://doi.org/10.1016/j.theriogenology.2013.11.005 [Google Scholar] [PubMed] [CrossRef]
Yang CX, Wu ZW, Liu XM, Liang H, Gao ZR, Wang Y, Fang T, Liu YH, Miao YL, Du ZQ (2021). Single-cell RNA-seq reveals mRNAs and lncRNAs important for oocytes in vitro matured in pigs. Reproduction Domestic Animals 56: 642–657. https://doi.org/10.1111/rda.13901 [Google Scholar] [PubMed] [CrossRef]
Yang J, Zhang Y, Xu X, Li J, Yuan F, Bo S, Qiao J, Xia G, Su Y, Zhang M (2019). Transforming growth factor-β is involved in maintaining oocyte meiotic arrest by promoting natriuretic peptide type C expression in mouse granulosa cells. Cell Death and Disease 10: 558. https://doi.org/10.1038/s41419-019-1797-5 [Google Scholar] [PubMed] [CrossRef]
Yun Y, An P, Ning J, Zhao G, Yang W, Lei A (2015). H1foo is essential for in vitro meiotic maturation of bovine oocytes. Zygote 23: 416–425. https://doi.org/10.1017/S0967199414000021 [Google Scholar] [PubMed] [CrossRef]
Zeinab D, Samira M, Delsuz R, Mohammad S (2021). MicroRNA-21 is involved in oocyte maturation, blastocyst formation, and pre-implantation embryo development. Developmental Biology 480: 69–77. https://doi.org/10.1016/j.ydbio.2021.08.008 [Google Scholar] [PubMed] [CrossRef]
Zhang J, Guan Y, Shen C, Zhang L, Wang X (2019). MicroRNA-375 regulates oocyte in vitro maturation by targeting ADAMTS1 and PGR in bovine cumulus cells. Biomedic and Pharmacotherapy 118: 109350. https://doi.org/10.1016/j.biopha.2019.109350 [Google Scholar] [PubMed] [CrossRef]
Zhang K, Hansen PJ, Ealy AD (2010). Fibroblast growth factor 10 enhances bovine oocyte maturation and developmental competence in vitro. Reproduction 140: 815–826. https://doi.org/10.1530/REP-10-0190 [Google Scholar] [PubMed] [CrossRef]
Zhang K, Rajput SK, Lee KB, Wang D, Huang J, Folger JK, Knott JG, Zhang J, Smith GW (2015). Evidence supporting a role for SMAD2/3 in bovine early embryonic development: Potential implications for embryotropic actions of follistatin. Biology of Reproduction 93: 1–11. https://doi.org/10.1095/biolreprod.115.130278 [Google Scholar] [PubMed] [CrossRef]
Zhang R, Yu Z, Zeng L, Zhang S, Bai Y, Miao J, Chen L, Xie J, Zhang Z (2016). SHP2 phosphatase as a novel therapeutic target for melanoma treatment. Oncotarget 7: 73817–73829. https://doi.org/10.18632/oncotarget.12074 [Google Scholar] [PubMed] [CrossRef]
Zhang B, Zhang Y, Li R, Zhao L, Qin S, Pan L, Gao Y (2020). MiR-217 inhibits apoptosis of atherosclerotic endothelial cells via the TLR4/PI3K/Akt/NF-κB pathway. European Review for Medical and Pharmacological Sciences 24: 12867–12877. https://doi.org/10.26355/eurrev_202012_24190 [Google Scholar] [PubMed] [CrossRef]
Zhong Z, Wen Z, Darnell JJE (1994). Stat3: A STAT family member activated by tyrosine phosphorylation in response to epidermal growth factor and interleukin-6. Science 264: 95–98. https://doi.org/10.1126/science.8140422 [Google Scholar] [PubMed] [CrossRef]
Cite This Article
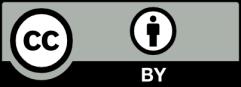