Open Access
ARTICLE
RASAL2 acts as a tumor suppressor in cervical cancer cells
1 Department of Obstetrics and Gynecology, Qilu Hospital, Cheeloo College of Medicine, Shandong University, Jinan, 250012, China
2 Department of Obstetrics and Gynecology, Binzhou Medical University Hospital, Binzhou, 256603, China
* Corresponding Authors: Sai Han, . cn; Youzhong Zhang,
BIOCELL 2023, 47(7), 1549-1560. https://doi.org/10.32604/biocell.2023.027308
Received 24 October 2022; Accepted 10 February 2023; Issue published 21 June 2023
Abstract
Background: This study was designed to investigate the roles of RASAL2 in cervical cancer (CC). Methods: Fifty-four CC tissues and 33 adjacent tissues were obtained from CC patients admitted to our hospital between March 2012 and June 2014. Real-time polymerase chain reaction and western blotting were performed to analyze the expression of RASAL2 mRNA and protein in these tissues, CC cell lines, and normal cervical cells. Over-expression and silencing of RASAL2 were induced after transfection, and the migration, invasion, and proliferation of the CC cell lines were examined. Results: RASAL2 mRNA and protein expressions were significantly down-regulated in CC tissues and cell lines than in adjacent tissues and normal cervical cells, respectively. While low RASAL2 expression correlated with advanced stage and metastasis of CC, its over-expression significantly inhibited proliferation and metastasis of CC cells and induced apoptosis. Under in vitro conditions, silencing of RASAL2 expression could significantly increase the proliferation, invasion, and migration of CC cells. Conclusion: RASAL2 functioned as a tumor suppressor in CC, and was down-regulated in CC tissue samples and cell lines. tumor suppressor in CC, and was down-regulated in CC tissue samples and cell lines.Keywords
Cervical cancer (CC) is the most common type of gynecological malignancy worldwide, serving as one of the leading causes of cancer-related death in females (Zhang et al., 2020). In developing countries, the 5-year survival rate of CC is reported to be less than 50%, while in economically advanced regions, the 5-year survival is about 66% (Wassie et al., 2019). Currently, the treatment of CC mainly relies on surgery, chemotherapy, radiotherapy, and targeted therapy, but the overall survival time is still not high for patients with advanced CC despite great improvements in the treatment (Wipperman et al., 2018). The failure in treatment is mainly due to local recurrence or distant metastasis (Delli Carpini et al., 2010; Zagouri et al., 2012). Therefore, there is an urgent need to further explore novel biomarkers for early diagnosis and better prognosis.
Most mutations affect the tumor suppressor genes associated with CC pathogenesis and treating CC with an aim to restore the normal function of these tumor suppressor genes may be a novel strategy. Therefore, extensive studies have focused on understanding the mechanisms of these tumor suppressors in the pathogenesis and progression of CC (Cheng et al., 2016; Han et al., 2018; Áyen et al., 2020). The Ras pathway is one of the most studied and frequently dysregulated pathways in human cancers (Downward, 2003). Mutations in RAS genes contribute to 20%–30% of all human cancers (Maertens and Cichowski, 2014). Ras is negatively regulated by Ras GTPase-activating proteins (RasGAPs), which catalyze the hydrolysis of Ras-GTP to Ras-GDP (Bernards, 2003). RasGAP, the disabled homolog 2-interacting protein, has been reported to act as an efficient tumor suppressor in prostate cancer (Min et al., 2010). Moreover, the RasGAP, RASA4, could inhibit the proliferation of cervical cancer cells by inactivating the HIFα signaling pathway (Chen et al., 2021). Therefore, RasGAPs are poised to function as potential tumor suppressors.
RAS protein activator like 2 (RASAL2) is a member of the RasGAP family. In most cancers, RASAL2 is inhibited and downregulated so that the cancer cells escape from its preventative effects. In a Luminal-B mammary tumor mouse model, the loss of RASAL2 enhanced metastasis (Sears and Gray, 2017). Downregulation of RASAL2 promotes the proliferation, epithelial-mesenchymal transition, and metastasis of colorectal cancer cells (Jia et al., 2017). In lung adenocarcinoma, downregulation of RASAL2 promotes migration capability due to epithelial-mesenchymal transition via extracellular signal-regulated kinase (ERK) regulation (Li and Li, 2014). RASAL2 was downregulated in ovarian cancer, especially in patients with advanced stages and grades (Huang et al., 2014). These studies highlight that RASAL2 plays a crucial role in suppressing cancer progression. In contrast, RASAL2 also functions as an oncogene in many malignancies such as triple-negative breast cancer (Feng et al., 2014) and liver cancer (Bellazzo and Collavin, 2020). However, the roles of RASAL2 in CC development are still unclear.
This study was designed to investigate the roles of RASAL2 in the pathogenesis of CC using tissue samples from patients with CC and cultured cell lines. Additionally, the bioinformatic data were utilized to predict the potential association between RASAL2 and the other molecules and markers that have been considered to be highly involved in cancer pathogenesis.
The RASAL2 expression in the tumor tissues was searched in the Oncomine database. The gene expression profiling interactive analysis (GEPIA) database was utilized to validate the expression of RASAL2 in the CC tissues. For the survival analysis, the Kaplan–Meier Plotter database was utilized. The patients were categorized as high and low RASAL2 expression groups based on the quartile, followed by subgroup survival analysis.
Patients with CC admitted to our hospital between March 2012 and June 2014 were included in this study. The diagnosis of CC was based on the guidelines proposed by the World Health Organization for the screening and treatment of patients with CC (Sawaya and Huchko, 2017). In total, 54 CC tissues and 33 adjacent tissues were obtained after obtaining the signed informed consent. Patients who had received radiotherapy or chemotherapy were excluded from this study. The research related to the use of human tissues complied with all relevant national regulations and institutional policies in accordance with the tenets of the Helsinki Declaration, and has been approved by the Ethics Committee of Qilu Hospital (Approval No. KYLL-2017-550).
CC cell lines (i.e., SiHa, HeLa, CaSki, and C-33Acells) and normal human cervix epithelial cell line (i.e., Ect1/E6E7), purchased from Cell Bank of Chinese Science Academy (Shanghai, China), were cultured in Dulbecco’s modified Eagle’s medium (DMEM, Thermo Fisher, Shanghai, China) with 10% fetal bovine serum (FBS, Thermo Fisher, Shanghai, China).
SiHa and HeLa cells were transfected with the pcDNA3.1-RASAL2 plasmid to induce over-expression of RASAL2. Cells transfected with blank pcDNA3.1 vector served as the control. To induce RASAL2 silencing, the cells were transfected with the RASAL2 siRNA (sequence: 5′-TTTGCTCGTACAACCAGCA-3′). Cells transfected with scrambled sequences were utilized as the control. All the transfection procedures were conducted using Lipofectamine 2000 (Thermo Fisher, Shanghai, China), according to the manufacturer’s instructions.
Real-time polymerase chain reaction (RT-PCR)
Total RNA was extracted from the tissues and cell lines (i.e., HeLa and SiHa cells) using TRIzol Reagent (Thermo Fisher, Shanghai, China). The first cDNA was synthesized using Takara-PrimeScript RT reagent Kit (TaKaRa, Dalian, China) according to the manufacturer’s instructions. PCR amplification was conducted with One-Step qRT-PCR Kit (Thermo Fisher, Shanghai, China), using the specific primers: forward primer, 5′-AGCAGAAAGGTCCCCTCGTAG-3′; reverse primer, 5′-AGGGTGAGGTATTTGCAGTGT-3′. The amplification conditions were as follows: 95°C for 3 min, followed by 40 cycles of 95°C for 15 s, 58°C for 15 s, and 72°C for 15 s, as well as 72°C for 5 min. GAPDH was used as an internal reference. Finally, the amplification results were analyzed using the 2−ΔΔCt method according to the previous description (Livak and Schmittgen, 2001).
Total protein was extracted from the tissues and cell lines after lysis using RIPA lysate solution (Solarbio, Beijing, China). The protein concentration was determined using the conventional BCA method. Then 50 µg protein was separated using 10% sodium dodecyl sulfate-polyacrylamide gel electrophoresis, followed by transfer onto a polyvinylidene fluoride (PVDF) membrane. The membranes were blocked with 5% skim milk at room temperature for 3 h and then were incubated with primary antibodies against RASAL2 (ab121578, Abcam, Shanghai, China), BCL-associated X (Bax; ab32503, Abcam, Shanghai, China), B-cell lymphoma 2 (Bcl-2; ab32124, Abcam, Shanghai, China), and glyceraldehyde 3-phosphate dehydrogenase (GAPDH; ab8245, Abcam, Shanghai, China) for 4 h at room temperature. Afterwards, the mixture was incubated with horseradish peroxidase-conjugated secondary antibodies (Abcam, Shanghai, China) for 1 h at room temperature. Chemiluminescence was detected using an enhanced chemiluminescence kit (Thermo Fisher, Shanghai, China). Image-J software was utilized for determining the protein expression levels. All the tests were performed at least in triplicate.
The transfected cells (3 × 103 cells) were incubated in a 96-well plate at 37°C. The cellular proliferation was determined using Cell Counting kit-8 (CCK-8, Dojindo, Kumamoto, Japan), with strict adhesion to the manufacturer’s instructions. The cellular mixture was incubated at 37°C for 2 h, and the cellular viability was determined at a wavelength of 450 nm using a microplate reader.
The cellular apoptosis was determined as previously described (Rieger et al., 2011). Briefly, the Annexin V-FITC Apoptosis Detection Kit (Thermo Fisher, Shanghai, China) was used for cell apoptosis assays in accordance with the manufacturer’s protocols. Following re-suspending in PBS buffer, the transfected cells were added with propidium iodide (PI) and Annexin V-FITC solution. Eventually, the apoptosis was measured using the flow cytometry upon incubation at 4°C for 30 min.
Transfected cells were seeded in a 24-well plate and scratched by a 200 μL tip perpendicular to the horizontal line. Each well was washed with Dulbecco’ phosphate-buffered saline to remove scratched cells, and images were captured under a light microscope. Then, DMEM was added to the cells. After incubation at 37°C for 24 h, images were captured under a light microscope.
Transwell assay was utilized to analyze the cell invasion and migration in different treatments. In brief, the chambers (BD Biosciences, San Diego, USA) were pre-coated with commercial Matrigel at room temperature for 1 h. Specifically, serum-free DMEM was added to the upper chamber with transfected cells (1 × 106 cells/mL), while to the lower chamber, DMEM with 10% FBS was added. Afterwards, all the Transwell chambers were incubated at 37°C for 24 h. The cells with no invasion to the upper Transwell were wiped using a cotton-tipped swab. The cells on the other side were stained and photographed with a light microscope under a magnification of 200×.
SPSS 21.0 software was used for the statistical analysis. All data were expressed as mean ± standard deviation (SD). The comparison between the two groups was performed by Student’s t-test. Comparison among groups was conducted by one-way ANOVA. A p-value of < 0.05 was considered to be statistically significant.
Expression of RAS protein activator like 2 in the cervical cancer tissues according to the bioinformatic analysis
Based on the Oncomine database, we searched studies on RASAL2, this was further validated using the GEPIA database, which revealed that the expression of RASAL2 in the CC tissues was significantly lower compared to the adjacent tissues (p < 0.01, Fig. 1A). In contrast to our expectation, patients with low expression of RASAL2 showed a higher overall survival compared with the counterparts with high expression of RASAL2 (p = 0.041, Fig. 1B).
Figure 1: Bioinformatic analysis for the RASAL2 expression in the Oncomine database. (A) The GEPIA database was utilized to validate the expression of RASAL2 in the CC tissues. (B) Kaplan–Meier plotter database was utilized for the survival analysis (CC, cervical cancer; RASAL2, RAS protein activator like 2; GEPIA, gene expression profiling interactive analysis).
The patient characteristics are shown in Table 1. The included 54 patients were divided into the low RASAL2 group (n = 29) and the high RASAL2 group (n = 25). No statistical differences were noticed in age, tumor size, and differentiation of the tissues (p > 0.05). Significant differences were noticed in the clinical stage (p = 0.024), lymph node metastasis (p = 0.039), and distal metastasis (p = 0.025). Patients with high expression of RASAL2 showed a significantly lower incidence of advanced-stage, lymph node metastasis, and distal metastasis.
RAS protein activator like 2 is downregulated in CC tissues and cell lines
A significant decrease in the expression of RASAL2 mRNA and protein was seen in the CC tissues than in the adjacent normal tissues (p < 0.05, Figs. 2A and 2B). Patients with a high RASAL2 expression showed a higher survival rate compared with their counterparts with low expression of RASAL2 (p < 0.05, Fig. 2E). Then we determined the expression of RASAL2 mRNA and protein in the CC cell lines, including HeLa, C-33A, SiHa, and CaSki cells, with Ect1/E6E7 as the control. As shown in Figs. 2C and 2D, the expressions of RASAL2 mRNA and protein in the CC lines were significantly lower than that in the normal control (all p < 0.05). These findings indicate that low RASAL2 expression is closely associated with the pathogenesis of CC.
Figure 2: Downregulation of RASAL2 in cervical cancer tissues and cell lines was associated with poor survival among patients with CC. (A, B) RASAL2 mRNA and protein in cervical cancer tissues and adjacent tissues. **p < 0.01 vs. adjacent tissues. (C, D) The mRNA and protein expression of RASAL2 in CC cell lines and Ect1/E6E7 cells. #p < 0.05 and ##p < 0.01 vs. Ect1/E6E7. (E) CC patients with low RASAL2 expression showed a shorter survival time than those with high expression (CC, cervical cancer; RASAL2, RAS protein activator like 2; GEPIA, gene expression profiling interactive analysis).
RAS protein activator like 2 triggers CC cell proliferation inhibition and CC cell apoptosis
To induce the over-expression of RASAL2, the cells were transfected with pcDNA3.1-RASAL2 plasmid. The RASAL2 mRNA and protein expressions were significantly upregulated in the RASAL2 group than in the blank group (Figs. 3A and 3B). RASAL2 over-expression could significantly inhibit the proliferation of SiHa and HeLa cells compared to the blank group in a time-dependent manner (Figs. 3C and 3D). In addition, RASAL2 could induce significant apoptosis compared with that in the blank group (p < 0.05, Fig. 3E). Then we determined the expression of Bax and Bcl-2 as pro-apoptotic and anti-apoptotic factors in these cells, respectively. Our data showed significant down-regulation of Bcl-2 and significant upregulation of Bax in RASAL2 over-expressing CC cells (Fig. 3F). Thus, RASAL2 over-expression triggered the inhibition of cell proliferation and induced cell apoptosis in HeLa and SiHa cells.
Figure 3: High RASAL2 expression triggered the inhibition of CC cell proliferation and induction of cell apoptosis. (A, B) RASAL2 mRNA and protein expression in SiHa and HeLa cells transfected with blank vector or RASAL2 plasmid. (C, D) High RASAL2 expression triggered the inhibition of cell proliferation in CC cell lines. (E) High RASAL2 expression induced a significant increase in cell apoptosis compared with that in the blank control. (F) RASAL2 expression induced the upregulation of Bax and the down-regulation of Bcl-2. ## or **p < 0.01 vs. blank control (Bax, BCL-associated X; Bcl2, B-cell lymphoma 2; CC, cervical cancer; RASAL2, RAS protein activator like 2).
Inhibition of CC cell invasion and migration in vitro by RAS protein activator like 2 over-expression
Wound healing analysis showed that RASAL2 over-expression induced significant inhibition of the CC cell line migration (Figs. 4A and 4B). Transwell assay indicated that RASAL2 could significantly decrease the number of invaded CC cells compared with the control (Figs. 4C and 4D). Therefore, RASAL2 over-expression inhibited the invasion and migration of CC cell lines under in vitro conditions.
Figure 4: RASAL2 over-expression inhibited the migration and invasion of CC cell lines. (A, B) Wound healing analysis indicated that the transfection of RASAL2 using RASAL2 plasmid could significantly decrease the cell migration at 0 and 24 h, respectively. **p < 0.01 vs. blank control. (C, D) Transwell assay indicated a significant decrease in the invasion of SiHa and HeLa cells transfected with RASAL2 plasmid compared with the blank control. **p < 0.01 vs. blank control (CC, cervical cancer; RASAL2, RAS protein activator like 2).
Knockdown of RAS protein activator like 2 contributed to the proliferation of CC cell lines
The roles of RASAL2 in these above-mentioned experiments were then validated. We silenced the RASAL2 and determined the effects of RASAL2 inhibition on the proliferation and apoptosis of CC cell lines. In the si-RASAL2 group, the expression of RASAL2 was significantly lowered compared with that of the control group (Figs. 5A and 5B). RASAL2 knockdown contributed to the proliferation of SiHa and HeLa cells compared with that of the control (Figs. 5C and 5D).
Figure 5: RASAL2 knockdown contributed to CC cell proliferation. (A, B) RASAL2 mRNA and protein expression in SiHa and HeLa cells that had been transfected with RASAL2 siRNA or NC siRNA. (C, D) RASAL2 knockdown contributed to SiHa and HeLa cell proliferation. **p < 0.01 vs. NC siRNA (CC, cervical cancer; NC, negative control; RASAL2, RAS protein activator like 2).
Silencing of RAS protein activator like 2 contributed to the invasion and migration of CC cell lines
The wound healing assay indicated that the cell migration was significantly upregulated in the si-RASAL2 group compared with that in the si-NC group (Figs. 6A and 6B). Similarly, the invasive capacity of CC cells also increased significantly in the si-RASAL2 group compared to the si-NC group (Figs. 6C and 6D). Thus, silencing of RASAL2 expression promoted the invasion and migration of CC cell lines under in vitro condition.
Figure 6: RASAL2 silencing triggered the increase of migration and invasion of CC cells. (A, B) Wound healing indicated that RASAL2 silencing contributed to the cell migration of SiHa cells and HeLa cells. (C, D) Transwell assay indicated that RASAL2 silencing contributed to SiHa and HeLa cell invasion. **p < 0.01 vs. NC siRNA (CC, cervical cancer; NC, negative control; RASAL2, RAS protein activator like 2).
CC is a gynecological cancer and causes cancer-related deaths worldwide. Human papillomavirus (HPV) is implicated in the etiology of cervical malignancy. However, much evidence indicates that HPV infection is necessary but not sufficient to cause cervical carcinogenesis (Sung et al., 2021). Additional factors may contribute to the pathogenesis of CC (Reshmi and Pillai, 2008; Łaniewski et al., 2019). Therefore, the pathogenesis of CC remains worthy of study.
Ras protein, a molecular switch regulating cellular biological functions, is negatively regulated by RasGAPs (Xu et al., 2014). RAS gene mutation has been reported in many types of malignancies but occurs at a low frequency in CC (Qiu et al., 2022). However, studies have also confirmed significantly higher levels of K-RAS and H-RAS genes in CC tissues than those in normal cervical tissues, and they were found to be involved in the occurrence and metastasis of tumors (Tatli and Dinler Doganay, 2021). These suggested that the RAS gene may be activated by mechanisms other than acquired mutations in CC. As a RasGAP, RASAL2 can catalyze the hydrolysis of Ras-GTP to Ras-GDP, thereby inhibiting the RAS gene activity (Mosaddeghzadeh and Ahmadian, 2021). RASAL2 was found to act as a tumor suppressor in breast cancer (McLaughlin et al., 2013), and the phosphorylated RASAL2 promoted tumor progression in ER+ and ER-breast cancers (Wang et al., 2019), although it played an oncogenic role in some triple-negative breast cancers (Kreider-Letterman et al., 2022). The promoter region of the RASAL2 gene was generally in an ultra-low methylation state in hepatocellular carcinoma tissues, and the downregulation of this gene could significantly inhibit the growth and invasion of hepatocellular carcinoma cells (Harrell Stewart and Clark, 2020). These controversial results suggested different roles of RASAL2 in the development of human cancers (Zhou et al., 2019). To our best knowledge, only a few studies have investigated the expression of RASAL2 in CC.
In this study, we investigated the potential roles of RASAL2 in the pathogenesis of CC using tissue samples from patients with CC and cultured cell lines. Our findings indicated a significant decrease in the expression of RASAL2 mRNA and protein in CC tissues than in the adjacent normal tissues, and the low expression of RASAL2 was correlated with advanced cancer stage and metastasis. In addition, the expression of RASAL2 mRNA and protein in the CC cell lines (i.e., SiHa, HeLa, CaSki, and C-33A cells) was significantly lower than in the human cervix epithelial cells (i.e., Ect1/E6E7). Thus, our data showed that RASAL2 contributed to the pathogenesis and development of CC under in vitro and in vivo conditions.
Upon determining the expression of RASAL2 in CC samples and cell lines, we investigated the potential roles of RASAL2 in CC. Our data showed that over-expression of RASAL2 inhibited the proliferation, migration, and invasion of CC cell lines, together with inducing significant cellular apoptosis. The knockdown of RASAL2 was performed to validate its pro-oncogenic role, and all the above-mentioned phenomena were reversed after RASAL2 silencing, which indicated the function of RASAL2 as a tumor suppressor in CC. Accordingly, the pro-oncogenic role of RASAL2 has been reported in several human malignancies such as luminal B breast cancer (McLaughlin et al., 2013; Shen et al., 2013; Sears and Gray, 2017), renal cancer (Hui et al., 2018b), bladder cancer (Hui et al., 2017, 2018a), astrocytoma (Weeks et al., 2012), nasopharyngeal cancer (Wang et al., 2015), pancreatic cancer (Yin et al., 2019), ovarian cancer (Huang et al., 2014), and lung cancer (Li and Li, 2014).
To further illustrate the effects of RASAL2 expression on cell apoptosis, we determined Bax and Bcl-2 expressions in the presence of RASAL2 over-expression or silencing. Bax is well-known to serve as an apoptotic activator by forming a heterodimer with Bcl-2 and plays important roles in the pathogenesis of cancer (Liu et al., 2016), while Bcl2, an integral outer mitochondrial membrane protein, is also crucial for the pathogenesis of cancer as it can block cell apoptosis (Frenzel et al., 2009). In this study, in the cells with over-expressing RASAL2, Bax was significantly upregulated, and Bcl-2 was significantly downregulated compared with their levels in the normal control. On this basis, it is reasonable to assume that RASAL2 may promote CC cell apoptosis by modulating the expression of Bax and Bcl2. However, the related mechanisms are still not well defined.
Cancer cell migration and invasion are essential for metastasis (van Zijl et al., 2011). RASAL2 functions as a tumor and metastasis suppressor (McLaughlin et al., 2013). The knockdown of RASAL2 could reduce the invasion of HCC cells, while the downregulation of RASAL2 enhanced the migration and invasion of colorectal cancer cells (Zhou et al., 2019) in the data from this study indicated that RASAL2 over-expression inhibited cellular migration and invasion, while RASAL2 silencing contributed to the migration and invasion of SiHa and HeLa cells. Zhao et al. (2016) reported that overexpression of RASAL2 inhibited the migration of the SNU-1 gastric cancer cell line (Zhao et al., 2016). Hui et al. (2017) also found that overexpression of RASAL2 inhibited migration and invasion of the T24 bladder cancer cell line (Hui et al., 2017). Therefore, we assumed that RASAL2 acted as a suppressor gene in CC cell migration and invasion and metastasis of CC tissues.
As the information on the expression of RASAL2 in the CC tissues is rare, we then utilized bioinformatic data to predict the potential association between RASAL2 and the other molecules and markers that have been considered to be highly involved in the pathogenesis of cancer. Our data showed a positive correlation of RASAL2 with the cell division cycle 42 (CDC42), ribosomal protein S6 kinase beta-1 (RPS6KB1), Janus kinase 1 (JAK1), mothers against decapentaplegic homolog 1 (SMAD1), SMAD4, SMAD5, tuberous sclerosis complex subunit 1 (TSC1), signal transducer and activator of transcription 6 (STAT6), and STAT3, respectively (Fig. 7). Thus, RASAL2 downregulation in CC tissues may be accompanied by silencing of these molecules. Consistently, SMAD4 inactivation was best recognized in pancreatic cancer (McCarthy and Chetty, 2018), and TSC1 loss of heterozygosity was seen in 54% of bladder cancers (Guo et al., 2013). Likewise, low STAT6 expression was associated with worse survival (Wang et al., 2020). However, recent studies have found that CDC42 (Ye et al., 2015), RPS6KB1 (Wang et al., 2021), SMAD1 (Xu et al., 2021), JAK1 and STAT3 (Liu et al., 2022), and SMAD5 (Ke et al., 2021) are highly expressed in many malignant tumors, and closely related to tumorigenesis, invasion, and metastasis. Compared with the database, our data showed a non-consistent trend of the relationship between overall survival and RASAL2 expression. These may lead to ambiguity in understanding the roles of RASAL2 in metastasis. Indeed, the roles of RASAL2 in the pathogenesis of malignancies are still controversial. The biological function of RASAL2 is influenced by cellular context to affect its pro or anti-oncogenic activity in human cancers (Hui et al., 2018b). Mechanistically, previous studies have shown that different signaling pathways (as well as cross-talk) of RAS, including the RAS-ERK pathway, phosphoinositide-3-kinase (PI3K)/AKT/mechanistic target of rapamycin (mTOR) signaling pathway, and nuclear factor (NF)-κB pathway may account for different biological outcomes of activity (Zhou et al., 2019). In the future, more studies are required to illustrate the potential roles of RASAL2 in the metastasis of CC.
Figure 7: Potential markers associated with the RASAL2 expression. The screened makers and molecules were SMAD1 (A), SMAD4 (B), SMAD5 (C), STAT3 (D), STAT6 (E), TSC1 (F), RPS6KB1 (G), JAK1 (H) and CDC42 (I) (RASAL2, RAS protein activator like 2; SMAD, mothers against decapentaplegic homolog 1; STAT, signal transducer and activator of transcription 6; TSC1, tuberous sclerosis complex subunit 1; RPS6KB1, ribosomal protein S6 kinase beta-1; JAK1, Janus kinase 1; CDC42, cell division cycle 42).
Indeed, there are some limitations to this study. First, the included sample size is not large, and in the subsequent analysis, more studies involving a large sample size are required. Second, our data showed that RASAL2 could act as a suppressor for CC pathogenesis, but the exact mechanisms are still not well defined. In addition, the tissues from patients were not selected according to the degree of malignancy and cell passage information. More studies are needed in the future to clarify the potential association between clinical stage and RASAL2, which will benefit the formulation of individualized clinical strategies.
In summary, RASAL2 acted as a tumor suppressor for CC, which was featured by down-regulation in the CC tissue samples and cell lines. Its over-expression could inhibit the invasion, migration, and metastasis of cancer cells under in vitro and in vivo conditions. The inhibitory effect of RASAL2 on CC development may be related to the expression of Bax, Bcl2, CDC42, RPS6KB1, JAK1, SMAD1, SMAD4, SMAD5, TSC1, STAT6, and STAT3. This study provides a reference for the research on targeted therapy of CC and enriches the knowledge of the function of RASAL2 in different tissues and genetic backgrounds.
Funding Statement: This study was supported by the National Natural Science Foundation of China (Grant No. 81572559) and the Shandong Key Research and Development Plan (Grant No. 2017CXGC1210).
Author Contributions: Study conception and design: Sai Han, Youzhong Zhang; data collection: Fangfang Li, Shouyan Cao, Xia Li, Chao Zhou; analysis and interpretation of results: Li Chen; draft manuscript preparation: Li Chen. All authors reviewed the results and approved the final version of the manuscript.
Availability of Data and Materials: The datasets generated during and/or analyzed during the current study are available from the corresponding author upon reasonable request.
Ethics Approval: The research related to the sue of human tissues complied with all the relevant national regulations and institutional policies and was in accordance with the tenets of the Helsinki Declaration and has been approved by the Ethics Committee of Qilu Hospital (Approval No. KYLL-2017-550). Informed consent has been obtained from all individuals included in this study.
Conflicts of Interest: The authors declare that they have no conflicts of interest to report regarding the present study.
References
Áyen Á, Jiménez Martínez Y, Boulaiz H (2020). Targeted gene delivery therapies for cervical cancer. Cancers 12: 1301. https://doi.org/10.3390/cancers12051301 [Google Scholar] [PubMed] [CrossRef]
Bellazzo A, Collavin L (2020). Cutting the brakes on Ras-cytoplasmic GAPs as targets of inactivation in cancer. Cancers 12: 3066. https://doi.org/10.3390/cancers12103066 [Google Scholar] [PubMed] [CrossRef]
Bernards A (2003). GAPs galore! A survey of putative Ras superfamily GTPase activating proteins in man and Drosophila. Biochimica et Biophysica Acta 1603: 47–82. https://doi.org/10.1016/s0304-419x(02)00082-3 [Google Scholar] [PubMed] [CrossRef]
Chen J, Huang J, Huang Q, Li J, Chen E, Xu W (2021). RASA4 inhibits the HIFα signaling pathway to suppress proliferation of cervical cancer cells. Bioengineered 12: 10723–10733. https://doi.org/10.1080/21655979.2021.2002499 [Google Scholar] [PubMed] [CrossRef]
Cheng HY, Zhang T, Qu Y, Shi WJ, Lou G, Liu YX, Zhang YY, Cheng L (2016). Synergism between RIZ1 gene therapy and paclitaxel in SiHa cervical cancer cells. Cancer Gene Therapy 23: 392–395. https://doi.org/10.1038/cgt.2016.44 [Google Scholar] [PubMed] [CrossRef]
Delli Carpini J, Karam AK, Montgomery L (2010). Vascular endothelial growth factor and its relationship to the prognosis and treatment of breast, ovarian, and cervical cancer. Angiogenesis 13: 43–58. https://doi.org/10.1007/s10456-010-9163-3 [Google Scholar] [PubMed] [CrossRef]
Downward J (2003). Targeting RAS signalling pathways in cancer therapy. Nature Reviews Cancer 3: 11–22. https://doi.org/10.1038/nrc969 [Google Scholar] [PubMed] [CrossRef]
Feng M, Bao Y, Li Z, Li J, Gong M et al. (2014). RASAL2 activates RAC1 to promote triple-negative breast cancer progression. The Journal of Clinical Investigation 124: 5291–5304. https://doi.org/10.1172/JCI76711 [Google Scholar] [PubMed] [CrossRef]
Frenzel A, Grespi F, Chmelewskij W, Villunger A (2009). Bcl2 family proteins in carcinogenesis and the treatment of cancer. Apoptosis 14: 584–596. https://doi.org/10.1007/s10495-008-0300-z [Google Scholar] [PubMed] [CrossRef]
Guo Y, Chekaluk Y, Zhang J, Du J, Gray NS, Wu CL, Kwiatkowski DJ (2013). TSC1 involvement in bladder cancer: diverse effects and therapeutic implications. Journal of Pathology 230: 17–27. https://doi.org/10.1002/path.4176 [Google Scholar] [PubMed] [CrossRef]
Han H, Chen W, Yang J, Liang X, Wang Y, Li Q, Yang Y, Li K (2018). Inhibition of cell proliferation and migration through nucleobase-modified polyamidoamine-mediated p53 delivery. International Journal of Nanomedicine 13: 1297–1311. https://doi.org/10.2147/ijn.S146917 [Google Scholar] [PubMed] [CrossRef]
Harrell Stewart DR, Clark GJ (2020). Pumping the brakes on RAS—negative regulators and death effectors of RAS. Journal of Cell Science 133: jcs238865. https://doi.org/10.1242/jcs.238865 [Google Scholar] [PubMed] [CrossRef]
Huang Y, Zhao M, Xu H, Wang K, Fu Z, Jiang Y, Yao Z (2014). RASAL2 down-regulation in ovarian cancer promotes epithelial-mesenchymal transition and metastasis. Oncotarget 5: 6734–6745. https://doi.org/10.18632/oncotarget.2244 [Google Scholar] [PubMed] [CrossRef]
Hui K, Gao Y, Huang J, Xu S, Wang B et al. (2017). RASAL2, a RAS GTPase-activating protein, inhibits stemness and epithelial-mesenchymal transition via MAPK/SOX2 pathway in bladder cancer. Cell Death & Disease 8: e2600. https://doi.org/10.1038/cddis.2017.9 [Google Scholar] [PubMed] [CrossRef]
Hui K, Wu S, Yue Y, Gu Y, Guan B, Wang X, Hsieh JT, Chang LS, He D, Wu K (2018a). RASAL2 inhibits tumor angiogenesis via p-AKT/ETS1 signaling in bladder cancer. Cellular Signalling 48: 38–44. https://doi.org/10.1016/j.cellsig.2018.04.006 [Google Scholar] [PubMed] [CrossRef]
Hui K, Yue Y, Wu S, Gu Y, Guan B, Wang X, Hsieh JT, Chang LS, He D, Wu K (2018b). The expression and function of RASAL2 in renal cell carcinoma angiogenesis. Cell Death & Disease 9: 881. https://doi.org/10.1038/s41419-018-0898-x [Google Scholar] [PubMed] [CrossRef]
Jia Z, Liu W, Gong L, Xiao Z (2017). Downregulation of RASAL2 promotes the proliferation, epithelial-mesenchymal transition and metastasis of colorectal cancer cells. Oncology Letters 13: 1379–1385. https://doi.org/10.3892/ol.2017.5581 [Google Scholar] [PubMed] [CrossRef]
Ke H, Zhang J, Wang F, Xiong Y (2021). ZNF652-induced circRHOT1 promotes SMAD5 expression to modulate tumorigenic properties and nature killer cell-mediated toxicity in bladder cancer via targeting miR-3666. Journal of Immunology Research 2021: 7608178. https://doi.org/10.1155/2021/7608178 [Google Scholar] [PubMed] [CrossRef]
Kreider-Letterman G, Carr NM, Garcia-Mata R (2022). Fixing the GAP: The role of RhoGAPs in cancer. European Journal of Cell Biology 101: 151209. https://doi.org/10.1016/j.ejcb.2022.151209 [Google Scholar] [PubMed] [CrossRef]
Łaniewski P, Cui H, Roe DJ, Barnes D, Goulder A, Monk BJ, Greenspan DL, Chase DM, Herbst-Kralovetz MM (2019). Features of the cervicovaginal microenvironment drive cancer biomarker signatures in patients across cervical carcinogenesis. Scientific Reports 9: 7333. https://doi.org/10.1038/s41598-019-43849-5 [Google Scholar] [PubMed] [CrossRef]
Li N, Li S (2014). RASAL2 promotes lung cancer metastasis through epithelial-mesenchymal transition. Biochemical and Biophysical Research Communications 455: 358–362. https://doi.org/10.1016/j.bbrc.2014.11.020 [Google Scholar] [PubMed] [CrossRef]
Liu Z, Ding Y, Ye N, Wild C, Chen H, Zhou J (2016). Direct activation of bax protein for cancer therapy. Medicinal Research Reviews 36: 313–341. https://doi.org/10.1002/med.21379 [Google Scholar] [PubMed] [CrossRef]
Liu C, Feng H, Song L, Li S, Wu Y, Yang L (2022). Synergistic effects of thalidomide and cisplatin are mediated via the PI3K/AKT and JAK1/STAT3 signaling pathways in cervical cancer. Oncology Reports 48: 457. https://doi.org/10.3892/or.2022.8384 [Google Scholar] [PubMed] [CrossRef]
Livak KJ, Schmittgen TD (2001). Analysis of relative gene expression data using real-time quantitative PCR and the 2-ΔΔCT method. Methods 25: 402–408. https://doi.org/10.1006/meth.2001.1262 [Google Scholar] [PubMed] [CrossRef]
Maertens O, Cichowski K (2014). An expanding role for RAS GTPase activating proteins (RAS GAPs) in cancer. Advances in Biological Regulation 55: 1–14. https://doi.org/10.1016/j.jbior.2014.04.002 [Google Scholar] [PubMed] [CrossRef]
McCarthy AJ, Chetty R (2018). Smad4/DPC4. Journal of Clinical Pathology 71: 661–664. https://doi.org/10.1136/jclinpath-2018-205095 [Google Scholar] [PubMed] [CrossRef]
McLaughlin SK, Olsen SN, Dake B, de Raedt T, Lim E et al. (2013). The RasGAP gene, RASAL2, is a tumor and metastasis suppressor. Cancer Cell 24: 365–378. https://doi.org/10.1016/j.ccr.2013.08.004 [Google Scholar] [PubMed] [CrossRef]
Min J, Zaslavsky A, Fedele G, McLaughlin SK, Reczek EE et al. (2010). An oncogene-tumor suppressor cascade drives metastatic prostate cancer by coordinately activating Ras and nuclear factor-κB. Nature Medicine 16: 286–294. https://doi.org/10.1038/nm.2100 [Google Scholar] [PubMed] [CrossRef]
Mosaddeghzadeh N, Ahmadian MR (2021). The RHO family GTPases: Mechanisms of regulation and signaling. Cells 10: 1831. https://doi.org/10.3390/cells10071831 [Google Scholar] [PubMed] [CrossRef]
Qiu L, Feng H, Yu H, Li M, You Y, Zhu S, Yang W, Jiang H, Wu X (2022). Characterization of the genomic landscape in cervical cancer by next generation sequencing. Genes 13: 287. https://doi.org/10.3390/genes13020287 [Google Scholar] [PubMed] [CrossRef]
Reshmi G, Pillai MR (2008). Beyond HPV: Oncomirs as new players in cervical cancer. FEBS Letters 582: 4113–4116. https://doi.org/10.1016/j.febslet.2008.11.011 [Google Scholar] [PubMed] [CrossRef]
Rieger AM, Nelson KL, Konowalchuk JD, Barreda DR (2011). Modified annexin V/propidium iodide apoptosis assay for accurate assessment of cell death. Journal of Visualized Experiments 50: 2597. https://doi.org/10.3791/2597 [Google Scholar] [PubMed] [CrossRef]
Sawaya GF, Huchko MJ (2017). Cervical cancer screening. Medical Clinics of North America 101: 743–753. https://doi.org/10.1016/j.mcna.2017.03.006 [Google Scholar] [PubMed] [CrossRef]
Sears R, Gray JW (2017). Epigenomic inactivation of RasGAPs Activates RAS signaling in a subset of luminal B breast cancers. Cancer Discovery 7: 131–133. https://doi.org/10.1158/2159-8290.CD-16-1423 [Google Scholar] [PubMed] [CrossRef]
Shen J, Wang Y, Hung MC (2013). RASAL2: Wrestling in the combat of Ras activation. Cancer Cell 24: 277–279. https://doi.org/10.1016/j.ccr.2013.08.024 [Google Scholar] [PubMed] [CrossRef]
Sung H, Ferlay J, Siegel RL, Laversanne M, Soerjomataram I, Jemal A, Bray F (2021). Global cancer statistics 2020: GLOBOCAN estimates of incidence and mortality worldwide for 36 cancers in 185 countries. CA: A Cancer Journal for Clinicians 71: 209–249. https://doi.org/10.3322/caac.21660 [Google Scholar] [PubMed] [CrossRef]
Tatli O, Dinler Doganay G (2021). Recent developments in targeting RAS downstream effectors for RAS-driven cancer therapy. Molecules 26: 7561. https://doi.org/10.3390/molecules26247561 [Google Scholar] [PubMed] [CrossRef]
van Zijl F, Krupitza G, Mikulits W (2011). Initial steps of metastasis: Cell invasion and endothelial transmigration. Mutation Research 728: 23–34. https://doi.org/10.1016/j.mrrev.2011.05.002 [Google Scholar] [PubMed] [CrossRef]
Wang L, Ji XB, Wang LH, Xia ZK, Xie YX, Liu WJ, Qiu JG, Jiang BH, Liu LZ (2021). MiRNA-30e downregulation increases cancer cell proliferation, invasion and tumor growth through targeting RPS6KB1. Sedentary Life and Nutrition 13: 24037–24049. https://doi.org/10.18632/aging.203665 [Google Scholar] [PubMed] [CrossRef]
Wang Z, Wang J, Su Y, Zeng Z (2015). RASAL2 inhibited the proliferation and metastasis capability of nasopharyngeal carcinoma. International Journal of Clinical and Experimental Medicine 8: 18765–18771. [Google Scholar] [PubMed]
Wang X, Yin X, Yang Y (2019). Rasal2 suppresses breast cancer cell proliferation modulated by secretory autophagy. Molecular and Cellular Biochemistry 462: 115–122. https://doi.org/10.1007/s11010-019-03615-7 [Google Scholar] [PubMed] [CrossRef]
Wang L, Yu S, Yin Y, Hao Y (2020). STAT6 correlates with response to immune checkpoint blockade therapy and predicts worse survival in thyroid cancer. Biomarkers in Medicine 14: 955–967. https://doi.org/10.2217/bmm-2020-0125 [Google Scholar] [PubMed] [CrossRef]
Wassie M, Argaw Z, Tsige Y, Abebe M, Kisa S (2019). Survival status and associated factors of death among cervical cancer patients attending at Tikur Anbesa Specialized Hospital, Addis Ababa, Ethiopia: A retrospective cohort study. BMC Cancer 19: 1221. https://doi.org/10.1186/s12885-019-6447-x [Google Scholar] [PubMed] [CrossRef]
Weeks A, Okolowsky N, Golbourn B, Ivanchuk S, Smith C, Rutka JT (2012). ECT2 and RASAL2 mediate mesenchymal-amoeboid transition in human astrocytoma cells. The American Journal of Pathology 181: 662–674. https://doi.org/10.1016/j.ajpath.2012.04.011 [Google Scholar] [PubMed] [CrossRef]
Wipperman J, Neil T, Williams T (2018). Cervical cancer: Evaluation and management. American Family Physician 97: 449–454. [Google Scholar] [PubMed]
Xu Y, Deng Y, Ji Z, Liu H, Liu Y, Peng H, Wu J, Fan J (2014). Identification of thyroid carcinoma related genes with mRMR and shortest path approaches. PLoS One 9: e94022. https://doi.org/10.1371/journal.pone.0094022 [Google Scholar] [PubMed] [CrossRef]
Xu F, Shangguan X, Pan J, Yue Z, Shen K et al. (2021). HOXD13 suppresses prostate cancer metastasis and BMP4-induced epithelial-mesenchymal transition by inhibiting SMAD1. International Journal of Cancer 148: 3060–3070. https://doi.org/10.1002/ijc.33494 [Google Scholar] [PubMed] [CrossRef]
Ye H, Zhang Y, Geng L, Li Z (2015). Cdc42 expression in cervical cancer and its effects on cervical tumor invasion and migration. International Journal of Oncology 46: 757–763. https://doi.org/10.3892/ijo.2014.2748 [Google Scholar] [PubMed] [CrossRef]
Yin L, Xiao X, Georgikou C, Luo Y, Liu L, Gladkich J, Gross W, Herr I (2019). Sulforaphane induces miR135b-5p and its target gene, RASAL2, thereby inhibiting the progression of pancreatic cancer. Molecular Therapy Oncolytics 14: 74–81. https://doi.org/10.1016/j.omto.2019.03.011 [Google Scholar] [PubMed] [CrossRef]
Zagouri F, Sergentanis TN, Chrysikos D, Filipits M, Bartsch R (2012). Molecularly targeted therapies in cervical cancer. A systematic review. Gynecologic Oncology 126: 291–303. https://doi.org/10.1016/j.ygyno.2012.04.007 [Google Scholar] [PubMed] [CrossRef]
Zhang S, Xu H, Zhang L, Qiao Y (2020). Cervical cancer: Epidemiology, risk factors and screening. Chinese Journal of Cancer Research 32: 720–728. https://doi.org/10.21147/j.issn.1000-9604.2020.06.05 [Google Scholar] [PubMed] [CrossRef]
Zhao M, Zhao X, Li Z, Ren L (2016). Effects of RASAL2 on invasion and epithelial-masenchymal transition of gastric cancer. Chinese Journal of Clinical Oncology 43: 691–696. https://doi.org/10.3969/j.issn.1000-8179.2016.16.726 [Google Scholar] [CrossRef]
Zhou B, Zhu W, Jiang X, Ren C (2019). RASAL2 plays inconsistent roles in different cancers. Frontiers in Oncology 9: 1235. https://doi.org/10.3389/fonc.2019.01235 [Google Scholar] [PubMed] [CrossRef]
Cite This Article
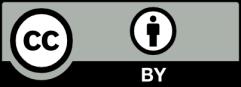
This work is licensed under a Creative Commons Attribution 4.0 International License , which permits unrestricted use, distribution, and reproduction in any medium, provided the original work is properly cited.