Open Access
ARTICLE
Zinc alpha 2 glycoprotein (ZAG): A potential novel pharmacological target in diabetic retinopathy
1 R. S. Mehta Jain Department of Biochemistry and Cell Biology, KNBIRVO, Vision Research Foundation, Sankara Nethralaya, Chennai, 600006, India
2 Shri Bhagwan Mahavir Vitreoretinal Services, Sankara Nethralaya, Chennai, 600006, India
* Corresponding Author: Subramaniam Rajesh Bharathidevi,
(This article belongs to the Special Issue: Genetic Polymorphism and Metabolic inflammation in Development of Insulin Resistance and Metabolic Syndrome)
BIOCELL 2023, 47(7), 1473-1482. https://doi.org/10.32604/biocell.2023.027804
Received 03 January 2023; Accepted 14 April 2023; Issue published 21 June 2023
Abstract
Zinc alpha 2 glycoprotein (ZAG) is a 41 KDa secretory soluble glycoprotein found in different body fluids like the serum, saliva, sweat, breast milk, and urine. It is also found in tissues like the testis, epididymis, kidney, spleen, liver, lungs, heart, and brain. ZAG is an adipokine with multiple roles, including lipid mobilization, modulating glucose metabolisms, improving insulin sensitivity, inhibiting tumor proliferation through RNAse activity, and suppressing inflammation. Low levels of zinc and ZAG are linked to metabolic syndrome and are also reported as potential biomarkers for diabetic nephropathy. Interestingly zinc has been found to regulate the binding of ZAG to fatty acids. Based on very few reports on the vitreous ZAG and based on its known functions, we speculate that ZAG has a potential role in diabetic retinopathy. In this article, we discuss the structural component of the protein, its secretion from various tissues, and its distribution in multiple tissues in normal and disease conditions, especially in diabetes and its complications.Keywords
Zinc is an essential trace element engaged in the body’s growth and development, as well as the metabolism of lipids, proteins, and carbohydrates. It controls the activation and expression of over 300 metalloenzymes. Zinc deficiency leads to impaired growth, reproduction, and immunity in humans and animals (Hara et al., 2017; Huang et al., 2017; Olechnowicz et al., 2018; McCall et al., 2000). Zinc is a regulator of zinc alpha 2 glycoprotein (ZAG), a multifunctional glycoprotein (Severo et al., 2020). ZAG has two strong and 15 weak sites for zinc binding. The attachment of zinc to ZAG enables fatty acids and β-adrenergic receptors to bind (Kumar et al., 2013; Zahid et al., 2016; Wei et al., 2019; Severo et al., 2020). Recently ZAG protein has been reported to have a role in the development of many systemic diseases, including diabetes and diabetic nephropathy. This review discusses the structure, function, and role of ZAG in several diseases. We have also speculated the possible role of this protein as a therapeutic target in diabetic retinopathy.
Structure of Human Zinc Alpha 2 Glycoprotein
ZAG is a 41 kDa glycoprotein named after it tended to precipitate with zinc and its electrophoretic migration is similar to that of α2-globulins (Schmitt et al., 2008). ZAG resembles the major histocompatibility complex (MHC) class I protein and shares 30% to 40% of amino acids sequence identity with the MHC complex heavy chain. It contains three domains, α1, α2, and α3 domains, and is not anchored to plasma membranes, being a soluble protein and does not associate with α2-microglobulin (Sánchez et al., 1999). ZAG’s ligand binding site is predominately occupied by polar amino acid side chains, except for a prominent arginine (Arg-73) that protrudes from the side of the groove and acts as a strut to hold the groove open (McDermott et al., 2006). ZAG has two different fatty acid binding sites similar to those seen in the cluster of differentiation-1e (CD1e) (Blumberg et al., 1995). ZAG groove binds hydrophobic ligands such as polyunsaturated fatty acids (Kennedy et al., 2001; Delker et al., 2004). The first crystal structure of ZAG, bound to Dancy amino undecanoic acid (DAUDA), has identified that the ZAG α1-α2 groove was flexible and able to accommodate DAUDA in two different positions as in Fig. 1 (Zahid et al., 2016; Lau et al., 2019). Further, Zahid et al. (2022) also revealed that the whole ZAG groove is dynamic in lipid binding and can lodge a broader range of signaling lipids, and its function is dictated by its bound lipids.
Figure 1: The cartoon representation of human zinc-alpha 2-glycoprotein bound to fatty acid.
The 11-{[5-(dimethylamino) naphthalen-1-yl] sulfonyl} amino undecanoic acid (11D) in complex with Chain B (blue color), chain C (pink color) shown as sticks in yellow color, and the azide ion (AZI) is shown as orange spheres.
Site of Zinc Alpha 2 Glycoprotein Expression
ZAG was first identified in 1961 in human blood (Burgi and Schmid, 1961). Epithelial cells in the liver synthesize and secrete ZAG. Jirka and Blanický (1980) identified three isoforms of ZAG in normal human serum in 1980 through crossed starch gel immune electrophoresis. Other than serum, it is reported in various body fluids like sweat, saliva, cerebrospinal fluids, milk (Bundred et al., 1987), urine (Jain et al., 2005), and amniotic fluid (Ding et al., 1990). It is expressed in several human tissues like the kidney, spleen, and liver (Table 1). Its level increases from lowest to highest from children to adults (Jirka and Blanický, 1980). ZAG plasma levels range from 8–12 mg/dL in young people to 18–30 mg/dL in healthy adults (Cabassi and Tedeschi, 2013). ZAG is reported to act in both autocrine and paracrine modes (Mracek et al., 2010).
The Role of Zinc Alpha 2 Glycoprotein in Energy Metabolism
ZAG expression is induced by glucocorticoids, adrenergic receptor agonists, thyroid hormones, and growth hormone in adipocytes. ZAG stimulates the expression of peroxisome proliferator-activated receptor (PPAR) and early B cell factor 2, which binds to PR SET Domain 16 (PRDM16) and uncoupling protein-1 (UCP-1) initiates browning of adipocytes (Elattar et al., 2018). The ZAG-induced increase in UCP-1 in brown adipose tissue helps modulate the body temperature and decreases body weight and fat (Sanders and Tisdale, 2004; Tisdale, 2009; Russell and Tisdale, 2011a; Xiao et al., 2018). ZAG also helps in the up-regulation of thermogenin through adrenergic receptor activation (Bao et al., 2005; Stejskal et al., 2008). Other than this, adipose triglyceride lipase (ATGL), hormone-sensitive lipase (HSL), carnitine palmitoyl transferase I (CPT1-A), and p-acyl-CoA carboxylase are modulated by ZAG, which leads to browning of adipocytes and increases energy expenditure (Xiao et al., 2018). ZAG activates various proteins such as peroxisome proliferator-activated receptor gamma coactivator 1-alpha (PGC-1), nuclear respiratory factor 1 and 2 (NRF-1/2), human mitochondrial transcription factor A, which modulates the mitochondrial energetics (Scarpulla et al., 2012). These entire factors ultimately lead to increased lipolysis (Fig. 2).
Figure 2: Stimulation of zinc alpha 2 glycoprotein (ZAG) expression in the physiological state. In the physiological state glucocorticoids, thyroid and growth hormones stimulate zinc alpha 2 glycoprotein (ZAG). The secreted ZAG is the main player in converting white adipose tissue (WAT) into brown adipose tissue (BAT). Conversion of WAT into BAT increases ZAG leve; increased ZAG stimulates the expression of PPARγ and early B cell factor 2 (EBCF2), which binds to the promoter region of PRDM16 and UCP1 and converts WAT into BAT and increases the lipolysis activity. Increased ZAG also modulates protein kinase A and p38/MAPK signaling pathways and enhances lipolysis-related molecules like PRDM16, UCP1, ATGL, mtTFA, CIDEA, HSL, NRF-1/2, and CPT1A and increases the lipolysis activity.
Zinc Alpha 2 Glycoprotein as A Regulator of Lipid Metabolism
ZAG plays a significant role in multiple ways to regulate the fat mass of adipose tissue. It stimulates lipolysis, inhibits lipid accumulation in adipocytes, and regulates serum lipids by influencing adipokines. Zinc and ZAG levels are reduced in the blood of people with excessive body fat (Marreiro et al., 2006; de Luis et al., 2013; Suliburska et al., 2013; Yerlikaya et al., 2013; Severo et al., 2020) and are thought to play a role in the development of diabetes and obesity (Fukunaka and Fujitani, 2018). Human recombinant ZAG administration to ob/ob mice has shown reduced body weight and fat content and a 30% reduction in adipose mass (Hirai et al., 1998; Russell and Tisdale, 2010, 2011b). Further, mice deficient in ZAG showed reduced lipolysis even after treatment with FK-forskolin, IBMX-isobutyl methylxanthine, and isoprenaline (Banaszak et al., 2021).
ZAG regulates the metabolism of free fatty acids released during lipolysis (Marrades et al., 2008) by stimulating fatty acid oxidation (Russell and Tisdale, 2002, 2010) Hepatocytes overexpressed with ZAG show augmented lipolysis and β oxidation of fatty acids and inhibit the palmitic acid-induced lipogenesis and lipid accumulation in hepatocytes (Xiao et al., 2017). Low ZAG level is reported as a risk factor for metabolic syndrome. A potential future diagnostic biomarker for metabolic syndrome is the serum ZAG to fat mass ratio. Further, ZAG in adipose tissue and skeletal muscle activates the AMPK pathway by up-regulating the GLUT-4 and UCP isoforms (Eckardt et al., 2011). When the body is in a catabolic condition, ZAG is activated, inducing lipolysis to increase the amount of free fatty acids that are accessible as an energy source for cancer, cachexia, or inadequate dietary intake. On the other hand, it is reported that increased serum leptin levels and chronic inflammation levels reduce ZAG secretion in the adipose tissue (Fig. 3).
Figure 3: Speculated role of ZAG in diabetic retinopathy. In a chronic Inflammation state, adipose tissue hypertrophy leads to increased levels of adiponectin (APN), interleukin (IL)6, IL8, monocyte chemoattractant protein-1 (MCP1), Regulated upon Activation, Normal T cell Expressed, and Secreted (RANTES), and leptin level to down-regulates the zinc alpha 2 glycoprotein (ZAG).
The Role of Zinc Alpha 2 Glycoprotein in Systemic Diseases
ZAG is modified in various pathologies like coronary artery disease, brain disorders, liver disorders, cancers, and in diabetes (Liu et al., 2019, 2020). Huang et al. (2019) suggested that ZAG exerts an anti-inflammatory effect by regulating the c-Jun N-terminal kinase/activator protein-1 signaling pathway and could be used as a therapeutic target for cardiovascular disease.
Krabbe’s disease is an autosomal recessive disorder primarily affecting infants, and epilepsy seen in Krabbe’s disease is associated with ZAG (Maślińska et al., 2013). Its expression is also decreased in temporal epilepsy of rats and humans (Liu et al., 2017). These studies indicate that ZAG has the potential to cross the blood-retinal barrier.
In liver tissues of non-alcoholic fatty liver disease (NAFLD), ZAG level increased in males compared to females and correlated with metabolic syndrome (Yilmaz et al., 2011). Another study found that ZAG protects against NAFLD by ameliorating hepatic steatosis, insulin resistance, and inflammation. Overexpression of ZAG inhibits lipogenesis and fatty acid β-oxidation and attenuates palmitic acid-induced fat accumulation. It is proposed to have the potential to alleviate hepatosteatosis (Xiao et al., 2017).
The concentration of ZAG has been reported to increase in carcinomas (Hirai et al., 1998) and is considered a promising biomarker for prostate (Henshall et al., 2006) and breast (Sánchez et al., 1992) carcinomas. It stimulates lipid degradation in adipocytes and causes extensive fat loss in advance stage cancers. In vivo, ZAG decreased tumor cell growth and melanin synthesis more than in vitro. Cyclin-dependent kinases are necessary rate-limiting enzymes in the cell cycle downregulated by ZAG, indicating the role of ZAG in hindering tumor progression (He et al., 2001).
The Role of Zinc Alpha 2 Glycoprotein in Diabetes
Intravenous administration of ZAG in mice has been shown to reduce blood glucose and improve glucose tolerance (Wargent et al., 2013). In oral glucose tolerance tests in mice, ZAG could increase urinary glucose excretion and decrease plasma glucose and insulin (Russell and Tisdale, 2012). ZAG also increases glucose uptake into adipocytes and augments glucose transporter 4 (GLUT4) via β1-adrenergic receptors (Ceperuelo-Mallafré et al., 2015). β-adrenergic receptors are proposed to play an essential role in ZAG-regulated glucose metabolism, but the specific mechanism is still under investigation. Studies on the relationship between ZAG and insulin resistance have shown controversial results (Yeung et al., 2009; Liao et al., 2016; Qu et al., 2016; Tian et al., 2016) with most of them being negative relationships. Silencing ZAG in adipocytes decreases adiponectin, insulin receptor substrate 1, GLUT4, and PGC1α expression (Balaz et al., 2014). Treatment with dapagliflozin, an antagonist of sodium-dependent glucose transporter 2, in type 2 diabetes mellitus (T2DM) patients has been shown to increase serum ZAG and alleviate IR (Selva et al., 2009; Wei et al., 2019). Further, whether the alterations in ZAG occur before the onset of dysglycemia or vice versa is still uncertain. Additionally, more work is required to understand if adiposity is the leading player in ZAG and dysglycemia (Pearsey et al., 2020). Interestingly, ZAG level is reported to decrease in gestational diabetic mellitus patients as well (Näf et al., 2012).
The Role of Zinc Alpha 2 Glycoprotein in Diabetic Nephropathy
ZAG has been proposed as an early diagnostic marker of diabetic nephropathy (Wang et al., 2016). In T2DM, Urine ZAG was higher than in serum due to secretion by tubular epithelial cells (Vallon and Thomson, 2012). However, a few other studies suggest that urine ZAG levels gradually increase in diabetic patients with standard micro and macroalbuminuria, indicating its relationship with the development of diabetes nephropathy (Nielsen et al., 2011). Additionally, according to other research, ZAG was first found in albumin-negative urine samples before the appearance of albumin in patients with T2DM from South Asia. This finding suggests that ZAG may be an early novel urinary biomarker for screening non-albuminuric diabetic nephropathy (Levey et al., 1999). Further, a positive correlation was observed between urinary and serum ZAG with the duration of diabetes mellitus. Further, compared to T2DM patients with normal estimated glomerular filtration rate (eGFR), patients with T2DM who had greater eGFR had significantly higher ZAG urine concentration (Elsheikh et al., 2019).
There are various reports on the levels of ZAG in the serum, plasma, and urine. In different disease conditions, the ZAG levels vary from 500 ng/mL–200 µg/mL in serum, plasma, and urine (Table 2). In diabetes mellitus, its range varies from 1 to 150 µg/mL in serum and 21 to 47 mg/L in plasma samples. In obesity, the range is 100 ng to 134 µg/mL in serum, whereas in chronic kidney disease, its range is 12 to 200 µg/mL; in the case of metabolic syndrome, it varies from 24 to 41 µg/mL. The variation in the serum ZAG level in the disease cases shows that the quantification of ZAG has to be further standardized in relevance to sensitivity and specificity. This is crucial to decipher the specific range indicating the disease progression. To our knowledge, there are no reports of ZAG in the serum of diabetic retinopathy patients.
Possible Role of Zinc Alpha 2 Glycoprotein in Diabetic Retinopathy
In DR, there is vascular leakage due to endothelial permeability induced by signaling pathways like p38, mitogen-activated protein kinase (MAPK), and nuclear factor-κB, which contribute to this by regulating claudin-5 expression (Adachi et al., 2012). Further, Sorrentino et al. (2007) showed that in vivo re-endothelialization capacity of endothelial progenitor cells derived from individuals with diabetes mellitus is severely impaired and is restored by peroxisome proliferator-activated receptor gamma (PPARγ) ligand rosiglitazone. ZAG has been shown to regulate PPARγ. The role of ZAG in diabetic retinopathy is not yet inferred. Proteomics studies in vitreous of cases proliferative diabetic retinopathy have reported altered ZAG expression (García-Ramírez et al., 2007; Zou et al., 2020). In our earlier study in retinal cells, we found ZAG downregulation with high glucose treatment (Vidhya et al., 2018). Zinc regulates ZAG, and the dyshomeostasis of zinc is reported in DR. Zinc is identified to prevent neovascularization by inhibiting VEGF expression in DR (Deniro and Al-Mohanna, 2012). Ischemia in diabetic retinopathy is a crucial component. Studies have shown zinc transporter 8 is reduced in ischemic insults. Therefore, zinc supplementation might have an adjuvant role in ischemia in proliferative diabetic retinopathy. The mechanism may be through ZAG, which needs further studies.
ZAG is a multifaceted glycoprotein and has been reported to decrease in diabetes and diabetic nephropathy. Few proteomic studies showed altered ZAG levels in the vitreous of diabetic retinopathy patients. Its definite mechanism and role in diabetic retinopathy are yet to be explored. ZAG is a metabolic regulator and lipolytic agent and modulates inflammatory genes further; it regulates PPARγ. PPARγ agonists are gaining importance as anti-diabetic drugs; therefore, we hypothesize that ZAG could be an essential player in the pathophysiology of diabetic retinopathy, and further studies are required to understand its mechanism in the disease progress. With all the gathered evidence, there are still lacunae on the role of ZAG in diabetic retinopathy. Further studies are required to unravel the possibility of ZAG as an independent or adjuvant pharmacological modulator for the treatment of diabetic retinopathy.
Acknowledgement: We thank Vision Research Foundation and Medical Research Foundation for the support.
Funding Statement: The grant was provided by the Indian Council of Medical Research (ICMR—(5/4/6/1/OPH/2020-NCD-II)).
Author Contributions: S. R. Bharathidevi conceived and organized the framework and contents of this paper. S. R. Bharathidevi and U. Prakash wrote the manuscript, and Ramya R. edited the paper. Rajiv Raman, Girish Shiva Rao, and Muna Bhende revised the final draft. All authors have read and approved the review.
Conflicts of Interest: The authors declare that they have no conflicts of interest to report regarding the present study.
References
Adachi T, Teramachi M, Yasuda H, Kamiya T, Hara H (2012). Contribution of p38 MAPK, NF-κB and glucocorticoid signaling pathways to ER stress-induced increase in retinal endothelial permeability. Archives of Biochemistry and Biophysics 520: 30–35. https://doi.org/10.1016/j.abb.2012.01.014 [Google Scholar] [PubMed] [CrossRef]
Alipoor E, Yaseri M, Mehrdadi P, Mahdavi-Mazdeh M, Murphy T, Hosseinzadeh-Attar MJ (2020). The relationship between serum adipokines and glucose homeostasis in normal-weight and obese patients on hemodialysis: A preliminary study. International Urology and Nephrology 52: 2179–2187. https://doi.org/10.1007/s11255-020-02582-z [Google Scholar] [PubMed] [CrossRef]
Balaz M, Vician M, Janakova Z, Kurdiova T, Surova M et al. (2014). Subcutaneous adipose tissue zinc-α2-glycoprotein is associated with adipose tissue and whole-body insulin sensitivity. Obesity 22: 1821–1829. https://doi.org/10.1002/oby.20764 [Google Scholar] [PubMed] [CrossRef]
Banaszak M, Górna I, Przysławski J (2021). Zinc and the innovative Zinc-α2-glycoprotein adipokine play an important role in lipid metabolism: A critical review. Nutrients 13: 2023. https://doi.org/10.3390/nu13062023 [Google Scholar] [PubMed] [CrossRef]
Bao Y, Bing C, Hunter L, Jenkins JR, Wabitsch M, Trayhurn P (2005). Zinc-α2-glycoprotein, a lipid mobilizing factor, is expressed and secreted by human (SGBS) adipocytes. FEBS Letters 579: 41–47. https://doi.org/10.1016/j.febslet.2004.11.042 [Google Scholar] [PubMed] [CrossRef]
Blumberg RS, Gerdes D, Chott A, Porcelli SA, Balk SP (1995). Structure and function of the CD1 family of MHC-like cell surface proteins. Immunological Reviews 147: 5–29. https://doi.org/10.1111/j.1600-065X.1995.tb00085.x [Google Scholar] [PubMed] [CrossRef]
Bundred NJ, Miller WR, Walker RA (1987). An immunohistochemical study of the tissue distribution of the breast cyst fluid protein, zinc alpha2 glycoprotein. Histopathology 11: 603–610. https://doi.org/10.1111/j.1365-2559.1987.tb02670.x [Google Scholar] [PubMed] [CrossRef]
Burgi W, Schmid K (1961). Preparation and properties of Zn-α2-glycoprotein of normal human plasma. Journal of Biological Chemistry 236: 1066–1074. https://doi.org/10.1016/S0021-9258(18)64243-7 [Google Scholar] [CrossRef]
Cabassi A, Tedeschi S (2013). Zinc-α2-glycoprotein as a marker of fat catabolism in humans. Current Opinion in Clinical Nutrition & Metabolic Care 16: 267–271. https://doi.org/10.1097/MCO.0b013e32835f816c [Google Scholar] [PubMed] [CrossRef]
Ceperuelo-Mallafré V, Ejarque M, Duran X, Pachón G, Vázquez-Carballo A et al. (2015). Zinc-α2-glycoprotein modulates AKT-dependent insulin signaling in human adipocytes by activation of the PP2A phosphatase. PLoS One 10: e0129644. https://doi.org/10.1371/journal.pone.0129644 [Google Scholar] [PubMed] [CrossRef]
Chan GC, Then WH, Kwan BC, Lai KB, Chan RC et al. (2022). Adipose and serum zinc alpha-2-glycoprotein (ZAG) expressions predict longitudinal change of adiposity, wasting and predict survival in dialysis patients. Scientific Reports 12: 9087. https://doi.org/10.1038/s41598-022-13149-6 [Google Scholar] [PubMed] [CrossRef]
de Luis DA, Pacheco D, Izaola O, Terroba MC, Cuellar L, Cabezas G (2013). Micronutrient status in morbidly obese women before bariatric surgery. Surgery of Obesity and Related Diseases 9: 323–327. https://doi.org/10.1016/j.soard.2011.09.015 [Google Scholar] [PubMed] [CrossRef]
Delker SL, West Jr AP, McDermott L, Kennedy MW, Bjorkman PJ (2004). Crystallographic studies of ligand binding by Zn-alpha2-glycoprotein. Journal of Structural Biology 148: 205–213. https://doi.org/10.1016/j.jsb.2004.04.009 [Google Scholar] [PubMed] [CrossRef]
Deniro M, Al-Mohanna FA (2012). Zinc transporter 8 (ZnT8) expression is reduced by ischemic insults: A potential therapeutic target to prevent ischemic retinopathy. PLoS One 7: e50360. https://doi.org/10.1371/journal.pone.0050360 [Google Scholar] [PubMed] [CrossRef]
Ding M, Umetsu K, Nakayashiki N, Choi WY, Jia J, Suzuki T (1990). Distribution of human Zn-alpha-2-glycoprotein types in Chinese and Korean populations. Human Heredity 40: 311–312. https://doi.org/10.1159/000153952 [Google Scholar] [PubMed] [CrossRef]
Eckardt K, Schober A, Platzbecker B, Mracek T, Bing C, Trayhurn P, Eckel J (2011). The adipokine zinc-α2-glycoprotein activates AMP kinase in human primary skeletal muscle cells. Archives Physiology Biochemistry 117: 88–93. https://doi.org/10.3109/13813455.2011.560950 [Google Scholar] [PubMed] [CrossRef]
Elattar S, Dimri M, Satyanarayana A (2018). The tumor secretory factor ZAG promotes white adipose tissue browning and energy wasting. Federation of American Societies for Experimental Biology of journal 32: 4727–4743. https://doi.org/10.1096/fj.201701465RR [Google Scholar] [PubMed] [CrossRef]
Elsheikh M, Elhefnawy KA, Emad G, Ismail M, Borai M (2019). Zinc alpha 2 glycoprotein as an early biomarker of diabetic nephropathy in patients with type 2 diabetes mellitus. Brazilian Journal of Nephrology 41: 509–517. https://doi.org/10.1590/2175-8239-jbn-2018-0200 [Google Scholar] [PubMed] [CrossRef]
Frenette G, Dubé JY, Lazure C, Paradis G, Chrétien M, Tremblay RR (1987). The major 40-kDa glycoprotein in human prostatic fluid is identical to Zn-α2-glycoprotein. Prostate 11: 257–270. https://doi.org/10.1002/pros.2990110306 [Google Scholar] [PubMed] [CrossRef]
Fukunaka A, Fujitani Y (2018). Role of zinc homeostasis in the pathogenesis of diabetes and obesity. International Journal of Molecular Sciences 19: 476. https://doi.org/10.3390/ijms19020476 [Google Scholar] [PubMed] [CrossRef]
García-Ramírez M, Canals F, Hernández C, Colomé N, Ferrer C, Carrasco E, García-Arumí J, Simó R (2007). Proteomic analysis of human vitreous fluid by fluorescence-based difference gel electrophoresis (DIGEA new strategy for identifying potential candidates in the pathogenesis of proliferative diabetic retinopathy. Diabetologia 50: 1294–1303. https://doi.org/10.1007/s00125-007-0627-y [Google Scholar] [PubMed] [CrossRef]
Garrido-Sánchez L, García-Fuentes E, Fernández-García D, Escoté X, Alcaide J, Perez-Martinez P, Vendrell J, Tinahones FJ (2012). Zinc-alpha 2-glycoprotein gene expression in adipose tissue is related with insulin resistance and lipolytic genes in morbidly obese patients. PLoS One 7: e33264. https://doi.org/10.1371/journal.pone.0033264 [Google Scholar] [PubMed] [CrossRef]
Gerhard HS, Haupt H (1981). Purified human plasma proteins of unknown function. Japanese Journal of Medical Science and Biology 34: 299–327. https://doi.org/10.7883/yoken1952.34.299 [Google Scholar] [PubMed] [CrossRef]
Hara T, Takeda TA, Takagishi T, Fukue K, Kambe T, Fukada T (2017). Physiological roles of zinc transporters: Molecular and genetic importance in zinc homeostasis. Journal of Physiological Sciences 67: 283–301. https://doi.org/10.1007/s12576-017-0521-4 [Google Scholar] [PubMed] [CrossRef]
He N, Brysk H, Tyring SK, Ohkubo I, Brysk MM (2001). Zinc-alpha (2)-glycoprotein hinders cell proliferation and reduces cdc2 expression. Journal of Cellular Biochemistry 36: 162–169. [Google Scholar]
He S, He Y, Jin F, Liu Y (2021). Correlation analysis of IGF-1, ZAG, nesfatin-1, HbA1c levels, and type 2 diabetes mellitus complicated with hypothyroidism. Medicine 100: e25432. https://doi.org/10.1097/MD.0000000000025432 [Google Scholar] [PubMed] [CrossRef]
Henshall SM, Horvath LG, Quinn DI, Eggleton SA, Grygiel JJ, Stricker PD, Biankin AV, Kench JG, Sutherland RL (2006). Zinc-alpha2-glycoprotein expression as a predictor of metastatic prostate cancer following radical prostatectomy. Journal of National Cancer Institute 98: 1420–1424. https://doi.org/10.1093/jnci/djj378 [Google Scholar] [PubMed] [CrossRef]
Hirai K, Hussey HJ, Barber MD, Price SA, Tisdale MJ (1998). Biological evaluation of a lipid-mobilizing factor isolated from the urine of cancer patients. Cancer Research 58: 2359–2365. [Google Scholar] [PubMed]
Hosseinzadeh-Attar MJ, Mahdavi-Mazdeh M, Yaseri M, Zahed NS, Alipoor E (2017). Comparative assessment of serum adipokines zinc-α2-glycoprotein and adipose triglyceride lipase, and cardiovascular risk factors between normal weight and obese patients with hemodialysis. Archives Medical Research 48: 459–466. https://doi.org/10.1016/j.arcmed.2017.10.004 [Google Scholar] [PubMed] [CrossRef]
Huang X, Jiang D, Zhu Y, Fang Z, Che L et al. (2017). Chronic high dose zinc supplementation induces visceral adipose tissue hypertrophy without altering body weight in mice. Nutrients 9: 1138. https://doi.org/10.3390/nu9101138 [Google Scholar] [PubMed] [CrossRef]
Huang D, Mao X, Peng J, Cheng M, Bai T et al. (2019). Role of adipokine zinc-α2-glycoprotein in coronary heart disease. American Journal os Physiology and Endocrinology Metabolism 317: E1055–E1062. https://doi.org/10.1152/ajpendo.00075.2019 [Google Scholar] [PubMed] [CrossRef]
Jain S, Rajput A, Kumar Y, Uppuluri N, Arvind AS, Tatu U (2005). Proteomic analysis of urinary protein markers for accurate prediction of diabetic kidney disorder. Journal of Association and Physicians of India 53: 513–520. [Google Scholar]
Jirka M, Blanický P (1980). Polymorphism of human serum Zn-alpha 2-glycoprotein and its behaviour during ontogenesis using quantitative crossed starch gel immune electrophoresis. International Journal of Clinical Chemistry 103: 61–66. https://doi.org/10.1016/0009-8981(80)90231-4 [Google Scholar] [PubMed] [CrossRef]
Kennedy MW, Heikema AP, Cooper A, Bjorkman PJ, Sanchez LM (2001). Hydrophobic ligand binding by Zn-α2-glycoprotein, a soluble fat-depleting factor related to major histocompatibility complex proteins. Journal of Biological Chemistry 276: 35008–35013. https://doi.org/10.1074/jbc.C100301200 [Google Scholar] [PubMed] [CrossRef]
Kraemer TD, Soerensen-Zender I, Memaran N, Haller H, Melk A, Schmidt BMW, Schmitt R (2021). Changes in AZGP1 serum levels and correlation with pulse wave velocity after kidney transplantation. Frontiers in Cardiovascular Medecine 8: 692213. https://doi.org/10.3389/fcvm.2021.692213 [Google Scholar] [PubMed] [CrossRef]
Kumar AA, Hati D, Thaker TM, Miah L, Cunningham P, Domene C, Bui TT, Drake AF, McDermott LC (2013). Strong and weak zinc binding sites in human zinc-α2-glycoprotein. Federation of European Biochemical Societies Letter 587: 3949–3954. https://doi.org/10.1016/j.febslet.2013.10.026 [Google Scholar] [PubMed] [CrossRef]
Lau AM, Zahid H, Gor J, Perkins SJ, Coker AR, McDermott LC (2019). Crystal structure of zinc-α2-glycoprotein in complex with a fatty acid reveals multiple different modes of protein-lipid binding. Journal of Biochenistry 476: 2815–2834. https://doi.org/10.1042/BCJ20190354 [Google Scholar] [PubMed] [CrossRef]
Leal VO, Lobo JC, Stockler-Pinto MB, Farage NE, Abdalla DS, Leite Jr M, Mafra D (2012). Is zinc-α2-glycoprotein a cardiovascular protective factor for patients undergoing hemodialysis? International journal of Clinical Chemistry 413: 616–619. https://doi.org/10.1016/j.cca.2011.12.002 [Google Scholar] [PubMed] [CrossRef]
Lei L, Li K, Li L, Fang X, Zhou T et al. (2017). Circulating zinc-α2-glycoprotein levels are low in newly diagnosed patients with metabolic syndrome and correlate with adiponectin. Nutrition Metabolism 14: 53. https://doi.org/10.1186/s12986-017-0210-6 [Google Scholar] [PubMed] [CrossRef]
Levey AS, Bosch JP, Lewis JB, Greene T, Rogers N, Roth D (1999). A more accurate method to estimate glomerular filtration rate from serum creatinine: A new prediction equation. Modification of diet in renal disease study group. Annals of International Medecine 130: 461–470. https://doi.org/10.7326/0003-4819-130-6-199903160-00002 [Google Scholar] [PubMed] [CrossRef]
Liao X, Wang X, Li H, Li L, Zhang G, Yang M, Yuan L, Liu H, Yang G, Gao L (2016). Sodium-Glucose Cotransporter 2 (SGLT2) inhibitor increases circulating zinc-Α2-glycoprotein levels in patients with type 2 diabetes. Scitific Reports 6: 32887. https://doi.org/10.1038/srep32887 [Google Scholar] [PubMed] [CrossRef]
Liu M, Liu Z, Zhu H, Wang L, Yang H, Yan K, Gong F, Pan H, Zeng Y (2020). Serum Zinc-α2-glycoprotein levels in patients with or without coronary artery disease in Chinese north population. International Journal of Endocrinol 2020: 7864721. https://doi.org/10.1155/2020/7864721 [Google Scholar] [PubMed] [CrossRef]
Liu Y, Wang T, Liu X, Wei X, Xu T, Yin M, Ding X, Mo L, Chen L (2017). Neuronal zinc-α2-glycoprotein is decreased in temporal lobe epilepsy in patients and rats. Neuroscience 357: 56–66. https://doi.org/10.1016/j.neuroscience.2017.05.043 [Google Scholar] [PubMed] [CrossRef]
Liu M, Zhu H, Dai Y, Pan H, Li N, Wang L, Yang H, Yan K, Gong F (2018). Zinc-α2-glycoprotein is associated with obesity in chinese people and HFD-induced obese mice. Frontiers in Physiology 9: 62. https://doi.org/10.3389/fphys.2018.00062 [Google Scholar] [PubMed] [CrossRef]
Liu M, Zhu H, Zhai T, Pan H, Wang L, Yang H, Yan K, Zeng Y, Gong F (2019). Serum zinc-α2-glycoprotein levels were decreased in patients with premature coronary artery disease. Frontiers in Endocrinology 10: 197. https://doi.org/10.3389/fendo.2019.00197 [Google Scholar] [PubMed] [CrossRef]
Marrades MP, Martínez JA, Moreno-Aliaga MJ (2008). ZAG, a lipid mobilizing adipokine, is downregulated in human obesity. Journal of Physiology and Biochemistry 64: 61–66. https://doi.org/10.1007/BF03168235 [Google Scholar] [PubMed] [CrossRef]
Marreiro DN, Geloneze B, Tambascia MA, Lerário AC, Halpern A, Cozzolino SM (2006). Effect of zinc supplementation on serum leptin levels and insulin resistance of obese women. Biological Trace Element Research 112: 109–118. https://doi.org/10.1385/BTER:112:2:109 [Google Scholar] [PubMed] [CrossRef]
Maślińska D, Laure-Kamionowska M, Maśliński S (2013). Crosstalk in human brain between globoid cell leucodystrophy and zinc-α-2-glycoprotein (ZAGa biomarker of lipid catabolism. Folia Neuropathologica 51: 312–318. https://doi.org/10.5114/fn.2013.39720 [Google Scholar] [PubMed] [CrossRef]
McCall KA, Huang C, Fierke CA (2000). Function and mechanism of zinc metalloenzymes. Journal of Nutrition 130: 1437S–1446S. https://doi.org/10.1093/jn/130.5.1437S [Google Scholar] [PubMed] [CrossRef]
McDermott LC, Freel JA, West AP, Bjorkman PJ, Kennedy MW (2006). Zn-alpha2-glycoprotein, an MHC class I-related glycoprotein regulator of adipose tissues: Modification or abrogation of ligand binding by site-directed mutagenesis. Biochemistry 45: 2035–2041. https://doi.org/10.1021/bi051881v [Google Scholar] [PubMed] [CrossRef]
Mracek T, Ding Q, Tzanavari T, Kos K, Pinkney J, Wilding J, Trayhurn P, Bing C (2010). The adipokine zinc-alpha2-glycoprotein (ZAG) is downregulated with fat mass expansion in obesity. Clinical Endocrinology 72: 334–341. https://doi.org/10.1111/j.1365-2265.2009.03658.x [Google Scholar] [PubMed] [CrossRef]
Nielsen SE, Andersen S, Zdunek D, Hess G, Parving HH, Rossing P (2011). Tubular markers do not predict the decline in glomerular filtration rate in type 1 diabetic patients with overt nephropathy. Kidney International 79: 1113–1118. https://doi.org/10.1038/ki.2010.554 [Google Scholar] [PubMed] [CrossRef]
Näf S, Escote X, Yañez RE, Ballesteros M, Simón I, Gil P, Megia A, Vendrell J (2012). Zinc-α2-glycoprotein is unrelated to gestational diabetes: Anthropometric and metabolic determinants in pregnant women and their offspring. PLoS One 7: e47601. https://doi.org/10.1371/journal.pone.0047601 [Google Scholar] [PubMed] [CrossRef]
Olechnowicz J, Tinkov A, Skalny A, Suliburska J (2018). Zinc status is associated with inflammation, oxidative stress, lipid, and glucose metabolism. Journal of Physiological Sciences 68: 19–31. https://doi.org/10.1007/s12576-017-0571-7 [Google Scholar] [PubMed] [CrossRef]
Pearsey HM, Henson J, Sargeant JA, Davies MJ, Khunti K, Suzuki T, Bowden-Davies KA, Cuthbertson DJ, Yates TE (2020). Zinc-alpha2-glycoprotein, dysglycaemia and insulin resistance: A systematic review and meta-analysis. Reviews of Endocrine and Metabolic Disorders 21: 569–575. https://doi.org/10.1007/s11154-020-09553-w [Google Scholar] [PubMed] [CrossRef]
Pelletier CC, Koppe L, Croze ML, Kalbacher E, Vella RE, Guebre-Egziabher F, Géloën A, Badet L, Fouque D, Soulage CO (2013). White adipose tissue overproduces the lipid-mobilizing factor zinc α2-glycoprotein in chronic kidney disease. Kidney International 83: 878–886. https://doi.org/10.1038/ki.2013.9 [Google Scholar] [PubMed] [CrossRef]
Philipp A, Kralisch S, Bachmann A, Lossner U, Kratzsch J, Blüher M, Stumvoll M, Fasshauer M (2011). Serum levels of the adipokine zinc-α2-glycoprotein are increased in chronic hemodialysis. Metabolism-Clinical and Experimental 60: 669–672. https://doi.org/10.1016/j.metabol.2010.06.019 [Google Scholar] [PubMed] [CrossRef]
Qu C, Zhou X, Yang G, Li L, Liu H, Liang Z (2016). The natural logarithm of zinc-α2-glycoprotein/HOMA-IR is a better predictor of insulin sensitivity than the product of triglycerides and glucose and the other lipid ratios. Cytokine 79: 96–102. https://doi.org/10.1016/j.cyto.2015.12.024 [Google Scholar] [PubMed] [CrossRef]
Russell ST, Tisdale MJ (2002). Effect of a tumour-derived lipid-mobilising factor on glucose and lipid metabolism in vivo. British Journal of Cancer 87: 580–584. https://doi.org/10.1038/sj.bjc.6600493 [Google Scholar] [PubMed] [CrossRef]
Russell ST, Tisdale MJ (2010). Antidiabetic properties of zinc-alpha2-glycoprotein in ob/ob mice. Endocrinology 151: 948–957. https://doi.org/10.1210/en.2009-0827 [Google Scholar] [PubMed] [CrossRef]
Russell ST, Tisdale MJ (2011a). Studies on the anti-obesity activity of zinc-α2-glycoprotein in the rat. International Journal of Obesity 35: 658–665. https://doi.org/10.1038/ijo.2010.193 [Google Scholar] [PubMed] [CrossRef]
Russell ST, Tisdale MJ (2011b). Studies on the antiobesity effect of zinc-α2-glycoprotein in the ob/ob mouse. International Journal of Obesity 35: 345–354. https://doi.org/10.1038/ijo.2010.150 [Google Scholar] [PubMed] [CrossRef]
Russell ST, Tisdale MJ (2012). Role of β-adrenergic receptors in the anti-obesity and anti-diabetic effects of zinc-α2-glycoprotien (ZAG). Biochimica et Biophysica Acta 1821: 590–599. https://doi.org/10.1016/j.bbalip.2011.12.003 [Google Scholar] [PubMed] [CrossRef]
Sanders PM, Tisdale MJ (2004). Effect of zinc-alpha2-glycoprotein (ZAG) on expression of uncoupling proteins in skeletal muscle and adipose tissue. Cancer Letter 212: 71–81. https://doi.org/10.1016/j.canlet.2004.03.021 [Google Scholar] [PubMed] [CrossRef]
Sánchez LM, Chirino AJ, Bjorkman PJ (1999). Crystal structure of human ZAG, a fat-depleting factor related to MHC molecules. Science 283: 1914–1919. https://doi.org/10.1126/science.283.5409.1914 [Google Scholar] [PubMed] [CrossRef]
Sánchez LM, Freije JP, Merino AM, Vizoso F, Foltmann B, López-Otín C (1992). Isolation and characterization of a pepsin C zymogen produced by human breast tissues. Journal of Biological Chemistry 267: 24725–24731. https://doi.org/10.1016/S0021-9258(18)35824-1 [Google Scholar] [CrossRef]
Scarpulla RC, Vega RB, Kelly DP (2012). Transcriptional integration of mitochondrial biogenesis. Trends in Endocrinology and Metabolism 23: 459–466. https://doi.org/10.1016/j.tem.2012.06.006 [Google Scholar] [PubMed] [CrossRef]
Schmitt R, Marlier A, Cantley LG (2008). Zag expression during aging suppresses proliferation after kidney injury. Journal of the American Society of Nephrology 19: 2375–2383. https://doi.org/10.1681/ASN.2008010035 [Google Scholar] [PubMed] [CrossRef]
Selva DM, Lecube A, Hernández C, Baena JA, Fort JM, Simó R (2009). Lower zinc-alpha2-glycoprotein production by adipose tissue and liver in obese patients unrelated to insulin resistance. Journal of Clinical Endocrinology and Metabolism 94: 4499–4507. https://doi.org/10.1210/jc.2009-0758 [Google Scholar] [PubMed] [CrossRef]
Severo JS, Morais JBS, Beserra JB, Dos Santos LR, de Sousa Melo SR, de Sousa GS, de Matos Neto EM, Henriques GS, do Nascimento Marreiro D (2020). Role of zinc in zinc-α2-glycoprotein metabolism in obesity: A review of literature. Biological Trace Elements Research 193: 81–88. https://doi.org/10.1007/s12011-019-01702-w [Google Scholar] [PubMed] [CrossRef]
Sorrentino SA, Bahlmann FH, Besler C, Müller M, Schulz S et al. (2007). Oxidant stress impairs in vivo reendothelialization capacity of endothelial progenitor cells from patients with type 2 diabetes mellitus: Restoration by the peroxisome proliferator-activated receptor-gamma agonist rosiglitazone. Circulation 116: 163–173. https://doi.org/10.1161/CIRCULATIONAHA.106.684381 [Google Scholar] [PubMed] [CrossRef]
Stejskal D, Karpísek M, Reutová H, Stejskal P, Kotolová H, Kollár P (2008). Determination of serum zinc-alpha-2-glycoprotein in patients with metabolic syndrome by a new ELISA. Clinical Biochemistry 41: 313–316. https://doi.org/10.1016/j.clinbiochem.2007.11.010 [Google Scholar] [PubMed] [CrossRef]
Suliburska J, Cofta S, Gajewska E, Kalmus G, Sobieska M, Samborski W, Krejpcio Z, Drzymala-Czyz S, Bogdanski P (2013). The evaluation of selected serum mineral concentrations and their association with insulin resistance in obese adolescents. European Review for Medical and Pharmacological Sciences 17: 2396–2400. [Google Scholar] [PubMed]
Sörensen-Zender I, Beneke J, Schmidt BM, Menne J, Haller H, Schmitt R (2013). Zinc-alpha2-glycoprotein in patients with acute and chronic kidney disease. BMC Nephrology 14: 145. https://doi.org/10.1186/1471-2369-14-145 [Google Scholar] [PubMed] [CrossRef]
Tada T, Ohkubo I, Niwa M, Sasaki M, Tateyama H, Eimoto T (1991). Immunohistochemical localization of Zn-alpha 2-glycoprotein in normal human tissues. Journal of Histochemistry and Cytochemistry 39: 1221–1226. https://doi.org/10.1177/39.9.1918940 [Google Scholar] [PubMed] [CrossRef]
Tian M, Liang Z, Liu R, Li K, Tan X et al. (2016). Effects of sitagliptin on circulating zinc-α2-glycoprotein levels in newly diagnosed type 2 diabetes patients: A randomized trial. European Journal of Endocrinol 174: 147–155. https://doi.org/10.1530/EJE-15-0637 [Google Scholar] [PubMed] [CrossRef]
Tisdale MJ (2009). Zinc-α2-glycoprotein in cachexia and obesity. Current Opinion in Supportive and Palliative Care 3: 288–293. https://doi.org/10.1097/SPC.0b013e328331c897 [Google Scholar] [PubMed] [CrossRef]
Vallon V, Thomson SC (2012). Renal function in diabetic disease models: The tubular system in the pathophysiology of the diabetic kidney. Annual Review of Physiology 74: 351–375. https://doi.org/10.1146/annurev-physiol-020911-153333 [Google Scholar] [PubMed] [CrossRef]
Vidhya S, Ramya R, Coral K, Sulochana KN, Bharathidevi SR (2018). Free amino acids hydroxyproline, lysine, and glycine promote differentiation of retinal pericytes to adipocytes: A protective role against proliferative diabetic retinopathy. Experimental Eye Research 173: 179–187. https://doi.org/10.1016/j.exer.2018.05.004 [Google Scholar] [PubMed] [CrossRef]
Wang Y, Li YM, Zhang S, Zhao JY, Liu CY (2016). Adipokine zinc-alpha-2-glycoprotein as a novel urinary biomarker presents earlier than microalbuminuria in diabetic nephropathy. Journal of International Medical Research 44: 278–286. https://doi.org/10.1177/0300060515601699 [Google Scholar] [PubMed] [CrossRef]
Wang L, Liu M, Ning D, Zhu H, Shan G et al. (2020). Low serum ZAG levels correlate with determinants of the metabolic syndrome in Chinese subjects. Frontiers in Endocrinology 11: 154. https://doi.org/10.3389/fendo.2020.00154 [Google Scholar] [PubMed] [CrossRef]
Wargent ET, O’Dowd JF, Zaibi MS, Gao D, Bing C, Trayhurn P, Cawthorne MA, Arch JR, Stocker CJ (2013). Contrasts between the effects of zinc-α2-glycoprotein, a putative β3/2-adrenoceptor agonist and the β3/2-adrenoceptor agonist BRL35135 in C57Bl/6 (ob/ob) mice. Journal of Endocrinology 216: 157–168. https://doi.org/10.1530/JOE-12-0402 [Google Scholar] [PubMed] [CrossRef]
Wei X, Liu X, Tan C, Mo L, Wang H, Peng X, Deng F, Chen L (2019). Expression and function of zinc-α2-glycoprotein. Neuroscience Bulletin 35: 540–550. https://doi.org/10.1007/s12264-018-00332-x [Google Scholar] [PubMed] [CrossRef]
Xiao X, Li H, Qi X, Wang Y, Xu C, Liu G, Wen G, Liu J (2017). Zinc alpha2 glycoprotein alleviates palmitic acid-induced intracellular lipid accumulation in hepatocytes. Molecular and Cellular Endocrinology 439: 155–164. https://doi.org/10.1016/j.mce.2016.06.003 [Google Scholar] [PubMed] [CrossRef]
Xiao XH, Qi XY, Wang YD, Ran L, Yang J, Zhang HL, Xu CX, Wen GB, Liu JH (2018). Zinc alpha2 glycoprotein promotes browning in adipocytes. Biochemical and Biophysical Research Communications 496: 287–293. https://doi.org/10.1016/j.bbrc.2018.01.039 [Google Scholar] [PubMed] [CrossRef]
Xu D, You J, Chen G, Su H, Zhang L, Cui L, Li Z, Huang G, Feng C (2021). Changes of serum zinc-α2-glycoprotein level and analysis of its related factors in gestational diabetes mellitus. Journal of Diabetes Research, 8879786. https://doi.org/10.1155/2021/8879786 [Google Scholar] [PubMed] [CrossRef]
Xu L, Yu W, Niu M, Zheng C, Qu B, Li Y, Wang J, Huang P, Wang O, Gong F (2017). Serum ZAG levels were associated with eGFR mild decrease in T2DM patients with diabetic nephropathy. International Journal of Endocrinology, 5372625. https://doi.org/10.1155/2017/5372625 [Google Scholar] [PubMed] [CrossRef]
Yang M, Liu R, Li S, Luo Y, Zhang Y et al. (2013). Zinc-α2-glycoprotein is associated with insulin resistance in humans and is regulated by hyperglycemia, hyperinsulinemia, or liraglutide administration: cross-sectional and interventional studies in normal subjects, insulin-resistant subjects, and subjects with newly diagnosed diabetes. Diabetes Care 36: 1074–1082. https://doi.org/10.2337/dc12-0940 [Google Scholar] [PubMed] [CrossRef]
Yerlikaya FH, Toker A, Arıbaş A (2013). Serum trace elements in obese women with or without diabetes. Indian Journal of Medical Research 137: 339–345. [Google Scholar] [PubMed]
Yeung DC, Lam KS, Wang Y, Tso AW, Xu A (2009). Serum zinc-alpha2-glycoprotein correlates with adiposity, triglycerides, and the key components of the metabolic syndrome in Chinese subjects. Journal of Clinical Endocrinology and Metabolism 94: 2531–2536. https://doi.org/10.1210/jc.2009-0058 [Google Scholar] [PubMed] [CrossRef]
Yilmaz Y, Yonal O, Eren F, Kurt R, Celikel CA, Ozdogan O, Imeryuz N, Kalayci C, Avsar E (2011). Serum zinc-α2-glycoprotein concentrations in patients with non-alcoholic fatty liver disease. Clinical Chemistry and Laboratory Medicine 49: 93–97. https://doi.org/10.1515/CCLM.2011.022 [Google Scholar] [PubMed] [CrossRef]
Zahid H, Lau AM, Kelly SM, Karu K, Gor J, Perkins SJ, McDermott LC (2022). Identification of diverse lipid-binding modes in the groove of zinc α2 glycoprotein reveals its functional versatility. Federation of European Biochemical Societies 289: 1876–1896. https://doi.org/10.1111/febs.16293 [Google Scholar] [PubMed] [CrossRef]
Zahid H, Miah L, Lau AM, Brochard L, Hati D, Bui TT, Drake AF, Gor J, Perkins SJ, McDermott LC (2016). Zinc-induced oligomerization of zinc α2 glycoprotein reveals multiple fatty acid-binding sites. Journal of Biochemistry 473: 43–54. https://doi.org/10.1042/BJ20150836 [Google Scholar] [PubMed] [CrossRef]
Zhu H, Liu M, Zhang N, Pan H, Lin G, Li N, Wang L, Yang H, Yan K, Gong F (2018). Circulating and adipose tissue mRNA levels of zinc-α2-glycoprotein, leptin, high-molecular-weight adiponectin, and tumor necrosis factor-alpha in colorectal cancer patients with or without obesity. Frontiers in Endocrinology 9: 190. https://doi.org/10.3389/fendo.2018.00190 [Google Scholar] [PubMed] [CrossRef]
Zhu HJ, Wang XQ, Pan H, Gong FY, Zhang DX, Li NS, Wang LJ, Yang HB (2014). Serum levels of the adipokine zinc-α2-glycoprotein are decreased in patients with hypertension. ISRN Endocrinology, 374090. https://doi.org/10.1155/2014/374090 [Google Scholar] [PubMed] [CrossRef]
Zou X, Wang S, Zhang P, Lu L, Zou H (2020). Quantitative proteomics and weighted correlation network analysis of tear samples in adults and children with diabetes and dry eye. Translational Vision Science & Technology 9: 8. https://doi.org/10.1167/tvst.9.13.8 [Google Scholar] [PubMed] [CrossRef]
Cite This Article
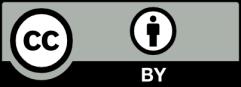