Open Access
ARTICLE
TianmaGouteng yin attenuates ischemic stroke-induced brain injury by inhibiting the AGE/RAGE pathway
Department of TCM Pharmacy, The First Affiliated Hospital of Zhejiang Chinese Medical University (Zhejiang Provincial Hospital of Chinese Medicine), Hangzhou, 310003, China
* Corresponding Author: DIWEN SHOU. Email:
(This article belongs to the Special Issue: Natural Products for Chronic Inflammatory Diseases: Pharmacology and Toxicology)
BIOCELL 2023, 47(6), 1345-1352. https://doi.org/10.32604/biocell.2023.028866
Received 13 January 2023; Accepted 16 February 2023; Issue published 19 May 2023
Abstract
Background: Ischemic stroke is characterized by permanent or transient obstruction of blood flow, leading to a growing risk factor and health burden. Tianmagouteng yin (TMG) is commonly used in Chinese medicine to treat cerebral ischemia. The aim of this study was to investigate the neuroprotective effects of TMG against ischemic stroke. Methods: Either permanent middle cerebral artery occlusion (pMCAO) or sham operation was performed on anesthetized Wistar male rats (n = 36). Results: Results demonstrated that TMG administration reduced the infarction volume and mitigated the neurobehavioral deficits. Hematoxylin and eosin (HE) staining and Prussian blue staining revealed that TMG attenuated tissue disruption and microbleeds in hippocampus tissues. In addition, TMG down-regulated the receptor of advanced glycation end products (RAGE) and p-JAK2. It also inhibited the concentrations of advanced glycation end products (AGEs), ferritin, malondialdehyde (MDA), and reactive oxygen species (ROS). Conclusion: As repetitive clinical trials of neuroprotectants targeting stroke have failed previously, our results suggested that the natural product, TMG, can probably help in the vicious cycles of ischemic stroke pathology.Keywords
Stroke is a major cause of physical disability and the second primary cause of global death, making up 5.2% of all deaths worldwide (Iadecola et al., 2020; Zhao et al., 2022b). The incidence of stroke is predicted to rise from about 3% of the US population in 2012 to about 4% by 2030, leading to an annual cost of approximately $200 billion (Iadecola et al., 2020). Stroke is mainly classified into ischemic or hemorrhagic stroke (Paul and Candelario-Jalil, 2021). Although the two types of stroke are severe and common, ischemic stroke is more prevalent, accounting for 87% of all stroke cases in the US (Barthels and Das, 2020). Ischemic stroke is caused by arterial blockages, commonly due to blood clots lodged in one of the arteries in the brain (Barthels and Das, 2020). Ischemic stroke and cerebral infarction can cause diverse degrees and types of brain injury, including cerebral tissue lesions and structural damage, and neuronal death and impairments (Zhao et al., 2022b). The pathological mechanisms of ischemic stroke are complicated. Oxidative stress and inflammation have been demonstrated to be the key mechanisms in the onset and development of ischemic stroke (Chen et al., 2020). Although neuroprotectants have exhibited therapeutic potential in experimental stroke models, the application of such neuroprotective treatments from animal models to humans is yet to yield a beneficial result (Paul and Candelario-Jalil, 2021). In the past few years, multiple neuroprotectants have been tested in clinical trials, however, the outcomes remain largely unknown. Unfortunately, molecules like Natalizumab and NA-1 have failed in phase II and phase III clinical trials, respectively (Paul and Candelario-Jalil, 2021). Currently, recombinant tissue plasminogen activator is still the only drug available for therapy for ischemic stroke (Tao et al., 2020). Nevertheless, individuals suffering from stroke symptoms must receive this treatment within 4.5 h or face the potential risk of hemorrhagic transformation, making it applicable to less than 10% of stroke patients (Barthels and Das, 2020; Tao et al., 2020). Therefore, it is imperative to develop more effective and practical therapeutic drugs and approaches for ischemic stroke.
Traditional Chinese herbal medicines have been found advantageous to attenuate or treat many diseases. So far, several traditional Chinese herbal formulas have exhibited their great potential to treat ischemic stroke such as BuyangHuanwu decoction (Gao et al., 2021) and Pien-Tze-Huang (Huang et al., 2021). Tianmagouteng yin (TMG) is a traditional Chinese herbal medicine, which is primarily composed of Gastrodia elata (Tianma) and Uncaria rhynchophylla (Gouteng). It is commonly used to treat cerebral ischemia and brain-related disorders (Xian et al., 2016). Extracts of Gastrodia elata or its active components possess various pharmacological effects, such as memory improving and neuroprotective activities (Heese, 2020). Besides, a recent study has revealed the neuroprotective effect of Uncaria rhynchophylla extracts on cerebral ischemia in rats (Deng et al., 2021). Although the neuroprotective role of TMG has been documented, the molecular mechanisms underlying TMG activity against ischemic stroke are still incompletely understood.
Advanced glycation end products (AGEs) and their receptor RAGE are implicated in the pathological process of neuro-inflammation in ischemic stroke complications (Liu et al., 2021). Interestingly, the latest network pharmacological analysis reveals the crucial role of the AGE/RAGE signaling pathway in anti-ischemic stroke mechanisms (Wang et al., 2023). The interplay between AGEs and cell surface receptors including RAGE can lead to the activation of certain signaling pathways and the overproduction of cytokines, chemokines, and other inflammatory mediators that ultimately trigger inflammation (Yu et al., 2018b). As a member of the immunoglobulin superfamily, RAGE is the most investigated receptor of AGEs. Its expression counts on the cell type and is modulated in reaction to the alterations of the extracellular environment (Yu et al., 2018b). Apart from AGEs, RAGE can bind with multiple ligands, such as high-mobility group box 1 and S100 proteins, resulting in pathological processes, including inflammation (Oshima et al., 2022). A recent study has indicated that inhibition of the AGE/RAGE axis could mitigate ischemic injury (Wang et al., 2021). However, the specific mechanisms of the AGE/RAGE pathway in anti-ischemic stroke and the role of TMG remain to be explored.
Janus kinase 2 (JAK2) is an important signal transduction protein that can be activated by ischemic stress (Zhong et al., 2021). Further, the interaction between RAGE and the JAK2 pathway has been revealed in research (Sun et al., 2022). Based on these facts, we hypothesized a possible crosstalk between RAGE and JAK2 underlying TMG alleviating ischemic stroke-induced injury.
In this work, we employed a permanent middle cerebral artery occlusion (pMCAO) model to figure out the neuroprotective effects of TMG in ischemic stroke. Besides, the oxidative stress mechanism and the AGE/RAGE pathway were explored. This study aimed at further investigating the therapeutic effects of TMG on brain injury induced by ischemic stroke and related mechanisms, thereby providing novel strategies for the treatment of ischemic stroke.
A total of 36 specific pathogen-free Wistar rats (male, 10–12 weeks old, 250–300 g) were obtained from GemPharmatech Co. Ltd., Nanjing, China. All rats were housed in cages under a 12-h light/dark cycle at a temperature of 22°C–25°C and a relative humidity of 40%–60% with free access to water and food. All experimental procedures were approved by the Institutional Animal Care and Use Committee, The First Affiliated Hospital of Zhejiang Chinese Medical University (Zhejiang Provincial Hospital of Traditional Chinese Medicine). Rats were anesthetized with isoflurane to perform pMCAO. The right common carotid artery (CCA), external carotid artery (ECA), and internal carotid artery (ICA) of rats were isolated through a cervical midline incision. The proximal CCA and distal ECA were ligated, meanwhile, a 4-0 nylon suture was inserted from the right CCA to the ICA and then to the circle of Willis for artery occlusion. Rats of the sham group were subjected to the same surgical procedures without artery occlusion treatment. The body temperature of rats was continually monitored and maintained at 37°C. All rats were sacrificed by chloroform anesthesia.
Drug administration and experimental groups
To determine the effects of TMG on ischemic stroke-induced brain injury, rats were arbitrarily separated into six groups (n = 6 per group): the sham + normal saline (NS) group, the model + NS group, the TMG + NS group, the model + N-Benzyl-4-chloro-N-cyclohexylbenzamide (FPS-ZM1) group, the TMG + FPS-ZM1 group, and the butylphthalide (NBP) + NS group. Rats in the sham + NS group served as the control, whereas rats in the remaining groups were subjected to pMCAO. After 6 h of surgery, rats in the model + NS group were administered saline intraperitoneally and by gavage. Rats in the TMG + NS group were treated with saline intraperitoneally and 288.6 mg/kg TMG by gavage. Rats in the model + FPS-ZM1 group were administered 1 mg/kg FPS-ZM1 intraperitoneally and saline by gavage. Rats in the TMG + FPS-ZM1 group were administered 1 mg/kg FPS-ZM1 intraperitoneally and 288.6 mg/kg TMG by gavage. Rats in the NBP + NS group were treated with saline intraperitoneally and NBP by gavage. All rats were weighed daily after surgery and the weight was expressed in grams. All the tissue samples were collected after seven days of administration.
Zea Longa neurological deficit score measurement
Neurological deficit was assessed after 6 h of pMCAO surgery and every day during treatment, according to the Zea Longa neurological deficit score scale (Longa et al., 1989). It is described here with: 0, no neurologic deficit symptoms, activity is completely normal; 1, mild neurologic deficit, unable to fully extend the opposite front paw; 2, moderate neurologic deficit, turning to the opposite side when crawling; 3, severe neurologic deficit, tilt towards the opposite side when crawling; 4, loss of consciousness, inability to crawl; 5, death.
2, 3, 5-triphenyltetrazolium chloride staining
Rats were injected with 40 mg/kg sodium pentobarbital intraperitoneally, and the brains of rats were immediately removed and frozen at −20°C for 30 min. Coronal slices (2 mm) were stained with 2% 2, 3, 5-triphenyltetrazolium chloride (TTC) for 20 min at 37°C in dark. Then, sections were fixed with 4% paraformaldehyde solution for 1 h. Normal brain tissues were stained in red, and the infarct area (cerebral infarction) was unstained. The percentage of cerebral infarction was calculated as follows: area of cerebral infarction (%) = infarct area/total area of the slice × 100 (Li et al., 2015).
Hematoxylin and eosin staining
Hematoxylin and eosin (HE) staining for histopathological observation was conducted as standard histological protocol (Yu et al., 2018a). Specifically, the hippocampus tissues of rats were fixed in 4% paraformaldehyde for 24 h. Then, the tissues were dehydrated in graded concentrations of ethanol (50%, 70%, 85%, 95%, and 100%), paraffin-embedded, and sliced into 5 μm sections. After deparaffinization, the sections were stained with hematoxylin for 5 min and eosin for 2 min. Then, the sections were mounted and photographed under a light microscope (DMi8, Leica, Wetzlar, Germany).
Paraffin-embedded hippocampus sections were de-waxed and rehydrated. Sections were then stained with a Prussian blue staining kit (Servicebio, Wuhan, China) for 15 min. After removing the excess dye, sections were further stained with hematoxylin for 30 s. Then, sections were dehydrated, made transparent, and observed under a fluorescence microscope (DMi8, Leica).
Enzyme linked immunosorbent assay
Brain tissues were isolated from rats and were homogenized using an extraction solution. The tissue homogenate was centrifuged for 10 min at 3,000 g and 4°C. The obtained supernatant was collected for Enzyme Linked Immunosorbent Assay (ELISA). Levels of AGEs, ferritin, ROS, and MDA in brain tissue supernatants were detected using ELISA (R&D Systems, Minneapolis, MN, USA).
Hippocampus tissue samples were homogenized in TRIzol reagent (Invitrogen, Carlsbad, CA). Quantitative real-time polymerase chain reaction (qRT-PCR) was performed as previously reported (Marín-Prida et al., 2013). Primer sequences used for qRT-PCR were as follows: RAGE-F: 5′-ACA GAA ACC GGT GAT GAA GGA-3′, RAGE-R 5′-TGT CGT TTT CGC CAC AGG AT-3′; β-actin-F: 5′-CAT CTG CTG GAA GGT GGA CA-3′, β-actin-R: 5′-GAG AGG GAA ATC GTG CGT GAC-3′. The relative mRNA expression of RAGE was calculated using the 2−∆∆Ct method. β-actin was used as an internal control.
Total protein was extracted from the hippocampus tissues of rats using radioimmunoprecipitation assay (RIPA) lysis buffer (Beyotime, Shanghai, China, No. P0013B) and quantified using the bicinchoninic acid assay (BCA) kit (Beyotime, No. P0010S). After electrophoresis (80 V, 2 h), proteins were transferred to polyvinylidene fluoride (PVDF; Roth, Karlsruhe, Germany) membranes, followed by blocking with 5% skim milk in TBST solution for 1 h. Membranes were incubated with rabbit anti-RAGE monoclonal antibody (1:1,000, Abcam, No. ab216329), rabbit anti-JAK2 monoclonal antibody (1:1,000, Abcam, No. 108596), rabbit anti-p-JAK2 monoclonal antibody (1:1,000, Abcam, No. 32101), and mouse anti-β-actin monoclonal antibody (1:1,000, Abcam, No. 8245) at 4°C overnight, followed by incubating with corresponding secondary antibodies for 1 h. Specific protein bands were visualized using the ECL reagent (GE Healthcare, Munich, Germany). Secondary antibodies used in western blotting were goat anti-rabbit IgG (H + L) conjugated to horseradish peroxidase (HRP) (1:100, Multi Science, No. 70-GAR0072) and goat anti-mouse IgG (H + L) conjugated to HRP (1:100, Multi Science, No. 70-GAM0072).
Data in this study were presented as the mean ± standard deviation. Statistical analyses were carried out using SPSS 17.0 software (IBM Corp., Armonk, NY, USA). Differences between two groups were analyzed by Student’s t-test, while those among more than two groups were analyzed by one-way analysis of variance (ANOVA) and then by the Student-Newman-Keuls test. p < 0.05 was considered to be statistically significant.
TMG improved neurological function and body weight of pMCAO rats
A rat model of ischemic stroke was established by pMCAO. As shown in Fig. 1A, the Zea Longa neurological deficit score of sham rats was 0. After surgery, the Zea Longa scores of all groups were similar. After treatments, the Zea Longa scores of the TMG + NS group, model + FPS-ZM1 group, TMG + FPS-ZM1 group, and NBP + NS group reduced on different levels (p < 0.05, Fig. 1A). FPS-ZM1 is an antagonist of the RAGE pathway and NBP is an approved drug for ischemic stroke. The administration of TMG, FPS-ZM1, or NBP led to obvious an improvement of neurological function in rats that underwent pMCAO surgery (p < 0.05, Fig. 1A). The trend of body weight change was similar to the Zea Longa neurological deficit score (Fig. 1B). pMCAO model rats showed continued body weight loss and foreleg disability and blindness compared to sham rats (p < 0.01). Treatments of TMG, FPS-ZM1 or NBP led to significant improvements in body weight and the health condition (p < 0.01, Fig. 1B).
Figure 1: Effects of Tianmagouteng yin (TMG) on the Zea Longa neurological deficit scores (A) and body weights (B) in rats that received permanent middle cerebral artery occlusion (pMCAO). Data were expressed as mean ± standard deviation (n = 3). *p < 0.05 and **p < 0.01 vs. the sham + NS group; ##p < 0.01 vs. the model + NS group; ^^p < 0.01 vs. the model + FPS-ZM1 group.
TMG reduced infarction volume and brain injury of pMCAO rats
TCC staining was applied to reflect the infarction degree of the rat brain. As shown in Fig. 2, the lesion areas in the brains of pMCAO rats were significantly larger than those of sham rats (p < 0.01). After treatments, reduced lesion areas were found. The statistical analysis demonstrated that TMG, FPS-ZM1, or NBP treatments induced significant decreases in the lesion area (p < 0.01, Fig. 2).
Figure 2: Tianmagouteng yin (TMG) reduced infarction volume and neuron injury of permanent middle cerebral artery occlusion (pMCAO) rats. Representative photograph of coronal brain sections stained with 2, 3, 5-triphenyltetrazolium chloride (TTC) and the quantification of cerebral infarction area in brains. Data were expressed as mean ± standard deviation (n = 3). **p < 0.01 vs. the sham + NS group; ##p < 0.01 vs. the model + NS group; ^^p < 0.01 vs. the model + FPS-ZM1 group.
TMG alleviated neuronal injury and iron deposition of pMCAO rats
HE staining was performed for histological evaluation within the hippocampus (Fig. 3A). In the sham + NS group, lots of closely arranged neurons and glial cells were observed. Reduced and unclear neurons with a lot of vacuolation were observed in the injured hippocampus induced by pMCAO (model + NS group). Treatments of TMG, FPS-ZM1 or NBP led to increased closely arranged neurons and less vacuolation (Fig. 3A). In addition, the iron accumulation and distribution in hippocampus tissues were assessed by Prussian blue staining. Significant increased Prussian blue staining spots were found in hippocampus tissues in pMCAO rats. Treatments with TMG, FPS-ZM1 or NBP reduced Prussian blue staining spots (Fig. 3B).
Figure 3: Tianmagouteng yin (TMG) alleviated neuron injury and iron deposition of permanent middle cerebral artery occlusion (pMCAO) rats. (A) Representative photograph of hematoxylin and eosin (HE) staining hippocampus sections (n = 3). Scale bar: 20 µm. (B) Representative Prussian blue staining of hippocampus sections (n = 3). Scale bar: 50 µm.
TMG relieved AGE biosynthesis and oxidative stress of pMCAO Rats
ELISA results from the peri-infarcted brain tissue showed significantly increased AGE levels and upregulated oxidative stress (ferritin, MDA, and ROS) compared with those in the healthy tissue (p < 0.01, Fig. 4). TMG treatment significantly reduced the AGE level in pMCAO rats (p < 0.01, Fig. 4), while FPS-ZM1 and NBP did not show a significant effect. TMG, FPS-ZM1 or NBP significantly reduced the levels of ferritin, MDA, and ROS (p < 0.05), indicating strong antioxidant abilities (Fig. 4).
Figure 4: Tianmagouteng yin (TMG) rescued the increased levels of advanced glycation end products (AGEs), ferritin, malondialdehyde (MDA), and reactive oxygen species (ROS) induced by ischemia in the peri-infarcted brain tissues. Statistical results of AGEs, Ferritin, MDA, and ROS were shown. Data were expressed as mean ± standard deviation (n = 3). *p < 0.05 and **p < 0.01 vs. the sham + NS group; #p < 0.05 and ##p < 0.01 vs. the model + NS group; ^p < 0.05 and ^^p < 0.01 vs. the model + FPS-ZM1 group.
TMG inhibited the AGE/RAGE pathway in pMCAO rats
As shown in Fig. 5A, the mRNA level of RAGE was markedly increased in pMCAO rats compared with that in sham rats (p < 0.01). Treatments with TMG, FPS-ZM1 or NBP significantly decreased the mRNA level of RAGE in pMCAO rats (p < 0.01, Fig. 5A). Western blotting demonstrated that the activity of RAGE and phosphorylation of JAK2 (p-JAK2) were significantly elevated in pMCAO rats compared with those in sham rats (p < 0.01, Fig. 5B). Treatments with TMG, FPS-ZM1 or NBP significantly decreased RAGE and p-JAK2 expression (p < 0.01, Fig. 5B).
Figure 5: Tianmagouteng yin (TMG) inhibited the advanced glycation end products (AGE)/receptor of advanced glycation end products/(RAGE) pathway in permanent middle cerebral artery occlusion (pMCAO) rats. (A) Transcriptional level of RAGE evaluated by quantitative real-time polymerase chain reaction (qRT-PCR); (B) protein levels of RAGE and p-JAK2/JAK2 evaluated by western blot assay. Data were expressed as mean ± standard deviation (n = 3). *p < 0.05 and **p < 0.01 vs. the sham + NS group; ##p < 0.01 vs. the model + NS group; ^p < 0.05 vs. the model + FPS-ZM1 group.
Chinese medicines have been widely applied to treat various brain diseases, and TMG, in particular, is commonly used for treating cerebral ischemia and brain-associated diseases (Xian et al., 2016). However, the therapeutic effects of TMG on ischemic stroke and the underlying mechanisms remain to be further researched. The pMCAO rat model is a reliable animal model of ischemic stroke, with a high degree of repeatability (Hua et al., 2019). Therefore, a pMCAO-induced ischemic stroke rat model was used in this study. Based on this model, the neuroprotective effect of TMG on ischemic stroke was studied. In this study, we found that TMG mitigated the pathological injury of ischemic stroke in ischemic stroke rats by blocking the AGE/RAGE axis.
Initially, significant neurological dysfunction and brain infarction were found in the pMCAO-induced ischemic stroke rats compared with those in sham rats, which were reversed by TMG treatment. Previous studies have demonstrated the neuroprotective function of the extracts of Gastrodia elata (Xiao et al., 2021) and Uncaria rhynchophylla (Deng et al., 2021), the main components of TMG in cerebral ischemia models, accounting for the above findings in the current study. Further, TMG has been revealed to effectively alleviate cerebral infarction and neurological injury in another report (Tang et al., 2021), which was consistent with our data.
Moreover, we observed elevated ferritin levels in ischemic stroke rat models compared with those in sham rats and TMG treatment abolished this increasing trend. Following cerebral hemorrhage, imbalanced iron metabolism occurs in the brain (Chen et al., 2022). The body activates the Fe-transferrin-transferrin receptor (TfR) pathway to maintain its homeostasis, facilitating the outflow of iron ions while storing them in the form of ferritin, generating high levels of oxygen radicals, ultimately inducing inflammatory responses and apoptosis (Chen et al., 2022). It has been suggested that the severity of neuronal injury following a stroke is positively related to the degree of iron accumulation in the brain (Shi et al., 2021). In line with our data, a previous study revealed iron deposition and increased ferritin level in a cerebral ischemia mice model (Hanke and Rami, 2022). Taken together, these findings indicate the beneficial effect of TGM in the regulation of iron metabolism in ischemic stroke.
Accumulating evidence shows that oxidative stress is the key mechanism in the development of ischemic stroke (Li et al., 2021, 2022). Oxidative stress is primarily induced by an imbalance between the production and consumption of ROS (Fan et al., 2022).In ischemic stroke, oxidative stress can trigger inflammation, neuronal apoptosis, excitotoxicity, and damage to the blood-brain barrier, leading to brain injury (Zhao et al., 2022a). Therefore, inhibition of oxidative stress has been a promising strategy for the treatment of ischemic stroke. MDA is a lipid peroxide product of ROS attacking protein, DNA, and polyunsaturated fatty acid. Thus, MDA is usually used as a biomarker of oxidative stress (Deng et al., 2022a).In this study, we found that TMG treatment mitigated the excessive production of MDA and ROS induced by the ischemic stroke-. Notably, studies have indicated the potent anti-oxidative stress effect of TMG (Deng et al., 2022b, Jin et al., 2022). Similar to the findings of our study, a previous investigation indicated that the water extract of TMG significantly alleviated the pathological damage of cerebral ischemia both in vitro and in vivo by inhibiting ROS-induced oxidative stress (Xian et al., 2016).
A recent study has demonstrated that the AGE/RAGE signaling pathway is the core pathway in the treatment of ischemic stroke (Wang et al., 2023). In ischemia models, increased RAGE activation triggers several intracellular signaling pathways like NF-κB, MAPK, PI3K/Akt, and JAK/STAT that lead to enhanced inflammatory response and elevated levels of oxidative stress (Ott et al., 2014; Sapkota et al., 2021). Previous studies reported that inhibition of RAGE or JAK2 could protect rat brains against permanent focal ischemia (Wang et al., 2010; Tian et al., 2019). Moreover, inhibition of the JAK2/STAT3 pathway was reported to alleviate inflammation, infarction, and neurological deficits in ischemic stroke (Tian et al., 2018). Hence, we hypothesized that TMG exerted its anti-ischemic stroke effect by inhibiting the AGE/RAGE pathway. As we predicted, we found that the AGE/RAGE/JAK2 signaling cascade was activated in the ischemic stroke rat models, which, however, was blocked by TMG treatment. To further validate our hypothesis, FPS-ZM1, a specific RAGE antagonist that blocks the association between RAGE and ligands such as AGEs (Hong et al., 2016) was used in this study. Significantly, FPS-ZM1 has been proven to exert no toxic effects when penetrating the blood-brain barrier due to its small molecular weight (MW = 327 Da) and good hydrophilicity (Deane et al., 2012). Our results showed that FPS-ZM1 strengthened the effects of TMG on ameliorating neurological dysfunction, mitigating brain infarction and neuronal injury, reducing iron deposition, and inhibiting oxidative stress. Collectively, these data suggest that TMG ameliorates the symptoms of ischemic stroke by suppressing the AGE/RAGE pathway.
This study demonstrated the potential anti-ischemic stroke activity of TMG and investigated a new pathway (AGE/RAGE pathway) behind it. This would provide a reference for follow-up research into novel drugs and deepen our insights into the pathological mechanisms of ischemic stroke. However, this study has several limitations. We investigated the efficacy of TMG in the treatment of ischemic stroke only at the rat model level and other animal models and in vitro models are required for further verification. Besides, the crosstalk between iron metabolism and oxidative stress in the pathological process of ischemic stroke remains to be further elucidated. These limitations will be addressed in our future and further studies.
In summary, this study showed that TMG alleviated brain injury and suppressed oxidative stress in ischemic stroke. Further, our data revealed that TMG exerted its neuroprotective effect by blocking the AGE/RAGE pathway. These findings suggest TMG can be a promising drug for ischemic stroke.
Funding Statement: This work was supported by Zhejiang TCM Science and Technology Program [Grant Number 2021ZA050].
Author Contributions: The authors confirm their contribution to the paper as follows: Conceptualization: Luojun Zheng; Methodology: Luojun Zheng, Luan Weng; Formal analysis and investigation: Luan Weng and Diwen Shou; Writing—original draft preparation: Luojun Zheng; Writing—review and editing: Luan Weng and Diwen Shou. All authors read and approved the final manuscript.
Availability of Data and Materials: The datasets analyzed during the current study are available from the corresponding author on reasonable request.
Ethics Approval: The experimental and animal ethics were approved by the Ethics Committee of the First Affiliated Hospital of Zhejiang Chinese Medical University (Zhejiang Provincial Hospital of Traditional Chinese Medicine).
Conflicts of Interest: The authors declare that they have no conflicts of interest to report regarding the present study.
References
Barthels D, Das H (2020). Current advances in ischemic stroke research and therapies. Biochimica et Biophysica Acta. Molecular Basis of Disease 1866: 165260. https://doi.org/10.1016/j.bbadis.2018.09.012 [Google Scholar] [PubMed] [CrossRef]
Chen S, Chen H, Du Q, Shen J (2020). Targeting myeloperoxidase (MPO) mediated oxidative stress and inflammation for reducing brain ischemia injury: Potential application of natural compounds. Frontiers in Physiology 11: 433. https://doi.org/10.3389/fphys.2020.00433 [Google Scholar] [PubMed] [CrossRef]
Chen Q, Song W, Tang Y, Tang Y, Kang Y, Zhu L (2022). Electroacupuncture reduces cerebral hemorrhage injury in rats by improving cerebral iron metabolism. Mediators of Inflammation 2022: 6943438. https://doi.org/10.1155/2022/6943438 [Google Scholar] [PubMed] [CrossRef]
Deane R, Singh I, Sagare AP, Bell RD, Ross NT et al. (2012). A multimodal RAGE-specific inhibitor reduces amyloid β–mediated brain disorder in a mouse model of Alzheimer disease. The Journal of Clinical Investigation 122: 1377–1392. https://doi.org/10.1172/JCI58642 [Google Scholar] [PubMed] [CrossRef]
Deng Z, Li J, Tang X, Li D, Wang Y, Wu S, Fan K, Ma Y (2022a). Leonurine reduces oxidative stress and provides neuroprotection against ischemic injury via modulating oxidative and NO/NOS pathway. International Journal of Molecular Sciences 23: 10188. https://doi.org/10.3390/ijms231710188 [Google Scholar] [PubMed] [CrossRef]
Deng L, Liu W, Xu Q, Guo R, Zhang D, Ni J, Li L, Cai X, Fan G, Zhao Y (2022b). Tianma Gouteng Decoction regulates oxidative stress and inflammation in AngII-induced hypertensive mice via transcription factor EB to exert anti-hypertension effect. Biomedicine & Pharmacotherapy 145: 112383. https://doi.org/10.1016/j.biopha.2021.112383 [Google Scholar] [PubMed] [CrossRef]
Deng Y, Tan R, Li F, Liu Y, Shi J, Gong Q (2021). Isorhynchophylline ameliorates cerebral ischemia/reperfusion injury by inhibiting CX3CR1-mediated microglial activation and neuroinflammation. Frontiers in Pharmacology 12: 574793. https://doi.org/10.3389/fphar.2021.574793 [Google Scholar] [PubMed] [CrossRef]
Fan J, Cao S, Chen M, Yao Q, Zhang X et al. (2022). Investigating the AC079305/DUSP1 axis as oxidative stress-related signatures and immune infiltration characteristics in ischemic stroke. Oxidative Medicine and Cellular Longevity 2022: 8432352. https://doi.org/10.1155/2022/8432352 [Google Scholar] [PubMed] [CrossRef]
Gao L, Xiao Z, Jia C, Wang W (2021). Effect of Buyang Huanwu decoction for the rehabilitation of ischemic stroke patients: A meta-analysis of randomized controlled trials. Health and Quality of Life Outcomes 19: 79. https://doi.org/10.1186/s12955-021-01728-6 [Google Scholar] [PubMed] [CrossRef]
Hanke N, Rami A (2022). Cerebral ischemia induces iron deposit, ferritin accumulation, nuclear receptor coactivator 4-depletion, and ferroptosis. Current Neurovascular Research 19: 47–60. https://doi.org/10.2174/1567202619666220321120954 [Google Scholar] [PubMed] [CrossRef]
Heese K (2020). Gastrodia elata Blume (TianmaHope for brain aging and dementia. Evidence-Based Complementary and Alternative Medicine 2020: 8870148. https://doi.org/10.1155/2020/8870148 [Google Scholar] [PubMed] [CrossRef]
Hong Y, Shen C, Yin Q, Sun M, Ma Y, Liu X (2016). Effects of RAGE-specific inhibitor FPS-ZM1 on Amyloid-β Metabolism and AGEs-induced inflammation and oxidative stress in rat hippocampus. Neurochemical Research 41: 1192–1199. https://doi.org/10.1007/s11064-015-1814-8 [Google Scholar] [PubMed] [CrossRef]
Hua S, Wang B, Chen R, Zhang Y, Zhang Y, Li T, Dong L, Fu X (2019). Neuroprotective effect of dichloromethane extraction from Piper nigrum L. and Piper longum L. on permanent focal cerebral ischemia injury in rats. Journal of Stroke and Cerebrovascular Diseases 28: 751–760. https://doi.org/10.1016/j.jstrokecerebrovasdis.2018.11.018 [Google Scholar] [PubMed] [CrossRef]
Huang Z, Zhou X, Zhang X, Huang L, Sun Y, Cheng Z, Xu W, Li CG, Zheng Y, Huang M (2021). Pien-Tze-Huang, a Chinese patent formula, attenuates NLRP3 inflammasome-related neuroinflammation by enhancing autophagy via the AMPK/mTOR/ULK1 signaling pathway. Biomedicine & Pharmacotherapy 141: 111814. https://doi.org/10.1016/j.biopha.2021.111814 [Google Scholar] [PubMed] [CrossRef]
Iadecola C, Buckwalter MS, Anrather J (2020). Immune responses to stroke: Mechanisms, modulation, and therapeutic potential. The Journal of Clinical Investigation 130: 2777–2788. https://doi.org/10.1172/JCI135530 [Google Scholar] [PubMed] [CrossRef]
Jin M, Cao B, Lin C, Li J, Xu Q, Ren Q, Xu S, Tang C (2022). Tianma Gouteng Decoction exerts pregnancy-protective effects against preeclampsia via regulation of oxidative stress and NO signaling. Frontiers in Pharmacology 13: 849074. https://doi.org/10.3389/fphar.2022.849074 [Google Scholar] [PubMed] [CrossRef]
Li Z, Bi R, Sun S, Chen S, Chen J, Hu B, Jin H (2022). The role of oxidative stress in acute ischemic stroke-related thrombosis. Oxidative Medicine and Cellular Longevity 2022: 8418820. https://doi.org/10.1155/2022/8418820 [Google Scholar] [PubMed] [CrossRef]
Li W, Yang Y, Hu Z, Ling S, Fang M (2015). Neuroprotective effects of DAHP and Triptolide in focal cerebral ischemia via apoptosis inhibition and PI3K/Akt/mTOR pathway activation. Frontiers in Neuroanatomy 9: 48. https://doi.org/10.3389/fnana.2015.00048 [Google Scholar] [PubMed] [CrossRef]
Li C, Zhao Z, Luo Y, Ning T, Liu P et al. (2021). Macrophage-disguised manganese dioxide nanoparticles for neuroprotection by reducing oxidative stress and modulating inflammatory microenvironment in acute ischemic stroke. Advanced Science 8: e2101526. https://doi.org/10.1002/advs.202101526 [Google Scholar] [PubMed] [CrossRef]
Liu N, Liu C, Yang Y, Ma G, Wei G, Liu S, Kong L, Du G (2021). Xiao-Xu-Ming decoction prevented hemorrhagic transformation induced by acute hyperglycemia through inhibiting AGE-RAGE-mediated neuroinflammation. Pharmacological Research 169: 105650. https://doi.org/10.1016/j.phrs.2021.105650 [Google Scholar] [PubMed] [CrossRef]
Longa EZ, Weinstein PR, Carlson S, Cummins R (1989). Reversible middle cerebral artery occlusion without craniectomy in rats. Stroke 20: 84–91. https://doi.org/10.1161/01.STR.20.1.84 [Google Scholar] [PubMed] [CrossRef]
Marín-Prida J, Pavón-Fuentes N, Llópiz-Arzuaga A, Fernández-Massó JR, Delgado-Roche L et al. (2013). Phycocyanobilin promotes PC12 cell survival and modulates immune and inflammatory genes and oxidative stress markers in acute cerebral hypoperfusion in rats. Toxicology and Applied Pharmacology 272: 49–60. https://doi.org/10.1016/j.taap.2013.05.021 [Google Scholar] [PubMed] [CrossRef]
Oshima Y, Harashima A, Munesue S, Kimura K, Leerach N et al. (2022). Dual nature of RAGE in host reaction and nurturing the mother-infant bond. International Journal of Molecular Sciences 23: 2086. https://doi.org/10.3390/ijms23042086 [Google Scholar] [PubMed] [CrossRef]
Ott C, Jacobs K, Haucke E, Navarrete Santos A, Grune T, Simm A (2014). Role of advanced glycation end products in cellular signaling. Redox Biology 2: 411–429. https://doi.org/10.1016/j.redox.2013.12.016 [Google Scholar] [PubMed] [CrossRef]
Paul S, Candelario-Jalil E (2021). Emerging neuroprotective strategies for the treatment of ischemic stroke: An overview of clinical and preclinical studies. Experimental Neurology 335: 113518. https://doi.org/10.1016/j.expneurol.2020.113518 [Google Scholar] [PubMed] [CrossRef]
Sapkota A, Park SJ, Choi JW (2021). Receptor for advanced glycation end products is involved in LPA5-mediated brain damage after a transient ischemic stroke. Life 11: 80. https://doi.org/10.3390/life11020080 [Google Scholar] [PubMed] [CrossRef]
Shi H, Almutairi M, Moskovitz J, Xu YG (2021). Recent advances in iron homeostasis and regulation—A focus on epigenetic regulation and stroke. Free Radical Research 55: 375–383. https://doi.org/10.1080/10715762.2020.1867314 [Google Scholar] [PubMed] [CrossRef]
Sun S, Chen Q, Wu B, Huang Q, Maimaitijiang A (2022). RAGE regulating vascular remodeling in diabetes by regulating mitochondrial dynamics with JAK2/STAT3 pathway. Computational Intelligence and Neuroscience 2022: 2685648. https://doi.org/10.1155/2022/2685648 [Google Scholar] [PubMed] [CrossRef]
Tang X, Lu J, Chen H, Zhai L, Zhang Y, Lou H, Wang Y, Sun L, Song B (2021). Underlying mechanism and active ingredients of tianma gouteng acting on cerebral infarction as determined via network pharmacology analysis combined with experimental validation. Frontiers in Pharmacology 12: 760503. https://doi.org/10.3389/fphar.2021.760503 [Google Scholar] [PubMed] [CrossRef]
Tao T, Liu M, Chen M, Luo Y, Wang C, Xu T, Jiang Y, Guo Y, Zhang JH (2020). Natural medicine in neuroprotection for ischemic stroke: Challenges and prospective. Pharmacology & Therapeutics 216: 107695. https://doi.org/10.1016/j.pharmthera.2020.107695 [Google Scholar] [PubMed] [CrossRef]
Tian YS, Zhong D, Liu QQ, Zhao XL, Sun HX, Jin J, Wang HN, Li GZ (2018). Upregulation of miR-216a exerts neuroprotective effects against ischemic injury through negatively regulating JAK2/STAT3-involved apoptosis and inflammatory pathways. Journal of Neurosurgery 130: 977–988. https://doi.org/10.3171/2017.5.JNS163165 [Google Scholar] [PubMed] [CrossRef]
Tian YS, Zhong D, Liu QQ, Zhao XL, Sun HX, Jin J, Wang HN, Li GZ (2019). Upregulation of miR-216a exerts neuroprotective effects against ischemic injury through negatively regulating JAK2/STAT3-involved apoptosis and inflammatory pathways. Journal of Neurosurgery JNS 130: 977–988. https://doi.org/10.3171/2017.5.JNS163165 [Google Scholar] [PubMed] [CrossRef]
Wang C, Deng X, Wang Z, Wang S, Tian J et al. (2021). PNS protects brain against ischemic injury by acting as an antagonist for AGE/RAGE signaling. Clinical and Translational Medicine 11: e532. https://doi.org/10.1002/ctm2.532 [Google Scholar] [PubMed] [CrossRef]
Wang L, Zhang X, Liu L, Yang R, Cui L, Li M (2010). Atorvastatin protects rat brains against permanent focal ischemia and downregulates HMGB1, HMGB1 receptors (RAGE and TLR4NF-κB expression. Neuroscience Letters 471: 152–156. https://doi.org/10.1016/j.neulet.2010.01.030 [Google Scholar] [PubMed] [CrossRef]
Wang Y, Zu G, Yu Y, Tang J, Han T, Zhang C (2023). Curcumin’s mechanism of action against ischemic stroke: A network pharmacology and molecular dynamics study. PLoS One 18: e0280112. https://doi.org/10.1371/journal.pone.0280112 [Google Scholar] [PubMed] [CrossRef]
Xian JW, Choi AY, Lau CB, Leung WN, Ng CF, Chan CW (2016). Gastrodia and Uncaria (tianma gouteng) water extract exerts antioxidative and antiapoptotic effects against cerebral ischemia in vitro and in vivo. Chinese Medicine 11: 27. https://doi.org/10.1186/s13020-016-0097-6 [Google Scholar] [PubMed] [CrossRef]
Xiao H, Jiang Q, Qiu H, Wu K, Ma X, Yang J, Cheng O (2021). Gastrodin promotes hippocampal neurogenesis via PDE9-cGMP-PKG pathway in mice following cerebral ischemia. Neurochemistry International 150: 105171. https://doi.org/10.1016/j.neuint.2021.105171 [Google Scholar] [PubMed] [CrossRef]
Yu SY, Dong B, Fang ZF, Hu XQ, Tang L, Zhou SH (2018a). Knockdown of lncRNA AK139328 alleviates myocardial ischaemia/reperfusion injury in diabetic mice via modulating miR-204-3p and inhibiting autophagy. Journal of Cellular and Molecular Medicine 22: 4886–4898. https://doi.org/10.1111/jcmm.13754 [Google Scholar] [PubMed] [CrossRef]
Yu W, Tao M, Zhao Y, Hu X, Wang M (2018b). 4′-methoxyresveratrol alleviated AGE-induced inflammation via RAGE-mediated NF-κB and NLRP3 inflammasome pathway. Molecules 23: 1447. https://doi.org/10.3390/molecules23061447 [Google Scholar] [PubMed] [CrossRef]
Zhao X, He Y, Zhang Y, Wan H, Wan H, Yang J (2022a). Inhibition of oxidative stress: An important molecular mechanism of chinese herbal medicine (Astragalus membranaceus, Carthamus tinctorius L., Radix Salvia Miltiorrhizae, etc.) in the treatment of ischemic stroke by regulating the antioxidant system. Oxidative Medicine and Cellular Longevity 2022: 1425369. https://doi.org/10.1155/2022/1425369 [Google Scholar] [PubMed] [CrossRef]
Zhao Y, Zhang X, Chen X, Wei Y (2022b). Neuronal injuries in cerebral infarction and ischemic stroke: From mechanisms to treatment (Review). International Journal of Molecular Medicine 49: 15. https://doi.org/10.3892/ijmm.2021.5070 [Google Scholar] [PubMed] [CrossRef]
Zhong Y, Yin B, Ye Y, Dekhel OYAT, Xiong X, Jian Z, Gu L (2021). The bidirectional role of the JAK2/STAT3 signaling pathway and related mechanisms in cerebral ischemia-reperfusion injury. Experimental Neurology 341: 113690. https://doi.org/10.1016/j.expneurol.2021.113690 [Google Scholar] [PubMed] [CrossRef]
Cite This Article
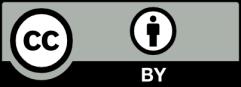