Open Access
ARTICLE
Therapeutic targets and signal transduction mechanisms of medicinal plant formula Gancao Xiexin decoction against ulcerative colitis: A network pharmacological study
1 The First School of Clinical Medicine, Zhejiang Chinese Medical University, Hangzhou, China
2 Department of Respiratory Medicine, Xiaoshan Third People’s Hospital, Hangzhou, China
3 Department of Emergency Medicine, Xiaoshan Third People’s Hospital, Hangzhou, China
* Corresponding Author: GUANHUA XU. Email:
(This article belongs to the Special Issue: Natural Products for Chronic Inflammatory Diseases: Pharmacology and Toxicology)
BIOCELL 2023, 47(6), 1329-1344. https://doi.org/10.32604/biocell.2023.028381
Received 15 December 2022; Accepted 30 January 2023; Issue published 19 May 2023
Abstract
Background: Ulcerative colitis (UC) is a chronic disease that often presents with abdominal pain, diarrhea, hematochezia, and significant morbidity. Gancao Xiexin decoction (GXD), a traditional Chinese medicine, has been applied for the clinical treatment of UC, while its action mechanisms are unclear. Methods: The active ingredients and their targets of GXD, and UC-related targets, were derived from public databases. Protein-protein interaction, Gene Ontology (GO), and the Kyoto Encyclopedia of Genes and Genomes (KEGG) were used to analyze the important active compounds, key targets, and signaling pathways. Then, molecular docking and animal experiments were performed to verify the findings. A total of 213 active compounds and 89 common targets of GXD for UC were obtained. Results: The hub gene network showed ALB, AKT1, IL6, TNF, VEGFA, TP53, CXCL8, MAPK1, PTGS2, and IL1β may be potential targets of GXD against UC. GO and KEGG pathway enrichment analyses suggested that the action of GXD against UC was mainly related to response to oxygen levels, lipopolysaccharide, and molecule of bacterial origin, etc., and achieved by advanced glycation endproducts/receptors for advanced glycation endproducts signaling pathway in diabetic complications, hypoxia-inducible factor (HIF)-1 signaling pathway, interleukin-17/HIF-1 signaling pathway, TNF signaling pathway, etc. Molecular docking results showed that the GXD had good potency of action with the hub target. In vivo experiments showed that GXD significantly alleviated the symptoms of UC and down-regulated the expression of inflammatory factors, nuclear factor-κB and signal transducer and activator of transcription 3. Conclusions: The anti-UC action of GXD is mainly attributed to its anti-oxidative stress, anti-inflammatory, and immunomodulatory functions.Keywords
Ulcerative colitis (UC) is a chronic inflammatory colon disease that often presents with abdominal pain, diarrhea, and hematochezia (Kobayashi et al., 2020). The incidence and prevalence of UC are on the rise in many developing countries in Asia and the Middle East (Ng et al., 2019; Kotze et al., 2020), and its causative factors involve genetic background, environmental factors, and immune dysfunction (Wei et al., 2021). Currently, drugs commonly used in the clinical treatment of UC include 5-aminosalicylates, corticosteroids, cyclosporine, etc. (Luo and Luo, 2021); however, these drugs have limited therapeutic effects and have notable side effects. Therefore, finding a new drug for the treatment of UC has become an urgent clinical problem.
To date, traditional Chinese medicine (TCM) has had an influence on various diseases, including UC. Gancao Xiexin decoction (GXD) consists of six herbs, namely Gancao (licorice), Banxia (Arum Ternatum Thunb.), Huanglian (Coptidis Rhizoma), Ganjiang (Zingiberis Rhizoma), Huangqin (Scutellariae Radix), and Dazao (Jujubae Fructus), was first recorded in the ‘Shanghanlun,’ written by Zhang Zhongjing (Luo et al., 2022). Modern pharmacological studies have found that GXD has the ability to regulate the immune function of the body, enhance anti-hypoxia ability (Zhang et al., 1997), regulate gastric mucosal secretion (Gao et al., 2005), and anti-ulcer (Hu and Zhang, 2008). It is widely used in the clinical treatment of diseases of the digestive and immune systems (Zhao et al., 2013a; Yan et al., 2021). Meanwhile, some studies have suggested that GXD can treat UC, but the exact mechanism of action is not clear.
In the present study, we systematically introduced the compounds, targets, and action mechanisms of GXD to alleviate UC through a network pharmacology approach and then performed molecular docking simulation analysis to verify the binding affinity of the compounds and hub targets. Finally, we constructed a mouse model of UC to verify the effects of GXD on UC. Our study will lay the theoretical research foundation for subsequent studies of GXD for the treatment of UC and also provide potential clinical therapeutic targets for the treatment of UC.
Collection of targets for ulcerative colitis
Therapeutic targets for UC were mined from GeneCard (https://www.genecards.org/) (Safran et al., 2010), Searching Online Mendelian Inheritance in Man (OMIM, http://www.omim.org/) (Amberger and Hamosh, 2017), and DisGeNET (http://www.disgenet.org) (Piñero et al., 2017) databases with “ulcerative colitis” as index word and limiting to “Homo sapiens” species. The overlapped targets from these three databases were removed.
Prediction of active compounds and targets of Gancao Xiexin decoction
The active compounds of GXD were screened from public databases, including TCM Integrated Database (TCMID, http://www.bidd.group/TCMID/) (Huang et al., 2018), TCM system pharmacology technology platform (TCMSP, https://tcmsp-e.com/) (Ru et al., 2014), and Herb Ingredient’ Targets (HIT, http://hit2.badd-cao.net/) (Ye et al., 2011) and deleted some active compounds, for which no targets information is available.
Subsequently, the potential targets of GXD active ingredients were retrieved from TCMID, TCMSP, HIT, and Search Tool for Interacting Chemicals (STITCH, http://stitch.embl.de) (Szklarczyk et al., 2016) databases. Then, the targets with compound-target associated scores >400 in STITCH databases remained and were normalized the name with the Gene module of the NCBI database.
To further screen the active compounds and potential targets of GXD, several fishing methods were implemented. Drug-likeness (DL) is usually used to signify whether the compound has acceptable ADME (absorption, distribution, metabolism, and elimination) properties, and DL assessment of compounds helps to identify the active ingredients in TCM formulas (Yang et al., 2018). The quantitative estimate of DL (QED) (Bickerton et al., 2012) was used to further screen pharmaceutically active compounds in GXD and set 0.2 as the threshold value in this work. Then, the potential targets of GXD were evaluated using binomial statistical mode (Yang et al., 2018; Liang et al., 2014).
Protein-protein interaction (PPI) network
The common targets related to GXD and UC were retrieved by Venny 2.1.0 database (https://bioinfogp.cnb.csic.es/tools/venny/) and then analyzed using the STRING database (https://string-db.org/). Subsequently, files of the PPI network were input into Cytoscape 3.8.0 software to visualize it.
Gene ontology (GO) and Kyoto Encyclopedia of Gene and Genomes (KEGG) pathway enrichment analysis was carried out by DAVID 6.8 (https://david.ncifcrf.gov/tools.jsp). In this study, the GO and KEGG analysis results were thought as significant using adjusted p < 0.01 corrected by the Bonferroni algorithm for each term.
Chemical structures of the active compounds were derived from the Zinc website (http://zinc15.docking.org) (Sterling and Irwin, 2015). Crystal structures of the hub proteins were obtained from the RCSB Protein Data Bank (PDB, http://www.rcsb.org/) (Burley et al., 2017). Then, molecular docking was analyzed using PyMol 2.3.0 (Seeliger and de Groot, 2010), AutoDockTools 1.5.6 (Morris et al., 2009), and AutoDock Vina 1.1.2 (Trott and Olson, 2010).
Construction of the mouse model for ulcerative colitis
All animal protocols were approved by the Animal Experimental Ethics Committee of Xiaoshan Third People’s Hospital. Thirty female C57BL/6 mice (6–8 weeks old, 18–20 g, from GemPharmatech Co., Ltd., Nanjing, China) were housed at room temperature (24°C–26°C) with free access to food and the water cycle.
After 7 days, mice were randomized into six groups (n = 5), including control, model, low-dose GXD, middle-dose GXD, high-dose GXD, and positive control-sulfasalazine (SASP) groups. Mice in the control group received sterile water, while mice in other groups were treated with 2.5% dextran sodium sulfate (DSS, MP Biomedicals, CA, USA) for 7 days. Meanwhile, the mice in the GXD and SASP administer groups were given GXD at 3.7 g/kg (low-dose), 7.4 g/kg (middle-dose), 14.8 g/kg (high-dose), and SASP at 125 mg/kg, respectively. In comparison, mice in the control and model groups received 0.5% CMC-Na.
Weight assessment: no loss was scored as 0 points, 1%–5% loss: 1-point, 6%–10% loss: 2-points, 11%–15% loss: 3-points, more than 15% loss: 4-points. Stool assessment: good stool pattern was scored as 0-points, a loose stool: 2-points, and diarrhea: 4-points. Bleeding assessment: no rectal bleeding was scored as 0-point, no rectal bleeding: 2=points and heavy bleeding: 4-points.
Hematoxylin and eosin (H&E) staining
Colon tissues were fixed in 4% paraformaldehyde, dehydrated in a different concentration of ethanol, embedded in paraffin, and cut into 5 μm thick slices. Slices were stained with H&E (Beijing Solarbio Science & Technology, Beijing, China). The pathological morphology of colon tissue was observed under a microscope (Olympus, Tokyo, Japan).
Quantitative real-time polymerase chain reaction (qRT-PCR) assay
Total RNA from colon tissues was isolated and reverse-transcribed into cDNA by TRIzol reagent (Invitrogen, Carlsbad, CA, USA) and PrimeScript RT Master Mix (TaKaRa, Dalian, China), respectively. Each well (20 μL PCR reaction volume) included 10 μL of SYBR Green Mix (Applied Biosystems, CA, USA), 1 μL of cDNA, 2 μL of primer pair mix, and 7 μL of ddH2O. PCR was carried out on a system (MX3000P, Agilent Stratagene, Santa Clara, CA, USA) with the thermocycling conditions as follows: Initial denaturation at 95°C for 3 min, followed by 40 cycles of 95°C for 12 s and 62°C for 40 s. The levels of specific genes were calculated by the 2−∆∆CT method. GAPDH worked as a reference gene. Primer sequences are listed in Suppl. Table S1.
Total proteins were extracted from colon tissues using RIPA lysis buffer (Beyotime, Shanghai, China), separated by gel electrophoresis, and transferred to membranes. Membranes were blocked by 5% fat-free milk for 1 h and incubated with p65-nuclear factor-(NF)-ĸB (1:1000, ab207297, Abcam, Cambridge, UK), phosphorylated Signal transducer and activator of transcription 3 (p-STAT3; 1:1000, ab76315, Abcam), STAT3 (1:1000, ab68153, Abcam), and glyceraldehyde 3-phosphate dehydrogenase (GAPDH; 1:10000, ab181602, Abcam) antibodies at 4°C overnight, followed by incubation of secondary antibody (1:2000, ab6721, Abcam) for 1 h. Finally, the bands were visualized using an enhanced chemiluminescent reagents kit (Millipore, Darmstadt, Germany).
The Prism 8 (GraphPad, San Diego, CA, USA) software was used for statistical analyses. All data were exhibited with mean and standard deviation and analyzed using one-way ANOVA, followed by Tukey’s test. p < 0.05 was considered to reflect a significant difference.
Collection of therapeutic targets for ulcerative colitis
We found 63, 322, and 521 therapeutic targets for UC from GeneCard, OMIM, and DisGeNET databases, respectively. Then, 804 UC-related targets were finally retrieved after removing duplicate targets.
Identification of potential targets and potential targets of Gancao Xiexin decoction
From TCMID, TCMSP, and HIT databases, 371 active compounds of GXD were obtained. A total of 7154 potential targets of GXD active compounds were retrieved from TCMID, TCMSP, HIT, and STITCH databases. Next, 346 active compounds were suitable for DL screening (QED ≥ 0.2). Then, from 285 active compounds, 692 potential targets were collated by binomial statistical mode. Subsequently, UC-related targets and GXD potential targets were entered into Venny 2.1.0, and after eliminating the redundancy, 89 common targets between UC and GXD were obtained (Fig. 1). Finally, 213 active compounds were mapped to 89 common targets. These 213 active compounds and 89 common targets are detailed in Tables 1 and 2, respectively. Furthermore, we constructed a Drug-compounds-targets (D-C-T) network using Cytoscape software with 213 active compounds and 89 common targets. The D-C-T network includes 309 nodes and 1545 edges (Fig. 2).
Figure 1: Venn diagram of Gancao Xiexin decoction (GXD) active components and ulcerative colitis-related targets.
Figure 2: Drug-compounds-targets (D-C-T) network of Gancao Xiexin decoction (GXD). The yellow-green quadrilaterals represent the key targets. Different colored circles represent the compounds in different herbs. Grass-green circles represent compounds contained in a variety of herbs, and the red prisms represent different herbs.
Construction of protein-protein interaction network
Interaction relationships of common targets were obtained using the STING database. The PPI network comprised 89 nodes and 1367 edges, with an average degree of 30.7; the larger sizes of the target nodes indicate that nodes have more degrees in the PPI network (Fig. 3A). Then, the top 10 hub degree genes, including ALB, AKT1, IL6, TNF, VEGFA, TP53, CXCL8, MAPK1, PTGS2, and IL1β, were screened out by Cytoscapehubba, a Cytoscape plug-in. The hub gene network consisted of 10 nodes and 45 edges (Fig. 3B).
Figure 3: (A) Protein-protein interaction (PPI) network, (B) Hub genes network.
Gene ontology and kyoto encyclopedia of genes and genomes enrichment analysis
GO enrichment analysis results contained 1107 biological process (BP) terms, 43 molecular function (MF) terms, and 18 cellular component (CC) terms when adjusted at p < 0.01. For BP terms, the common targets were mainly related to response to oxygen levels, lipopolysaccharide, the molecule of bacterial origin, etc. For MF terms, the common targets were mainly phosphatase, cytokine receptors, protein phosphatase binding, etc. For CC terms, the common targets were primarily located in the membrane microdomain, membrane raft, membrane region, etc. (Figs. 4A–4C). KEGG analysis remarkably enriched 144 pathways (p < 0.01), of which the top 15 pathways are presented in Fig. 4D. Most of the common targets were enriched in the AGE-RAGE signaling pathway in diabetic complication (hsa04933), HIF-1 signaling patIy (hsa04066), endocrine resIance (hsa01522), etc. Additionally, to explain that GXD could treat UC, we used the key targets, top 15 KEGG pathways, and top 15 BP terms to construct a targets-pathways-BP network, containing 106 nodes and 673 edges (Fig. 5). Furthermore, to further illustrate the ability of GXD to ameliorate UC, we also evaluated the relationship between the hub target and BP, KEGG, as shown in Fig. 6, according to which, AKT1, PTGS2, IL1β, TNF, and MAPK1 may play a vital role during UC treatment.
Figure 4: Gene Ontology (GO) and Kyoto Encyclopedia of Gene and Genomes (KEGG) pathway enrichment analysis, (A) The top 15 biological processes (BP) bubble chart, (B) The top 15 molecular functions (MF) bubble chart, (C) The top 15 cellular components (CC) bubble chart, and (D) The top 15 KEGG bubble chart.
Figure 5: The key targets-pathways-biological process (BP) network.
Figure 6: Gene Ontology (GO) and Kyoto Encyclopedia of Gene and Genomes (KEGG) enrichment analysis of hub genes. (A) Biological process (BP); (B) KEGG pathway.
The interactions between the active compounds and hub targets were investigated to further explore the action mechanism of the GXD compounds. Nine active compounds (maslinic acid, baicalin, betulinic acid, protopine, liquiritin, baicalein, glycyrrhizic acid, isoliquiritigenin, and gingerol; the detailed information is elucidated in Table 3) and eight hub genes (MAPK1, PTGS2, AKT1, TP53, IL6, ALB, and TNF) were selected for molecular docking analysis. After setting the threshold affinity <−5 kcal/mol, we obtained 59 pairs of docking results (Table 4). The top 10 pairs of docking results are exhibited in Fig. 7. Among them, the binding affinity of maslinic acid with tumor necrosis factor (TNF) was −9.7 kcal/mol, which was contributed by bonding with SER-60 and LEU-120 residues. The binding affinity of baicalin with PTGS2 was −9.7 kcal/mol, which was contributed by bonding with HIS-388, TYR-385, THR-212, and HIS-214 residues. The binding affinity of betulinic acid with TNF was −9.6 kcal/mol, which was contributed by bonding with HIS-388, TYR-385, THR-212, and HIS-214 residues.
Figure 7: The top 10 molecular docking of the active compound-hub gene. (A) Maslinic acid to tumor necrosis factor (TNF), affinity = −9.7 kcal/mol, (B) Baicalin to Prostaglandin-endoperoxide synthase 2 (PTGS2), affinity = −9.7 kcal/mol, (C) Betulinic acid to TNF, affinity = −9.6 kcal/mol; (D) Protopine to albumin (ALB), affinity = −9.4 kcal/mol; (E) Baicalin to serine-threonine kinase 1 (AKT1), affinity = −9.3 kcal/mol; (F) Liquiritin to mitogen-activated protein kinase 1 (MAPK1), affinity = −9.2 kcal/mol; (G) Liquiritin to ALB, affinity = −9.2 kcal/mol; (H) Baicalein to Prostaglandin-endoperoxide synthase 2 (PTGS2), affinity = −8.8 kcal/mol; (I) Protopine to MAPK1, affinity = −8.6 kcal/mol; (J) Protopine to AKT1, affinity = −8.6 kcal/mol.
Verification through animal experiments
To verify the effect of GXD on UC, we established a UC mouse model by administering 2.5% DSS. DAI scores were used to assess the severity of colitis in mice. As shown in Fig. 8A, compared with the control group, DAI scores were significantly increased in the model group. While GXD and SASP administration decreased the DAI scores. Additionally, the colon length was significantly shorter in the model group than in the control group. GXD and SASP administration notably suppressed the shortening of colon length in UC mice (Figs. 8B and 8C). The colon in the model group showed tissue damage with mucosal architecture and apparent inflammatory cell infiltration. Likewise, GXD provided a therapeutic effect in a dose-dependent manner (Fig. 8D).
Figure 8: Gancao Xiexin decoction (GXD) treatment attenuates dextran sodium sulfate (DSS)-induced colitis in mice. (A) Disease activity index (DAI), (B–C) Colon length, (D) Representative hematoxylin and eosin staining of colon sections (n = 3). ***p < 0.001 vs. control group; ##p < 0.01 and ###p < 0.001 vs. model group; $p < 0.05, $$p < 0.01, and $$$p < 0.001 vs. low-dose GXD group.
To further verify whether GXD could act against UC, we detected the levels of inflammatory cytokines and potential targets using qRT-PCR and western blotting. The mRNA levels of inflammatory cytokines interleukin (IL)-1β, IL-17, IL-6, TNF-α, and cyclooxygenase (COX)-2 were markedly increased in the model group; then they were weakened by dose-dependent addition of GXD (Fig. 9A). Meanwhile, the protein expression of p65-NF-ĸB, p-STAT3, and STAT3 using western blotting assay revealed that oral administration of 2.5% DSS obviously enhanced p65-NF-ĸB and p-STAT3 expression levels, while GXD and SASP addition reversed this enhancement of DSS. Besides, the expression of STAT3 did not change in all groups (Fig. 9B).
Figure 9: (A) Effect of Gancao Xiexin decoction (GXD) on the expression of inflammatory cytokines, including cyclooxygenase (COX)-2, interleukin (IL)-17, IL-1β, IL-6, and tumor necrosis factor (TNF)-α were detected by the quantitative real-time polymerase chain reaction assay (n = 3). (B) Effects of GXD on the expression of p65-nuclear factor (NF)-ĸB, phosphorylated signal transducer and activator of transcription 3 (p-STAT3 and STAT3) were detected using western blot assay (n = 3). ***p < 0.001 vs. control group; #p < 0.05, ##p < 0.01, and ###p < 0.001 vs. model group; $p < 0.05, $$p < 0.01, and $$$p < 0.001 vs. low-dose GXD group.
Recently, clinical studies have found that GXD reduces the expression of inflammatory cytokines in the serum of UC patients (Zhao et al., 2013b; Guo, 2017; Wang et al., 2016). However, the anti-UC therapeutic mechanism of GXD has not been characterized. In this study, network pharmacology combined with molecular docking was used to unravel the therapeutic targets and action mechanisms of GXD against UC.
Using a network pharmacology assay, the active compounds, potential targets, and action mechanisms were obtained. The D-C-T network showed that quercetin, hexadecenoic acid, palmitic acid, baicalein, choline, baicalin, and so on may be the main active compounds of GXD against UC. Quercetin is a polyhydroxy flavonoid with a variety of biological functions, such as anti-oxidation, anti-inflammation, and anti-viral action. Quercetin inhibits the release of pro-inflammatory mediators and pro-inflammatory protein expression (Habtemariam and Belai, 2018). Baicalin inhibits the expression of IL-33 and NF-κB p65, increases IκB-α levels, and regulates T17/Treg balance and intestinal flora to exert anti-inflammatory effects (Zhang et al., 2017; Zhu et al., 2020). These suggest that GXD affects inflammation, which is also confirmed by suppressing the GXD-mediated up-regulation of inflammatory factors in UC mice.
Moreover, the hub genes network suggested that ALB, AKT1, IL6, TNF, VEGFA, TP53, CXCL8, MAPK1, PTGS2, and IL1β may be the potential targets of GXD against UC. TNF-α, a major inflammatory factor, is involved in the pathogenesis of inflammatory bowel disease (IBD), and UC is a subtype of IBD. Anti-TNF-α drugs are commonly used in the treatment of UC (Oliveira et al., 2021). IL-1β is a key mediator in the development of UC, leading to increased intestinal epithelial permeability and stimulating neutrophil recruitment to infected colonic tissue, resulting in colonic mucosal edema and necrosis (Neurath, 2014). TP53 is an oncogene closely associated with colon carcinogenesis and could be a key target for UC prevention and treatment (Lu et al., 2017; Elmashad et al., 2016). Additionally, PTGS2 (COX-2) plays a specific role in inflammation and is expressed in epithelial cells and monocytes in IBD (Farrokhyar et al., 2001; Cox et al., 2005). Here, the mRNA expression changes of IL6, TNF-α, IL1β, and COX-2 in UC mice were reversed by GXD addition, which indicates that these potential targets may be the therapeutic targets for UC treatment.
GO and KEGG enrichment analyses revealed the potential GXD anti-UC effect achieved via the advanced glycation endproducts/receptors for advanced glycation endproducts (AGE-RAGE) signaling pathway in diabetic complications, HIF-1, IL-17, and TNF signaling pathways, etc. One meta-analysis suggested that genetic polymorphisms in IL-17A and IL-17F may increase the risk of UC development in both allelic and dominant models (Li et al., 2014). AGE and RAGE are involved in and mediate various oxidative stress signaling pathways, inducing reactive oxygen species production and activating NF-κB, leading to inflammatory responses, apoptosis, and microvascular disease (Fukami et al., 2014). RAGE polymorphisms and increased levels of RAGE have been identified in patients with IBD, and AGE/RAGE involvement in inflammation is associated with the activation of NF-κB and its response to oxidative stress (Moura et al., 2020). All these indicate that GXD acts against UC via multiple pathways.
Additionally, molecular docking results showed GXD compounds have good binding ability to hub proteins. In vivo experiments showed that GXD could significantly alleviate the symptoms of UC, including reducing DAI score, improving colonic tissue damage and inflammatory cell infiltration, and increasing colonic length. Meanwhile, GXD treatment down-regulated the expression of inflammatory factors NF-κB and STAT3 in UC mice.
In conclusion, network pharmacological analysis revealed that GXD has 89 targets and 213 compounds potentially involved in the treatment of UC. GO and KEGG enrichment analyses showed that the action mechanism of GXD in the treatment of UC is related to its antioxidant, anti-inflammatory, and immunomodulatory functions. Molecular docking suggests that the hub genes MAPK1, PTGS2, AKT1, TP53, IL6, ALB, and TNF may be potential targets for GXD against UC. Animal experiments have shown that GXD can downregulate the inflammatory levels of UC mice, as well as the expression of NF-κB and p-STAT3. Our research provides a scientific basis for the subsequent development and development of GXD in the treatment of UC. However, our study also had shortcomings; for example, we did not explore the specific mechanisms by which GXD is used to treat UC.
Funding Statement: The authors received no specific funding for this study.
Author Contributions: The authors confirm contribution to the paper as follows: study conception and design: Chen-hao Shi, Guan-hua Xu; data collection: Chen-hao Shi, Mao-hong Hua; analysis and interpretation of results: Chen-hao Shi, Mao-hong Hua, Guan-hua Xu; draft manuscript preparation: Chen-hao Shi. All authors reviewed the results and approved the final version of the manuscript.
Availability of Data and Materials: The datasets generated during and/or analysed during the current study are available from the corresponding author on reasonable request.
Ethics Approval: All animal protocols were approved by the Animal Experimental Ethics Committee of Xiaoshan Third People’s Hospital.
Conflicts of Interest: The authors declare that they have no conflicts of interest to report regarding the present study.
References
Amberger JS, Hamosh A (2017). Searching online mendelian inheritance in man (OMIMA knowledgebase of human genes and genetic phenotypes. Current Protocols in Bioinformatics 58: 1.2.1–1.2.12. https://doi.org/10.1002/cpbi.27 [Google Scholar] [PubMed] [CrossRef]
Bickerton GR, Paolini GV, Besnard J, Muresan S, Hopkins AL (2012). Quantifying the chemical beauty of drugs. Nature Chemistry 4: 90–98. https://doi.org/10.1038/nchem.1243 [Google Scholar] [PubMed] [CrossRef]
Burley SK, Berman HM, Kleywegt GJ, Markley JL, Nakamura H, Velankar S (2017). Protein data bank (PDBThe single global macromolecular structure archive. Methods in Molecular Biology 1607: 627–641. https://doi.org/10.1007/978-1-4939-7000-1 [Google Scholar] [CrossRef]
Cox DG, Crusius JB, Peeters PH, Bueno-de-Mesquita HB, Pena AS, Canzian F (2005). Haplotype of prostaglandin synthase 2/cyclooxygenase 2 is involved in the susceptibility to inflammatory bowel disease. World Journal of Gastroenterology 11: 6003–6008. https://doi.org/10.3748/wjg.v11.i38.6003 [Google Scholar] [PubMed] [CrossRef]
Elmashad NM, Ziada DH, Hasby EA, Mohamed AEM (2016). Immunohistochemical expression of proinflammatory enzyme COX-2 and p53 in ulcerative colitis and its associated dysplasia and colorectal carcinoma. Journal of Microscopy and Ultrastructure 4: 195–202. https://doi.org/10.1016/j.jmau.2016.03.003 [Google Scholar] [PubMed] [CrossRef]
Farrokhyar F, Swarbrick ET, Irvine EJ (2001). A critical review of epidemiological studies in inflammatory bowel disease. Scandinavian Journal of Gastroenterology 36: 2–15. https://doi.org/10.1080/00365520150218002 [Google Scholar] [PubMed] [CrossRef]
Fukami K, Yamagishi S, Okuda S (2014). Role of AGEs-RAGE system in cardiovascular disease. Current Pharmaceutical Design 20: 2395–2402. https://doi.org/10.2174/13816128113199990475 [Google Scholar] [PubMed] [CrossRef]
Gao Y, Si Y, Shang J, Wen R, Niu X (2005). Effects of 3 kinds of Xiexin decoction and their different combinations on gastric mucus composition in normal rats. Chinese Traditional Patent Medicine 27: 69–74. [Google Scholar]
Guo S (2017). Modified Gancao Xiexin decoction in the treatment of ulcerative colitis for 57 cases. Guangming Journal of Chinese Medicine 32: 992–994. [Google Scholar]
Habtemariam S, Belai A (2018). Natural therapies of the inflammatory bowel disease: The case of Rutin and its Aglycone, Quercetin. Mini-Reviews in Medicinal Chemistry 18: 234–243. https://doi.org/10.2174/1389557517666170120152417 [Google Scholar] [PubMed] [CrossRef]
Hu Y, Zhang Y (2008). Effect of Gancao Xiexin decoction on serum NO and NOS in patients with recurrent aphthous ulcer. Practical Pharmacy and Clinical Remedies 11: 143–144. [Google Scholar]
Huang L, Xie D, Yu Y, Liu H, Shi Y, Shi T, Wen C (2018). TCMID 2.0: A comprehensive resource for TCM. Nucleic Acids Research 46: D1117–D1120. https://doi.org/10.1093/nar/gkx1028 [Google Scholar] [PubMed] [CrossRef]
Kobayashi T, Siegmund B, Le Berre C, Wei SC, Ferrante M, Shen B, Bernstein CN, Danese S, Peyrin-Biroulet L, Hibi T (2020). Ulcerative colitis. Nature Reviews Disease Primers 6: 74. https://doi.org/10.1038/s41572-020-0205-x [Google Scholar] [PubMed] [CrossRef]
Kotze PG, Underwood FE, Damiao A, Ferraz JGP, Saad-Hossne R et al. (2020). Progression of inflammatory bowel diseases throughout Latin America and the Caribbean: A systematic review. Clinical Gastroenterology and Hepatology 18: 304–312. https://doi.org/10.1016/j.cgh.2019.06.030 [Google Scholar] [PubMed] [CrossRef]
Li J, Tian H, Jiang HJ, Han B (2014). Interleukin-17 SNPs and serum levels increase ulcerative colitis risk: A meta-analysis. World Journal of Gastroenterology 20: 15899–15909. https://doi.org/10.3748/wjg.v20.i42.15899 [Google Scholar] [PubMed] [CrossRef]
Liang X, Li H, Li S (2014). A novel network pharmacology approach to analyse traditional herbal formulae: The Liu-Wei-Di-Huang pill as a case study. Molecular BioSystems 10: 1014–1022. https://doi.org/10.1039/C3MB70507B [Google Scholar] [PubMed] [CrossRef]
Lu X, Yu Y, Tan S (2017). p53 expression in patients with ulcerative colitis—associated with dysplasia and carcinoma: A systematic meta-analysis. BMC Gastroenterology 17: 111. https://doi.org/10.1186/s12876-017-0665-y [Google Scholar] [PubMed] [CrossRef]
Luo M, Luo Y (2021). Imperatorin relieved ulcerative colitis by regulating the Nrf-2/ARE/HO-1 pathway in rats. Inflammation 44: 558–569. https://doi.org/10.1007/s10753-020-01353-3 [Google Scholar] [PubMed] [CrossRef]
Luo YT, Wu J, Zhu FY, Wu JQ, Wu P, Liu YC (2022). Gancao Xiexin decoction ameliorates ulcerative colitis in mice via modulating gut microbiota and metabolites. Drug Design Development and Therapy 16: 1383–1405. https://doi.org/10.2147/DDDT.S352467 [Google Scholar] [PubMed] [CrossRef]
Morris GM, Huey R, Lindstrom W, Sanner MF, Belew RK, Goodsell DS, Olson AJ (2009). AutoDock4 and AutoDockTools4: Automated docking with selective receptor flexibility. Journal of Computational Chemistry 30: 2785–2791. https://doi.org/10.1002/jcc.21256 [Google Scholar] [PubMed] [CrossRef]
Moura FA, Goulart MOF, Campos SBG, da Paz Martins AS (2020). The close interplay of nitro-oxidative stress, advanced glycation end products and inflammation in inflammatory bowel diseases. Current Medicinal Chemistry 27: 2059–2076. https://doi.org/10.2174/0929867325666180904115633 [Google Scholar] [PubMed] [CrossRef]
Neurath MF (2014). Cytokines in inflammatory bowel disease. Nature Reviews Immunology 14: 329–342. https://doi.org/10.1038/nri3661 [Google Scholar] [PubMed] [CrossRef]
Ng SC, Kaplan GG, Tang W, Banerjee R, Adigopula B et al. (2019). Population density and risk of inflammatory bowel disease: A prospective population-based study in 13 countries or regions in Asia-Pacific. American Journal of Gastroenterology 114: 107–115. https://doi.org/10.1038/s41395-018-0233-2 [Google Scholar] [PubMed] [CrossRef]
Oliveira RG, Damazo AS, Antonielli LF, Miyajima F, Pavan E, Duckworth CA, Lima J, Arunachalam K, Martins DTO (2021). Dilodendron bipinnatum Radlk. Extract alleviates ulcerative colitis induced by TNBS in rats by reducing inflammatory cell infiltration, TNF-α and IL-1β concentrations, IL-17 and COX-2 expressions, supporting mucus production and promotes an antioxidant effect. Journal of Ethnopharmacology 269: 113735. https://doi.org/10.1016/j.jep.2020.113735 [Google Scholar] [PubMed] [CrossRef]
Piñero J, Bravo À, Queralt-Rosinach N, Gutiérrez-Sacristán A, Deu-Pons J, Centeno E, García-García J, Sanz F, Furlong LI (2017). DisGeNET: A comprehensive platform integrating information on human disease-associated genes and variants. Nucleic Acids Research 45: D833–d839. https://doi.org/10.1093/nar/gkw943 [Google Scholar] [PubMed] [CrossRef]
Ru J, Li P, Wang J, Zhou W, Li B et al. (2014). TCMSP: A database of systems pharmacology for drug discovery from herbal medicines. Journal of Cheminformatics 6: 13. https://doi.org/10.1186/1758-2946-6-13 [Google Scholar] [PubMed] [CrossRef]
Safran M, Dalah I, Alexander J, Rosen N, Iny Stein T et al. (2010). GeneCards version 3: The human gene integrator. Database 2010: baq020. https://doi.org/10.1093/database/baq020 [Google Scholar] [PubMed] [CrossRef]
Seeliger D, de Groot BL (2010). Ligand docking and binding site analysis with PyMOL and Autodock/Vina. Journal of Computer-Aided Molecular Design 24: 417–422. https://doi.org/10.1007/s10822-010-9352-6 [Google Scholar] [PubMed] [CrossRef]
Sterling T, Irwin JJ (2015). ZINC 15—ligand discovery for everyone. Journal of Chemical Information and Modeling 55: 2324–2337. https://doi.org/10.1021/acs.jcim.5b00559 [Google Scholar] [PubMed] [CrossRef]
Szklarczyk D, Santos A, von Mering C, Jensen LJ, Bork P, Kuhn M (2016). STITCH 5: Augmenting protein-chemical interaction networks with tissue and affinity data. Nucleic Acids Research 44: D380–D384. https://doi.org/10.1093/nar/gkv1277 [Google Scholar] [PubMed] [CrossRef]
Trott O, Olson AJ (2010). AutoDock Vina: Improving the speed and accuracy of docking with a new scoring function, efficient optimization, and multithreading. Journal of Computational Chemistry 31: 455–461. https://doi.org/10.1002/jcc.21334 [Google Scholar] [PubMed] [CrossRef]
Wang GQ, Wei WH, Yang J (2016). Effects of Gancao Xiexin decoction on serum levels of IL-17 and IL-23 in patients with ulcerative colitis. Journal of Nanjing University of Traditional Chinese Medicine 32: 25–28. [Google Scholar]
Wei YY, Fan YM, Ga Y, Zhang YN, Han JC, Hao ZH (2021). Shaoyao decoction attenuates DSS-induced ulcerative colitis, macrophage and NLRP3 inflammasome activation through the MKP1/NF-κB pathway. Phytomedicine 92: 153743. https://doi.org/10.1016/j.phymed.2021.153743 [Google Scholar] [PubMed] [CrossRef]
Yan J, Yan Y, Young A, Yan Z, Yan Z (2021). Effectiveness and safety of Chinese medicine decoctions for behcet’s disease: A systematic review and meta-analysis. Evidence-Based Complementary and Alternative Medicine 2021: 8202512. https://doi.org/10.1155/2021/8202512 [Google Scholar] [PubMed] [CrossRef]
Yang M, Chen J, Xu L, Shi X, Zhou X, An R, Wang X (2018). A network pharmacology approach to uncover the molecular mechanisms of herbal formula Ban-Xia-Xie-Xin-Tang. Evidence-Based Complementary and Alternative Medicine 2018: 4050714. https://doi.org/10.1155/2018/4050714 [Google Scholar] [PubMed] [CrossRef]
Ye H, Ye L, Kang H, Zhang D, Tao L et al. (2011). HIT: Linking herbal active ingredients to targets. Nucleic Acids Research 39: D1055–1059. https://doi.org/10.1093/nar/gkq1165 [Google Scholar] [PubMed] [CrossRef]
Zhang S, Hao L, Gong C, Song Y, Yi C (1997). Effects of Gancao Xiexin decoction on immune function and normobaric hypoxia tolerance in mice. Pharmacology and Clinic of Traditional Chinese Medicine 13: 12–13. [Google Scholar]
Zhang CL, Zhang S, He WX, Lu JL, Xu YJ, Yang JY, Liu D (2017). Baicalin may alleviate inflammatory infiltration in dextran sodium sulfate-induced chronic ulcerative colitis via inhibiting IL-33 expression. Life Sciences 186: 125–132. https://doi.org/10.1016/j.lfs.2017.08.010 [Google Scholar] [PubMed] [CrossRef]
Zhao C, Cai Z, Deng M (2013a). Observation on therapeutic effect of modified Gancao Xiexin decoction on pseudomembranous enteritis. Journal of Emergency in Traditional Chinese Medicine 22: 117–118. [Google Scholar]
Zhao Q, Wang S, Liang X (2013b). Gancao Xiexin decoction in the treatment of relapsing ulcerative colitis: Clinical observation and the effects of intestinal microflora and serum interleukin 6, 10. Chinese Archives of Traditional Chinese Medicine 31: 944–946. [Google Scholar]
Zhu L, Xu LZ, Zhao S, Shen ZF, Shen H, Zhan LB (2020). Protective effect of baicalin on the regulation of Treg/Th17 balance, gut microbiota and short-chain fatty acids in rats with ulcerative colitis. Applied Microbiology and Biotechnology 104: 5449–5460. https://doi.org/10.1007/s00253-020-10527-w [Google Scholar] [PubMed] [CrossRef]
Supplementary Materials
Cite This Article
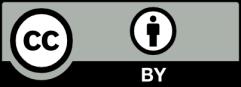
This work is licensed under a Creative Commons Attribution 4.0 International License , which permits unrestricted use, distribution, and reproduction in any medium, provided the original work is properly cited.