Open Access
ARTICLE
Capsaicin exerts anti-benign prostatic hyperplasia effects via inhibiting androgen receptor signaling pathway
1 School of Medicine, Yichun University, Yichun, 336000, China
2 College of Chemistry and Bio-Engineering, Yichun University, Yichun, 336000, China
3 School of Medical and Health Sciences, Fooyin University, Kaohsiung, 83102, Taiwan
* Corresponding Author: CHI-MING LIU. Email:
# These authors contributed equally to this work
BIOCELL 2023, 47(6), 1389-1396. https://doi.org/10.32604/biocell.2023.028222
Received 06 December 2022; Accepted 10 February 2023; Issue published 19 May 2023
Abstract
Background: Benign prostatic hyperplasia (BPH) is a common condition in middle-aged and elderly men. Enlargement of the prostate causes lower urinary tract symptoms. Capsaicin is a phytochemical extracted from chili peppers and exerts many pharmacological actions, such as anti-tumor and anti-inflammatory effects. Methods: Our study investigated the effect of capsaicin in vitro and in a mouse model in vivo. A prostatic stromal myofibroblast cell line (WPMY-1) was co-incubated with testosterone (1 µM) and different concentrations of capsaicin (10–100 µM) for 24 and 48 h. Capsaicin (10–100 µM) significantly inhibited testosterone-treated WPMY-1 cell growth at 48 h by MTT assay. The testosterone propionate (7.5 mg/kg)-induced BPH mouse model was used to examine the anti-proliferative effect of capsaicin. Treatment with capsaicin (10 mg/kg) for 14 days significantly attenuated prostatic hyperplasia. Finasteride was used as a positive control. Results: Capsaicin significantly decreased prostate weight and prostate index (prostate/body weight ratio) in BPH mice. The expression of 5α-reductase type II, androgen receptor (AR) and prostate specific antigen (PSA) protein expression and PSA serum were all significantly reduced in capsaicin-treated BPH mice. In addition, capsaicin also activated transient receptor potential vanilloid 1 mediated apoptosis and autophagy in BPH mice. Conclusion: These results demonstrate multiple positive effects of capsaicin in controlling prostate growth and suggest its therapeutic potential in the treatment of BPH.Keywords
Benign prostatic hyperplasia (BPH) is a common condition in men over 50 years of age and is accompanied by lower urinary tract symptoms (LUTS) (Foo, 2019; Lerner et al., 2021). BPH is directly related to a high circulating level of testosterone (Cleutjens et al., 1996). Testosterone and dihydrotestosterone (DHT) are very important in regulating prostatic growth and the progress of BPH. The enzyme 5α-reductase converts testosterone into DHT. DHT induces signal pathways and prostate growth by binding to AR, which has a higher binding affinity than testosterone in the prostate. Two medications mainly used for BPH are α-blockers and 5α-reductase type II inhibitors (Plochocki and King, 2022). α-blockers improve urinary flow rates and decrease smooth muscle tone. Finasteride significantly reduces prostate volume and improves symptoms (Füllhase and Schneider, 2016).
Regular consumption of chili peppers has been reported to prevent cardiovascular diseases and cancers (Sanati et al., 2018). Capsaicin is a pungent ingredient extracted from chili peppers which has been used for treating many diseases, including pain, the common cold, and rheumatism (Srinivasan, 2016). Capsaicin has been investigated for anti-inflammatory, analgesic, anesthetic, detoxification, anti-obesity, and anti-cancer effects (Barkin, 2013; Zheng et al., 2017; Qin et al., 2019; Persson et al., 2018; Chen et al., 2021b). Previous studies have reported that capsaicin induces apoptosis and autophagy in prostate cancer cells (Sánchez et al., 2007; Ramos-Torres et al., 2016). Capsaicin induced apoptosis, generated ROS production, and downregulated androgen receptor (AR) and prostate specific antigen (PSA) in LNCaP cells (Mori et al., 2006). Another study also showed restoration of miR-449a by capsaicin leading to inhibition of AR signaling (Zheng et al., 2015).
Apoptosis and autophagy regulate cell growth and death in a cooperative manner. Autophagy deactivation was observed in prostatic inflammation samples from patients with BPH and LUTS (de Nunzio et al., 2017; de Nunzio et al., 2021). Anti-apoptosis effects were increased in the BPH rat model (Choi et al., 2021). Many phytochemicals are able to suppress prostatic cell growth by inducing autophagy, apoptosis and are used in the treatment of prostate cancer and BPH (Sachan et al., 2018; Kang et al., 2021; Ashrafizadeh et al., 2022). We have found little research on the pharmacological mechanisms of action of capsaicin in relieving BPH. Whether capsaicin can inhibit prostate growth and downregulate AR signaling pathway activation in BPH remains unknown. Our study aimed to evaluate the effects of capsaicin on BPH in vitro and in vivo in an animal model.
The capsaicin (98% purity; cat. no. BP0312) was obtained from Chengdu Biopurify Phytochemicals, Ltd. (Chengdu, China). Dulbecco’s Modified Eagle Medium (DMEM) was supplemented with 10% fetal bovine serum (FBS), and both were obtained from Cellmax (Beijing, China). The antibiotics penicillin (100 U/mL)-streptomycin (100 µg/mL) were purchased from Beyotime Institute of Biotechnology (Haimen, China). Testosterone (cat. no. T102169) and testosterone propionate (cat. no. T101368) were purchased from Aladdin Biochemical Technology Co., Ltd. (Shanghai, China). PSA ELISA kits (cat. no. JM-02752M1) were purchased from Jiangsu Jingmei Biotechnology Co., Ltd. (Yancheng, China). Anti-β-actin antibodies were obtained from MilliporeSigma (cat. no. A5441; Massachusetts, USA). Anti-beclin-1 (cat. no. AB3219), anti-microtubule-associated proteins 1A/1B light chain 3B (anti-LC3B; cat. no. CY5992), anti-AR (cat. no. CY5030), and anti-5α-reductase type II (cat. no. CY8576) antibodies were purchased from Abways Technology (Shanghai, China). Primary antibodies against PSA (cat. no. ab76113) were purchased from Abcam (Shanghai, China). Primary antibodies against poly ADP-ribose polymerase (PARP) (cat. no. 9542) and caspase-3 (cat. no. 14220) were purchased from Cell Signaling Technology (Shanghai, China). Anti-transient receptor potential vanilloid 1 (TRPV1; cat. no. DF10320) was obtained from Affinity Biosciences (Changzhou, China).
Human prostatic stromal myofibroblast cell line (WPMY-1) was purchased from Shanghai Fuheng Biotechnology Co., Ltd. (Shanghai, China). Cells were cultured in DMEM medium supplemented with 1% penicillin-streptomycin and 10% FBS at 37°C in a humidified atmosphere with 5% CO2 in an incubator.
The cells were seeded in 96-well plates at a density of 1 × 104 per well. The cells were treated with different concentrations of capsaicin (1–100 µM), with or without testosterone (1 µM), for 24 h or 48 h. Cell survival rate was measured by MTT (3-(4,5-dimethylthiazol-2-yl)-2,5-diphenyl-2H-tetrazolium bromide) assay. After the each timepoint, 10 µL of MTT (5 mg/mL) was added to fresh medium (100 µL/well), and cells were incubated at room temperature for 4 h. Formazan crystals were dissolved in dimethyl sulfoxide (100 µL/well) with incubation at 37°C for 20 min. Absorbance was measured by a microplate reader at a wavelength of 570 nm.
Seven-week-old male ICR (Institute of Cancer Research) mice were obtained from Hunan SJA Laboratory Animal Co., Ltd. (Changsha, Hunan, China). All experiments were approved and conducted according to the guidelines of the Animal Care and Ethics Committee of Yichun University (Approval No. 2022026). Mice were maintained at 22 ± 2°C under 12-h light/dark cycle conditions. Capsaicin (10 mg/kg) and testosterone propionate (TP; 7.5 mg/kg) were dissolved in corn oil. The BPH induction model was established in mice by subcutaneous injection of testosterone propionate once daily. Capsaicin was given by intragastric administration once daily. Mice were randomly categorized into three groups (n = 6 per group): (A) control group given vehicle alone, (B) testosterone propionate-induced BPH group (TP, 7.5 mg/kg), (C) TP + capsaicin (10 mg/kg). The mice were weighed weekly over the duration of the experiment, which was 14 days and then weighed and sacrificed. Prostate/body weight ratio was calculated as the prostate index. Before sacrificing the animals, serum was collected for determination of PSA concentration using an ELISA kit, according to the manufacturer’s instructions.
Protein lysis buffer (T-PER) (cat. no. 78510; Thermo Fisher Scientific, Inc., Waltham, USA) was used to extract total prostatic proteins. Bradford protein assay was used to determine the protein concentration. Twenty micrograms of total protein were separated by sodium dodecyl sulfate-polyacrylamide gel electrophoresis. After electrophoresis, proteins were transferred to polyvinylidene difluoride membranes which were blocked with 5% non-fat dried milk in Tris-buffered saline with 0.1% Tween 20 at room temperature for 1 h. After washing at room temperature for 30 min, the membranes were mixed with primary antibody to 5α-reductase type II (1: 1000), AR (1: 1000), PSA (1: 1000), PARP (1: 1000), caspase-3 (1: 1000), LC3B (1: 1000), beclin-1(1: 1000), TRPV1(1: 1000), β-actin (1: 8000) and incubated overnight at 4°C. Subsequently, the membranes were washed and incubated with horseradish peroxidase-coupled secondary antibody (1:1000) at room temperature for 1 h. Protein expression was determined using an enhanced chemiluminescent kit (Shanghai Epizyme Biomedical Technology Co., Ltd., Shanghai, China). Protein bands were visualized by ChemiScope 3300 Mini equipment and software (Shanghai, China). The densitometry of protein expression was determined by ImageJ 1.52a software (National Institutes of Health, Bethesda, USA).
All data are presented as the mean ± S.D. (standard deviation) and using one way ANOVA, followed by Tukey’s post hoc test. p < 0.05 indicates a statistically significant difference.
Effect of capsaicin on the viability of WPMY-1 cells
The effects of capsaicin on WPMY-1 cell viability were determined by MTT assay. The effect of capsaicin (100 µM) in inducing cell death in WPMY-1 cells at 24 and 48 h was not so significant (Fig. 1A). Testosterone (1 µM) significantly induced cell growth at 24 and 48 h. The cell survival rate with testosterone (1 µM) at 48 h was 139.69 ± 9.15% compared with the control. However, capsaicin significantly inhibited cell growth in testosterone-treated WPMY-1 cells with a survival rate of 106.21 ± 7.07% compared with the control at 48 h (Fig. 1B).
Figure 1: Capsaicin-mediated inhibition of testosterone-stimulated growth of WPMY-1 cells. Survival rate of WPMY-1 cells on incubation with capsaicin (1–100 µM) and testosterone (1 µM) for 24 and 48 h. All the values are represented as mean ± SD for three separate experiments (*p < 0.05 compared with control group, #p < 0.05 compared with testosterone group).
Capsaicin attenuated prostatic hyperplasia in testosterone propionate-induced benign prostatic hyperplasia
The body weight of mice did not differ between the groups (Fig. 2A). In the testosterone-induced mouse model of BPH, prostate weight and prostate index of the capsaicin-treated group (10 mg/kg) were lower than those in BPH group (Figs. 2B and 2C). Treatment with capsaicin (10 mg/kg) and finasteride (10 mg/kg) both significantly suppressed the increased prostate weight by 68% and 54%, respectively, and the prostate index by 64% and 35%, respectively. Furthermore, serum PSA levels in the BPH group were higher than in the normal (vehicle) group but were significantly decreased in the capsaicin- and finasteride-treated groups (10 mg/kg) (Fig. 2D).
Figure 2: Effects of capsaicin (10 mg/kg) and finasteride (10 mg/kg) on prostate/body weight (A–B), prostate index (C) and serum prostate-specific antigen (PSA) level (D) in testosterone propionate (7.5 mg/kg)-induced benign prostatic hyperplasia (BPH) mice. Data are represented as mean ± SD (n = 6) (*p < 0.05 compared with control group, #p < 0.05 compared with testosterone group).
Capsaicin regulated the 5α-reductase-androgen receptor axis in testosterone propionate-induced benign prostatic hyperplasia mice
Normally DHT binds to the AR, and 5α-reductase type II then reduces the levels of testosterone. In BPH mice protein expression of 5α-reductase type II, AR and PSA were all significantly upregulated compared to the normal group (Fig. 3A). Furthermore, protein expression levels of 5α-reductase type II, AR, and PSA were significantly decreased by capsaicin treatment compared to the BPH group (Fig. 3A). This indicated that capsaicin is able to inhibit prostate growth by regulating the 5α-reductase-AR signaling pathway.
Figure 3: Effects of capsaicin (10 mg/kg) on the protein expression of 5α-reductase type II and androgen receptor signaling pathway (A), apoptosis, autophagy (B), and transient receptor potential vanilloid 1 (TRPV1) (C) in testosterone propionate (7.5 mg/kg)-induced benign prostatic hyperplasia (BPH) mouse model. Western blot analysis of protein expression of 5α-reductase type II, androgen receptor, prostate-specific antigen (PSA), (uncleaved) poly ADP-ribose polymerase (PARP), (pro-) caspase-3, microtubule-associated proteins 1A/1B light chain 3B (LC3B), and beclin-1. Data are represented as mean ± SD (*p < 0.05 compared with control group, #p < 0.05 compared with testosterone group).
Capsaicin-induced autophagy and apoptosis by regulating transient receptor potential vanilloid 1 (TRPV1) expression
We determined the effect of capsaicin on apoptosis and autophagy, in testosterone propionate-treated BPH mice, by measuring the protein expression of (uncleaved) PARP, (pro-) caspase-3, LC3B, and beclin-1. The expression levels of (uncleaved) PARP and (pro-) caspase-3 were significantly increased in the BPH group (Fig. 3B). Capsaicin treatment significantly inhibited (uncleaved) PARP and (pro-) caspase-3 expression (Fig. 3B). The expression of beclin-1 protein decreased significantly in the BPH group, and this effect was reversed by capsaicin treatment (Fig. 3B). These results demonstrated that capsaicin was able to induce apoptosis and autophagy in BPH mice and TRPV1 expression was significantly increased in the capsaicin treatment group (Fig. 3C). This is consistent with previous reports demonstrating that capsaicin elicits TRPV1 channel activation (Yang and Zheng, 2017).
BPH is a condition causing LUTS and bladder outlet obstruction that affects the quality of life in elderly men. Treatment involving 5α-reductase therapy is mainly used to reduce prostate volume and progressive hypertrophy. The 5α-reductase-AR signaling is considered a pivotal pathway in the treatment of BPH (Ilic and Misso, 2012). Discovering new, safe, effective therapies with few adverse effects would be important. Many researchers have focused on natural plant products. Previous studies have demonstrated that lycopene and curcumin displayed promising effects in the treatment of BPH (Alexandrov et al., 2021; Qiao et al., 2021).
Capsaicin is an active ingredient extracted from Capsicum annum (chili pepper). Its beneficial effects have been demonstrated in obesity, in inhibiting platelet aggregation, for analgesia, in inflammation, and in reducing hyperactive bladder symptoms by TRPV1-dependent or -independent pathways (Sharma et al., 2013). Previous studies have shown the chemotherapeutic effects of capsaicin in prostate cancer (Díaz-Laviada, 2010; Sánchez et al., 2019, 2022). The TP-induced BPH animal model employed in this study, has been widely studied (Dai et al., 2017; Zou et al., 2017). Testosterone can induce prostate cell growth. Several studies have indicated that testosterone (1–10 µM) in vitro can stimulate epithelial or stromal cellular growth (Hong et al., 2020; Karunasagara et al., 2020; Chen et al., 2021a; Baek et al., 2022). In this study, capsaicin treatment significantly suppressed TP-induced BPH and also inhibited testosterone-induced WPMY-1 prostate cell growth in vitro.
Previous studies have demonstrated that downregulation of the 5α-reductase-AR axis can improve BPH symptoms (Füllhase and Schneider, 2016). Our results demonstrated that capsaicin reduced the protein expression of AR, 5α-reductase type II, and PSA in TP-induced BPH mice. Furthermore, capsaicin also decreased serum PSA levels. These results suggest that the inhibitory effect of capsaicin on the androgen pathway affects the progression of BPH. In addition, capsaicin induced the expression of TRPV1 protein in TP-induced BPH mice suggesting its involvement in the inhibitory effects.
Apoptosis is a key event controlling cell survival and death. Apoptosis signaling involves an intrinsic pathway (Bcl-2-related cascade) and an extrinsic pathway (receptor-mediated). The 5α-reductase type II inhibitors induce prostate cell apoptosis in the treatment of BPH (Karunasagara et al., 2020; Park et al., 2019). Caspases are a family of protease enzymes that regulate apoptosis. Under stress or stimulation by phytochemicals, PARP, caspase-9, caspase-7, and caspase-3 are activated (Elkady, 2019; Qiu et al., 2019). In the current study, the protein expression of (pro-) caspase-3 and (uncleaved) PARP were upregulated in the BPH mice and expression of both was attenuated by capsaicin. The apoptotic effects of capsaicin were documented in the current study. Beclin-1 regulates autophagy, and the relationship between autophagy and apoptosis in cell death is complex (Xu and Qin, 2019). One study suggested that caspase-mediated cleavage of beclin-1 promoted crosstalk between apoptosis and autophagy (Djavaheri-Mergny et al., 2010). LC3 is a soluble protein that is converted to LC3-II, which is recruited to autophagosomal membranes and acts as an autophagy-specific marker (Tanida et al., 2008). In the current study, autophagy inhibition was observed in TP-induced BPH mice. Protein expression of LC3B-II and beclin-1 was decreased in the BPH mice, and capsaicin could induce autophagy by upregulating their expression.
In clinical practice, topical application of capsaicin can reduce pain and is particularly indicated in diabetic neuropathy and arthritis. Although the antioxidant and anti-inflammatory effects of capsaicin are well documented, its irritative characteristics limit its clinical use. Capsaicin derivatives, with retained potency and no irritating adverse effects, need to be developed.
In summary, capsaicin significantly reduced the levels of 5α-reductase type II, AR, and PSA in BPH mice and reduced the prostate index. It reduced WPMY-1 cell growth in vitro and mediated cell death by inducing apoptosis and autophagy in vivo (Fig. 4). This study represents novel but relatively small studies investigating various aspects of prostatic cells. However, we did not carry out a detailed histological examination of the prostate. We also studied a single prostatic (myofibroblast) cell line; in the future, it would be useful to repeat the experiments with other cell lines or even human prostatic samples. Stromal and epithelial cells play a vital role in BPH development and the effects of capsaicin require future study. Taken together, these findings strongly suggest the therapeutic potential of capsaicin in BPH.
Figure 4: Diagram demonstrates that capsaicin (Cap) inhibits prostate growth in testosterone propionate-induced benign prostatic hyperplasia (BPH) mouse model. (1) Capsaicin inhibits prostate growth by interfering in the androgen receptor signaling pathway; (2) Capsaicin induces apoptosis and autophagy.
Acknowledgement: The authors would like to express their gratitude to EditSprings (https://www.editsprings.cn) for the expert linguistic services provided.
Funding Statement: This work was financially supported by Yichun University Local Development Research Center (Grant No. DF2019002) and the PhD Research Foundation of Yichun University (Grant No. 211-3360118006).
Author Contributions: Study conception and design: CYC and CML; experiments performed: ZCS, HWC, XZC, HS; data collection: MQS, YDL; analysis and interpretation of results: BHT and ZTW; draft manuscript preparation: ZCS, CYC, CML. All authors reviewed the results and approved the final version of the manuscript.
Availability of Data and Materials:: The data generated during the current study are available from the corresponding author on reasonable request.
Ethics Approval: All experimental procedures were approved and supervised by the Animal Care and Ethics Committee of Yichun University (Approval No. 2022026).
Conflicts of Interest: The authors declare that they have no conflicts of interest to report regarding the present study.
References
Alexandrov VA, Tochilnikov GV, Zhilinskaya NT, Gubareva EA, Romanov VA, Ermakova ED, Dorofeeva AA, Zmitrichenko YG, Tumanyan IA, Semenov AL (2021). Therapeutic effect of iodised serum milk protein, lycopene and their combination on benign prostatic hyperplasia induced in rats. Andrologia 53: e14173. https://doi.org/10.1111/and.14173 [Google Scholar] [PubMed] [CrossRef]
Ashrafizadeh M, Paskeh MDA, Mirzaei S, Gholami MH, Zarrabi A et al. (2022). Targeting autophagy in prostate cancer: Preclinical and clinical evidence for therapeutic response. Journal of Experimental & Clinical Cancer Research 41: 105. https://doi.org/10.1186/s13046-022-02293-6 [Google Scholar] [PubMed] [CrossRef]
Baek EB, Hwang YH, Park S, Hong EJ, Won YS, Kwun HJ (2022). Eriochloa villosa alleviates progression of benign prostatic hyperplasia in vitro and in vivo. Research and Reports in Urology 14: 313–326. https://doi.org/10.2147/RRU.S381713 [Google Scholar] [PubMed] [CrossRef]
Barkin RL (2013). The pharmacology of topical analgesics. Postgraduate Medicine 125: 7–18. https://doi.org/10.1080/00325481.2013.1110566911 [Google Scholar] [PubMed] [CrossRef]
Chen J, Rong N, Liu M, Xu C, Xiong Q, Lei Y (2021a). The exosome-like vesicles derived from androgen exposed-prostate stromal cells promote epithelial cells proliferation and epithelial-mesenchymal transition. Toxicology and Applied Pharmacology 411: 115384. https://doi.org/10.1016/j.taap.2020.115384 [Google Scholar] [PubMed] [CrossRef]
Chen M, Xiao C, Jiang W, Yang W, Qin Q, Tan Q, Lian B, Liang Z, Wei C (2021b). Capsaicin inhibits proliferation and induces apoptosis in breast cancer by down-regulating FBI-1-mediated NF-κB pathway. Drug Design, Development and Therapy 15: 125–140. https://doi.org/10.2147/DDDT.S269901 [Google Scholar] [PubMed] [CrossRef]
Choi DH, Kim JY, An JH, Sung SH, Kong HS (2021). Effects of Saussurea costus on apoptosis imbalance and inflammation in benign prostatic hyperplasia. Journal of Ethnopharmacology 279: 114349. https://doi.org/10.1016/j.jep.2021.114349 [Google Scholar] [PubMed] [CrossRef]
Cleutjens KB, van Eekelen CC, van der Korput HA, Brinkmann AO, Trapman J (1996). Two androgen response regions cooperate in steroid hormone regulated activity of the prostate-specific antigen promoter. The Journal of Biological Chemistry 271: 6379–6388. https://doi.org/10.1074/jbc.271.11.6379 [Google Scholar] [PubMed] [CrossRef]
Dai GC, Hu B, Zhang WF, Peng F, Wang R, Liu ZY, Xue BX, Liu JY, Shan YX (2017). Chemical characterization, anti-benign prostatic hyperplasia effect and subchronic toxicity study of total flavonoid extract of Pteris multifida. Food and Chemical Toxicology 108: 524–531. https://doi.org/10.1016/j.fct.2016.11.010 [Google Scholar] [PubMed] [CrossRef]
de Nunzio CDE, Giglio S, Baldassarri V, Cirombella R, Mallel G, Nacchia A, Tubaro A, Vecchione A (2021). Impairment of autophagy may represent the molecular mechanism behind the relationship between obesity and inflammation in patients with BPH and LUTS. Minerva Urology and Nephrology 73: 631–637. https://doi.org/10.23736/s2724-6051.20.03992-2 [Google Scholar] [PubMed] [CrossRef]
de Nunzio C, Giglio S, Stoppacciaro A, Gacci M, Cirombella R, Luciani E, Tubaro A, Vecchione A (2017). Autophagy deactivation is associated with severe prostatic inflammation in patients with lower urinary tract symptoms and benign prostatic hyperplasia. Oncotarget 8: 50904–50910. https://doi.org/10.18632/oncotarget.15144 [Google Scholar] [PubMed] [CrossRef]
Djavaheri-Mergny M, Maiuri MC, Kroemer G (2010). Cross talk between apoptosis and autophagy by caspase-mediated cleavage of Beclin 1. Oncogene 29: 1717–1719. https://doi.org/10.1038/onc.2009.519 [Google Scholar] [PubMed] [CrossRef]
Díaz-Laviada I (2010). Effect of capsaicin on prostate cancer cells. Future Oncology 6: 1545–1550. https://doi.org/10.2217/fon.10.117 [Google Scholar] [PubMed] [CrossRef]
Elkady AI (2019). Targeting prostate cancer cell proliferation, stemness and metastatic potential using Costus speciosus derived phytochemicals. American Journal of Translational Research 11: 2550–2569. [Google Scholar] [PubMed]
Foo KT (2019). What is a disease? What is the disease clinical benign prostatic hyperplasia (BPH)? World Journal of Urology 37: 1293–1296. https://doi.org/10.1007/s00345-019-02691-0 [Google Scholar] [PubMed] [CrossRef]
Füllhase C, Schneider MP (2016). 5-Alpha-reductase inhibitors and combination therapy. The Urologic Clinics of North America 43: 325–336. https://doi.org/10.1016/j.ucl.2016.04.003 [Google Scholar] [PubMed] [CrossRef]
Hong GL, Park SR, Jung DY, Karunasagara S, Lee KP, Koh EJ, Cho K, Park SS, Jung JY (2020). The therapeutic effects of Stauntonia hexaphylla in benign prostate hyperplasia are mediated by the regulation of androgen receptors and 5α-reductase type 2. Journal of Ethnopharmacology 250: 112446. https://doi.org/10.1016/j.jep.2019.112446 [Google Scholar] [PubMed] [CrossRef]
Ilic D, Misso M (2012). Lycopene for the prevention and treatment of benign prostatic hyperplasia and prostate cancer: A systematic review. Maturitas 72: 269–276. https://doi.org/10.1016/j.maturitas.2012.04.014 [Google Scholar] [PubMed] [CrossRef]
Kang XC, Chen T, Zhou JL, Shen PY, Dai SH, Gao CQ, Zhang JY, Xiong XY, Liu DB (2021). Phytosterols in hull-less pumpkin seed oil, rich in ∆(7)-phytosterols, ameliorate benign prostatic hyperplasia by lowing 5α-reductase and regulating balance between cell proliferation and apoptosis in rats. Food & Nutrition Research 65: 7537. https://doi.org/10.29219/fnr.v65.7537 [Google Scholar] [PubMed] [CrossRef]
Karunasagara S, Hong GL, Jung DY, Kim KH, Cho K, Jung JY (2020). Protective effects of combination of Stauntonia hexaphylla and Cornus officinalis on testosterone-induced benign prostatic hyperplasia through inhibition of 5α-reductase type 2 and induced cell apoptosis. PLoS One 15: e0236879. https://doi.org/10.1371/journal.pone.0236879 [Google Scholar] [PubMed] [CrossRef]
Lerner LB, McVary KT, Barry MJ, Bixler BR, Dahm P et al. (2021). Management of lower urinary tract symptoms attributed to benign prostatic hyperplasia: AUA GUIDELINE PART I-initial work-up and medical management. The Journal of Urology 206: 806–817. https://doi.org/10.1097/JU.0000000000002183 [Google Scholar] [PubMed] [CrossRef]
Mori A, Lehmann S, O’Kelly J, Kumagai T, Desmond JC, Pervan M, McBride WH, Kizaki M, Koeffler HP (2006). Capsaicin, a component of red peppers, inhibits the growth of androgen-independent, p53 mutant prostate cancer cells. Cancer Research 66: 3222–3229. https://doi.org/10.1158/0008-5472.CAN-05-0087 [Google Scholar] [PubMed] [CrossRef]
Park J, Youn DH, Um JY (2019). Aconiti Lateralis Radix Preparata, the dried root of Aconitum carmichaelii Debx., improves benign prostatic hyperplasia via suppressing 5-alpha reductase and inducing prostate cell apoptosis. Evidence-Based Complementary and Alternative Medicine 2019: 6369132. https://doi.org/10.1155/2019/6369132 [Google Scholar] [PubMed] [CrossRef]
Persson MSM, Stocks J, Walsh DA, Doherty M, Zhang W (2018). The relative efficacy of topical non-steroidal anti-inflammatory drugs and capsaicin in osteoarthritis: A network meta-analysis of randomised controlled trials. Osteoarthritis and Cartilage 26: 1575–1582. https://doi.org/10.1016/j.joca.2018.08.008 [Google Scholar] [PubMed] [CrossRef]
Plochocki A, King B (2022). Medical treatment of benign prostatic hyperplasia. The Urologic Clinics of North America 49: 231–238. https://doi.org/10.1016/j.ucl.2021.12.003 [Google Scholar] [PubMed] [CrossRef]
Qiao J, Gan Y, Gong Y, Song Q, Zhang B, Li B, Ru F, Li Y, He Y (2021). Combination therapy with curcumin plus tamsulosin and finasteride in the treatment of men with benign prostatic hyperplasia: A single center, randomized control study. Translational Andrology and Urology 10: 3432–3439. https://doi.org/10.21037/tau-21-567 [Google Scholar] [PubMed] [CrossRef]
Qin L, Wang Y, Gong Y, Chen J, Xu B, Tang L, Guo L, Xie J (2019). Capsaicin metabolites and GSH-associated detoxification and biotransformation pathways in human liver microsomes revealed by LC-HRMS/MS with data-mining tools. Journal of Chromatography B 1133: 121843. https://doi.org/10.1016/j.jchromb.2019.121843 [Google Scholar] [PubMed] [CrossRef]
Qiu D, Zhou M, Lin T, Chen J, Wang G, Huang Y, Jiang X, Tian W, Chen H (2019). Cytotoxic components from hypericum elodeoides targeting RXRα and inducing HeLa cell apoptosis through caspase-8 activation and PARP cleavage. Journal of Natural Products 82: 1072–1080. https://doi.org/10.1021/acs.jnatprod.8b00680 [Google Scholar] [PubMed] [CrossRef]
Ramos-Torres Á, Bort A, Morell C, Rodríguez-Henche N, Díaz-Laviada I (2016). The pepper’s natural ingredient capsaicin induces autophagy blockage in prostate cancer cells. Oncotarget 7: 1569–1583. https://doi.org/10.18632/oncotarget.6415 [Google Scholar] [PubMed] [CrossRef]
Sachan R, Kundu A, Jeon Y, Choi WS, Yoon K, Kim IS, Kwak JH, Kim HS (2018). Afrocyclamin A, a triterpene saponin, induces apoptosis and autophagic cell death via the PI3K/Akt/mTOR pathway in human prostate cancer cells. Phytomedicine 51: 139–150. https://doi.org/10.1016/j.phymed.2018.10.012 [Google Scholar] [PubMed] [CrossRef]
Sanati S, Razavi BM, Hosseinzadeh H (2018). A review of the effects of Capsicum annuum L. and its constituent, capsaicin, in metabolic syndrome. Iranian Journal of Basic Medical Sciences 21: 439–448. https://doi.org/10.22038/ijbms.2018.25200.6238 [Google Scholar] [PubMed] [CrossRef]
Sharma SK, Vij AS, Sharma M (2013). Mechanisms and clinical uses of capsaicin. European Journal of Pharmacology 720: 55–62. https://doi.org/10.1016/j.ejphar.2013.10.053 [Google Scholar] [PubMed] [CrossRef]
Srinivasan K (2016). Biological activities of red pepper (Capsicum annuum) and its pungent principle capsaicin: A review. Critical Reviews in Food Science and Nutrition 56: 1488–1500. https://doi.org/10.1080/10408398.2013.772090 [Google Scholar] [PubMed] [CrossRef]
Sánchez BG, Bort A, Mateos-Gómez PA, Rodríguez-Henche N, Díaz-Laviada I (2019). Combination of the natural product capsaicin and docetaxel synergistically kills human prostate cancer cells through the metabolic regulator AMP-activated kinase. Cancer Cell International 19: 54. https://doi.org/10.1186/s12935-019-0769-2 [Google Scholar] [PubMed] [CrossRef]
Sánchez BG, Bort A, Mora-Rodríguez JM, Díaz-Laviada I (2022). The natural chemotherapeutic capsaicin activates AMPK through LKB1 kinase and TRPV1 receptors in prostate cancer cells. Pharmaceutics 14: 329. https://doi.org/10.3390/pharmaceutics14020329 [Google Scholar] [PubMed] [CrossRef]
Sánchez AM, Malagarie-Cazenave S, Olea N, Vara D, Chiloeches A, Díaz-Laviada I (2007). Apoptosis induced by capsaicin in prostate PC-3 cells involves ceramide accumulation, neutral sphingomyelinase, and JNK activation. Apoptosis 12: 2013–2024. https://doi.org/10.1007/s10495-007-0119-z [Google Scholar] [PubMed] [CrossRef]
Tanida I, Ueno T, Kominami E (2008). LC3 and autophagy. Methods in Molecular Biology 445: 77–88. https://doi.org/10.1007/978-1-59745-157-4 [Google Scholar] [CrossRef]
Xu HD, Qin ZH (2019). Beclin 1, Bcl-2 and autophagy. Advances in Experimental Medicine and Biology 1206: 109–126. https://doi.org/10.1007/978-981-15-0602-4 [Google Scholar] [CrossRef]
Yang F, Zheng J (2017). Understand spiciness: Mechanism of TRPV1 channel activation by capsaicin. Protein & Cell 8: 169–177. https://doi.org/10.1007/s13238-016-0353-7 [Google Scholar] [PubMed] [CrossRef]
Zheng L, Chen J, Ma Z, Liu W, Yang F, Yang Z, Wang K, Wang X, He D, Li L (2015). Capsaicin causes inactivation and degradation of the androgen receptor by inducing the restoration of miR-449a in prostate cancer. Oncology Reports 34: 1027–1034. https://doi.org/10.3892/or.2015.4055 [Google Scholar] [PubMed] [CrossRef]
Zheng J, Zheng S, Feng Q, Zhang Q, Xiao X (2017). Dietary capsaicin and its anti-obesity potency: From mechanism to clinical implications. Bioscience Reports 37: BSR20170286. https://doi.org/10.1042/BSR20170286 [Google Scholar] [PubMed] [CrossRef]
Zou Y, Aboshora W, Li J, Xiao T, Zhang L (2017). Protective effects of Lepidium meyenii (Maca) aqueous extract and lycopene on testosterone propionate-induced prostatic hyperplasia in mice. Phytotherapy Research 31: 1192–1198. https://doi.org/10.1002/ptr.5838 [Google Scholar] [PubMed] [CrossRef]
Cite This Article
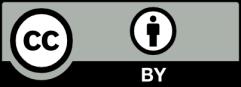