Open Access
ARTICLE
A Brachypodium distachyon calcineurin B-like protein-interacting protein kinase, BdCIPK26, enhances plant adaption to drought and high salinity stress
1 Hubei Key Laboratory of Purification and Application of Plant Anti-Cancer Active Ingredients, Department of Chemistry and Life Science, Hubei University of Education, Wuhan, China
2 School of Medicine, Wuhan University of Science and Technology, Wuhan, China
* Corresponding Author: QINGCHEN LUO. Email:
# These authors contributed equally to this work
BIOCELL 2023, 47(5), 1145-1158. https://doi.org/10.32604/biocell.2023.027847
Received 17 November 2022; Accepted 16 January 2023; Issue published 10 April 2023
Abstract
As sessile organisms, plants possess a complex system to cope with environmental changes. Ca2+ functions as a vital second messenger in the stress signaling of plants, and the CBL-interacting protein kinases (CIPKs) serve as essential elements in the plant Ca2+ signaling pathway. In this study, calcineurin B-like protein-interacting protein kinase 26 (BdCIPK26) from Brachypodium distachyon was characterized. Overexpression of BdCIPK26 enhanced tolerance to drought and salt stress of transgenic plants. Further investigations revealed that BdCIPK26 participated in abscisic acid (ABA) signaling, conferred hypersensitivity to exogenous ABA in transgenic plants, and promoted endogenous ABA biosynthesis. Moreover, BdCIPK26 was found to maintain ROS homeostasis in plants under stress conditions. Therefore, this study indicates that BdCIPK26 functions as a positive regulator in drought and salt stress response.Keywords
Global climate change tends to be extreme and causes plants to face drought or salt stress more frequently (Simpkins, 2021). As sessile organisms, plants have to passively adapt to the environment. Thus, precise control of stress signal transduction and response is crucial for plants (Zhang et al., 2022). As an indispensable second messenger, Ca participates in the plant stress-response regulatory network (Zhang et al., 2018b). Drought, high salt, cold, and heat trigger the alternation of cytosolic Ca2+ concentration, transforming direct stress signal to Ca2+ signal (Zhu, 2016). The calcineurin B-like proteins (CBLs) are unique Ca2+ sensors for plants. The CBL-interacting protein kinases (CIPKs) are responders for CBLs, and the CBLs can interact with CIPKs after binding to Ca2+. The CBL-CIPK complex then acts on various substrates soon afterward (Yu et al., 2014). The functional studies of CIPK involve the regulation of growth, developmental control, plant nutrition, and stress response (Ding et al., 2022). For example, tonoplast-localized CBL2 and CBL3 interact with CIPK12, thus participating in modulating polarized pollen tube growth (Steinhorst et al., 2015). Brachypodium distachyon calcineurin B-like protein-interacting protein kinases (BdCIPK31) promotes plant potassium uptake, conferring tolerance to enhanced low potassium stress (Luo et al., 2022). However, until now, the research on CIPK function has focused mainly on the stress response. The salt overly sensitive (SOS) pathway plays an important role in the salt stress response of plants. The Ca2+ sensor SOS3 (CBL4) interacts with SOS2 (CIPK24), forming SOS3-SOS2 complex phosphorylated Na+/H+ antiporter SOS1 (NHX7), enhancing Na+ extrusion under high salinity conditions (Liu and Zhu, 1998; Halfter et al., 2000; Qiu et al., 2002). After that, more CIPKs have been found to participate in stress response regulation. Hordeum brevisubulatum HbCIPK2 could enhance osmotic and salt stress resistance in Arabidopsis (Li et al., 2012). Likewise, transient overexpression of pepper CaCIPK3 promoted drought tolerance (Ma et al., 2021b). Therefore, it is important from a research point of view to discover more stress-related CIPKs.
In addition to Ca2+ signaling, plants also transmit stress signals through other signaling pathways. Among these signaling pathways, abscisic acid (ABA) and reactive oxygen species (ROS) are essential elements. Abiotic stress causes ABA synthesis (Boominathan et al., 2004), and the increased ABA content leads to stomatal closure (Kim et al., 2010), inhibition of germination (Thole et al., 2014), and growth repression (Sharp et al., 2004). ROS production is also induced by environmental stress (Mittler and Blumwald, 2010). A moderate amount of ROS act as signal molecules that modulate plant growth and stress response (Apel and Hirt, 2004). However, ROS overaccumulation is toxic to plants, causing oxidative damage to cells (Gill and Tuteja, 2010). The CIPKs are found to be involved in the crosstalk between ABA/Ca2+ signaling and ROS/Ca2+ signaling. Under water deficiency soybean GmCBL1-GmCIPK2 localized to the plasma membrane, participated in ABA-mediated stomatal movement and induced the transcription of ABA-related genes (Xu et al., 2021). Another soybean CIPK, GmCIPK21, enhanced ABA sensitivity and induced ABA-related genes under high salinity (Li et al., 2022). Furthermore, overexpression of GmCIPK21 elevated the antioxidative enzyme activity and contributed to maintaining ROS homeostasis (Li et al., 2022).
B. distachyon is a model plant of the Pooideae subfamily, the largest subfamily of grasses, which includes most seasonal cereals, forage, and turf grasses (Vogel et al., 2010). B. distachyon is more tolerant to abiotic stress than its related species, like cultivated rice (Luo et al., 2011). Therefore, B. distachyon is a valuable model species for exploring stress tolerance genes. In our previous study, the stress-repressed gene BdCIPK31 was found to confer tolerance to drought, high salinity, and cold stress in transgenic plants (Luo et al., 2017, 2018). However, due to the contradiction between expression and phenotype, the BdCIPK31 was hypothesized as a redundant member in stress response modulation. In the present study, we characterized BdCIPK26, a stress-induced CIPK gene in B. distachyon. BdCIPK26 positively regulates drought and salt response of plants. Overexpressed BdCIPK26 conferred hypersensitivity to ABA and promoted ABA biosynthesis under stress treatments. Moreover, BdCIPK26 promoted ROS-scavenging ability and accumulation of osmoprotectants and modulated the expression of stress-related transcription factors. In summary, our study demonstrated that BdCIPK26 functions positively in plant response to drought and salt stress.
B. distachyon inbred line Bd21 was selected to isolate and analyze BdCIPK26. The seeds of B. distachyon were sterilized by ethanol treatment. Afterward, the seeds were germinated at room temperature. The B. distachyon seedlings were cultured in modified Hoagland solution at 25°C, in a 16/8 h light/dark cycle. The Nicotina tabacum cv. Samsun was employed to generate transgenic tobaccos overexpressing BdCIPK26. The coding sequence (CDS) of BdCIPK26 (Suppl. Fig. S1) was amplified by polymerase chain reaction (PCR) using gene-specific primers with BglII (forward) and SpeI (reverse) restriction sites (Suppl. Table S1), and the cDNA obtained from normally growing 10-day-old B. distachyon seedlings was used as PCR template. Afterward, the cloned CDS was introduced into pCambia1303, downstream of the CaMV 35S promoter. The recombinant vector was then transformed into Agrobacterium strain EHA105 for Agrobacterium-mediated transformation (Sparkes et al., 2006). The plasmid pCambia1303 was also introduced into tobaccos to generate the vector control (VC) lines. The T0 transgenic seedlings were screened in half-strength Murashige and Skoog (1/2 MS) medium containing 20 mg/L hygromycin and further verified by PCR using gene-specific primers (Suppl. Table S1). The transcript level of BdCIPK26 in transgenic tobaccos of T2 generation was analyzed by quantitative-PCR (qPCR). Two T2 homozygote lines of these transgenic tobaccos (OX lines) were used in this study.
For treatments of B. distachyon seedlings, ten-day-old seedlings were treated with 20% (w/v) PEG6000, 200 mM NaCl, 10 mM H2O2, or 100 μM ABA. The treated samples were collected and divided into shoots and roots for RNA preparation.
For treatments of tobacco plants, watering of one-month-old tobacco plants were stopped or watered with 300 mM NaCl solution. The leaf samples were collected 3 days after treatments. The survival rates were calculated after treatments.
Phylogenic and multiple sequence alignment assay
The BdCIPK26 homologs were searched by NCBI BlastP (https://blast.ncbi.nlm.nih.gov/Blast.cgi). BdCIPK26 functional domains and motifs were analyzed using SMART tools (http://smart.embl-heidelberg.de) (Letunic et al., 2021). Multiple sequence alignment was performed by ClustalX. BdCIPKs and OsCIPKs phylogenetically analyzed by MEGA using the maximum likelihood method (Kumar et al., 2018), the sequences of the OsCIPKs were obtained from Xiang et al. (2007).
Subcellular localization assay
The BdCIPK26-pCambia1303 was introduced into N. tabacum leaves by Agrobacterium-mediated transformation. The BdCIPK26::green fluorescent protein (GFP) fusion protein was transiently expressed in the tobacco leaf epidermis and observed by fluorescence microscopy (Olympus IX71, Japan).
Gene expression and promoter analysis
The treated samples of B. distachyon seedlings were subjected to RNA extraction using Total RNA Extraction Kit (Solarbio, Beijing, China). The cDNA was synthesized by FastQuant RT Kit (TIANGEN, Beijing, China). The quantitative real-time PCR was performed to detect the expression of BdCIPK26 using 2 × SY BR Green PCR Mastermix (Solarbio, Beijing, China) on an ABI StepOnePlus Real-Time PCR System (Thermo Fisher, USA). The Bdactin gene was applied as endogenous control. The primers used in this assay are listed in Suppl. Table S1. The data were analyzed by the 2−ΔΔCT method (Livak and Schmittgen, 2001).
The promoter sequence of BdCIPK26 was obtained from Ensembl Plants database (http://plants.ensembl.org/index.html). The promoter sequence was analyzed by the PlantCARE database (http://bioinformatics.psb.ugent.be/webtools/plantcare/html/) (Lescot et al., 2002).
Tobacco seeds were germinated on 1/2 MS medium with 20 mg/L hygromycin. After germination, the seeds were transferred into 1/2 MS medium containing 200 mM mannitol, 150 mM NaCl, or 2.5 μM ABA for stress treatments. The root length and fresh weight were examined after each treatment.
Estimation of abscisic acid content
Tobacco leaf samples were collected 3 days after drought or salt treatment. The samples were pretreated as previously described (Yan et al., 2014), and the ABA content was determined by the ABA content detection ELISA kit (Jiancheng, Nanjing, China).
Reactive oxygen species-scavenging enzyme activity, H2O2 content, ion leakage, malondialdehyde (MDA) content, enzyme activity, and osmolyte content assay
Tobacco leaf samples used in these assays were collected 3 days after drought or salt treatment. The H2O2 content was determined by the H2O2 content detection kit (molybdic acid method, Jiancheng, Nanjing, China). The MDA content was determined by the MDA assay kit (TBA method, Jiancheng, Nanjing, China). The ion leakage was determined by the conductivity method as described before (Luo et al., 2017). The activities of the antioxidative enzymes were determined using corresponding kits (Jiancheng, Nanjing, China). The contents of proline and soluble sugar were also determined using suitable kits (Jiancheng, Nanjing, China).
Assay for the expression of stress-related genes
Tobacco leaf samples used in these assays were collected 3 days after drought or salt treatment. The RNA preparation was performed as mentioned above. The primers used in this assay are listed in Suppl. Table S1.
The data was analyzed by SPSS software (Chicago, IL, USA). The significance tests were performed by student’s t-test.
Characterization of Brachypodium distachyon calcineurin B-like protein-interacting protein kinase
The BdCIPK26 had been identified previously (Wang et al., 2015). Phylogenic analysis of BdCIPK26 and OsCIPKs showed the closest relationship between BdCIPK26 and OsCIPK26 (Fig. 1A). Multiple sequence alignment suggested that BdCIPK26 contains CIPK feature domains such as activation loop, the CBL interaction motif (NAF/FISL motif), and protein–phosphatase interaction domain (Fig. 1B).
Figure 1: Characterization of Brachypodium distachyon calcineurin B-like protein-interacting protein kinases (BdCIPK26). (A) Phylogenic analysis of BdCIPKs and OsCIPKs. The red color indicates OsCIPK26 and BdCIPK26. (B) Multiple sequence alignment of BdCIPK26, OsCIPK26, and TaCIPK26 (Sun et al., 2015). Black shadows indicate the same amino acid residues, and gray shadows indicate similar amino acid residues. The typical motifs of CIPKs are indicated by lines. (C) Subcellular localization of BdCIPK26. The GFP protein in the left column is applied as a positive control, and the fluorescence of the BdCIPK26::GFP fusion protein is shown in the right column. Three independent experiments exhibited the same results.
The subcellular localization assays were performed by introducing BdCIPK26::GFP fusion plasmids into tobacco leaves, and no specific localization of BdCIPK26 was observed in the tobacco epidermis cells (Fig. 1C).
Expression patterns of Brachypodium distachyon calcineurin B-like protein-interacting protein kinases (BdCIPK26)
The qPCR analyses were performed to determine whether the transcription of BdCIPK26 was responsive to abiotic stress and ABA treatments. The results suggested significant induction of BdCIPK26 by PEG6000, NaCl, H2O2, and ABA treatments in both shoots and roots of B. distachyon seedlings (Fig. 2). Analysis of the promoter sequence of BdCIPK26 revealed various stress-related cis-elements, including ABRE and DRE (Suppl. Table S2). These results suggested that BdCIPK26 was induced by osmotic, salt, oxidative stress, and ABA treatment.
Figure 2: The expression profiles of BdCIPK26. (A and B) The expression of BdCIPK26 in shoots or roots of Brachypodium distachyon seedlings under 20% PEG6000 treatment. (C and D) The expression of BdCIPK26 in shoots or roots of B. distachyon seedlings under 200 mM NaCl treatment. (E and F) The expression of BdCIPK26 in the shoots or roots of B. distachyon seedlings under 10 mM H2O2 treatment. (G and H) The expression of BdCIPK26 in the shoots or roots of B. distachyon seedlings under 100 μM ABA treatment. The bars exhibit the mean ± SE of three biological replicates (n = 3). The asterisks indicate a significant difference compared to the control (*p < 0.05, **p < 0.01).
Brachypodium distachyon calcineurin B-like protein-interacting protein kinases conferred enhanced drought and salt adaption in transgenic tobaccos
Since the transcription level of BdCIPK26 was induced by osmotic and salt stress treatment, we speculated the role of BdCIPK26 in plant response to corresponding stresses. Transgenic tobacco plants overexpressing the BdCIPK26 gene were generated as experimental materials. The expression of BdCIPK26 in six transgenic lines was detected by qPCR (Suppl. Fig. S2B). The T2 homozygous lines OX3 and OX5 were selected for the functional characterization of BdCIPK26.
The tobacco seeds were germinated on 1/2 MS medium and then moved to treatment medium containing 200 mM mannitol or 150 mM NaCl. After two weeks of stress treatment, the OX seedlings exhibited better growth status than the WT and VC control seedlings (Fig. 3A). The results were verified by root length and fresh weight assessments. The transgenic seedlings overexpressing BdCIPK26 displayed longer tap roots (Fig. 3B) and higher fresh weight (Fig. 3C) than the controls, suggesting that BdCIPK26 enhanced plant adaption to osmotic and high salinity stress in the seedling stage.
Figure 3: Phenotype analysis of transgenic tobacco seedlings under osmotic or high salinity stress. (A) The tobacco seedlings grown on an untreated 1/2 Murashige and Skoog (MS) medium (upper panel), 1/2 MS medium containing 200 mM mannitol (middle panel), and 1/2 MS medium containing 150 mM NaCl (lower panel). (B) The average root length of seedlings under mannitol or NaCl treatment. (C) The fresh weight analysis of seedlings under mannitol or NaCl treatment. The bars exhibit the mean ± SE of three biological replicates (n = 3). The asterisks indicate significant difference compared to the control (*p < 0.05, **p < 0.01).
In the vegetative growth stage, the watering of 28-day-old tobacco plants was stopped for drought treatment. After 40 days of treatment, all the tobacco plants were shriveled, especially the control plants, while the leaves of OX plants were still green and unfolded (Fig. 4A). All treated plants were rewatered for 21 days, and more than 30% OX plants were recovered, whereas less than 10% control plants survived after drought treatment (Fig. 4B).
Figure 4: The phenotype analysis of tobacco plants under drought or salt stress. (A) The tobacco plants grown in normal conditions (upper panel), in drought or high salinity treatment (middle panel), and were rewatered (lower panel). (B) The survival rate of tobacco plants after stress treatment. (C) The chlorophyll contents in tobacco leaves under high salinity treatment. The bars in (B) and (C) exhibit the mean ± SE of three biological replicates (n = 3). The asterisks indicate a significant difference compared to the control (*p < 0.05, **p < 0.01).
In the high salinity stress assay, tobacco plants were irrigated with 300 mM NaCl for 14 days. The treated plants exhibited phenotype representing salt damage, such as wilted and yellowing, whereas the leaves of OX plants remained greener than that in controls (Fig. 4A). About 22% of the OX3 line plants and 25% of the OX5 line plants survived after high salinity treatment. In contrast, only less than 10% of the control plants survived after the same treatment (Fig. 4B). In addition, the OX plants contained more chlorophyll than the controls during salt treatment (Fig. 4C), indicating that BdCIPK26 overexpression functioned in plant tolerance to salt stress.
Brachypodium distachyon calcineurin B-like protein-interacting protein kinases enhanced abscisic acid sensitivity and biosynthesis in plants
To identify whether BdCIPK26 participates in ABA signaling, the transgenic seedlings were subjected to 2.5 μM exogenous ABA. The OX seedlings exhibited a hypersensitive phenotype in the ABA treatment (Fig. 5A), while the control seedlings showed longer tap roots than those in OX seedlings (Fig. 5B). Further analysis revealed that transgenic tobacco plants overexpressing BdCIPK26 contained more endogenous ABA under drought or salt conditions (Fig. 5C), indicating the participation of BdCIPK26 in ABA synthesis. This result had been confirmed at the mRNA level. The expressions of NtNCED1 and NtNCED3 in tobacco plants were elevated by BdCIPK26 overexpression under the same conditions (Figs. 5D and 5E).
Figure 5: Analyses of abscisic acid (ABA) response, ABA content, and ABA synthesis-related gene expression in tobacco seedlings. (A) Tobacco seedlings under 2.5 μM ABA treatment. (B) The average root length of tobacco seedlings exposed to exogenous ABA. (C) ABA content in tobacco leaves under drought or high salinity treatment. (D) The expression of NtNCED1 in tobacco plants under drought or high salinity treatment. (E) The expression of NtNCED3 in tobacco plants under drought or high salinity treatment. The bars in (B–E) represent the mean ± SE of three biological replicates (n = 3). The asterisks indicate a significant difference compared to the control (*p < 0.05, **p < 0.01).
Brachypodium distachyon calcineurin B-like protein-interacting protein kinases alleviated oxidative damage in plants under drought and high salinity
Abiotic stresses trigger ROS accumulation and cause oxidative damage to plants (Rodrigo-Moreno et al., 2013). Induction of BdCIPK26 transcripts by H2O2 treatment (Figs. 2E and 2F) implied that BdCIPK26 may be involved in ROS homeostasis. In the present study, H2O2 content was examined to estimate the ROS level. Ion leakage and MDA content were analyzed to assess oxidative damage. BdCIPK26 overexpression reduced H2O2 content in transgenic tobacco plants under drought or salt treatment (Fig. 6A). Furthermore, ion leakage and MDA content level were lowered in transgenic tobacco plants (Figs. 6B and 6C), suggesting that BdCIPK26 alleviated the membrane damage caused by ROS overaccumulation.
Figure 6: Analyses of reactive oxygen species (ROS)-related indexes. (A–C) H2O2 content (A), relative ion leakage (B), and Malondialdehyde (MDA) content (C) in tobacco plants under stress treatments. (D–I) Catalase (CAT) (D), guaiacol peroxidase (POD) (E), superoxide dismutase SOD (F), and glutathione s-transferase (GST) (G) activities in tobacco plants under stress treatments. (H–I) Ascorbic acid content (H) and relative anthocyanin content (I) in tobacco plants under stress treatments. The bars exhibit the mean ± SE of three biological replicates (n = 3). The asterisks indicate a significant difference compared to the control (*p < 0.05, **p < 0.01).
Plant ROS-scavenging system consists of antioxidative enzymes and antioxidants. In this study, catalase (CAT), guaiacol peroxidase (POD), superoxide dismutase (SOD), glutathione s-transferase (GST), ascorbic acid, and anthocyanin were selected as the representative members of ROS-scavenging system. The enzyme activity assay revealed that the activities of CAT, POD, SOD, and GST were elevated by BdCIPK26 overexpression in the transgenic tobaccos under stress treatments (Figs. 6D–6G). Additionally, the treated transgenic tobaccos also showed higher ascorbic acid and anthocyanin content (Figs. 6H and 6I), suggesting the participation of BdCIPK26 in ROS-scavenging regulation. These effects were also verified at the transcriptional level; the transcripts of NtCAT1, NtPOX2, NtSOD1, NtGST, NtAPX, and NtDFR were all induced by BdCIPK26 overexpression under stress conditions (Suppl. Fig. S3).
Brachypodium distachyon calcineurin B-like protein-interacting protein kinases enhanced osmolyte accumulation in plants
Osmotic pressure unbalance is caused by water and salt stress. The production and accumulation of osmoprotectants such as proline and soluble sugar can maintain the osmotic equilibrium of plant cells. To investigate whether BdCIPK26 functions in osmolytes accumulation, the proline and soluble sugar contents were examined. The results showed higher proline, and soluble sugar content in transgenic tobaccos than the control plants under drought or salt stress treatment (Figs. 7A and 7C). Furthermore, the expressions of proline synthesis-related gene NtP5CS1 and sucrose synthesis-related gene NtSUS1 were also elevated due to BdCIPK26 overexpression under the same conditions (Figs. 7B and 7D). These results indicated that BdCIPK26 enhanced the accumulation of osmoprotectants.
Figure 7: Analyses of osmolytes in tobacco plants. (A) The proline content in tobacco plants under stress treatments. (B) The level of NtP5CS1 mRNA in tobacco plants under drought or salt stress. (C) The soluble sugar content in tobacco plants under drought or salt stress. (D) The expression of NtSUS1 in tobacco plants under drought or salt stress. The bars exhibit the mean ± SE of three biological replicates (n = 3). The asterisks indicate a significant difference compared to the control (*p < 0.05, **p < 0.01).
Brachypodium distachyon calcineurin B-like protein-interacting protein kinases affected the transcription of stress-related transcription factors
The transcripts of some typical transcription factors related to abiotic stress response were examined, the ABA-responsive element binding factor NtABF2 and the dehydration-responsive element binding protein NtDREB3 exhibited higher transcripts under stress treatments (Suppl. Fig. S4). These results indicated that BdCIPK26 can modulate the expressions of stress-related transcription factors.
The Ca2+ signaling pathway is one of the most important signaling pathways which regulate the physiological and transcriptional processes of plants. As unique members in plant Ca2+ signaling, CBL-CIPK complexes are extensively involved in growth, development, reproduction, and environment adaptation (Mao et al., 2022). The study of CIPK function focuses on plant response to external stresses (Tang et al., 2020). BdCIPK31, a stress-repressed CIPK, was characterized as a potential positive regulator in abiotic stress tolerance and plant nutrition in our previous study (Luo et al., 2017, 2018, 2022). In this study, a multiple-stress-induced CIPK (Fig. 2), BdCIPK26, was discovered. Further, overexpression of BdCIPK26 conferred enhanced adaption to drought and high salinity in plants (Figs. 3 and 4).
As the key phytohormone in stress signal transduction, ABA functions in modulating plant growth under stress conditions (Raghavendra et al., 2010). The CIPKs have been reported to be involved in ABA signaling. Most CIPK genes in potatoes (Solanum tuberosum L.) are induced by ABA treatment (Ma et al., 2021a). AtCIPK26 was found to interact with a series of transcription factors and keep-on-going (KEG), a RING-type E3 ligase, in ABA signaling (Lyzenga et al., 2013). The AtCIPK26 was degraded by the ubiquitin-proteasome system mediated by KEG, and the AtCIPK26-mediated phosphorylation led to ABA-mediated degradation of KEG (Lyzenga et al., 2017). The OsCBL8-OsCIPK17 complex negatively regulates seed germination and seedling growth by interacting with OsPP2C77, which is an ABA-mediated phosphatase (Gao et al., 2022). AcCIPK5 regulates ABA signaling to mediate stress response in Arabidopsis (Aslam et al., 2022). In the present study, BdCIPK26 was found to be induced by ABA treatment (Figs. 2G and 2H). In addition, BdCIPK26 overexpression conferred a hypersensitive phenotype to transgenic tobacco seedlings exposed to exogenous ABA (Figs. 5A and 5B), suggesting that BdCIPK26 functioned as a positive regulator in ABA signaling. Unlike BdCIPK31, which did not affect ABA synthesis (Luo et al., 2017), further investigations revealed that BdCIPK26 enhanced endogenous ABA production under drought and salt stresses (Fig. 5C). The transcription of tobacco 9-cis-epoxycarotenoid dioxygenase genes (NCED), which encode rate-limiting ABA biosynthesis enzymes, were also increased in the treated transgenic plants (Figs. 5D and 5E). These results implied that BdCIPK26 might be an upstream regulator of ABA synthesis.
Besides Ca2+, ROS are also essential second messenger molecules in plant stress signal transduction (Bartels and Sunkar, 2005). Environmental changes trigger the accumulation of ROS and then alter the plant responses to exogenous stimuli (Zhu, 2016). On the other hand, overproduced ROS are reactive in cells and cause oxidative damage to proteins, nucleic acids, organelles, and membranes (You and Chan, 2015). The CIPKs have been reported to affect the production of ROS. The AtCBL1/AtCBL9-AtCIPK26 complexes reduced ROS-producing activity by interacting and phosphorylating NADPH oxidase AtRBOHF (Drerup et al., 2013; Kimura et al., 2013; Lyzenga et al., 2013). The AtCBL1-AtCIPK26 also mediated the phosphorylation of AtRBOHC, enhanced AtRBOHC activity, and affected root hair growth (Zhang et al., 2018a). In addition, the plant ROS-managing system consists of enzymatic and non-enzymatic components responsible for scavenging excess ROS in cells (Gill and Tuteja, 2010). The CIPK protein kinases also take part in the regulation of ROS-managing system. Knockdown of pepper CaCIPK13 resulted in hypersensitivity to cold stress with higher H2O2 content (Ma et al., 2022). GmPKS4, a soybean CIPK, increased the activities of antioxidative enzymes under salt and alkali stress (Ketehouli et al., 2021). The CcCBL1-CcCIPK14 complex in pigeon peas was found to positively modulate drought tolerance by enhancing flavonoid biosynthesis (Meng et al., 2021). In this study, overexpression of BdCIPK26 elevated the activities of representative ROS-scavenging enzymes, including CAT, POD, SOD, and GST, under stress conditions (Figs. 6D–6G). BdCIPK26 also affected the biosynthesis of antioxidants. During drought and salt treatments, transgenic tobacco plants exhibited higher ascorbic acid and anthocyanin levels than the control plants (Figs. 6H and 6I). Furthermore, our study revealed that BdCIPK26 modulates the ROS-scavenging system at the transcriptional level. In stress conditions, the transcripts of NtCAT1, NtPOX2, NtSOD1, and NtGST were induced by BdCIPK26 overexpression (Suppl. Figs. S3A–S3D), together with the transcripts of tobacco ascorbate peroxidase gene NtAPX and dihydroflavonol 4-reductase gene NtDFR (Suppl. Figs. S3E and S3F). Therefore, our results reveal that BdCIPK26 contributes to maintaining ROS homeostasis in plant response to abiotic stress.
Both water shortage and salt stress induce cell dehydration, leading to osmotic stress (Zhu, 2016). Osmotic stress triggers an alternation of cytosolic Ca2+ concentration and transforms the stress signal into a Ca2+ signal (Yuan et al., 2014). Natural osmolytes such as soluble amino acids and sugar play important roles in maintaining cell osmotic pressure. One previous study indicated that wheat TaCBL1-TaCIPK23 enhanced the accumulation of proline and soluble sugar in Arabidopsis under drought stress (Cui et al., 2018). In apples, MdCIPK22 phosphorylated sucrose transporter MdSUT2.2 increased sucrose content in response to dehydration (Ma et al., 2019). In the present study, BdCIPK26 overexpression significantly improved the soluble sugar and proline contents in plants under drought and salt stress (Figs. 7A and 7C), the expression of proline synthesis-related gene NtP5CS1 and sucrose synthesis-related gene NtSUS1 (Figs. 7B and 7D). Therefore, our results suggested that BdCIPK26 had a positive function in adjusting osmolality in plant response to abiotic stress.
In addition to physiological regulation, CIPKs have also been identified to participate in transcriptional regulation (Tang et al., 2020). The abiotic stress-related transcriptional regulatory network can be categorized into ABA-dependent and ABA-independent pathways (Yamaguchi-Shinozaki and Shinozaki, 2006). In our study, a series of transcriptional factors were analyzed at the transcriptional level, and we discovered that the NtABF2 and NtDREB3 transcripts were upregulated by BdCIPK26 (Suppl. Figs. S4A and S4B). The ABA-responsive element binding factor (ABF) is recognized as a key transcriptional factor in ABA-dependent pathway, while the dehydration-responsive element binding protein (DREB) is the primary transcriptional factor in the ABA-independent pathway (Yoshida et al., 2014). Hence, the BdCIPK26 is demonstrated to be a regulator in plant stress-related transcriptional regulatory network.
In conclusion, BdCIPK26 is a positive regulator in plant response to drought and salt stress. The expression of BdCIPK26 is induced by osmotic, salt, H2O2, and ABA treatment. Overexpression of BdCIPK26 enhances plant tolerance to drought and salt stress and alleviates oxidative damage by promoting the activity of the ROS-scavenging system. Additionally, BdCIPK26 participates in transcriptional regulation through both ABA-dependent and ABA-independent pathways.
Funding Statement: This study was funded by the Natural Science Foundation of Hubei Province, China (2019CFB388) and the Guiding Project of Scientific Research Plan of the Hubei Provincial Department of Education (B2022007).
Author Contributions: Q. L. conceived the study. Q. L. and J. F. performed the experiments and analyzed the data. Q. L. wrote the manuscript. X. D. performed the gene expression assays and analyzed the data.
Availability of Data and Materials: All data generated or analyzed during this study are included in this published article (and its supplementary information files).
Ethics Approval: Not applicable.
Conflicts of Interest: The authors declare that they have no conflicts of interest to report regarding the present study.
Supplementary Materials: The supplementary material is available online at DOI 10.32604/biocell.2023.027847.
References
Apel K, Hirt H (2004). Reactive oxygen species: Metabolism, oxidative stress, and signal transduction. Annual Review of Plant Biology 55: 373–399. https://doi.org/10.1146/annurev.arplant.55.031903.141701 [Google Scholar] [PubMed] [CrossRef]
Aslam M, Greaves J, Jakada B, Fakher B, Wang X, Qin YJ (2022). AcCIPK5, a pineapple CBL-interacting protein kinase, confers salt, osmotic and cold stress tolerance in transgenic Arabidopsis. Plant Science 320: 111284. https://doi.org/10.1016/j.plantsci.2022.111284 [Google Scholar] [PubMed] [CrossRef]
Bartels D, Sunkar R (2005). Drought and salt tolerance in plants. Critical Reviews in Plant Sciences 24: 23–58. https://doi.org/10.1080/07352680590910410 [Google Scholar] [CrossRef]
Boominathan P, Shukla R, Kumar A, Manna D, Negi D, Verma PK, Chattopadhyay D (2004). Long term transcript accumulation during the development of dehydration adaptation in Cicer arietinum. Plant Physiology 135: 1608–1620. https://doi.org/10.1104/pp.104.043141 [Google Scholar] [PubMed] [CrossRef]
Cui XY, Du YT, Fu JD, Yu TF, Wang CT, Chen M, Chen J, Ma YZ, Xu ZS (2018). Wheat CBL-interacting protein kinase 23 positively regulates drought stress and ABA responses. BMC Plant Biology 18: 93. https://doi.org/10.1186/s12870-018-1306-5 [Google Scholar] [PubMed] [CrossRef]
Ding X, Liu B, Sun X, Sun X, Zheng C (2022). New functions of CIPK gene family are continue to emerging. Molecular Biology Reports 49: 6647–6658. https://doi.org/10.1007/s11033-022-07255-x [Google Scholar] [PubMed] [CrossRef]
Drerup MM, Schlucking K, Hashimoto K, Manishankar P, Steinhorst L, Kuchitsu K, Kudla J (2013). The calcineurin B-like calcium sensors CBL1 and CBL9 together with their interacting protein kinase CIPK26 regulate the arabidopsis NADPH oxidase RBOHF. Molecular Plant 6: 559–569. https://doi.org/10.1093/mp/sst009 [Google Scholar] [PubMed] [CrossRef]
Gao C, Lu S, Zhou R, Wang Z, Li Y, Fang H, Wang B, Chen M, Cao Y (2022). The OsCBL8-OsCIPK17 module regulates seedling growth and confers resistance to heat and drought in rice. International Journal of Molecular Sciences 23: 12451. https://doi.org/10.3390/ijms232012451 [Google Scholar] [PubMed] [CrossRef]
Gill SS, Tuteja N (2010). Reactive oxygen species and antioxidant machinery in abiotic stress tolerance in crop plants. Plant Physiology Biochemistry 48: 909–930. https://doi.org/10.1016/j.plaphy.2010.08.016 [Google Scholar] [PubMed] [CrossRef]
Halfter U, Ishitani M, Zhu JK (2000). The Arabidopsis SOS2 protein kinase physically interacts with and is activated by the calcium-binding protein SOS3. Proceedings of the National Academy of Sciences of the United States of America 97: 3735–3740. https://doi.org/10.1073/pnas.040577697 [Google Scholar] [CrossRef]
Ketehouli T, Zhou YG, Dai SY, Carther KFI, Sun DQ et al. (2021). A soybean calcineurin B-like protein-interacting protein kinase, GmPKS4, regulates plant responses to salt and alkali stresses. Journal of Plant Physiology 256: 153331. https://doi.org/10.1016/j.jplph.2020.153331 [Google Scholar] [PubMed] [CrossRef]
Kim TH, Böhmer M, Hu H, Nishimura N, Schroeder JI (2010). Guard cell signal transduction network: Advances in understanding abscisic acid, CO2, and Ca2+ signaling. Annual Review of Plant Biology 61: 561–591. https://doi.org/10.1146/annurev-arplant-042809-112226 [Google Scholar] [PubMed] [CrossRef]
Kimura S, Kawarazaki T, Nibori H, Michikawa M, Imai A, Kaya H, Kuchitsu K (2013). The CBL-interacting protein kinase CIPK26 is a novel interactor of Arabidopsis NADPH oxidase AtRbohF that negatively modulates its ROS-producing activity in a heterologous expression system. Journal of Biochemistry 153: 191–195. https://doi.org/10.1093/jb/mvs132 [Google Scholar] [PubMed] [CrossRef]
Kumar S, Stecher G, Li M, Knyaz C, Tamura K (2018). MEGA X: Molecular evolutionary genetics analysis across computing platforms. Molecular Biology and Evolution 35: 1547–1549. https://doi.org/10.1093/molbev/msy096 [Google Scholar] [PubMed] [CrossRef]
Lescot M, Déhais P, Thijs G, Marchal K, Moreau Y, van de Peer Y, Rouzé P, Rombauts S (2002). PlantCARE, a database of plant cis-acting regulatory elements and a portal to tools for in silico analysis of promoter sequences. Nucleic Acids Research 30: 325–327. https://doi.org/10.1093/nar/30.1.325 [Google Scholar] [PubMed] [CrossRef]
Letunic I, Khedkar S, Bork P (2021). SMART: Recent updates, new developments and status in 2020. Nucleic Acids Research 49: D458–D460. https://doi.org/10.1093/nar/gkaa937 [Google Scholar] [PubMed] [CrossRef]
Li H, Wang XH, Li Q, Xu P, Liu ZN, Xu M, Cui XY (2022). GmCIPK21, a CBL-interacting protein kinase confers salt tolerance in soybean (Glycine max. L). Plant Physiology Biochemistry 184: 47–55. https://doi.org/10.1016/j.plaphy.2022.05.027 [Google Scholar] [PubMed] [CrossRef]
Li R, Zhang J, Wu G, Wang H, Chen Y, Wei J (2012). HbCIPK2, a novel CBL-interacting protein kinase from halophyte Hordeum brevisubulatum, confers salt and osmotic stress tolerance. Plant Cell & Environment 35: 1582–1600. https://doi.org/10.1111/j.1365-3040.2012.02511.x [Google Scholar] [PubMed] [CrossRef]
Liu J, Zhu JK (1998). A calcium sensor homolog required for plant salt tolerance. Science 280: 1943–1945. https://doi.org/10.1126/science.280.5371.1943 [Google Scholar] [PubMed] [CrossRef]
Livak KJ, Schmittgen TD (2001). Analysis of relative gene expression data using real-time quantitative PCR and the 2−ΔΔCT) method. Methods 25: 402–408. https://doi.org/10.1006/meth.2001.1262 [Google Scholar] [PubMed] [CrossRef]
Luo Q, Feng J, Yang G, He G (2022). Functional characterization of BdCIPK31 in plant response to potassium deficiency stress. Plant Physiology and Biochemistry 192: 243–251. https://doi.org/10.1016/j.plaphy.2022.10.014 [Google Scholar] [PubMed] [CrossRef]
Luo N, Liu J, Yu X, Jiang Y (2011). Natural variation of drought response in Brachypodium distachyon. Physiologia Plantarum 141: 19–29. https://doi.org/10.1111/j.1399-3054.2010.01413.x [Google Scholar] [PubMed] [CrossRef]
Luo Q, Wei Q, Wang R, Zhang Y, Zhang F, He Y, Yang G, He G (2018). Ectopic expression of BdCIPK31 confers enhanced low-temperature tolerance in transgenic tobacco plants. Acta Biochimica et Biophysica Sinica 50: 199–208. https://doi.org/10.1093/abbs/gmx140 [Google Scholar] [PubMed] [CrossRef]
Luo Q, Wei Q, Wang R, Zhang Y, Zhang F, He Y, Zhou S, Feng J, Yang G, He G (2017). BdCIPK31, a calcineurin B-like protein-interacting protein kinase, regulates plant response to drought and salt stress. Frontiers in Plant Science 8: 1184. https://doi.org/10.3389/fpls.2017.01184 [Google Scholar] [PubMed] [CrossRef]
Lyzenga WJ, Liu HX, Schofield A, Muise-Hennessey A, Stone SL (2013). Arabidopsis CIPK26 interacts with KEG, components of the ABA signalling network and is degraded by the ubiquitin-proteasome system. Journal of Experimental Botany 64: 2779–2791. https://doi.org/10.1093/jxb/ert123 [Google Scholar] [PubMed] [CrossRef]
Lyzenga WJ, Sullivan V, Liu HX, Stone SL (2017). The kinase activity of calcineurin B-like interacting protein kinase 26 (CIPK26) influences its own stability and that of the ABA-regulated ubiquitin ligase, keep on going (KEG). Frontiers in Plant Science 8: 502. https://doi.org/10.3389/fpls.2017.00502 [Google Scholar] [PubMed] [CrossRef]
Ma X, Gai W, Li Y, Yu Y, Ali M, Gong Z (2022). The CBL-interacting protein kinase CaCIPK13 positively regulates defence mechanisms against cold stress in pepper. Journal of Experimental Botany 73: 1655–1667. https://doi.org/10.1093/jxb/erab505 [Google Scholar] [PubMed] [CrossRef]
Ma X, Li Y, Gai W, Li C, Gong Z (2021b). The CaCIPK3 gene positively regulates drought tolerance in pepper. Horticulture Research 8: 216. https://doi.org/10.1038/s41438-021-00651-7 [Google Scholar] [PubMed] [CrossRef]
Ma R, Liu W, Li S, Zhu X, Yang J, Zhang N, Si H (2021a). Genome-wide identification, characterization and expression analysis of the CIPK gene family in potato (Solanum tuberosum L.) and the role of StCIPK10 in response to drought and osmotic stress. International Journal of Molecular Sciences 22: 13535. https://doi.org/10.3390/ijms222413535 [Google Scholar] [PubMed] [CrossRef]
Ma QJ, Sun MH, Lu J, Kang H, You CX, Hao YJ (2019). An apple sucrose transporter MdSUT2.2 is a phosphorylation target for protein kinase MdCIPK22 in response to drought. Plant Biotechnology Journal 17: 625–637. https://doi.org/10.1111/pbi.13003 [Google Scholar] [PubMed] [CrossRef]
Mao J, Mo Z, Yuan G, Xiang H, Visser R, Bai Y, Liu H, Wang Q, van der Linden C (2022). The CBL-CIPK network is involved in the physiological crosstalk between plant growth and stress adaptation. Plant Cell & Environment 44: 3589. https://doi.org/10.1111/pce.14396 [Google Scholar] [PubMed] [CrossRef]
Meng D, Dong B, Niu L, Song Z, Wang L, Amin R, Cao H, Li H, Yang Q, Fu Y (2021). The pigeon pea CcCIPK14-CcCBL1 pair positively modulates drought tolerance by enhancing flavonoid biosynthesis. Plant Journal 106: 1278–1297. https://doi.org/10.1111/tpj.15234 [Google Scholar] [PubMed] [CrossRef]
Mittler R, Blumwald E (2010). Genetic engineering for modern agriculture: Challenges and perspectives. Annual Review of Plant Biology 61: 443–462. https://doi.org/10.1146/annurev-arplant-042809-112116 [Google Scholar] [PubMed] [CrossRef]
Qiu QS, Guo Y, Dietrich MA, Schumaker KS, Zhu JK (2002). Regulation of SOS1, a plasma membrane Na+/H+ exchanger in Arabidopsis thaliana, by SOS2 and SOS3. Proceedings of the National Academy of Sciences of the United States of America 99: 8436–8441. https://doi.org/10.1073/pnas.122224699 [Google Scholar] [PubMed] [CrossRef]
Raghavendra AS, Gonugunta VK, Christmann A, Grill E (2010). ABA perception and signalling. Trends Plant Science 15: 395–401. https://doi.org/10.1016/j.tplants.2010.04.006 [Google Scholar] [PubMed] [CrossRef]
Rodrigo-Moreno A, Poschenrieder C, Shabala S (2013). Transition metals: A double edge sward in ROS generation and signaling. Plant Signaling & Behavior 8: e23425. https://doi.org/10.4161/psb.23425 [Google Scholar] [PubMed] [CrossRef]
Sharp RE, Poroyko V, Hejlek LG, Spollen WG, Springer GK, Bohnert HJ, Nguyen HT (2004). Root growth maintenance during water deficits: Physiology to functional genomics. Journal of Experimental Botany 55: 2343–2351. https://doi.org/10.1093/jxb/erh276 [Google Scholar] [PubMed] [CrossRef]
Simpkins G (2021). Drought-related warming. Nature Climate Change 11: 719. https://doi.org/10.1038/s41558-021-01147-x [Google Scholar] [CrossRef]
Sparkes IA, Runions J, Kearns A, Hawes C (2006). Rapid, transient expression of fluorescent fusion proteins in tobacco plants and generation of stably transformed plants. Nature Protocols 1: 2019–2025. https://doi.org/10.1038/nprot.2006.286 [Google Scholar] [PubMed] [CrossRef]
Steinhorst L, Mähs A, Ischebeck T, Zhang C, Zhang X, Arendt S, Schültke S, Heilmann I, Kudla J (2015). Vacuolar CBL-CIPK12 Ca2+-sensor-kinase complexes are required for polarized pollen tube growth. Current Biology 25: 1475–1482. https://doi.org/10.1016/j.cub.2015.03.053 [Google Scholar] [PubMed] [CrossRef]
Sun T, Wang Y, Wang M, Li T, Zhou Y, Wang X, Wei S, He G, Yang G (2015). Identification and comprehensive analyses of the CBL and CIPK gene families in wheat (Triticum aestivum L.). BMC Plant Biology 15: 269. https://doi.org/10.1186/s12870-015-0657-4 [Google Scholar] [PubMed] [CrossRef]
Tang R, Wang C, Li K, Luan S (2020). The CBL-CIPK calcium signaling network: Unified paradigm from 20 years of discoveries. Trends in Plant Science 25: 604–617. https://doi.org/10.1016/j.tplants.2020.01.009 [Google Scholar] [PubMed] [CrossRef]
Thole JM, Beisner ER, Liu J, Venkova SV, Strader LC (2014). Abscisic acid regulates root elongation through the activities of auxin and ethylene in Arabidopsis thaliana. G3: Genes, Genomes, Genetics 4: 1259–1274. https://doi.org/10.1534/g3.114.011080 [Google Scholar] [PubMed] [CrossRef]
Vogel JP, Garvin DF, Mockler TC, Schmutz J, Rokhsar D et al. (2010). Genome sequencing and analysis of the model grass Brachypodium distachyon. Nature 463: 763–768. https://doi.org/10.1038/nature08747 [Google Scholar] [PubMed] [CrossRef]
Wang L, Hu W, Sun J, Liang X, Yang X et al. (2015). Genome-wide analysis of SnRK gene family in Brachypodium distachyon and functional characterization of BdSnRK2.9. Plant Science 237: 33–45. https://doi.org/10.1016/j.plantsci.2015.05.008 [Google Scholar] [PubMed] [CrossRef]
Xiang Y, Huang Y, Xiong L (2007). Characterization of stress-responsive CIPK genes in rice for stress tolerance improvement. Plant Physiology 144: 1416–1428. https://doi.org/10.1104/pp.107.101295 [Google Scholar] [PubMed] [CrossRef]
Xu M, Li H, Liu ZN, Wang XH, Xu P, Dai SJ, Cao X, Cui XY (2021). The soybean CBL-interacting protein kinase, GmCIPK2, positively regulates drought tolerance and ABA signaling. Plant Physiology and Biochemistry 167: 980–989. https://doi.org/10.1016/j.plaphy.2021.09.026 [Google Scholar] [PubMed] [CrossRef]
Yamaguchi-Shinozaki K, Shinozaki K (2006). Transcriptional regulatory networks in cellular responses and tolerance to dehydration and cold stresses. Annual Review of Plant Biology 57: 781–803. https://doi.org/10.1146/annurev.arplant.57.032905.105444 [Google Scholar] [PubMed] [CrossRef]
Yan J, Niu F, Liu WZ, Zhang H, Wang B, Lan W, Che Y, Yang B, Luan S, Jiang YQ (2014). Arabidopsis CIPK14 positively regulates glucose response. Biochemical and Biophysical Research Communications 450: 1679–1683. https://doi.org/10.1016/j.bbrc.2014.07.064 [Google Scholar] [PubMed] [CrossRef]
Yoshida T, Mogami J, Yamaguchi-Shinozaki K (2014). ABA-dependent and ABA-independent signaling in response to osmotic stress in plants. Current Opinion in Plant Biology 21: 133–139. https://doi.org/10.1016/j.pbi.2014.07.009 [Google Scholar] [PubMed] [CrossRef]
You J, Chan ZL (2015). ROS regulation during abiotic stress responses in crop plants. Frontiers in Plant Science 6: 1092. https://doi.org/10.3389/fpls.2015.01092 [Google Scholar] [PubMed] [CrossRef]
Yu Q, An L, Li W (2014). The CBL-CIPK network mediates different signaling pathways in plants. Plant Cell Reports 33: 203–214. https://doi.org/10.1007/s00299-013-1507-1 [Google Scholar] [PubMed] [CrossRef]
Yuan F, Yang H, Xue Y, Kong D, Ye R et al. (2014). OSCA1 mediates osmotic-stress-evoked Ca2+ increases vital for osmosensing in Arabidopsis. Nature 514: 367–371. https://doi.org/10.1038/nature13593 [Google Scholar] [PubMed] [CrossRef]
Zhang XX, Koster P, Schlucking K, Balcerowicz D, Hashimoto K, Kuchitsu K, Kudla J (2018a). CBL1-CIPK26-mediated phosphorylation enhances activity of the NADPH oxidase RBOHC, but is dispensable for root hair growth. FEBS Letters 592: 2582–2593. https://doi.org/10.1002/1873-3468.13187 [Google Scholar] [PubMed] [CrossRef]
Zhang Y, Lv Y, Jahan N, Chen G, Ren D, Guo L (2018b). Sensing of abiotic stress and ionic stress responses in plants. International Journal of Molecular Sciences 19: 3298. https://doi.org/10.3390/ijms19113298 [Google Scholar] [PubMed] [CrossRef]
Zhang Y, Zhou Y, Zhu W, Liu J, Cheng F (2022). Non-coding RNAs fine-tune the balance between plant growth and abiotic stress tolerance. Frontiers in Plant Science 13: 965745. https://doi.org/10.3389/fpls.2022.965745 [Google Scholar] [PubMed] [CrossRef]
Zhu JK (2016). Abiotic stress signaling and responses in plants. Cell 167: 313–324. https://doi.org/10.1016/j.cell.2016.08.029 [Google Scholar] [PubMed] [CrossRef]
Supplementary Materials
Figure S1: The coding sequence of BdCIPK26 gene (LOC100830445).
Figure S2: Generation of transgenic tobacco plants overexpressing BdCIPK26. (A) PCR screening of T0 transgenic tobacco plants. + indicates the positive control, WT indicates the wildtype control using genome DNA extracted from WT tobaccos as PCR template. NTC indicates non-template control using ddH2O as PCR template. (B) The expression of BdCIPK26 in different transgenic tobacco lines. The bars exhibit the mean ± SE of three biological replicates (n = 3). The asterisks indicate significant difference compares to the control (*p < 0.05, **p < 0.01).
Figure S3: Expression of ROS-related genes. Expression of (A) NtCAT1, (B) NtPOX2, (C) NtSOD1, (D) NtGST, (E) NtAPX, and (F) NtDFR in transgenic tobacco plants under drought or salt treatment. The bars exhibit the mean ± SE of three biological replicates (n = 3). The asterisks indicate significant difference compares to the control (*p < 0.05, **p < 0.01).
Figure S4: Expression of stress-related transcription factors. Expression of (A) NtABF2 and (B) NtDREB3 in transgenic tobacco plants under drought or salt treatment. The bars exhibit the mean ± SE of three biological replicates (n = 3). The asterisks indicate significant difference compares to the control (*p < 0.05, **p < 0.01).
Cite This Article
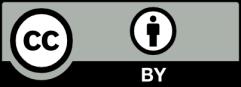
This work is licensed under a Creative Commons Attribution 4.0 International License , which permits unrestricted use, distribution, and reproduction in any medium, provided the original work is properly cited.