Open Access
ARTICLE
Immunoregulatory effects of human amniotic mesenchymal stem cells and their exosomes on human peripheral blood mononuclear cells
Department of Hematology and Oncology, Children’s Medical Center, The First Affiliated Hospital of Hunan Normal University, Changsha, 410005, China
* Corresponding Author: XIANGLING HE. Email:
BIOCELL 2023, 47(5), 1085-1093. https://doi.org/10.32604/biocell.2023.027090
Received 13 October 2022; Accepted 16 January 2023; Issue published 10 April 2023
Abstract
Background: The immunomodulatory effects of mesenchymal stem cells (MSCs) and their exosomes have been receiving increasing attention. This study investigated the immunoregulatory effects of human amniotic mesenchymal stem cells (hAMSCs) and their exosomes on phytohemagglutinin (PHA)-induced peripheral blood mononuclear cells (PBMCs). Methods: The hAMSCs used in the experiment were identified by light microscopy and flow cytometry, and the differentiation ability of the cells was determined by Oil Red O and Alizarin Red staining. The expressions of transforming growth factor (TGF)-β, indoleamine 2,3-dioxygenase (IDO), cyclooxygenase-2 (COX-2), hepatocyte growth factor (HGF), and interleukin (IL)-6 were detected by quantitative real-time polymerase chain reaction and western blotting. PBMCs, hAMSCs, and their exosomes were collected for in vitro group culture. Then the immunoregulatory ability of hAMSCs and their exosomes were analyzed by flow cytometry and Enzyme-linked immunosorbent assay. Results: The hAMSCs and exosomes were successfully extracted from the human amniotic membrane. TGF-β, IDO, COX-2, HGF, and IL-6 were significantly expressed in hAMSCs. In vitro co-culture showed that hAMSCs promoted the proliferation of Th2 cells in PHA-induced PBMCs, while hAMSCs and exosomes inhibited the secretion of TNF-α in PHA-induced PBMCs, and promoted the secretion of IL-4 and IL-10, and hAMSCs had more significant effects than exosomes. Conclusions: hAMSCs or exosomes could exert immunoregulatory effects on PHA-induced PBMCs by affecting Th2 cell proliferation and cytokine secretion.Keywords
Mesenchymal stem cells (MSCs), a group of stem cells with self-renewal and multi-lineage differentiation potential, are isolated mainly from bone marrow, fat, umbilical cord, placenta, and other tissues (Hass et al., 2011; Elahi et al., 2016). MSCs spontaneously differentiate into osteoblasts, chondrocytes, adipocytes, and nerve cells to regulate the normal turnover and homeostasis of human mesenchymal tissue, which is a new tool for the clinical application of stem cell therapy (Pittenger et al., 1999; Abumaree et al., 2012). In recent years, the immunomodulatory effects of MSCs have attracted increasing attention. MSCs regulate the proliferation, activation, and effector function of immune cells (macrophages, T lymphocytes, dendritic cells, and natural killer cells), indicating their therapeutic potential in the treatment of autoimmune and inflammatory diseases (Harrell et al., 2019). For example, MSCs are widely used to treat a variety of immune-related diseases, including systemic lupus erythematosus (Kamen et al., 2022), Crohn’s disease (Garcia-Olmo et al., 2022), graft-vs.-host disease (Tang et al., 2018), novel coronavirus pneumonia (Karyana et al., 2022; Monsel et al., 2022). Subsequent studies on the mechanism of action of MSCs revealed that the therapeutic effect of MSCs was mainly related to paracrine function, and exosomes may be an important basic substance for this function (Baron and Storb, 2012; Kim et al., 2012).
Exosomes are small vesicles of 30–100 nm in diameter secreted by a variety of cells. The outer membrane is mainly composed of lipids and proteins, which are secreted extracellularly by membrane fusion (Raposo and Stoorvogel, 2013; Wang et al., 2017). Exosomes carry different molecules, such as nucleic acids (DNA, RNA, mRNA, and miRNA), pro-inflammatory and anti-inflammatory cytokines, enzymes, and various other proteins, that mediate intercellular communication (Forsberg et al., 2020). Exosomes act on target cells in an endocrine or paracrine manner and fuse or endocytosis with target cell membranes to transmit information to target cells and then perform biological functions (Zhao et al., 2018; Harrell et al., 2019). Studies have shown that exosomes derived from MSCs can exert biological functions similar to MSCs. Blazquez et al. (2014) found that exosomes derived from human adipose-derived MSCs inhibited the differentiation and activation of T cells and reduced the proliferation of T cells and the release of interferon (IFN)-γ in vitro, indicating that mesenchymal stem cell exosomes also had immunomodulatory effects (Blazquez et al., 2014).
In this study, MSCs were extracted from human amniotic tissue and identified. Then exosomes were isolated from human amniotic mesenchymal stem cells (hAMSCs), and their morphology and surface markers were identified. The immunoregulatory effects of hAMSCs and exosomes on human peripheral blood mononuclear cells (PBMCs) were further explored with the aim of laying an experimental foundation for the clinical application of hAMSCs and exosomes.
Culture and identification of human amniotic mesenchymal stem cells
The hAMSCs used in the experiment were purchased from iCell Bioscience Inc. (HUM-iCell-e012, Shanghai, China) and cultured with Roswell Park Memorial Institute-1640 medium (R8758, Sigma, St. Louis, USA) containing 10% fetal bovine serum (FBS) and 2 mM glutamine at 37°C at 5% CO2. The hAMSCs were centrifuged to prepare a single-cell suspension. Of the above single-cell suspension, 100 μL was added to the following antibodies: PE-CD73 (11-0739-42, eBioscience, Santiago, USA), fluorescein isothiocyanate (FITC)-CD90 (11-0909-42, eBioscience, Santiago, USA), PE-CD73 (11-0739-42, eBioscience, Santiago, USA), PE-CD73 (11-0739-42, eBioscience, Santiago, USA), PE-CD105 (MA1-19594, eBioscience, Santiago, USA), PE-CD19 (MA1-19595, eBioscience, Santiago, USA), FITC-CD45 (11-0459-42, eBioscience, Santiago, USA), PE-CD11b (11-0118-42, eBioscience, Santiago, USA), FITC-HLA-DR (11-9956-42, eBioscience, Santiago, USA). Then, they were incubated at room temperature for 30 min. Phosphate buffer saline (PBS, 200 μL; AWI0137A, Abiowell, Changsha, China) was added to the suspension and centrifuged again, and the cell pellet was collected. The expression of surface marker antigens was detected on a BD CantoII flow cytometer (A00-1-1102, Beckman Coulter, Brea, USA).
Induction of adipogenic and osteogenic differentiation of human amniotic mesenchymal stem cells
The hAMSCs were digested with trypsin digestion solution (C0201, Beyotime Biotechnology, Shanghai, China) and inoculated into 6-well plates. After the cells adhered to the wells, adipogenic induction supporting medium (RBXMD-90031, Cyagen Biosciences, Guangzhou, China) or osteogenic induction supporting medium (RAWMD-90021, Cyagen Biosciences, Guangzhou, China) were replaced to induce adipogenic and osteogenic differentiation, respectively. After about 4 weeks, the adipogenic differentiation was stained with Oil Red O, and the osteogenic differentiation was stained with Alizarin red. After staining, the cells were washed with PBS (AWI0137a, Abiowell, Changsha, China), and the adipogenic and osteogenic staining effects of hAMSCs were observed under a microscope.
The hAMSCs were digested with trypsin digestion solution (C0201, Beyotime Biotechnology, Shanghai, China) and cultured in 96-well plates at 1 × 104 cells/well. The cells were observed for adherence and treated according to each group. After reaching the predetermined time, the 10% CCK-8 (NU679, DOJINDO LABORATORIES, Tokyo, Japan) solution was replaced and incubated for 2 h. The corresponding absorbance value was measured at 450 nm with a microplate reader (MB-530, Heales, Shenzhen, China). Each group was duplicated and the assay was performed three times.
Quantitative real-time polymerase chain reaction (qRT-qPCR)
Trizol kit (15596026, Thermo, Waltham, USA) was used to extract total RNA from the hAMSCs. The mRNA was reverse-transcribed into cDNA using the HiFiScript kit (CW2569, Beijing CWBIO Biotech Co., Ltd., Beijing, China). The expression of transforming growth factor (TGF)-β, indoleamine 2,3-dioxygenase (IDO), cyclooxygenase (COX)-2, hepatocyte growth factor (HGF), and interleukin (IL)-6 were detected by a fluorescence quantitative PCR instrument (PIKOREAL96, Thermo, USA). The relative expression of genes was calculated by the 2-ΔΔCt method. The primer sequences were designed by Sangon Biotech (Table 1).
RIPA lysis solution (583794, Beijing Gentihold Co., Ltd., Beijing, China) was added to hAMSCs. The samples were lysed on ice for 10 min and centrifuged to obtain the cell supernatant. A small amount of supernatant was taken to measure the protein concentration in the sample by Bicinchoninic Acid (BCA) method. Referring to the protein quantification results, the sample solution was boiled, denatured, and added to the gel wells. After the electrophoretic separation, the target protein was transferred to the nitrocellulose membrane, washed, and left overnight. The primary antibodies [TGF-β (21898-1-AP, 1:1000), IDO (13268-1-AP, 1:5000), COX-2 (66351-1-Ig, 1:2500), HGF (26881-1-AP, 1:1000), IL-6 (66146-1-Ig, 1:3000), CD63 (67605-1-Ig, 1: 5000), CD81 (66866-1-Ig, 1: 5000), β-actin (66009-1-Ig, 1: 5000), and glyceraldehyde 3-phosphate dehydrogenase (GAPDH, 10494-1-AP, 1:5000) were obtained from Proteintech (Chicago, USA) and added to the membrane and incubated overnight. Secondary antibodies: horseradish peroxidase goat anti-mouse IgG (SA00001-1, 1:5000) and HRP goat anti-rabbit IgG (SA00001-2, 1:6000) were from Proteintech (Chicago, USA); these were added to the membrane and incubated for 2 h. The GAPDH and β-actin acted as internal reference proteins. The membrane was colored using SuperECL Plus Luminescent Solution (Awestern0005, Abiowell, Changsha, China) to facilitate the analysis of protein imaging band intensity.
Identification of exosomes secreted by human amniotic mesenchymal stem cells
Exosomes extraction reagent (EXOQ5A-1, SBI, San Francisco, USA) was added to hAMSCs to collect the secreted exosomes. They were washed with distilled water, fixed with 2.5% glutaraldehyde (DF0156, Leagene, Beijing, China) for 2 min, and stained with 2% uranyl acetate. The stained exosomes were rinsed with distilled water again, dried, and placed under a Hitachi 7700 transmission electron microscope (TEM) (JEM1400, JEOL, Tokyo, Japan) structure. The Nanoparticle tracking assay (NTA) (ZetaView© ×30, Particle Metrix, Munich, Germany) was used to identify exosome diameter.
In vitro co-culturing of human amniotic mesenchymal stem cells and peripheral blood mononuclear cells
Human PBMCs purchased from Procell (CP-H-158, Wuhan, China) were seeded in a cell proliferation kit. The cells were cultured in four groups: (1). PBMCs group (PBMCs were cultured alone for 5 d), (2). PBMCs+PHA group (2.5 mg/L plant lectins were added to PBMCs and cultured for 5 d), (3). PBMCs+PHA+MSCs group (2.5 mg/L plant lectins were added to PBMCs and co-cultured with hAMSCs for 5 d), (4). PBMCs+PHA+Exos group (2.5 mg/L plant lectins were added to PBMCs and co-cultured with 30 μg/mL hAMSCs-Exos for 5 d).
Enzyme-linked immunosorbent assay (ELISA)
The supernatants of the four groups of cells co-cultured with hAMSCs and PBMCs were taken for examination. The levels of TNF-α, IL-4, and IL-10 in the cells of each group were measured using aTNF-α kit (KE00154, Proteintech, Chicago, USA), IL-4 kit (KE00016, Proteintech, Chicago, USA), and IL-10 kit (KE00170, Proteintech, Chicago, USA), respectively.
The hAMSCs were centrifuged after washing with PBS (AWI0137a, Abiowell, Changsha, China). The supernatant was discarded. Subsequently, FITC-labeled monoclonal antibodies [CD73 (11-0739-42), CD90 (11-0909-42), CD105 (MA1-19594), CD11b (11-0118-42), CD19 (MA1-19595), CD45 (11-0459-42), CD34 (11-0349-42, 11-0349-42), and HLA-DR (11-9956-42)] were all procured from eBioscience (Santiago, USA) and added for respective detection. The cells above were stained at room temperature for 30 min in the dark and washed with PBS again. Precipitates after centrifugation were collected for detection by flow cytometry (A00-1-1102, Beckman Coulter, Brea, USA).
PBMCs of each group were resuspended in 500 μL of RPMI-1640 containing 10% FBS, to which 1 μL Cell Stimulation cocktail (containing protein transport inhibitors) was added, stimulated, and cultured at 37°C for 4 h. After that, the cells were centrifuged at 350 g for 5 min, and the supernatant was discarded. One milliliter of 0.5% BSA-PBS was added to wash the cells, and centrifuged again. Permeabilization buffer (1 mL, 1×) was added to resuspend the pellet. After centrifuging, the pellet was resuspended in 100 μL of 1× permeabilization Buffer. The corresponding antibodies: CD4 (17-0049-42) and IFN-γ (12-7319-42) (both from eBioscience, Santiago, USA), were added and mixed well. The cells were washed with 1 mL of 0.5% BSA-PBS, and centrifuged for 5 min at room temperature and 400 g. The cells were resuspended in 150 μL of 0.5% BSA-PBS and tested by flow cytometry (A00-1-1102, Beckman Coulter, Brea, USA).
Statistical and analysis of data
GraphPad Prism 9.0 software was used to analyze and graph the resulting data. Univariate analysis was used to compare differences between multiple groups. p < 0.05 indicated a statistically significant difference.
Culture and identification of human amniotic mesenchymal stem cells
We found hAMSCs were mostly spindle-shaped and distributed radially (Fig. 1A). Besides, the surface markers CD73, CD90, and CD105 of the hAMSCs were noticeably expressed in the cells, with respective proliferation ratio of 95.61 ± 0.70%, 95.24 ± 0.59%, and 95.00 ± 0.37%. The antigens CD11b, CD19, CD34, CD45, and HLA-DR were not expressed in the cells (Fig. 1B). These findings indicated that the hAMSCs met the International Society for Cell Therapy minimum criteria for MSCs (Witwer et al., 2019), confirming that the cells used in our experiments were indeed hAMSCs.
Figure 1: Culture and identification of human amniotic mesenchymal stem cells (hAMSCs). (A) The morphology of hAMSCs was observed under a light microscope; ×100, scale bar = 100 μm; ×200, scale bar = 50 μm; ×400, scale bar = 25 μm. (B) Flow cytometry was applied to detect the expression of Hamsc surface molecules CD11b, CD19, CD34, CD45, CD73, CD90, CD105, and HLA-DR. These experiments were performed three times with similar results.
Differentiation and proliferation ability of human amniotic mesenchymal stem cells
The hAMSCs were observed after adipogenic and osteogenic differentiation. As shown in Fig. 2A, the cytoplasm of the cells was filled with many red lipid droplets of different sizes. Alizarin Red showed obvious red calcified nodules (Fig. 2B). The cells were cultured according to the time gradient, and the cell proliferation was observed to draw the hAMSCs growth curve. The initial OD value of hAMSCs was 0.8759 ± 0.0059. The cells grew rapidly in the first 3 days, and the OD values on days 1, 2, and 3 increased to 0.9268 ± 0.0024, 1.0209 ± 0.0073, and 1.2523 ± 0.0076, respectively. After that, the growth rate began to slow down, and the OD values on days 4, 5, 6, and 7 were 1.2840 ± 0.0098, 1.3085 ± 0.0038, 1.3358 ± 0.0010, and 1.3583 ± 0.0042, respectively (Fig. 2C). These results showed good differentiation and proliferation abilities of hAMSCs in this study.
Figure 2: Differentiation and proliferation capacity of human amniotic mesenchymal stem cells (hAMSCs). (A) Oil red O adipogenic staining observation, ×100, scale bar = 100 μm, (B) Alizarin red osteogenic staining observation, ×100, scale bar = 100 μm, (C) The growth curve of hAMSCs. n = 3. Error bars represent standard deviation.
Expression of immunoregulatory factors in human amniotic mesenchymal stem cells
We further detected the expression of immunomodulatory factors in extracted hAMSCs. The results of Qrt-PCR showed that the relative expression levels of TGF-β, IDO, COX-2, HGF, and IL-6 were 26.27 ± 0.10, 28.58 ± 0.18, 25.54 ± 0.05, 23.56 ± 0.15, and 28.79 ± 0.41, respectively (Fig. 3A). Western blotting showed that the relative expression levels of TGF-β, IDO, COX-2, HGF, and IL-6 were 59.22 ± 4.07, 51.58 ± 4.34, 64.58 ± 9.01, 91.13 ± 10.95, and 52.61 ± 3.02, respectively (Fig. 3B). These results showed that TGF-β, IDO, COX-2, HGF, and IL-6 were expressed significantly in cells, indicating the immunomodulatory effects of these hAMSCs.
Figure 3: Expression of immunoregulatory factors in human amniotic mesenchymal stem cells (hAMSCs). (A) The expression of TGF-β, IDO, COX-2, HGF, and IL-6 in hAMSCs was detected through a quantitative real-time polymerase chain reaction, (B) Western blotting was performed to detect TGF-β, IDO, COX-2, HGF, IL-6 expressions in hAMSCs. n = 3. Columns and error bars represent mean ± SD.
Identification of exosomes secreted by human amniotic mesenchymal stem cells
In the TEM view, exosomes secreted by hAMSCs were mostly 30–100 nm microbubbles with a slight depression in the middle and a clear membrane (Fig. 4A). The detection results of NTA showed that the peak diameter of the sample was 117.8 nm and the particles with diameters between 117.8 ± 62.3 nm accounted for 93.8% of total nanoparticles. This result was in line with the diameter of exosomes (Fig. 4B). Western blotting was used to measure the expression of exosome markers CD63 and CD81 (Fig. 4C). Thus, the above results indicated that hAMSCs indeed secreted exosomes.
Figure 4: Identification of exosomes secreted by human amniotic mesenchymal stem cells (hAMSCs). (A) Transmission electron microscopy was used to observe the structure of exosomes, (B) Nanoparticle tracking analysis was performed to identify the diameter of exosomes, (C) Western blot was utilized to analyze the expression of CD63 and CD81 in exosomes secreted by hAMSCs. These experiments were performed three times with similar results.
Immunoregulatory effects of human amniotic mesenchymal stem cells and exosomes on phytohemagglutinin-induced peripheral blood mononuclear cells
Among the four groups of cells co-cultured with hAMSCs and PBMCs in vitro, we found the highest proportion of Th2 cells in the PBMCs group. Compared with the PBMCs group, the proportion of Th2 cells decreased significantly in the PBMCs+PHA group. Notably, the proportion of Th2 cells in the PBMCs+PHA+MSCs group was higher than that in PBMCs+PHA group. However, the difference between the PBMCs+PHA+Exos group and the PBMCs+PHA group was not significant (Fig. 5A). From the results of ELISA (Fig. 5B), we found a significantly lowered protein concentration of IL-4 and IL-10 in cells in the PBMCs+PHA group than in the PBMCs group. Besides, the protein concentration of IL-4 and IL-10 was significantly higher in cells in the PBMCs+PHA+MSCs group and PBMCs+PHA+Exos group compared to those in the PBMCs+PHA group. However, the protein concentration of TNF-α in cells in the PBMCs+PHA group was significantly higher than that in the PBMCs group, and the protein concentration of TNF-α was significantly lower in cells in the PBMCs+PHA+MSCs group and PBMCs+PHA+Exos group than in the PBMCs+PHA group. These results indicated that hAMSCs or exosomes could exert immunomodulatory effects on PHA-induced PBMCs by affecting Th2 cell proliferation and cytokine secretion, and hAMSCs had more significant effects than exosomes.
Figure 5: Immunoregulatory effects of human amniotic mesenchymal stem cells (and exosomes on phytohemagglutinin (PHA)-induced peripheral blood mononuclear cells (PBMCs). (A) The proportion of Th2 cells in peripheral blood mononuclear cells was detected through flow cytometry, (B) Enzyme-linked immunosorbent assay was used to determine the secretion of interleukin (IL)-4, IL-10, and tumor necrosis factor-α in the co-culture supernatant. *p < 0.05 vs. PBMCs. #p < 0.05 vs. PBMCs+PHA. &p < 0.05 vs. PBMCs+PHA+MSCs. n = 3. Columns and error bars represent mean ± SD.
Immunomodulation has always been a hot topic of research on immune-related diseases. Finding cheap and effective immunomodulatory agents to improve the immune microenvironment in vivo is a common problem. MSCs and their exosomes have broad application prospects in the treatment of various immune diseases as immunomodulators due to their multiple advantages in source and function (Shi et al., 2018; Zhang et al., 2018; Huang et al., 2022). In this study, hAMSCs were successfully extracted from human amniotic membrane tissue, and exosomes were isolated from hAMSCs. Furthermore, the immunomodulatory effects of hAMSCs and exosomes on PHA-induced PBMCs were also confirmed.
MSCs are pluripotent stem cells derived from the mesoderm, which can self-renew and differentiate into a variety of mesenchymal cells in vitro, including osteoblasts, chondrocytes, adipocytes, and nerve cells. At present, MSCs are mainly isolated and prepared from bone marrow, fat, umbilical cord, placenta, and other tissues, most of which are derived from bone marrow (Pittenger et al., 1999; Hass et al., 2011; Abumaree et al., 2012; Elahi et al., 2016). However, the source of bone marrow mesenchymal stem cells and the ability to proliferate and differentiate in vitro is limited, with difficult access, thus limiting their clinical application (Lee et al., 2007; Choumerianou et al., 2010; Chou et al., 2013). Postpartum discarded placental tissue is an abundant source of hAMSCs, avoiding ethical limitations (Nazarov et al., 2012). The morphology, phenotype, three-directional differentiation potential, and amplification ability in vitro are the main criteria for the identification of MSCs (Horwitz et al., 2005). MSCs are morphologically heterogeneous, including cells from narrow fusiform to large polygonal and slightly cuboidal (Pittenger et al., 1999). Phenotypically, the surface of MSCs lacks specific surface molecules, thus, the MSCs are usually identified by combined markers. MSCs express CD29, CD90, CD73, CD44, CD105, and other surface molecules, while hematopoietic lineage markers such as CD34, CD45, HLA-DR, CD11a, and CD14 and endothelial lineage markers such as CD11b and CD31 are not expressed (Bernardo et al., 2009). Zhang et al. (2006) isolated hAMSCs from human placental villi by external implantation. The isolated hAMSCs were fibrous and expressed CD29, CD44, CD105, CD90, and CD73, while CD31, CD45, CD34, Tie-2, CD133, and HLA-DR were not expressed; besides, the hAMSCs had obvious cartilage differentiation potential. Our results are consistent with these findings, indicating that we could successfully isolate hAMSCs.
Exosomes are membrane vesicles with a diameter of 30–100 nm that are secreted extracellularly by membrane fusion (Raposo and Stoorvogel, 2013; Wang et al., 2017). Exosomes contain nucleic acids, proteins, and other active substances, which are transferred to target tissues to perform the functions of nucleic acids, proteins, and other active substances (Zhao et al., 2018, Harrell et al., 2019, Forsberg et al., 2020). Exosomes highly express CD63 and CD81, the tetraspanin superfamily members, and can interact with major histocompatibility complex, integrin, and other proteins (Yu et al., 2014). Chen et al. (2020) successfully isolated exosomes from hAMSCs. The obtained exosomes were round, oval, or saucer-shaped, with an average particle size of 103 nm, and were positive for the expression of surface markers CD63 and CD81. In this study, the material obtained by ultracentrifugation was identified by NTA and TEM and was identified to be microbubbles with a diameter of 117.8 ± 62.3 nm, with high expression of CD63 and CD81, indicating that we successfully isolated exosomes from hAMSCs.
In recent years, more attention has been paid to the immunomodulatory effect of MSCs. Further studies have found that the immunomodulatory effect of MSCs is mainly through the production of exosomes and soluble paracrine factors (Li and Hua, 2017). Important immunoregulatory factors include TGF-β, COX-2, IDO, HGF, IL-6, PGE2, etc. (Shi et al., 2018; Huang et al., 2022). MSCs can inhibit T lymphocytes, B lymphocytes, NK cells, macrophages, and proliferation, differentiation, and secretion of dendritic cells while promoting the proliferation of regulatory T cells and innate and adaptive immune processes (Harrell et al., 2019). MSCs have also been used clinically to treat a variety of immune-related diseases, including systemic lupus erythematosus (Kamen et al., 2022), Crohn’s disease (Garcia-Olmo et al., 2022), graft-versus-host disease (Tang et al., 2018), and novel coronavirus pneumonia (Karyana et al., 2022; Monsel et al., 2022). Our study shows that TGF-β, IDO, COX-2, HGF, and IL-6 are significantly expressed in hAMSCs. Stem cell exosomes have been suggested to have the potential to perform similar biological functions as stem cells (Kim et al., 2012; Lee et al., 2013; Zhang et al., 2018). Blazquez et al. (2014) found that exosomes derived from human adipose-derived MSCs inhibited the differentiation and activation of T cells and reduced T cell proliferation and the release of IFN-γ in vitro, indicating that mesenchymal stem cell exosomes also had immunomodulatory effects. Our study showed that hAMSCs promote Th2 cell proliferation in PHA-induced PBMCs; hAMSCs and exosomes inhibit the secretion of TNF-α and promote the secretion of IL-4 and IL-10 in PHA-induced PBMCs; hAMSCs had more significant effect than exosomes. These results thus indicate that hAMSCs or exosomes could exert immunomodulatory effects on PHA-induced PBMCs by affecting Th2 cell proliferation and cytokine secretion.
To conclude, hAMSCs were extracted from human amniotic membrane tissue and exosomes were isolated successfully from hAMSCs, and the immunomodulatory effects of hAMSCs and exosomes on PHA-induced PBMCs were further confirmed. However, before mesenchymal stem cells are widely used in clinical practice, obstacles and challenges need to be overcome. For example, MSCs are typically heterogeneous and vary widely in inflammatory or anti-inflammatory microenvironments, leading to different effects of MSCs-mediated immune regulation. In addition, the effects of acute or chronic inflammation on MSCs-mediated immune regulation also need to be further explored in the future.
Funding Statement: Research Program of Health Committee of Hunan Province (202106010870) to Xin Tian.
Author Contributions: Study conception and design: XIN TIAN; XIANGLING HE. Data collection: XIN TIAN; XIANGLING HE; SHUQIN QIAN; RUNYING ZOU; KEKE CHEN; CHENGGUANG ZHU; ZEXI YIN. Analysis and interpretation of results: XIN TIAN; XIANGLING HE; SHUQIN QIAN. Draft manuscript preparation: XIN TIAN; XIANGLING HE. All authors reviewed the results and approved the final version of the manuscript.
Availability of Data and Materials: All data generated or analyzed during this study are included in this published article (and its supplementary information files).
Ethics Approval: Not applicable.
Conflicts of Interest: The authors declare that they have no conflicts of interest to report regarding the present study.
References
Abumaree M, Al Jumah M, Pace RA, Kalionis B (2012). Immunosuppressive properties of mesenchymal stem cells. Stem Cell Reviews and Reports 8: 375–392. https://doi.org/10.1007/s12015-011-9312-0 [Google Scholar] [PubMed] [CrossRef]
Baron F, Storb R (2012). Mesenchymal stromal cells: A new tool against graft-versus-host disease? Biology of Blood and Marrow Transplantation 18: 822–840. https://doi.org/10.1016/j.bbmt.2011.09.003 [Google Scholar] [PubMed] [CrossRef]
Bernardo ME, Locatelli F, Fibbe W (2009). Mesenchymal stromal cells: A novel treatment modality for tissue repair. Annals of the New York Academy of Sciences 1176: 101–117. https://doi.org/10.1111/j.1749-6632.2009.04607.x [Google Scholar] [PubMed] [CrossRef]
Blazquez R, Sanchez-Margallo FM, de la Rosa O, Dalemans W, Alvarez V, Tarazona R, Casado JG (2014). Immunomodulatory potential of human adipose mesenchymal stem cells derived exosomes on in vitro stimulated T cells. Frontiers in Immunology 5: 556. https://doi.org/10.3389/fimmu.2014.00556 [Google Scholar] [PubMed] [CrossRef]
Chen T, Gao S, Hao Y, Zhang F, Tang X, Wei Z, Wang D, Qi J (2020). Experimental study of human amniotic mesenchymal stem cell exosome promoting fibroblasts migration through microRNA-135a. Zhongguo Xiu Fu Chong Jian Wai Ke Za Zhi 34: 234–239. https://doi.org/10.7507/1002-1892.201907136 [Google Scholar] [PubMed] [CrossRef]
Chou SH, Lin SZ, Day CH, Kuo WW, Shen CY, Hsieh DJ, Lin JY, Tsai FJ, Tsai CH, Huang CY (2013). Mesenchymal stem cell insights: Prospects in hematological transplantation. Cell Transplantation 22: 711–721. https://doi.org/10.3727/096368912X655172 [Google Scholar] [PubMed] [CrossRef]
Choumerianou DM, Martimianaki G, Stiakaki E, Kalmanti L, Kalmanti M, Dimitriou H (2010). Comparative study of stemness characteristics of mesenchymal cells from bone marrow of children and adults. Cytotherapy 12: 881–887. https://doi.org/10.3109/14653249.2010.501790 [Google Scholar] [PubMed] [CrossRef]
Elahi KC, Klein G, Avci-Adali M, Sievert KD, MacNeil S, Aicher WK (2016). Human mesenchymal stromal cells from different sources diverge in their expression of cell surface proteins and display distinct differentiation patterns. Stem Cells International 2016: 5646384. https://doi.org/10.1155/2016/5646384 [Google Scholar] [PubMed] [CrossRef]
Forsberg MH, Kink JA, Hematti P, Capitini CM (2020). Mesenchymal stromal cells and exosomes: Progress and challenges. Frontiers in Cell and Developmental Biology 8: 665. https://doi.org/10.3389/fcell.2020.00665 [Google Scholar] [PubMed] [CrossRef]
Garcia-Olmo D, Gilaberte I, Binek M, D.H. AJL, Lindner D, Selvaggi F, Spinelli A, Panés J (2022). Follow-up study to evaluate the long-term safety and efficacy of darvadstrocel (mesenchymal stem cell treatment) in patients with perianal fistulizing crohn’s disease: ADMIRE-CD phase 3 randomized controlled trial. Diseases of the Colon & Rectum 65: 713–720. https://doi.org/10.1097/dcr.0000000000002325 [Google Scholar] [PubMed] [CrossRef]
Harrell CR, Fellabaum C, Jovicic N, Djonov V, Arsenijevic N, Volarevic V (2019). Molecular mechanisms responsible for therapeutic potential of mesenchymal stem cell-derived secretome. Cells 8: 467. https://doi.org/10.3390/cells8050467 [Google Scholar] [PubMed] [CrossRef]
Hass R, Kasper C, Böhm S, Jacobs R (2011). Different populations and sources of human mesenchymal stem cells (MSCA comparison of adult and neonatal tissue-derived MSC. Cell Communication and Signaling 9: 12. https://doi.org/10.1186/1478-811X-9-12 [Google Scholar] [PubMed] [CrossRef]
Horwitz EM, Le Blanc K, Dominici M, Mueller I, Slaper-Cortenbach I, Marini FC, Deans RJ, Krause DS, Keating A (2005). Clarification of the nomenclature for MSC: The international society for cellular therapy position statement. Cytotherapy 7: 393–395. https://doi.org/10.1080/14653240500319234 [Google Scholar] [PubMed] [CrossRef]
Huang Y, Wu Q, Tam PKH (2022). Immunomodulatory mechanisms of mesenchymal stem cells and their potential clinical applications. International Journal of Molecular Sciences 23: 10023. https://doi.org/10.3390/ijms231710023 [Google Scholar] [PubMed] [CrossRef]
Kamen DL, Wallace C, Li Z, Wyatt M, Paulos C, Wei C, Wang H, Wolf BJ, Nietert PJ, Gilkeson G (2022). Safety, immunological effects and clinical response in a phase I trial of umbilical cord mesenchymal stromal cells in patients with treatment refractory SLE. Lupus Science & Medicine 9: e000704. https://doi.org/10.1136/lupus-2022-000704 [Google Scholar] [PubMed] [CrossRef]
Karyana M, Djaharuddin I, Rif'ati L, Arif M, Choi MK et al. (2022). Safety of DW-MSC infusion in patients with low clinical risk COVID-19 infection: A randomized, double-blind, placebo-controlled trial. Stem Cell Research & Therapy 13: 134. https://doi.org/10.1186/s13287-022-02812-4 [Google Scholar] [PubMed] [CrossRef]
Kim HS, Choi DY, Yun SJ, Choi SM, Kang JW, Jung JW, Hwang D, Kim KP, Kim DW (2012). Proteomic analysis of microvesicles derived from human mesenchymal stem cells. Journal of Proteome Research 11: 839–849. https://doi.org/10.1021/pr200682z [Google Scholar] [PubMed] [CrossRef]
Lee SY, Miwa M, Sakai Y, Kuroda R, Matsumoto T, Iwakura T, Fujioka H, Doita M, Kurosaka M (2007). In vitro multipotentiality and characterization of human unfractured traumatic hemarthrosis-derived progenitor cells: A potential cell source for tissue repair. Journal of Cellular Physiology 210: 561–566. https://doi.org/10.1002/(ISSN)1097-4652 [Google Scholar] [CrossRef]
Lee JK, Park SR, Jung BK, Jeon YK, Lee YS, Kim MK, Kim YG, Jang JY, Kim CW (2013). Exosomes derived from mesenchymal stem cells suppress angiogenesis by down-regulating VEGF expression in breast cancer cells. PLoS One 8: e84256. https://doi.org/10.1371/journal.pone.0084256 [Google Scholar] [PubMed] [CrossRef]
Li N, Hua J (2017). Interactions between mesenchymal stem cells and the immune system. Cellular and Molecular Life Sciences 74: 2345–2360. https://doi.org/10.1007/s00018-017-2473-5 [Google Scholar] [PubMed] [CrossRef]
Monsel A, Hauw-Berlemont C, Mebarki M, Heming N, Mayaux J et al. (2022). Treatment of COVID-19-associated ARDS with mesenchymal stromal cells: A multicenter randomized double-blind trial. Critical Care 26: 48. https://doi.org/10.1186/s13054-022-03930-4 [Google Scholar] [PubMed] [CrossRef]
Nazarov I, Lee JW, Soupene E, Etemad S, Knapik D et al. (2012). Multipotent stromal stem cells from human placenta demonstrate high therapeutic potential. Stem Cells Translational Medicine 1: 359–372. https://doi.org/10.5966/sctm.2011-0021 [Google Scholar] [PubMed] [CrossRef]
Pittenger MF, Mackay AM, Beck SC, Jaiswal RK, Douglas R, Mosca JD, Moorman MA, Simonetti DW, Craig S, Marshak DR (1999). Multilineage potential of adult human mesenchymal stem cells. Science 284: 143–147. https://doi.org/10.1126/science.284.5411.143 [Google Scholar] [PubMed] [CrossRef]
Raposo G, Stoorvogel W (2013). Extracellular vesicles: Exosomes, microvesicles, and friends. Journal of Cell Biology 200: 373–383. https://doi.org/10.1083/jcb.201211138 [Google Scholar] [PubMed] [CrossRef]
Shi Y, Wang Y, Li Q, Liu K, Hou J, Shao C, Wang Y (2018). Immunoregulatory mechanisms of mesenchymal stem and stromal cells in inflammatory diseases. Nature Reviews Nephrology 14: 493–507. https://doi.org/10.1038/s41581-018-0023-5 [Google Scholar] [PubMed] [CrossRef]
Tang B, Li X, Liu Y, Chen X, Li X et al. (2018). The therapeutic effect of ICAM-1-overexpressing mesenchymal stem cells on acute graft-versus-host disease. Cellular Physiology and Biochemistry 46: 2624–2635. https://doi.org/10.1159/000489689 [Google Scholar] [PubMed] [CrossRef]
Wang J, Yeung BZ, Cui M, Peer CJ, Lu Z, Figg WD, Guillaume Wientjes M, Woo S, Au JL (2017). Exosome is a mechanism of intercellular drug transfer: Application of quantitative pharmacology. Journal of Controlled Release 268: 147–158. https://doi.org/10.1016/j.jconrel.2017.10.020 [Google Scholar] [PubMed] [CrossRef]
Witwer KW, Van Balkom BWM, Bruno S, Choo A, Dominici M et al. (2019). Defining mesenchymal stromal cell (MSC)-derived small extracellular vesicles for therapeutic applications. Journal of Extracellular Vesicles 8: 1609206. https://doi.org/10.1080/20013078.2019.1609206 [Google Scholar] [PubMed] [CrossRef]
Yu B, Zhang X, Li X (2014). Exosomes derived from mesenchymal stem cells. International Journal of Molecular Sciences 15: 4142–4157. https://doi.org/10.3390/ijms15034142 [Google Scholar] [PubMed] [CrossRef]
Zhang Q, Fu L, Liang Y, Guo Z, Wang L, Ma C, Wang H (2018). Exosomes originating from MSCs stimulated with TGF-β and IFN-γ promote Treg differentiation. Journal of Cellular Physiology 233: 6832–6840. https://doi.org/10.1002/jcp.26436 [Google Scholar] [PubMed] [CrossRef]
Zhao H, Shang Q, Pan Z, Bai Y, Li Z, Zhang H, Zhang Q, Guo C, Zhang L, Wang Q (2018). Exosomes from adipose-derived stem cells attenuate adipose inflammation and beiging in white adipose tissues. Diabetes 67: 235–247. https://doi.org/10.2337/db17-0356 [Google Scholar] [PubMed] [CrossRef]
Zhang X, Mitsuru A, Igura K, Takahashi K, Ichinose S, Yamaguchi S, Takahashi TA (2006). Mesenchymal progenitor cells derived from chorionic villi of human placenta for cartilage tissue engineering. Biochemical and Biophysical Research Communications 340: 944–952. https://doi.org/10.1016/j.bbrc.2005.12.091 [Google Scholar] [PubMed] [CrossRef]
Cite This Article
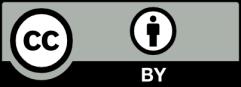
This work is licensed under a Creative Commons Attribution 4.0 International License , which permits unrestricted use, distribution, and reproduction in any medium, provided the original work is properly cited.