Open Access
ARTICLE
Dihydroartemisinin enhances cell apoptosis in diffuse large B cell lymphoma by inhibiting the STAT3 activity
1 Qingdao University, Qingdao, 266000, China
2 Department of Hematology, The Affiliated Hospital, Qingdao University, Qingdao, 266000, China
* Corresponding Author: SHAOLING WU. Email:
# These authors contributed to the work equally and should be regarded as co-first authors
BIOCELL 2023, 47(5), 1075-1083. https://doi.org/10.32604/biocell.2023.027027
Received 10 October 2022; Accepted 16 January 2023; Issue published 10 April 2023
Abstract
Background: Dihydroartemisinin (DHA) is reported to be a potential anticancer agent, and the mechanisms underlying the effects of DHA on diffuse large B cell lymphoma however are still obscure. This study aimed to assess the antitumor effect of DHA on diffuse large B cell lymphoma cells and to determine the potential underlying mechanisms of DHA-induced cell apoptosis. Methods: Here, the Cell Counting Kit 8 assay was conducted to study cell proliferation. We performed Annexin V-FITC/propidium iodide staining, real-time polymerase chain reaction, and western blot analysis to analyze cell apoptosis and potential molecular mechanisms. Results: The results showed that DHA substantially suppressed cell proliferation and induced cell apoptosis in vitro in a time- and concentration-dependent fashion. Moreover, STAT3 activity could be inhibited after stimulation with DHA. Conclusion: These results imply that the underlying anti-tumoral effect of DHA may increase apoptosis in diffuse large B cell lymphoma cells via the STAT3 signaling pathway. In addition, DHA might be an effective drug for diffuse large B cell lymphoma therapy.Keywords
Diffuse large B cell lymphoma (DLBCL) is the most common form of non-Hodgkin lymphoma, with an extensive global influence. Progression-free survival (PFS) at 10 years was 36.5% when patients were treated with the conventional regimen of rituximab, cyclophosphamide, doxorubicin, vincristine, and prednisone (R-CHOP). Unfortunately, after an initial response to first-line treatment, one-third of patients relapse or experience refractory disease and require subsequent treatments, which remains a leading cause of morbidity and mortality (Coiffier et al., 2010). Hence, it is urgent to find new effective antitumor drugs. Herbs known and used today in traditional Chinese medicine present a large source of active substances for developing new chemotherapeutics against cancer. Therefore, it is particularly important to find effective traditional Chinese medicine monomers for the treatment of DLBCL.
Signal transducers and activators of transcription 3 (STAT3), as a transcription factor, can enter the nucleus and bind to DNA fragments of the target gene promoter following activation, to regulate the expression of associated genes, resulting in biological effects (Sanchez-Lopez et al., 2016). STAT3 is known to be activated in numerous primary tumors, including lymphoma, and abnormally activated STAT3 is considered to be an indicator of poor prognosis in DLBCL (Gu et al., 2016; Huang et al., 2013; Xu et al., 2018). STAT3 with ectopic activation can prevent apoptosis and promote proliferation by controlling B-cell lymphoma (Bcl)-2, Bcl-xL, myeloid cell leukemia-1 (Mcl-1), and cellular-myelocytomatosis viral oncogene (c-Myc) expression associated with survival and proliferation to promote tumorigenesis (Li et al., 2015). Previous studies have shown that dihydroartemisinin (DHA), as a putative STAT3 inhibitor, could inhibit head and neck squamous cell carcinoma by blocking STAT3 activation, and thus downregulates a series of its downstream genes including cyclin D1, Bcl-xL, Bcl-1, E-cadherin, matrix metalloproteinase-2 (MMP2), MMP9, etc., of which proteins are associated with regulation of cell cycle, apoptosis, and epithelial-mesenchymal transition (Yang et al., 2015). In DLBCL, MYC is the third most frequently translocated oncogene (in 5%–17% of cases (Aukema et al., 2014)). Typically, c-Myc is associated with other gene abnormalities, including Bcl-2 translocations or overexpression, leading to extremely poor prognosis after standard treatment (Green et al., 2012; Horn et al., 2013; Johnson et al., 2012; Valera et al., 2013).
Traditional Chinese monomer compounds for the treatment of malignant tumors have become a research hot point because of their multiple targets and low toxicity. DHA, one of the most effective artemisinin derivatives, plays a crucial role in antiviral and antibacterial activities in addition to its antimalarial activity (Cheong et al., 2020), without manifesting any toxicity in normal cells (Liu et al., 2018). Recently, the antitumor effect of DHA has been a research focus. At present, a large number of studies have shown that DHA has inhibitory effects on lung cancer, prostate cancer, and epithelial ovarian cancer (Paccez et al., 2019; Yuan et al., 2020). Our previous research also supports this view (Wei et al., 2018). The research on the mechanism is mainly concentrated on DHA-induced autophagy, cell cycle blocking, and apoptosis, which in turn leads to restricted tumor cell proliferation. Apoptosis is a physiological cell suicide process to maintain homeostasis in the tissue and eliminate gene changes and unstable cells; the process is greatly weakened in cancer. There are mainly endogenous and exogenous apoptotic pathways in various cells. The endogenous apoptotic pathway, also known as the mitochondrial pathway, is an important way for chemotherapeutic drugs to induce apoptosis. Beyond its prominent pro-apoptotic role, DHA affects cancer cell functions, including angiogenesis (Wang et al., 2011), and immune regulation (Zhao et al., 2012). However, only limited research has examined the potentiality of DHA for the treatment of DLBCL. This study focuses on the effect of DHA on the proliferation of diffuse large B-cell lymphoma and the potential molecular mechanism, hoping to provide reference and inspiration to DLBCL patients with poor response to traditional treatment.
Antibodies against STAT3, phospho-STAT3 (Tyr705), and c-Myc were purchased from Cell Signaling Technology (CST, Danvers, MA, USA), and Bcl-2, Bax, caspase 9, caspase 3, and cleaved-poly(ADP-ribose) polymerase (PARP) were from Abcam (Cambridge, MA, USA). Anti-β-actin antibody was purchased from Elabscience (Wuhan, China). DHA was obtained from MedChemExpress (Monmouth Junction, NJ, USA) and dissolved in dimethyl sulfoxide (Sigma Aldrich, St.Louis, USA) as a stock solution of 8,000 µM at −20°C. Roswell Park Memorial Institute (RPMI)-1640 medium and fetal bovine serum were bought from Biological Industries (Kibbutz Beit Haemek, Israel).
Human DLBCL cell line SU-DHL-4 was obtained from Deutsche Sammlung von Mikroorganismen und Zellkulturen. SU-DHL-4 cells were cultured in RPMI 1640 medium supplemented with 10% fetal bovine serum and 1% antibiotics. Cells were incubated at 37°C in a humidified 5% CO2 atmosphere.
Cell viability was determined by Cell Counting Kit-8 (CCK8, MedChemExpress, Monmouth Junction, NJ, USA) according to the manufacturer’s instructions. SU-DHL-4 cells were seeded at a density of 5 × 104 cells/well in a 96-well plate (BIOFIL, JET, Guangzhou, China). Cells were incubated for 24, 48, and 72 h with DHA (0, 2.5, 5, 10, 20, 40, or 80 µM) added to the culture medium. At each time point, 10 µL CCK-8 was added to each well and the cells were subsequently incubated at 37°C for 2 h. The absorbance value of each well was measured at 450 nm on a microplate absorbance reader in triplicate (Sunrise; Tecan Group, Ltd., Männedorf, Switzerland). The percent inhibition was calculated utilizing the equation:
Apoptosis induction in SU-DHL-4 cells by DHA treatment was measured by the Annexin V-FITC Apoptosis Detection kit (BD Bioscience, San Jose, CA, USA). The cells after incubation for 24 h in 6-well plates at a density of 1 × 106 cells per well were treated with various concentrations (0, 10, 20, and 40 μM) of DHA for 24 h at 37°C. Following incubation, the cells were washed two times with ice-cold phosphate-buffered saline (PBS) and subsequently re-suspended in 200 µL binding buffer. The cells were treated with 5 µL Annexin V-fluorescein isothiocyanate (BD Biosciences) for 15 min in the dark at room temperature, and then 5 of µL od propidium iodide (PI; BD Biosciences) was added and incubated for another 5 min in the same environment, and another 200 µL binding buffer was added. A flow cytometer (BD Biosciences, Franklin Lakes, NJ, USA) was used to determine fluorescent intensity three times. All data were collected and analyzed by the FlowJo software (FlowJo LLC, Ashland, Oregon, USA).
Quantitative fluorescence real-time polymerase chain reaction (RT-qPCR)
Total RNA was extracted from the cultured cells using TRIzol™ Reagent (Takara, Otsu, Shiga, Japan). Cells were harvested and washed twice with ice-cold PBS. Ultramicro spectrophotometer (Molecular Devices, Shanghai, China) was used to determine the concentration and purity of total RNA and was reversely transcribed into single-stranded cDNA using a Prime Script™ RT reagent kit (Vazyme, China), according to the manufacturer’s protocols. Next, the reverse transcribed single-stranded cDNA was amplified by SYBR1 Premix Ex Taq II (Vazyme, China) on a Roche LightCycler 480II System (Roche, Basel, Switzerland). The primers were synthesized by Sangon Biotech Co., Ltd. (Shanghai, Beijing, China; Table 1). Thermocycling conditions included a pre-incubation stage at 95°C for 30 s, 40 cycles of amplification stage at 95°C for 5 s and 60°C for 30 s, and a melt curve stage at 95°C for 15 s, 65°C for 60 s, and 95°C for 15 s. Relative expression of GAPDH mRNA was used as an endogenous internal control. Gene expression changes were assessed with the 2−ΔΔCq method. The primer sequences used are listed in Table 1.
RIPA lysis buffer (Elabscience, Wuhan, China) containing protease inhibitors (1%) and phosphatase inhibitors (1%) was utilized to lyse the cells for 30 min, and then total protein was harvested by centrifugation (12,000 rpm) at 4°C for 10 min. The extracted protein concentration of each lysate was determined and quantified with a BCA Protein Assay Kit (Elabscience, Wuhan, China), prior to being boiled at 100°C for 8 min. Equal quantities (40 µg) of proteins were separated by 10% or 12% sodium dodecyl sulfate-polyacrylamide gels and electrotransferred onto polyvinylidene difluoride membranes (Solarbio, Beijing, China), and blocked in 5% skimmed milk (for nonphosphorylated proteins) or 5% bovine serum albumin (for phosphorylated proteins) for 2 h at room temperature. Each membrane was incubated with primary antibodies overnight at 4°C, washed three times with tris-buffered saline with Tween 20 (TBST) for 15 min each time, and incubated with goat anti-rabbit secondary antibody for 1 h at room temperature. Subsequently, the membranes were washed three times with TBST for 15 min each time, and the immunoblots were measured with a Fusion FX7 system (Vilber Lourmat, Marne-la-Vallée, France) using Immobilon Western Chemilum HRP Substrate (EMD Millipore, Billerica, MA, USA). Relative expression of β-actin served as an endogenous internal control for protein loading.
Data from at least triplicate experiments were expressed by mean ± SEM. All statistical analyses between groups were performed by GraphPad Prism 8.0 (San Diego, CA, USA). One-way ANOVA was used to compare the statistical significance of between-group differences. Data significance is expressed as significant (*p < 0.05), very significant (**p < 0.01), and highly significant (****p < 0.0001).
Dihydroartemisinin inhibits the proliferation of SU-DHL-4
To detect the anti-proliferative effects of DHA in vitro, SU-DHL-4 cells were intervened with DHA of 0, 2.5, 5, 10, 20, 40, and 80 µM for 24, 48, and 72 h, and then, cell proliferation and cytotoxicity assay were evaluated by CCK-8 assay kit assessing cell viability. We found that a higher concentration of DHA had a more obvious inhibitory effect on SU-DHL-4, and the rate of inhibition increased over time. The concentration that inhibits 50% (IC50) of DHA after 24 h of treatment was 33.14 μM. As shown in Fig. 1A, the proliferation rate reached 70.87% ± 5.18% after cells were treated with DHA for 24 h, while it was 44.25% ± 8.58% after 48 h of treatment. Thus, DHA significantly reduced the viability of SU-DHL-4 cells in a dose- and time-dependent manner compared with the control group (Fig. 1B). Cell viability had statistical significance in different time points/concentrations (all p < 0.0001).
Figure 1: Dihydroartemisinin (DHA) inhibits the proliferation of SU-DHL-4 cells in vitro. (A) Cells were treated with different concentrations of DHA for 24, 48, and 72 h. Cell viability was assessed using CCK8 assay. (B) Cell viability of SU-DHL-4 cells treated with 0, 2.5, 5, 10, 20, 40, or 80 µM DHA after 24, 48, and 72 h. Data are expressed as the mean ± SEM of three independent experiments. ****p < 0.0001, compared with the control group.
Dihydroartemisinin induces apoptosis in SU-DHL-4 cells
To further investigate the mechanism of DHA-mediated antitumor activity, SU-DHL-4 cells were treated with 10, 20, and 40 µM DHA for 24 h, and then apoptosis was examined by Annexin V/PI double-staining assay. Flow cytometric analyses demonstrated that, compared with the control group, which exhibited an apoptosis rate of 23.01% ± 2.98%, the apoptosis rate of the DHA treatment groups with 10, 20, and 40 µM DHA respectively, reached 52.36% ± 4.54%, 60.03% ± 0.67%, and 74.3% ± 2.6% (Figs. 2A and 2B). The differences were statistically significant. The above data suggest that DHA has the effect of inducing apoptosis in SU-DHL-4 cells in a concentration-dependent manner.
Figure 2: Dihydroartemisinin induces apoptosis in SU-DHL-4 cells. (A) Measurement of apoptosis in SU-DHL-4 cells by Annexin V-FITC Apoptosis Detection Kit I and flow cytometry following treatment with different doses of DHA for 24 h. Q3 represents early apoptosis. (B) Data from A are presented as the mean ± SD. ****p < 0.0001, compared to controls.
Dihydroartemisinin inhibits signal transducers and activators of transcription 3 activity
Next, we sought to explore the mechanisms of DHA-induced apoptosis in DLBCL cells. Studies have shown that STAT3, as a transcription factor, regulates genes concerning cell proliferation and apoptosis. SU-DHL-4 cells were treated with 10, 20, and 40 µM DHA for 6 h, and the protein expression level changes of STAT3 and p-STAT3 (Try705) were detected by western blot. SU-DHL-4 cells were treated with these concentrations of DHA for 24 h, and the relative expression levels of c-Myc mRNA and protein, and Bcl-2, Bax protein were detected by RT-qPCR and western blot. It was revealed that p-STAT3 (Tyr505) protein was expressed optimally in SU-DHL-4 cells in the absence of DHA treatment. P-STAT3 (Try705) protein expression was downregulated in SU-DHL-4 cells treated with 20 and 40 µM DHA in comparison with mock-treated cells and the differences were statistically significant (Figs. 3A and 3B). While all concentrations of DHA had no significant effect on the total relative expression of STAT3 protein. The above findings illustrate that STAT3 is constitutively activated in DLBCL cells, and STAT3 activation can be suppressed in SU-DHL-4 cells by higher concentrations of DHA, which may block the STAT3 signaling pathway. Interestingly, c-Myc mRNA and protein in treatment groups were both downregulated, compared with controls, and the differences were statistically significant (Figs. 3C–3E). However, we were pleasantly surprised to observe that DHA did not change Bcl-2 and Bax expression at the protein level (Fig. 4), indicating that these molecules may not be the key to combination-induced apoptosis. The data presented above indicate that the inhibition of STAT3 activity may be the main mechanism by which DHA exerts anti-tumor activity. DHA may block the STAT3 phosphorylation, which plays an antitumor activity mainly by down-regulating c-Myc expression but not Bcl-2 or Bax, as well as c-Myc-dependent apoptosis, which indicates that the mode of antitumor action of DHA may vary in different types of cancers.
Figure 3: Dihydroartemisinin (DHA) inhibits STAT3 activity. (A) Protein expression of STAT3, p-STAT3 in SU-DHL-4 cells following treatment with DHA for 6 h measured by western blot; β-actin served as an internal control. (B) Bar graphs representing protein expression of p-STAT3. (C) Protein expression of c-Myc, Bcl-2, and Bax in SU-DHL-4 cells following treatment with DHA for 24 h measured by western blot; β-actin served as an internal control. (D) Bar graphs representing protein expression of c-Myc, Bcl-2, and Bax. (E) Quantitative real-time polymerase chain reaction analysis of c-Myc mRNA expression in SU-DHL-4 cells following treatment with DHA (0–40 µM) for 24 h and results were normalized to GAPDH to correct for loading. **p < 0.01 and ****p < 0.0001, vs. the control group. p‑, phosphorylated; STAT3, signal transducer and activator of transcription 3; c-Myc, cellular-myelocytomatosis viral oncogene; Bcl-2, B-cell lymphoma 2; Bax, Bcl‑2‑associated X protein.
Figure 4: The intrinsic apoptosis pathway is involved in dihydroartemisinin (DHA)-induced apoptosis of SU-DHL-4 cells. Apoptosis-related proteins after treatment with various concentrations of DHA for 24 h were subjected to western blot analysis.
The intrinsic apoptosis pathway is involved in the dihydroartemisinin-induced apoptosis of SU-DHL-4 cell.
As shown in Fig. 4, SU-DHL-4 cells in experimental groups were treated with 10, 20, and 40 µM DHA for 24 h, and the expression of caspase 9, the essential initiator caspase via the mitochondrial pathway, was significantly decreased in DHA-treated groups compared with controls. In addition, DHA could downregulate the expression of caspase 3 and upregulate the expression of cleaved-caspase 3 and cleaved-PARP, which was statistically significant. Of interest, caspase 9 and caspase 3 proteins demonstrated greater suppression, while cleaved-caspase 3 and cleaved-PARP were gradually increased in response to increasing drug concentrations. These results indicated that the endogenous apoptosis pathway was involved in the DHA-induced apoptosis of SU-DHL-4 in a dose-dependent manner.
Diffuse large B cell lymphoma is the most common non-Hodgkin lymphoma, and its occurrence is associated with alterations in critical genes and activation of pivotal signaling pathways. About 40% of patients with diffuse large B-cell lymphoma do not respond well to conventional chemotherapy, including the majority of patients with double-expressed lymphoma. Therefore, additional effective and well-tolerated drugs are needed. DHA is known to be an effective drug in treating patients with malaria (Mohamed et al., 2017). Recent studies have revealed that DHA has antitumor activity with few side effects in various types of tumors in vivo and in vitro (Du et al., 2015; Greenshields et al., 2017; Wang et al., 2015; Zhang et al., 2012). In this work, we confirmed the effect of DHA inhibition on DLBCL development in vitro. Furthermore, all our data indicate that DHA can significantly induce apoptosis of DLBCL at 20 μM and higher concentrations. Therefore, we used 10, 20, and 40 μM DHA for follow-up studies.
It has been established that DHA is involved in the treatment of breast cancer, liver cancer, lung cancer, and hematologic neoplasms, by regulating cancer cell apoptosis and inhibiting invasion (Bai et al., 2019; Du et al., 2019; Gao et al., 2020). Also, DHA can induce inhibition of tumor cell proliferation, through the induction of cell ferroptosis (Du et al., 2015; Greenshields et al., 2017; Wang et al., 2015; Zhang et al., 2012) and autophagy (Tao et al., 2021). Chen et al. (2021) found that artesunate (ART) inhibits the proliferation of DLBCL cells by inhibiting the STAT3 signaling pathway to induce apoptosis and cell cycle arrest. STAT3 pathway plays a significant role in regulating the proliferation and apoptosis of cancer cells. Recent studies have revealed that STAT3 is constitutionally activated in hematological tumors and can promote tumor progression by regulating a variety of genes. Moreover, clinical data indicated that aberrant STAT3 activation is closely associated with poor prognosis, recurrence, and drug resistance in patients with hematologic tumors (Brachet-Botineau et al., 2020). The STAT3 signaling pathway is activated upon phosphorylation, which in turn regulates downstream genes linked to cell proliferation and apoptosis. Much attention has been paid to c-Myc among many downstream target genes regulated by STAT3.
The c-Myc protein, a short-lived nuclear phosphoprotein, is a multifunctional transcription factor that serves a vital role in regulating cell growth, differentiation, and apoptosis, as well as a major oncogene when expressed inappropriately (Jia et al., 2016). Notably, overexpression of c-Myc has been associated with the occurrence of hematopoietic malignancies (Zhou et al., 2014). Evidently, c-Myc has a complicated story in aggressive B-cell lymphomas and other hematologic tumors as well as many solid tumors (Dang, 2012; Ott et al., 2013). Therefore, we suggest that c-Myc is a molecular hallmark of cancer. Indeed, c-Myc activation alone is generally not sufficient to exert this oncogenic effect. MYC is limited by several physiological mechanisms that lead to cell cycle arrest and apoptosis. For instance, uncontrolled MYC activation induces the expression of tumor protein 53 (TP53) (Fang et al., 2019), Bcl-2 (Teitz et al., 2000), and cyclin-dependent kinase inhibitor 2A (CDKN2A) (Vaux et al., 1988). Accordingly, activation of Bcl-2, and inactivation of TP53 or CDKN2A work in coordination with MYC signaling to block cell cycle arrest and apoptosis, which drive cancer. Additionally, c-Myc, as the downstream target gene of STAT3, also has multiple functions in hematological tumors. DHA has previously been shown to inhibit STAT3 signal transduction and McL-1 and survivin expression to enhance apoptosis in NSCLC (Yan et al., 2018). In another study, the anti-tumor activity of diosmetin on human osteosarcoma cells was illustrated by inhibiting the activity of STAT3/c-MYC signaling (Ning et al., 2021).
Apoptosis, the programmed death of cells is of great importance for the removal of unwanted cells from the body. Our previous study found that DHA induced apoptosis in Jurkat T lymphoma cells through a Bak-dependent intrinsic pathway (Handrick et al., 2010). Findings from another study also indicated that DHA induced apoptosis of colorectal cancer cells through a mitochondrial-dependent pathway (Lu et al., 2014). In the present investigation, we provided more profound insights into the regulation of DHA on apoptosis. Consistent with previous studies, here, we reported that the proapoptotic effect of DHA needed to activate caspase 9 and caspase 3. However, the mechanism of DHA-mediated inhibition of the growth of diffuse large B cell lymphoma has not been studied. Based on the above-mentioned finding, this study explored the therapeutic effect of DHA in diffuse large B cell lymphoma and its potential molecular mechanism. Our research group also found that DHA could inhibit T-cell lymphoma cell survival and proliferation by inhibiting protein kinase B/Glycogen synthase kinase β signaling pathway, decreasing c-Myc protein expression, and upregulating Bax/Bcl-2 ratio, and we also found that DHA combined with siNotch1 could inhibit T-cell lymphoma cell proliferation, promote apoptosis, and downregulate Notch1/c Myc signaling pathway (Huo et al., 2018). In this work, we investigated the potential of DHA as a novel antitumor agent for the treatment of DLBCL. DHA inhibited the proliferation of the DLBCL cell line SU-DHL-4 in a concentration- and time-dependent manner, consistent with the findings of DHA on Jurkat and ovarian cancer cells (Liu et al., 2018; Wei et al., 2018). Then we further explored the underlying mechanism. Our results demonstrated that DHA exerted anti-lymphoma effects by enhancing apoptosis in diffuse large B-cell lymphoma through down-regulation of c-Myc expression by inhibiting STAT3 activity. To begin with, DHA treatment reduced p-STAT3 protein level, and C-Myc mRNA and protein levels, which showed that DHA inhibited STAT3 activation, and then regulated the expression of the downstream molecule c-Myc, which is consistent with previous reports that suppression of STAT3 activation after DHA treatment in non-small cell lung cancer cells (Yan et al., 2018). Next, DHA could induce the apoptosis of SU-DHL-4 cells in a drug-dependent manner. DHA-induced apoptosis in SU-DHL-4 cells in a drug dose-dependent manner. Interestingly, we also found that caspase 9, caspase 3, and cleaved-PARP were down-regulated after DHA intervention, illustrating that the endogenous apoptosis pathway was also involved in DHA-induced apoptosis. However, DHA could not downregulate Bcl-2 or upregulate the expression of Bax protein in the present study, which we speculated to occur for the following possible reasons. In this research, we chose the SU-DHL4 cell line, a germinal center B-cell (GCB) subtype of DLBCL cells, as an in vitro model of DLBCL. According to previous studies, the overexpression of Bcl-2 in the GCB subtype of DLBCL is mainly due to the rearrangement of the Bcl-2 gene (Riedell and Smith, 2018), so we considered that its regulation by STAT3 is weak, and the suppression of STAT3 activity cannot downregulate Bcl-2 protein expression. On the other hand, previous studies reported that Bcl-2 mainly prevents apoptosis from occurring by inhibiting Bax (Barclay et al., 2015), and we speculate that if Bcl-2 protein expression cannot be downregulated, it will be difficult to upregulate Bax protein expression. Therefore, we extrapolate that the key factor in apoptosis of SU-DHL-4 cells is the downregulation or inhibition of c-Myc instead of Bcl-2 or Bax.
In summary, the results of this study showed that DHA significantly inhibited the growth of human DLBCL SU-DHL-4 cells in vitro, which may be attributed to enhanced cell apoptosis through the decreased level of phosphorylated STAT3 (Tyr705). The results of the present study aid in clarifying the mechanism of antitumor activity of DHA in diffuse large B cell lymphoma cells. Therefore, we provided further solid experimental evidence to support DHA as a potential therapeutic agent for human DLBCL. However, the effect of DHA on the patient samples and xenograft mouse model in DLBCL was not assessed in the present study; therefore, we will further investigate the effect of DHA in future in vivo studies.
Funding Statement: This present work was supported by the Shandong Provincial Natural Science Foundation of China (ZR2019MH096).
Author Contributions: The authors confirm their contributions to the paper as follows: CZ and ZCX contributed equally to this work. CZ conceived and designed the experiments. ZCX wrote the manuscript and analyzed all data. ZXD and WSL guided the work. WZM, WCY, ZW, and CSL revised the manuscript critically. All authors reviewed the results and approved the final version of the manuscript.
Availability of Data and Materials: All data generated or analyzed during this study are included in this article.
Ethics Approval: Not applicable.
Conflicts of Interest: The authors declare that they have no conflicts of interest to report regarding the present study.
References
Aukema SM, Kreuz M, Kohler CW, Rosolowski M, Hasenclever D, et al. (2014). Biological characterization of adult MYC-translocation-positive mature B-cell lymphomas other than molecular Burkitt lymphoma. Haematologica 99: 726–735. https://doi.org/10.3324/haematol.2013.091827 [Google Scholar] [PubMed] [CrossRef]
Bai X, Shao J, Zhou S, Zhao Z, Li F, et al. (2019). Inhibition of lung cancer growth and metastasis by DHA and its metabolite, RvD1, through miR-138-5p/FOXC1 pathway. Journal of Experimental and Clinical Cancer Research 38: 479. https://doi.org/10.1186/s13046-019-1478-3 [Google Scholar] [PubMed] [CrossRef]
Barclay LA, Wales TE, Garner TP, Wachter F, Lee S et al. (2015). Inhibition of pro-apoptotic BAX by a noncanonical interaction mechanism. Molecular Cell 57: 873–886. https://doi.org/10.1016/j.molcel.2015.01.014 [Google Scholar] [PubMed] [CrossRef]
Brachet-Botineau M, Polomski M, Neubauer HA, Juen L, Hédou D et al. (2020). Pharmacological inhibition of oncogenic STAT3 and STAT5 signaling in hematopoietic cancers. Cancers 12: 240. https://doi.org/10.3390/cancers12010240 [Google Scholar] [PubMed] [CrossRef]
Chen Y, Wang F, Wu P, Gong S, Gao J et al. (2021). Artesunate induces apoptosis, autophagy and ferroptosis in diffuse large B cell lymphoma cells by impairing STAT3 signaling. Cellular Signalling 88: 110167. https://doi.org/10.1016/j.cellsig.2021.110167 [Google Scholar] [PubMed] [CrossRef]
Cheong DHJ, Tan DWS, Wong FWS, Tran T (2020). Anti-malarial drug, artemisinin and its derivatives for the treatment of respiratory diseases. Pharmacological Research 158: 104901. https://doi.org/10.1016/j.phrs.2020.104901 [Google Scholar] [PubMed] [CrossRef]
Coiffier B, Thieblemont C, Van Den Neste E, Lepeu G, Plantier I et al. (2010). Long-term outcome of patients in the LNH-98.5 trial, the first randomized study comparing rituximab-CHOP to standard CHOP chemotherapy in DLBCL patients: A study by the Groupe d’Etudes des Lymphomes de l’Adulte. Blood 116: 2040–2045. https://doi.org/10.1182/blood-2010-03-276246 [Google Scholar] [PubMed] [CrossRef]
Dang CV (2012). MYC on the path to cancer. Cell 149: 22–35. https://doi.org/10.1016/j.cell.2012.03.003 [Google Scholar] [PubMed] [CrossRef]
Du W, Pang C, Xue Y, Zhang Q, Wei X (2015). Dihydroartemisinin inhibits the Raf/ERK/MEK and PI3K/AKT pathways in glioma cells. Oncology Letters 10: 3266–3270. https://doi.org/10.3892/ol.2015.3699 [Google Scholar] [PubMed] [CrossRef]
Du J, Wang T, Li Y, Zhou Y, Wang X et al. (2019). DHA inhibits proliferation and induces ferroptosis of leukemia cells through autophagy dependent degradation of ferritin. Free Radical Biology and Medicine 131: 356–369. https://doi.org/10.1016/j.freeradbiomed.2018.12.011 [Google Scholar] [PubMed] [CrossRef]
Fang Y, Shen ZY, Zhan YZ, Feng XC, Chen KL et al. (2019). CD36 inhibits β-catenin/c-myc-mediated glycolysis through ubiquitination of GPC4 to repress colorectal tumorigenesis. Nature Communications 10: 3981. https://doi.org/10.1038/s41467-019-11662-3 [Google Scholar] [PubMed] [CrossRef]
Gao J, Ma F, Wang X, Li G (2020). Combination of dihydroartemisinin and resveratrol effectively inhibits cancer cell migration via regulation of the DLC1/TCTP/Cdc42 pathway. Food & Function 11: 9573–9584. https://doi.org/10.1039/D0FO00996B [Google Scholar] [PubMed] [CrossRef]
Green TM, Young KH, Visco C, Xu-Monette ZY, Orazi A et al. (2012). Immunohistochemical double-hit score is a strong predictor of outcome in patients with diffuse large B-cell lymphoma treated with rituximab plus cyclophosphamide, doxorubicin, vincristine, and prednisone. Journal of Clinical Oncology 30: 3460–3467. https://doi.org/10.1200/JCO.2011.41.4342 [Google Scholar] [PubMed] [CrossRef]
Greenshields AL, Shepherd TG, Hoskin DW (2017). Contribution of reactive oxygen species to ovarian cancer cell growth arrest and killing by the anti-malarial drug artesunate. Molecular Carcinogenesis 56: 75–93. https://doi.org/10.1002/mc.22474 [Google Scholar] [PubMed] [CrossRef]
Gu H, Feng J, Wang H, Qian Y, Yang L et al. (2016). Celastrus orbiculatus extract inhibits the migration and invasion of human glioblastoma cells in vitro. BMC Complementary and Alternative Medicine 16: 387. https://doi.org/10.1186/s12906-016-1232-8 [Google Scholar] [PubMed] [CrossRef]
Handrick R, Ontikatze T, Bauer KD, Freier F, Rübel A et al. (2010). Dihydroartemisinin induces apoptosis by a Bak-dependent intrinsic pathway. Molecular Cancer Therapeutics 9: 2497–2510. https://doi.org/10.1158/1535-7163.MCT-10-0051 [Google Scholar] [PubMed] [CrossRef]
Horn H, Ziepert M, Becher C, Barth TF, Bernd HW et al. (2013). MYC status in concert with BCL2 and BCL6 expression predicts outcome in diffuse large B-cell lymphoma. Blood 121: 2253–2263. https://doi.org/10.1182/blood-2012-06-435842 [Google Scholar] [PubMed] [CrossRef]
Huang X, Meng B, Iqbal J, Ding BB, Perry AM et al. (2013). Activation of the STAT3 signaling pathway is associated with poor survival in diffuse large B-cell lymphoma treated with R-CHOP. Journal of Clinical Oncology 31: 4520–4528. https://doi.org/10.1200/JCO.2012.45.6004 [Google Scholar] [PubMed] [CrossRef]
Huo L, Wei W, Wu S, Zhao X, Zhao C, et al. (2018). Effect of dihydroarteminin combined with siRNA targeting Notch1 on Notch1/c-Myc signaling in T-cell lymphoma cells. Experimental and Therapeutic Medicine 15: 3059–3065. https://doi.org/10.3892/etm.2018.5784 [Google Scholar] [PubMed] [CrossRef]
Jia L, Song Q, Zhou C, Li X, Pi L et al. (2016). Dihydroartemisinin as a putative STAT3 inhibitor, suppresses the growth of head and neck squamous cell carcinoma by targeting Jak2/STAT3 signaling. PLoS One 11: e0147157. https://doi.org/10.1371/journal.pone.0147157 [Google Scholar] [PubMed] [CrossRef]
Johnson NA, Slack GW, Savage KJ, Connors JM, Ben-Neriah S et al. (2012). Concurrent expression of MYC and BCL2 in diffuse large B-cell lymphoma treated with rituximab plus cyclophosphamide, doxorubicin, vincristine, and prednisone. Journal of Clinical Oncology 30: 3452–3459. https://doi.org/10.1200/JCO.2011.41.0985 [Google Scholar] [PubMed] [CrossRef]
Li Y, Rogoff HA, Keates S, Gao Y, Murikipudi S et al. (2015). Suppression of cancer relapse and metastasis by inhibiting cancer stemness. Proceedings of the National Academy of Sciences of the United States of America 112: 1839–1844. https://doi.org/10.1073/pnas.1424171112 [Google Scholar] [PubMed] [CrossRef]
Liu Y, Gao S, Zhu J, Zheng Y, Zhang H et al. (2018). Dihydroartemisinin induces apoptosis and inhibits proliferation, migration, and invasion in epithelial ovarian cancer via inhibition of the hedgehog signaling pathway. Cancer Medicine 7: 5704–5715. https://doi.org/10.1002/cam4.1827 [Google Scholar] [PubMed] [CrossRef]
Lu M, Sun L, Zhou J, Yang J (2014). Dihydroartemisinin induces apoptosis in colorectal cancer cells through the mitochondria-dependent pathway. Tumour Biology 35: 5307–5314. https://doi.org/10.1007/s13277-014-1691-9 [Google Scholar] [PubMed] [CrossRef]
Mohamed AO, Abdel Hamid MM, Mohamed OS, Elkando NS, Suliman A et al. (2017). Efficacies of DHA-PPQ and AS/SP in patients with uncomplicated Plasmodium falciparum malaria in an area of an unstable seasonal transmission in Sudan. Malaria Journal 16: 163. https://doi.org/10.1186/s12936-017-1817-9 [Google Scholar] [PubMed] [CrossRef]
Ning R, Chen G, Fang R, Zhang Y, Zhao W et al. (2021). Diosmetin inhibits cell proliferation and promotes apoptosis through STAT3/c-Myc signaling pathway in human osteosarcoma cells. Biological Research 54: 40. https://doi.org/10.1186/s40659-021-00363-1 [Google Scholar] [PubMed] [CrossRef]
Ott G, Rosenwald A, Campo E (2013). Understanding MYC-driven aggressive B-cell lymphomas: Pathogenesis and classification. Hematology 2013, the American Society of Hematology Education Program Book 2013: 575–583. https://doi.org/10.1182/asheducation-2013.1.575 [Google Scholar] [PubMed] [CrossRef]
Paccez JD, Duncan K, Sekar D, Correa RG, Wang Y et al. (2019). Dihydroartemisinin inhibits prostate cancer via JARID2/miR-7/miR-34a-dependent downregulation of Axl. Oncogenesis 8: 14. https://doi.org/10.1038/s41389-019-0122-6 [Google Scholar] [PubMed] [CrossRef]
Riedell PA, Smith SM (2018). Double hit and double expressors in lymphoma: Definition and treatment. Cancer 124: 4622–4632. https://doi.org/10.1002/cncr.31646 [Google Scholar] [PubMed] [CrossRef]
Sanchez-Lopez E, Flashner-Abramson E, Shalapour S, Zhong Z, Taniguchi K et al. (2016). Targeting colorectal cancer via its microenvironment by inhibiting IGF-1 receptor-insulin receptor substrate and STAT3 signaling. Oncogene 35: 2634–2644. https://doi.org/10.1038/onc.2015.326 [Google Scholar] [PubMed] [CrossRef]
Tao J, Diao L, Chen F, Shen A, Wang S et al. (2021). pH-sensitive nanoparticles codelivering docetaxel and dihydroartemisinin effectively treat breast cancer by enhancing reactive oxidative species-mediated mitochondrial apoptosis. Molecular Pharmaceutics 18: 74–86. https://doi.org/10.1021/acs.molpharmaceut.0c00432 [Google Scholar] [PubMed] [CrossRef]
Teitz T, Wei T, Valentine MB, Vanin EF, Grenet J et al. (2000). Caspase 8 is deleted or silenced preferentially in childhood neuroblastomas with amplification of MYCN. Nature Medicine 6: 529–535. https://doi.org/10.1038/75007 [Google Scholar] [PubMed] [CrossRef]
Valera A, López-Guillermo A, Cardesa-Salzmann T, Climent F, González-Barca E et al. (2013). MYC protein expression and genetic alterations have prognostic impact in patients with diffuse large B-cell lymphoma treated with immunochemotherapy. Haematologica 98: 1554–1562. https://doi.org/10.3324/haematol.2013.086173 [Google Scholar] [PubMed] [CrossRef]
Vaux DL, Cory S, Adams JM (1988). Bcl-2 gene promotes haemopoietic cell survival and cooperates with c-myc to immortalize pre-B cells. Nature 335: 440–442. https://doi.org/10.1038/335440a0 [Google Scholar] [PubMed] [CrossRef]
Wang SJ, Sun B, Cheng ZX, Zhou HX, Gao Y et al. (2011). Dihydroartemisinin inhibits angiogenesis in pancreatic cancer by targeting the NF-κB pathway. Cancer Chemotherapy and Pharmacology 68: 1421–1430. https://doi.org/10.1007/s00280-011-1643-7 [Google Scholar] [PubMed] [CrossRef]
Wang Q, Wu S, Zhao X, Zhao C, Zhao H et al. (2015). Mechanisms of dihydroartemisinin and dihydroartemisinin/holotransferrin Cytotoxicity in T-cell lymphoma cells. PLoS One 10: e0137331. https://doi.org/10.1371/journal.pone.0137331 [Google Scholar] [PubMed] [CrossRef]
Wei W, Zhao X, Wu S, Zhao C, Zhao H et al. (2018). Dihydroartemisinin triggers c-Myc proteolysis and inhibits protein kinase B/glycogen synthase kinase 3β pathway in T-cell lymphoma cells. Oncology Letters 16: 6838–6846. https://doi.org/10.3892/ol.2018.9450 [Google Scholar] [PubMed] [CrossRef]
Xu F, Cui WQ, Wei Y, Cui J, Qiu J et al. (2018). Astragaloside IV inhibits lung cancer progression and metastasis by modulating macrophage polarization through AMPK signaling. Journal of Experimental and Clinical Cancer Research 37: 207. https://doi.org/10.1186/s13046-018-0878-0 [Google Scholar] [PubMed] [CrossRef]
Yan X, Li P, Zhan Y, Qi M, Liu J et al. (2018). Dihydroartemisinin suppresses STAT3 signaling and Mcl-1 and Survivin expression to potentiate ABT-263-induced apoptosis in Non-small Cell Lung Cancer cells harboring EGFR or RAS mutation. Biochemical Pharmacology 150: 72–85. https://doi.org/10.1016/j.bcp.2018.01.031 [Google Scholar] [PubMed] [CrossRef]
Yang H, Yamazaki T, Pietrocola F, Zhou H, Zitvogel L et al. (2015). STAT3 inhibition enhances the therapeutic efficacy of immunogenic chemotherapy by stimulating type 1 interferon production by cancer cells. Cancer Research 75: 3812–3822. https://doi.org/10.1158/0008-5472.CAN-15-1122 [Google Scholar] [PubMed] [CrossRef]
Yuan B, Liao F, Shi ZZ, Ren Y, Deng XL et al. (2020). Dihydroartemisinin inhibits the proliferation, colony formation and induces ferroptosis of lung cancer cells by inhibiting PRIM2/SLC7A11 axis. OncoTargets and Therapy 13: 10829–10840. https://doi.org/10.2147/ott.S248492 [Google Scholar] [PubMed] [CrossRef]
Zhang CZ, Zhang H, Yun J, Chen GG, Lai PB (2012). Dihydroartemisinin exhibits antitumor activity toward hepatocellular carcinoma in vitro and in vivo. Biochemical Pharmacology 83: 1278–1289. https://doi.org/10.1016/j.bcp.2012.02.002 [Google Scholar] [PubMed] [CrossRef]
Zhao YG, Wang Y, Guo Z, Gu AD, Dan HC et al. (2012). Dihydroartemisinin ameliorates inflammatory disease by its reciprocal effects on Th and regulatory T cell function via modulating the mammalian target of rapamycin pathway. Journal of Immunology 189: 4417–4425. https://doi.org/10.4049/jimmunol.1200919 [Google Scholar] [PubMed] [CrossRef]
Zhou K, Xu D, Cao Y, Wang J, Yang Y et al. (2014). C-MYC aberrations as prognostic factors in diffuse large B-cell lymphoma: A meta-analysis of epidemiological studies. PLoS One 9: e95020. https://doi.org/10.1371/journal.pone.0095020 [Google Scholar] [PubMed] [CrossRef]
Cite This Article
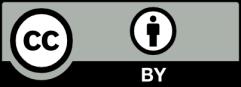
This work is licensed under a Creative Commons Attribution 4.0 International License , which permits unrestricted use, distribution, and reproduction in any medium, provided the original work is properly cited.