Open Access
ARTICLE
LINC00609 inhibits A549 cells progression through the regulation of miR-128-3p/RND3 axis
1 Department of Thoracic Surgery, Renmin Hospital of Wuhan University, Wuhan, 430060, China
2 Department of Respiratory and Critical Care Medicine, Renmin Hospital of Wuhan University, Wuhan, 430060, China
* Corresponding Author: HUIQING LIN. Email:
# Co-first authors: Xiangchao Ding, Yang Zhao
BIOCELL 2023, 47(5), 1117-1126. https://doi.org/10.32604/biocell.2023.026715
Received 21 September 2022; Accepted 26 December 2022; Issue published 10 April 2023
Abstract
Background: Long-chain non-coding RNA (lncRNA) LINC00609 is a potential tumor suppressor, but the mechanism of action in non-small cell lung cancer (NSCLC) is yet to be understood.Objectives: The effects of LINC00609 on A549 cell proliferation, apoptosis, and cell cycle arrest were investigated. Methods: The LINC00609 levels in NSCLC and normal tissues were analyzed by bioinformatics. Expressions of LINC00609, miR-128-3p, and Rho family GTPase 3 (RND3) in NSCLC cells (A549) were determined by qRT-PCR. Bioinformatics analysis predicted target genes and dual-luciferase reporter assays to ensure that LINC00609 targeted miR-128-3p and miR-128-3p targeted RND3. The proliferation of cells was determined using EDU and CCK-8. Flow cytometry was used to evaluate cell apoptosis rate and cell cycle. The western blotting assay identified proteins related to proliferation and apoptosis. Results: In NSCLC tissues, LINC00609 was expressed in low levels, while its high expression was associated with a higher survival rate. LINC00609 affected cell proliferation, apoptosis, cell cycle arrest, and expression of related proteins. Dual-luciferase reporter assay showed that LINC00609 binds specifically to miR-128-3p, and miR-128-3p binds to RND3. MiR-128-3p overexpression could neutralize the effects of LINC00609. A siRNA targeting RND3 could reverse the effect of the miR-128-3p inhibitor. Silencing RND3 resulted in a decrease in apoptosis rate and the number of cells in the S-phase and an increase in the number of cells in the G1-phase. Furthermore, phosphorylation levels of the AKT protein and mTOR protein, and Bcl2 expression, increased; however, the expression of RND3, Bax, and caspase3 decreased. Conclusions: LINC00609 regulated miR-128-3p/RND3 axis to modulate A549 cell proliferation, apoptosis, and cell cycle arrest. In the case of NSCLC, LINC00609 could be a potential target for therapy.Keywords
Lung cancer causes millions of deaths annually due to related high morbidity and mortality (Printz, 2019). Nearly 80% of these are histologically classified as non-small cell lung cancer (NSCLC) (Rolfo et al., 2018). Most NSCLC patients are diagnosed at the stage of advanced or metastatic (Wang and Adjei, 2015). The treatment of NSCLC includes chemotherapy, radiotherapy, and surgery, and primarily, chemotherapy for advanced NSCLC. These approaches have made significant progress in the treatment of NSCLC, but the overall prognosis is still not optimistic (Alexander et al., 2020; Pignon et al., 2008). Therefore, searching for new molecular targets and therapeutic strategies is critical for NSCLC treatment.
Long non-coding RNAs (lncRNAs) are an abundant class of endogenous RNA molecules with more than 200 nucleotides (Wang et al., 2022; Statello et al., 2021). LncRNAs are crucial in regulating mRNA degradation and translation, cell cycle regulation, gene imprinting, splicing, chromatin remodeling, and other biological processes (Engreitz et al., 2016; Liu et al., 2016; Duan et al., 2017). Many studies have shown that lncRNAs play a considerable role in tumorigenesis and development (Lei et al., 2019; Li et al., 2020). In NSCLC, lncRNAs were aberrantly expressed and stimulated or inhibited biological processes, including tumor invasion, migration, and cell cycle control (Ricciuti et al., 2016; Zhang et al., 2017). Metastasis-associated lung adenocarcinoma transcript 1 promoted lung tumor progression in patients with metastatic lung adenocarcinoma (Li et al., 2016). NSCLCs with high gene expression have a poor prognosis (Shen et al., 2015). LNC00609 is an emerging lncRNA, and its role in NSCLC has not been studied.
A series of studies have shown that miRNAs and lncRNAs can jointly affect the progression of malignant tumors (Zhao et al., 2018; Paraskevopoulou and Hatzigeorgiou, 2016; Zhao et al., 2018; Gupta et al., 2022). As one of miRNAs, miR-128-3p is associated with the development of a variety of tumors, including lung cancer (Pan et al., 2018), breast cancer (Li et al., 2019), glioma (Fu et al., 2018), pancreatic cancer (Han et al., 2018), and thyroid cancer (Cao et al., 2019). Rho family GTPase 3 (RND3) is a specific Rho GTP-binding protein, which is closely related to the occurrence and development of cancer. It acts as a tumor suppressor or tumor promoter in different types of cancer (Paysan et al., 2016; Garg et al., 2020). By using the TargetScan database for prediction, we found that miR-128-3p is a suitable target for LINC00609 and targets RND3. Based on these, we set out to reveal the molecular mechanism of LINC00609-mediated regulation of NSCLC, hoping to provide some insights into the causal mechanism of NSCLC development.
A research hotspot has emerged in recent years regarding the regulatory relationships between lncRNA, miRNA, and mRNA, which have been validated to involve in the development and progression of cancer, including lung cancer. The regulatory relationships between lncRNA, miRNA, and mRNA have become a research hotspot in recent years, and the role of these interactions in tumor development and progression has been verified, including those in lung cancer (Li et al., 2017), pancreatic cancer (Ye et al., 2014; Yao et al., 2017), gastric carcinoma (Liu et al., 2019). For example, lncRNAs ANRIL and UFC1 inhibited NSCLC progression by regulating the miR-34a/Myc axis to induce apoptosis and cell cycle arrest (Gupta and Hashimoto, 2022). The clinical potential of identifying biomarkers and therapeutic targets for various tumor types may be enormous, attributed to the interaction between lncRNAs, miRNAs, and mRNAs. In this study, the A549 cell line was used to investigate how LINC00609 affects the proliferation, apoptosis, and cell cycle arrest of A549 cells and to propose new drug targets based on this evidence.
A heat map of the differential expression of lncRNAs was drawn using the DEseq2 package in R from The Cancer Genome Atlas (TCGA) database (https://portal.gdc.cancer.gov/). Selection criteria for |logFC| > 2 and p < 0.05. Application of the LNCipedia database for annotation of differential expressions. According to the heat map results, a box chart was drawn for LINC00609 with a significantly differential expression. A survival analysis chart of differentially expressed LINC00609 was drawn using RTCGA lung cancer clinical data.
A549 cells (AW-CCH011, Abiowell, Changsha, China) were cultured in RPMI 1640 medium (Thermo Fisher Scientific, Waltham, USA) containing 10% fetal bovine serum. The cells were incubated at 37°C and 5% CO2. The A549 cells were divided into two groups, including the NC group and LINC00609 group, transfected with negative control or overexpressed LINC00609, respectively.
The oe-LINC00609 (HG-HO073454), si-LINC00609 (HG-Si073454), miR-128-3p mimic (HG-MI000424), miR-128-3p inhibitor (HG-IN000424), and si-RND3 (HG-SI005168) were purchased from HonorGene (Changsha, China). Lipofectamine 2000 (11668-019, Invitrogen, Waltham, USA) was used to transfect oligonucleotides and vectors into A549 cells, referring to the manufacturer’s directions. Prior to harvesting the treated cells for further experimentation, the cells were incubated at 37°C with 5% CO2.
Quantitative real-time PCR (qRT-PCR)
Cells were extracted with TRIzol reagent (Thermo, USA), and cDNA was reverse transcribed using the extracted total RNA as a template using the mRNA reverse transcription kit (CW2569, CWBIO, Beijing, China). QRT-PCR was performed on a PCR system (QuantStudio1, Thermo Fisher Scientific, Waltham, USA) using UltraSYBR Mixture (CW2601,CWBIO, Beijing, China), cDNA, and specific primers. β-actin and U6 were selected as internal references for analysis of target gene expression. The qRT-PCR primers used in this study are listed in Table 1.
Dual-luciferase reporter assay
The binding site of miR-128-3p was synthesized from LINC00609 or RND3 3′UTR, and its mutant form was inserted into the vector, named WT/MUT-LINC00609 and WT/MUT-RND3, for the luciferase reporter construction. These luciferase reporters were co-transfected with miR-128-3p mimic or miR-NC along with Lipofectamine 2000. Forty-eight hours after luciferase transfection, their activity was measured using a dual luciferase reporter assay (E1910, Promega, USA).
The total proteins were lysed with RIPA buffer (P0013B, Beyotime, Shanghai, China). The proteins were resolved by polyacrylamide gel electrophoresis and transferred onto a nitrocellulose filter membrane. The membrane was blocked for 1.5 h at room temperature. PBST was applied to wash the membranes 3 times after incubation overnight at 4°C with primary antibody. Primary antibodies included RND3 (66228-1-Ig, 1:2000), protein kinase B (AKT; 10176-2-AP, 1:1000), p-AKT (66444-1-Ig, 1:1500), mammalian target of rapamycin (mTOR; 66888-1-Ig, 1:2000), p-mTOR (67778-1-Ig, 1:1000), B-cell lymphoma 2 (Bcl2; 1:2000, Abcam,Cambridge, UK), Bcl-2-associated X protein (Bax; ab32503, 1:5000, Abcam, Cambridge, UK), Caspase3 (#9661, 1:1000, CST, Boston, USA), and β-actin (66009-1-Ig, 1:5000). Then, secondary antibodies against mouse IgG (SA00001-1, 1:5000) and rabbit IgG (SA00001-2, 1:6000) were added and incubated for 1.5 h at room temperature. RND3, AKT, p-AKT, mTOR, p-mTOR, β-actin and all secondary antibodies were purchased from Proteintech (Chicago, USA). We used ECL chromogenic substrate to visualize protein bands. For scanning and imaging, chemiluminescence imaging was used.
5-Ethynyl-2′-deoxyuridine (EDU) assay
The medium for the test was prepared by dilution of EDU solution with cell medium at a ratio of 1000:1. To each well of a 96-well plate, 2000 cells/well and 100 μL EDU culture medium were added and incubated overnight. The medium was discarded, and wells were washed with phosphate-buffered saline (PBS). The plates were incubated for 30 min at about 20°C with 50 μL cell fixation solution (4% paraformaldehyde). To each well, 100 μL of 1 × Apollo staining solution was applied to stain, followed by 100 μL of 1 × Hoechst 33342 solution to fix and then washed 1~3 times with PBS. Observations were made immediately after staining.
In 96-well plates (0030730119, Eppendorf, Hamburg, Germany), approximately 5 × 104 cells were seeded for CCK-8 assay (NU679, Dojindo, Tokyo, Japan). Each group was equipped with 3 multiple holes. The plates were then incubated at 37°C for 4 h, and then 30 μL CCK-8 solution was added after cell adherence and 24 h of culture. In the following step, we measured the absorbance at 450 nm using a Heales Bio-Tek microplate (MB-530, Heales, Shenzhen, China).
Cells were double-stained cells with Annexin V-fluorescein isothiocyanate (Annexin V-FITC) and propidium iodide (PI) to identify apoptotic cells. We collected cells after trypsin digestion without EDTA, washed, and resuspended in the binding buffer. Annexin V-FITC staining and PI staining were performed according to the kit instructions (KGA108; KeyGEN Biotech Cor., Ltd., Nanjing, China). To determine the cell apoptotic rate, an A00-1-11102 flow cytometer (Beckman Coulter, Brea, USA) was used.
The single-cell suspension was prepared by collecting cells from each group cultured for 48 h. The cells were fixed with 75% ethanol and left overnight at 4°C. The suspension was centrifuged at 1000 rpm for 5 min to remove ethanol. Then the cells were labeled with 150 μL PI solution and kept away from light at 4°C for 30 min. The cell cycle distribution was detected and analyzed by flow cytometry.
Graphpad Prism 8.0 (GraphPad Software, San Diego, USA) and IBM SPSS 25 software (IBM, Chicago, USA) was used to perform the statistical analysis. The Shapiro-Wilk test was used to check the normality of the data distribution. Non-normally distributed data were tested with the Wilcoxon rank-sum test, while normal data were tested with the unpaired t-test. The threshold for a statistically significant difference was p-values < 0.05. Each experiment had three replications.
Bioinformatics analysis of the low expression of LINC00609 in non-small cell lung cancer tissues
Lung cancer-related lncRNAs were screened from the TCGA database (Fig. 1A). LINC00609 is a novel up-and-coming lncRNA with fewer reports at present. The heat map shows that LINC00609 was down-regulated in NLCSC tissues (Fig. 1B). A box diagram illustrated that lung cancer tissues expressed LINC00609 at a significantly lower level than normal lungs (Fig. 1C). A survival analysis showed a higher survival rate for lung cancer patients with high LINC00609 expression than those with low expression (Fig. 1D).
Figure 1: Bioinformatics analysis of the low expression of LINC00609 in NSCLC tissues, Tumor (n = 85), Normal (n = 14) (A) The Cancer Genome Atlas (TCGA) screening lncRNAs biogenic volcanic map with differential expression associated with NSCLC (B) Heat map of candidate lncRNAs. (C) TCGA was used to analyze ’the expression level of LINC00609. (D) The Kaplan-Meier survival chart. ***p < 0.001 vs. the normal tissues.
LINC00609 affects the progression of A549 cells
We constructed NSCLC cell lines (A549) overexpressing LINC00609 and silencing LINC00609, respectively, and the results of qRT-PCR showed that the cell model of overexpression LINC00609 and silencing LINC00609 was successfully constructed (Fig. 2A). WB analysis showed that compared with the NC group, LINC00609 overexpression reduced the phosphorylation levels of AKT and mTOR proteins, while LINC00609 silencing increased the phosphorylation levels of AKT and mTOR proteins (Fig. 2B). In the EDU experiment, LINC00609 overexpression inhibited cell proliferation, while silencing LINC00609 promoted cell proliferation (Fig. 2C). Flow cytometry analysis of cell cycle and apoptosis of A549 cells showed that LINC00609 overexpression led to a decrease in the number of cells in the G1-phase, an increase in the number of cells in the S-phase, and an increase in the apoptosis rate, while the effect of LINC00609 silencing was the opposite (Figs. 2D and 2E). WB results showed that LINC00609 overexpression resulted in an increase in the relative expression of Bax and Caspase3 proteins and a decrease in the relative expression of Bcl2 protein, which was opposite to the effect observed after LINC00609 silencing (Fig. 2F). The above results indicated that LINC00609 affects the progression of A549 cells.
Figure 2: LINC00609 affected the progression of A549 cells, (n = 3) (A) Quantitative real-time polymerase chain reaction to assess the expression of LINC00609 in each group. (B) Western blotting (WB) to assess the relative protein expression levels of phosphorylated protein kinase B (p-AKT) and phosphorylated mammalian target of rapamycin (p-mTOR) in A549 cells. (C) 5-Ethynyl-2′-deoxyuridine (EDU) assay was applied to evaluate A549 cell proliferation. Scale bar = 50 μm. (D) Cell distribution at different cell cycle stages was assayed by flow cytometry. (E) The A549 cells were assayed by flow cytometry for apoptosis. (F) WB to assess B-cell lymphoma 2 (Bcl2), Bcl2 associated X protein (Bax), and Caspase3 protein expression in A549 cells. *p < 0.05 vs. the NC group.
LINC00609 affected A549 cells by targeting miR-128-3p
Starbase (http://starbase.sysu.edu.cn/) was employed to predict that miR-128-3p was an appropriate target for LINC00609 (Fig. 3A). To validate the predicted results, we performed a dual-luciferase reporter assay. Compared to mimic-NC, miR-128-3p mimic decreased WT-LINC00609 luciferase activity, but MUT-LINC00609 did not show any significant difference (Fig. 3B). LINC00609 expression decreased, whereas miR-128-3p expression increased after overexpression of miR-128-3p (Fig. 3C). Measurement of cell proliferation in A549 cells by CCK-8 assay. The oe-LINC00609+miR-128-3p mimic group had a greater proliferation rate than the oe-LINC00609+miR-128-3p mimic group (Fig. 3D). Compared with the oe-LINC00609+mimic NC group, the apoptosis rate and the number of cells in the S-phase decreased, while the number of cells in G1 and G2 phases increased of oe-LINC00609+miR-128-3p mimic group (Figs. 3E and 3F). Thus, LINC00609 could affect A549 cells by targeting miR-128-3p.
Figure 3: LINC00609 affects A549 cells by targeting miR-128-3p, (n = 3) (A) A putative target sequence for LINC00609 in miR-128-3p was noted at the 3′-UTR. (B) Luciferase activity was detected with the dual-luciferase reporter assay. (C) Expression levels of LINC00609 and miR-128-3p in the groups were analyzed by quantitative real-time polymerase chain reaction. (D) CCK-8 assay was applied to evaluate A549 cell proliferation. (E) The A549 cells were assayed by flow cytometry for apoptosis. (F) Cell distribution at different cell cycle stages was assayed by flow cytometry. *p < 0.05 vs. the oe-NC+mimic NC group, #p < 0.05 vs. the oe-LINC00609+mimic NC group.
LINC00609 targeted miR-128-3p to regulate RND3 expression in A549 cells
According to bioinformatic predictions, miR-128-3p targeted RND3 directly (Fig. 4A). A dual-luciferase reporter assay further confirmed this relationship. MiR-128-3p mimic reduced WT-RND3 luciferase activity compared to mimic NC, while MUT-RND3 luciferase activity was unaffected (Fig. 4B). Additionally, miR-128-3p expression increased when RND3 was silenced, whereas RND3 expression decreased (Fig. 4C). After silencing RND3, the expression of RND3 protein decreased, while the expression of proteins related to cell proliferation pathway increased (Figs. 4D and 4E). EDU data showed that RND3 silencing promoted proliferation in A549 cells (Fig. 4F). WB analysis displayed a decrease in the relative expression of Bax and Caspase3 proteins, while that for Bcl2 increased (Fig. 4G). The apoptosis rate and cell cycle of A549 cells were analyzed by flow cytometry. The results showed that silencing RND3 resulted in a decrease in apoptosis rate and the number of cells in the S-phase and an increase in the number of cells in the G1-phase (Figs. 4H and 4I). The above data suggested that LINC00609 affected the occurrence and development of A549 cells by targeting miR-128-3p to regulate the expression of RND3.
Figure 4: LINC00609 targets miR-128-3p to regulate Rho family GTPase 3 (RND3) expression in A549 cells, (n = 3) (A) A putative target sequence for RND3 in miR-128-3p was found at the 3’-UTR. (B) Luciferase activity was determined using the dual-luciferase reporter assay. (C) LINC00609 and miR-128-3p expression levels of the groups were analyzed by quantitative real-time polymerase chain reaction. (D and E) Western blotting (WB) to assess the protein expression levels of RND3, protein kinase B (p-AKT), and phosphorylated mammalian target of rapamycin (p-mTOR) in A549 cells. (F) 5-Ethynyl-2’-deoxyuridine (EDU) assay was applied to evaluate A549 cell proliferation. Scale bar = 25 µm. (G) WB to assess the protein expression levels of Bcl2 associated X protein (Bax), Caspase3, and B-cell lymphoma 2 (Bcl2). (H) The A549 cells was assayed by flow cytometry for apoptosis. (I) Cell distribution at different cell cycle stages were assayed by flow cytometry. p < 0.05 vs. the si−NC+inhibitor NC group, #p < 0.05 vs. the miR-128-3p inhibitor+si−NC group.
Our current results indicated low levels of LINC00609 expression in NSCLC tissues, while high expression was connected with a higher survival rate. We also found that LINC00609 affected cell proliferation, apoptosis, cell cycle arrest, and the expression of related proteins. Analysis of bioinformatics data and dual-luciferase reporter assays confirmed the targeted binding of LINC00609 to miR-128-3p and binding of miR-128-3p to RND3. Based on the above results, LINC00609 regulated the miR-128-3p/RND3 axis to modulate A549 cell proliferation and apoptosis.
LncRNAs may be evidently involved in gene expression, proliferation, and apoptosis of tumor cells and influence cancer development (Lei et al., 2019; Li et al., 2020). With the gradual advancement for the past few years, various lncRNAs have been found to inhibit or promote cancer in NSCLC (Zhang et al., 2019). For example, PVT1 regulates the epigenetic expression of large tumor suppressor kinase 2 and promotes the proliferation of NSCLC cells (Wan et al., 2016). LINC00609 has received increasing attention as an emerging lncRNA. However, no studies have been conducted on its role in lung cancer. We first evaluated the expression of LINC00609 in NSCLC tissues by bioinformatics analysis and found its low expression in NSCLC tissues, while its high expression was associated with a higher survival rate, which needs to be further verified by qRT-PCR experiments in the future. To better understand the role of LINC00609 in NSCLC, we constructed an LINC00609 overexpression vector and a silencing vector. LINC00609 affected A549 cell proliferation, apoptosis, cell cycle arrest, and the expression of related proteins. Based on these findings, NSCLC genes LINC00609 appear to function as tumor suppressors.
A series of studies have indicated that miRNAs and lncRNAs could target each other and jointly contribute to the progression of malignant tumors (Zhao et al., 2018; Paraskevopoulou and Hatzigeorgiou, 2016; Zhao et al., 2018). MiRNAs are crucial in cell proliferation, infiltration, and migration (Yu et al., 2017). NSCLC was found to be strongly linked to lncRNAs that regulate gene and target expression (Zheng et al., 2017). LncRNA HOXA11-As, for instance, inhibited the miR-214-3p expression and induced epithelial-mesenchymal transformation in hepatocellular carcinoma cells by increasing proliferation and invasion (Zhan et al., 2018). As an miRNA, miR-128-3p has been relevant to the proliferation, differentiation, apoptosis, and invasion of certain tumor cells (Fang et al., 2022; Palumbo et al., 2013). The results of bioinformatics analysis and dual-luciferase experiments demonstrated that LINC00609 was capable of binding to miR-128-3p in a competitive manner. Overexpression of miR-128-3p could eliminate the effect of LINC00609, demonstrating that miR-128-3p appeared to participate in regulating LINC00609's effect on proliferation and apoptosis in A549 cells.
A specific Rho GTP-binding protein, RND3, regulates cell morphology, proliferation, division, and migration (Garg et al., 2020). RND3 appears as a tumor suppressor or promoter, depending on tumor type. For example, RND3 expression was decreased in prostate, hepatocellular, and squamous cell cancers while up-regulated in the brain and gastric cancers (Paysan et al., 2016). In the current study, miR-128-3p directly targeted RND3. Silencing RND3 resulted in a decrease in apoptosis rate and the number of cells in the S-phase and an increase in the number of cells in the G1-phase. Furthermore, phosphorylation levels of the AKT protein and mTOR protein and the relative expression levels of Bcl2 increased; however, Bax and caspase3 expression levels decreased. In conclusion, LINC00609 could influence A549 cell proliferative and apoptotic processes by regulating the miR-128-3p/RND3 axis.
There are some limitations in this study. First, the role of LINC00609 in other NSCLC cell lines cannot be determined by using only the A549 cell line, and its exact role needs to be explored by multiple cell lines. Second, LINC00609 is a novel lncRNA that has not been reported previously, and its exact regulatory mechanism still needs to be analyzed in a sufficient number of NSCLC samples. Finally, the downstream mechanisms and molecular pathways still need to be further explored to clarify the specific molecular mode of action behind the biological functions of LINC00609 in the development of NSCLC. In general, this study reported that LINC00609 regulated the miR-128-3p/RND3 axis to modulate A549 cell proliferation and apoptosis, which may assist in developing future diagnostic targets for NSCLC.
Acknowledgement: The authors would like to thank the Renmin Hospital of Wuhan University for their technical assistance.
Funding Statement: This research was supported by the Fundamental Research Funds for the Central Universities (No. 2042021kf1038).
Author Contributions: Conceptualization: Huiqing Lin; Data curation: Xiangchao Ding, Yang Zhao, Huiqing Lin; Formal analysis: Xinghua Zhang; Funding acquisition: Huiqing Lin; Methodology and visualization: Xiangchao Ding; Writing—original draft: Xiangchao Ding; Writing—review & editing: All authors.
Availability of Data and Matrials: Data sharing not applicable to this article as no datasets were generated or analyzed during the current study.
Ethics Approval: Not applicable.
Conflicts of Interest: The authors declare that they have no conflicts of interest to report regarding the present study.
References
Alexander M, Kim SY, Cheng H (2020). Update 2020: Management of non-small cell lung cancer. Lung 198: 897–907. https://doi.org/10.1007/s00408-020-00407-5 [Google Scholar] [PubMed] [CrossRef]
Cao XZ, Bin H, Zang ZN (2019). MiR-128 suppresses the growth of thyroid carcinoma by negatively regulating SPHK1. Biomedicine & Pharmacotherapy 109: 1960–1966. https://doi.org/10.1016/j.biopha.2018.08.052 [Google Scholar] [PubMed] [CrossRef]
Duan L, Cheng M, Niu Y, Chi X, Liu X, Fan J, Fan H, Chang Y, Yang W (2017). Identification of a novel human long non-coding RNA that regulates hepatic lipid metabolism by inhibiting SREBP-1c. International Journal of Biological Sciences 13: 349–357. https://doi.org/10.7150/ijbs.16635 [Google Scholar] [PubMed] [CrossRef]
Engreitz JM, Haines JE, Perez EM, Munson G, Chen J, Kane M, McDonel PE, Guttman M, Lander ES (2016). Local regulation of gene expression by lncRNA promoters, transcription and splicing. Nature 539: 452–455. https://doi.org/10.1038/nature20149 [Google Scholar] [PubMed] [CrossRef]
Fang W, Shi C, Wang Y, Song J (2022). Zhang L microRNA-128-3p inhibits CD4+ regulatory T cells enrichment by targeting interleukin 16 in gastric cancer. Bioengineered 13: 1025–1038. https://doi.org/10.1080/21655979.2021.2017566 [Google Scholar] [PubMed] [CrossRef]
Fu C, Li D, Zhang X, Liu N, Chi G, Jin X (2018). LncRNA PVT1 facilitates tumorigenesis and progression of glioma via regulation of miR-128-3p/GREM1 axis and BMP signaling pathway. Neurotherapeutics 15: 1139–1157. https://doi.org/10.1007/s13311-018-0649-9 [Google Scholar] [PubMed] [CrossRef]
Garg R, Koo CY, Infante E, Giacomini C, Ridley AJ, Morris JDH (2020). Rnd3 interacts with TAO kinases and contributes to mitotic cell rounding and spindle positioning. Journal of Cell Science 133: jcs235895. https://doi.org/10.1242/jcs.235895 [Google Scholar] [PubMed] [CrossRef]
Gupta S, Hashimoto RF (2022). Dynamical analysis of a boolean network model of the oncogene role of lncRNA ANRIL and lncRNA UFC1 in non-small cell lung cancer. Biomolecules 12: 420. https://doi.org/10.3390/biom12030420 [Google Scholar] [PubMed] [CrossRef]
Gupta S, Panda PK, Luo W, Hashimoto RF, Ahuja R (2022). Network analysis reveals that the tumor suppressor lncRNA GAS5 acts as a double-edged sword in response to DNA damage in gastric cancer. Scientific Reports 12: 18312. https://doi.org/10.1038/s41598-022-21492-x [Google Scholar] [PubMed] [CrossRef]
Han H, Wang L, Xu J, Wang A (2018). miR-128 induces pancreas cancer cell apoptosis by targeting MDM4. Experimental and Therapeutic Medicine 15: 5017–5022. https://doi.org/10.3892/etm.2018.6047 [Google Scholar] [PubMed] [CrossRef]
Lei Y, Shi Y, Duan J, Liu Y, Lv G, Shi R, Zhang F, Yang Q, Zhao W (2019). Identification of alternative splicing and lncRNA genes in pathogenesis of small cell lung cancer based on their RNA sequencing. Advances in Clinical and Experimental Medicine 28: 1043–1050. https://doi.org/10.17219/acem/94392 [Google Scholar] [PubMed] [CrossRef]
Li DY, Chen WJ, Luo L, Wang YK, Shang J, Zhang Y, Chen G, Li SK (2017). Prospective lncRNA-miRNA-mRNA regulatory network of long non-coding RNA LINC00968 in non-small cell lung cancer A549 cells: A miRNA microarray and bioinformatics investigation. International Journal of Molecular Medicine 40: 1895–1906. https://doi.org/10.3892/ijmm.2017.3187 [Google Scholar] [PubMed] [CrossRef]
Li Y, Jiang T, Zhou W, Li J, Li X et al. (2020). Pan-cancer characterization of immune-related lncRNAs identifies potential oncogenic biomarkers. Nature Communications 11: 1000. https://doi.org/10.1038/s41467-020-14802-2 [Google Scholar] [PubMed] [CrossRef]
Li J, Wang J, Chen Y, Li S, Jin M, Wang H, Chen Z, Yu W (2016). LncRNA MALAT1 exerts oncogenic functions in lung adenocarcinoma by targeting miR-204. American Journal of Cancer Research 6: 1099–1107. [Google Scholar] [PubMed]
Li Y, Wang Y, Shen X, Han X (2019). miR-128 functions as an oncomiR for the downregulation of HIC1 in breast cancer. Frontiers in Pharmacology 10: 1202. https://doi.org/10.3389/fphar.2019.01202 [Google Scholar] [PubMed] [CrossRef]
Liu X, Xiao ZD, Han L, Zhang J, Lee SW, Wang W, Lee H, Zhuang L, Chen J, Lin HK (2016). LncRNA NBR2 engages a metabolic checkpoint by regulating AMPK under energy stress. Nature Cell Biology 18: 431–442. https://doi.org/10.1038/ncb3328 [Google Scholar] [PubMed] [CrossRef]
Liu Y, Zhu J, Ma X, Han S, Xiao D, Jia Y, Wang Y (2019). ceRNA network construction and comparison of gastric cancer with or without Helicobacter pylori infection. Journal of Cellular Physiology 234: 7128–7140. https://doi.org/10.1002/jcp.27467 [Google Scholar] [PubMed] [CrossRef]
Palumbo T, Faucz FR, Azevedo M, Xekouki P, Stratakis CA (2013). Functional screen analysis reveals miR-26b and miR-128 as central regulators of pituitary somatomammotrophic tumor growth through activation of the PTEN-AKT pathway. Oncogene 32: 1651–1659. https://doi.org/10.1038/onc.2012.190 [Google Scholar] [PubMed] [CrossRef]
Pan J, Zhou C, Zhao X, He J, Tian H et al. (2018). A two-miRNA signature (miR-33a-5p and miR-128-3p) in whole blood as potential biomarker for early diagnosis of lung cancer. Scientific Reports 8: 16699. https://doi.org/10.1038/s41598-018-35139-3 [Google Scholar] [PubMed] [CrossRef]
Paraskevopoulou M, Hatzigeorgiou AG (2016). Analyzing MiRNA-LncRNA interactions. Methods in Molecular Biology 1402: 271–286. https://doi.org/10.1007/978-1-4939-3378-5_21 [Google Scholar] [PubMed] [CrossRef]
Paysan L, Piguet L, Saltel F, Moreau V (2016). Rnd3 in cancer: A review of the evidence for tumor promoter or suppressor. Molecular Cancer Research 14: 1033–1044. https://doi.org/10.1158/1541-7786.MCR-16-0164 [Google Scholar] [PubMed] [CrossRef]
Pignon JP, Tribodet H, Scagliotti GV, Douillard JY, Shepherd FA et al. (2008). Lung adjuvant cisplatin evaluation: A pooled analysis by the LACE collaborative group. Journal of Clinical Oncology 26: 3552–3559. https://doi.org/10.1200/jco.2007.13.9030 [Google Scholar] [PubMed] [CrossRef]
Printz C (2019). Study projects a 43% increase in lung cancer mortality among women: New research suggests that, among women, strides in reducing global deaths from breast cancer could be tempered by rising lung cancer deaths. However, according to experts, the projections may not reveal the full picture. Cancer 125: 831–832. https://doi.org/10.1002/cncr.32036 [Google Scholar] [PubMed] [CrossRef]
Ricciuti B, Mencaroni C, Paglialunga L, Paciullo F, Crinò L, Chiari R, Metro G (2016). Long noncoding RNAs: New insights into non-small cell lung cancer biology, diagnosis and therapy. Medical Oncology 33: 18. https://doi.org/10.1007/s12032-016-0731-2 [Google Scholar] [PubMed] [CrossRef]
Rolfo C, Mack PC, Scagliotti GV, Baas P, Barlesi F et al. (2018). Liquid biopsy for advanced non-small cell lung cancer (NSCLCA statement paper from the IASLC. Journal of Thoracic Oncology 13: 1248–1268. https://doi.org/10.1016/j.jtho.2018.05.030 [Google Scholar] [PubMed] [CrossRef]
Shen L, Chen L, Wang Y, Jiang X, Xia H, Zhuang Z (2015). Long noncoding RNA MALAT1 promotes brain metastasis by inducing epithelial-mesenchymal transition in lung cancer. Journal of Neuro-Oncology 121: 101–108. https://doi.org/10.1007/s11060-014-1613-0 [Google Scholar] [PubMed] [CrossRef]
Statello L, Guo CJ, Chen LL, Huarte M (2021). Gene regulation by long non-coding RNAs and its biological functions. Nature Reviews Molecular Cell Biology 22: 96–118. https://doi.org/10.1038/s41580-020-00315-9 [Google Scholar] [PubMed] [CrossRef]
Wan L, Sun M, Liu GJ, Wei CC, Zhang EB, Kong R, Xu TP, Huang MD, Wang ZX (2016). Noncoding RNA PVT1 promotes non-small cell lung cancer cell proliferation through epigenetically regulating LATS2 expression. Molecular Cancer Therapeutics 15: 1082–1094. https://doi.org/10.1158/1535-7163.MCT-15-0707 [Google Scholar] [PubMed] [CrossRef]
Wang X, Adjei AA (2015). Lung cancer and metastasis: New opportunities and challenges. Cancer Metastasis Reviews 34: 169–171. https://doi.org/10.1007/s10555-015-9562-4 [Google Scholar] [PubMed] [CrossRef]
Wang H, Meng Q, Qian J, Li M, Gu C, Yang Y (2022). Review: RNA-based diagnostic markers discovery and therapeutic targets development in cancer. Pharmacology & Therapeutics 234: 108123. https://doi.org/10.1016/j.pharmthera.2022.108123 [Google Scholar] [PubMed] [CrossRef]
Yao K, Wang Q, Jia J, Zhao H (2017). A competing endogenous RNA network identifies novel mRNA, miRNA and lncRNA markers for the prognosis of diabetic pancreatic cancer. Tumour Biology 39: 1010428317707882. https://doi.org/10.1177/1010428317707882 [Google Scholar] [PubMed] [CrossRef]
Ye S, Yang L, Zhao X, Song W, Wang W, Zheng S (2014). Bioinformatics method to predict two regulation mechanism: TF-miRNA-mRNA and lncRNA-miRNA-mRNA in pancreatic cancer. Cell Biochemistry and Biophysics 70: 1849–1858. https://doi.org/10.1007/s12013-014-0142-y [Google Scholar] [PubMed] [CrossRef]
Yu N, Zhang Q, Liu Q, Yang J, Zhang S (2017). A meta-analysis: MicroRNAs’ prognostic function in patients with nonsmall cell lung cancer. Cancer Medicine 6: 2098–2105. https://doi.org/10.1002/cam4.1158 [Google Scholar] [PubMed] [CrossRef]
Zhan M, He K, Xiao J, Liu F, Wang H et al. (2018). LncRNA HOXA11-AS promotes hepatocellular carcinoma progression by repressing miR-214-3p. Journal of Cellular and Molecular Medicine 22: 3758–3767. https://doi.org/10.1111/jcmm.13633 [Google Scholar] [PubMed] [CrossRef]
Zhang Y, Chen WJ, Gan TQ, Zhang XL, Xie ZC et al. (2017). Clinical significance and effect of lncRNA HOXA11-AS in NSCLC: A study based on bioinformatics, in vitro and in vivo verification. Scientific Reports 7: 5567. https://doi.org/10.1038/s41598-017-05856-2 [Google Scholar] [PubMed] [CrossRef]
Zhang Z, Peng Z, Cao J, Wang J, Hao Y, Song K, Wang Y, Hu W, Zhang X (2019). Long noncoding RNA PXN-AS1-L promotes non-small cell lung cancer progression via regulating PXN. Cancer Cell International 19: 20. https://doi.org/10.1186/s12935-019-0734-0 [Google Scholar] [PubMed] [CrossRef]
Zhao CX, Wei Z, Ba ZQ, Xu HJ, Liu WD (2018). The regulatory network of nasopharyngeal carcinoma metastasis with a focus on EBV, lncRNAs and miRNAs. American Journal of Cancer Research 8: 2185–2209. [Google Scholar] [PubMed]
Zheng Y, Deng Z, Yin J, Wang S, Lu D, Wen X, Li X, Xiao D, Hu C, Chen X et al. (2017). The association of genetic variations in DNA repair pathways with severe toxicities in NSCLC patients undergoing platinum-based chemotherapy. International Journal of Cancer 141: 2336–2347. https://doi.org/10.1002/ijc.30921 [Google Scholar] [PubMed] [CrossRef]
Cite This Article
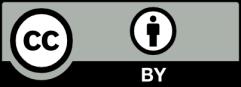
This work is licensed under a Creative Commons Attribution 4.0 International License , which permits unrestricted use, distribution, and reproduction in any medium, provided the original work is properly cited.