Open Access
ARTICLE
UCK2 promotes the proliferation, migration, and invasion of trophoblast cells in preeclampsia by activating the STAT3 pathway
1 Department of Cardiology, Tianjin First Central Hospital, Tianjin, 300192, China
2 Department of Hypertension, TEDA International Cardiovascular Hospital, Tianjin, 300457, China
3 Department of Dermatology, Tianjin Children’s Hospital, Tianjin, 300134, China
4 TEDA International Cardiovascular Hospital, Tianjin, 300457, China
5 School of Medicine, Nankai University, Tianjin, 300071, China
* Corresponding Authors: Chengzhi Lu, ; Yuming Li,
BIOCELL 2023, 47(4), 837-847. https://doi.org/10.32604/biocell.2023.026161
Received 19 August 2022; Accepted 15 November 2022; Issue published 08 March 2023
Abstract
Background: Preeclampsia (PE), characterized by hypertension and proteinuria, leads to serious maternal and infant complications. Uridine-cytidine kinase 2 (UCK2) belongs to the UCK family, a class of enzymes that catalyzes the conversion of uridine and cytidine to monophosphate form. However, the role of UCK2 in PE has not been reported. Methods: The expression of UCK2 was detected in the placenta of PE patients and N(ω)-nitro-L-arginine methyl esterinduced PE mouse model. Through forced up-regulation or down-regulation of UCK2 in vitro, we examined the effects of UCK2 on the proliferation, apoptosis, migration, and invasion of trophoblast cells. Stattic, the inhibitor of STAT3 pathway, was used to investigate whether the STAT3 pathway mediates the biological function of UCK2 in trophoblast cells. Results: The present study found that UCK2 showed low expression in the placenta of PE patients and PE mouse model. MTT (3-(4,5-dimethylthiazol-2-yl)-2,5-diphenyltetrazolium bromide) and flow cytometry assays verified that up-regulation of UCK2 promoted the proliferation of trophoblast cells, while the silence of UCK2 suppressed cell proliferation. Besides, flow cytometry and TdT-mediated dUTP Nick-End Labeling assays demonstrated that knockdown of UCK2 resulted in apoptosis of trophoblast cells. The wound healing and transwell assays showed that the migration and invasion activities of the trophoblast cells were facilitated by the overexpression of UCK2 and were blocked by the silence of UCK2. Furthermore, the expression of phosphorylated STAT3 was increased with the upregulation of UCK2 and decreased with the inhibition of UCK2. When the STAT3 pathway was blocked by its inhibitor stattic, the promotion effects of UCK2 on trophoblast cells were suppressed. Conclusion: UCK2 promotes the proliferation, migration, and invasion of trophoblast cells, and these effects may be partly mediated by the activation of the STAT3 pathway.Keywords
Preeclampsia (PE) is an obstetric disorder characterized by hypertension and proteinuria (Smyth et al., 2017), and it leads to serious maternal and infant complications (Mol et al., 2016; Murray et al., 2021). A more serious condition is a seizure or coma that develops into eclampsia (North et al., 1994; Lin et al., 1995). Meanwhile, one of the leading causes of PE is hypertensive disorders in pregnancy, which is an important cause of cardiovascular disease in women (Chen et al., 2014). In many countries, complications of pregnancy and childbirth result in a high mortality rate for fertile women. Preeclampsia and eclampsia are the important causes of high mortality (Duley, 2009). There is currently no effective treatment other than the termination of pregnancy. Therefore, investigating the pathogenesis of PE may be an effective strategy to improve cardiovascular disease in females and reduce mortality (Paauw and Lely, 2018; Neerukonda et al., 2020). Previous studies have shown that the invasion and migration abilities of trophoblast cells are closely related to the process of placenta formation. The lack of trophoblast cell migration and invasion ability leads to spiral artery trophoblast recasting disorder (Gao et al., 2021), resulting in insufficient blood supply to the placenta and even malformation, which is one of the important reasons for PE (Turco and Moffett, 2019; Staff et al., 2020). With the development of technologies and biomedical sciences, the mechanism affecting the migration and invasion ability of trophoblast cells needs to be further explored.
Uridine-cytidine kinase (UCK) is an enzyme that catalyzes the conversion of uridine and cytidine to monophosphate form (van Rompay et al., 2001). There are two members of UCK family existing in human cells, UCK1 and UCK2. UCK2 is located on chromosome 1q23 (Schumacher et al., 2013), and the catalytic efficiency of UCK2 is 15–20 times higher than that of UCK1. Till now, UCK2 is only observed in the placenta and testis of normal humans (Yu et al., 2019). It has been documented that UCK2 plays a vital role in the chemotherapy of various cancers, such as hepatocellular carcinoma (Yu et al., 2019; Cai et al., 2020), lung cancer (Wu et al., 2019), and melanoma (Wu et al., 2021). However, the role of UCK2 in pregnancy-related diseases has not been reported. According to the bioinformatic analysis of GSE147776 datasets, we found that UCK2 expression is reduced in PE placental tissues compared with normal tissues. Therefore, we further explored and verified the function and mechanism of UCK2 in trophoblast cells.
The signal transducer and activator of transcription 3 (STAT3), which mediates various cellular signaling pathways (Darnell et al., 1994), is a crucial factor involved in numerous diseases (Yue and Turkson, 2009). It has been verified to be involved in plenty of biological processes, including cell proliferation, apoptosis, migration, and invasion (Rawlings et al., 2004; Hanlon et al., 2019). At the same time, overactivation of STAT3 is found in multiple human cancers and is associated with poor prognosis (Johnson et al., 2018). The important role of STAT3 in tumor formation and development makes it a potential target for tumor therapy (Bromberg et al., 1999; Ishibashi et al., 2018; Priego et al., 2018). Stattic, as an inhibitor, suppresses the phosphorylation of STAT3 by acting on STAT3 SH2 (Schust et al., 2006). The present study aims to investigate whether UCK2 affects the biological behaviors of trophoblast cells and whether STAT3 plays a role in these processes.
Bioinformatics analysis of Gene Expression Omnibus (GEO) database
GSE147776 is a gene expression profile obtained from placental samples by microarray analysis (Medina-Bastidas et al., 2020). GEO2R (https://www.ncbi.nlm.nih.gov/geo/geo2r/), as a convenient online tool, was used to screen the significantly-downregulated genes (log2FC < −1, p < 0.05) in PE placental tissues comparing to normal samples. Gene Ontology (GO) enrichment analysis of these genes was performed using the database for annotation, visualization and, integrated discovery (DAVID, https://david.ncifcrf.gov/home.jsp) (Sherman et al., 2022).
Healthy female mice (10–15 weeks old) were prepared for the experiment. The mice were kept in a stable environment of free food and water, temperature (22 ± 1°C), and lighting for 12 h light/dark cycle. When the female mice were mated with male mice, the day that a vaginal plug appeared was regarded as day 0 of pregnancy. Pregnant mice were randomly divided into the control group and the PE group. The mice in PE group were injected with 50 mg/kg N(ω)-nitro-L-arginine methyl ester (L-NAME) subcutaneously from day 7 to day 17, and the mice in the control group were injected with the same amount of solvent daily. Mean arterial pressure (MAP) was monitored every 2 days from day 2 to day 18. The 24 h-urine was collected on day 17 to detect total protein content using a urine protein test kit (Nanjing Jiancheng, Nanjing, China). Placental tissues were collected on day 18 for follow-up experiments. Before being sacrificed, the mice were injected with 200 mg/kg of pentobarbital sodium. All efforts were made to minimize the suffering of animals. The procedures used in this research were in accordance with the Guidelines for the Care and Use of Laboratory Animals and were approved by the Ethical Committee of Nankai University (No. 2022-SYDWLL-000273).
Human chorionic trophoblast HTR-8/SVneo cells were purchased from Zhong Qiao Xin Zhou Biotechnology (Shanghai, China). The cells were cultured in RPMI-1640 (Solarbio, Beijing, China) with 10% fetal bovine serum (FBS) in an incubator with 5% CO2 at 37°C. We purchased a commercialized plasmid named pDONR223-UCK2 cDNA clone (Cat#G163428) from YouBio Inc. (Changsha, China) to upregulate UCK2 expression. UCK2 was knocked down using specific siRNAs synthesized by General Biol. (Chuzhou, China). The target mRNA sequences of UCK2 were as follows: siUCK2-1: 5′-AGAAAUCACUGAAGGGAAA-3′, siUCK2-2: 5′-CCCUAGAGGUGCAGAUAAU-3′. Vector and siRNAs were transfected into cells using lipofectamine 3000 (Cat#L3000008, Invitrogen, Carlsbad, CA, USA). After transfection, cells were treated with 1 μM stattic (Shanghai yuanye Bio-Technology, Shanghai, China) to block the expression of STAT3.
Real-time quantitative polymerase chain reaction (RT-qPCR)
Total RNA was isolated from cells or tissues by TRIpure (BioTeke, Beijing, China). The concentration of RNA was detected by ultraviolet spectrophotometer NANO 2000. RNA was reverse-transcribed using M-MLV reverse transcriptase (Beyotime, Shanghai, China) and RNase inhibitor (BioTeke, Beijing, China). 2 × Taq PCR MasterMix (Solarbio, Beijing, China), SYBR Green (Solarbio, Beijing, China), and primers were mixed with cDNA to examine the relative mRNA expression, which was normalized to GAPDH (glyceraldehyde 3-phosphate dehydrogenase) expression. Primer information is listed in Table 1.
Total proteins were extracted from trophoblast cells or the placenta tissues by RIPA and PMSF (Solarbio, Beijing, China). The protein concentrations were determined using Solarbio BCA Protein Assay Kit. Proteins separated by sodium dodecyl sulfate-polyacrylamide gel electrophoresis were transferred onto a polyvinylidene fluoride membrane (Millipore, Bedford, USA). After blocking by skim milk, the proteins were incubated with specific primary antibodies at 4°C overnight. Then the secondary antibodies were incubated for 1 h at 37°C. The details of the antibodies are listed in Table 2.
MTT (3-(4,5-dimethylthiazol-2-yl)-2,5-diphenyltetrazolium bromide) assay
The cells were seeded in the 96-well plates with 3 × 103 cells in each well, and five repeated wells were set in each group. When the cells were cultured for a specific time, the medium was removed, 20 μL of MTT staining solution (KeyGEN, Nanjing, China) was added, and the cells were incubated in the incubator for 4 h. After carefully absorbing the supernatant, 150 μL DMSO was added to cells for 10 min, then the optical density (OD) value was measured by an enzyme-labeled instrument (BIOTEK, VT, USA).
After 48 h transfection, the cells were washed with phosphate buffer-saline (PBS), fixed in 70% cold ethanol for 12 h, and then treated with 25 μL propidium iodide (PI) and 10 μL RNase A at 37°C for 30 min. According to the instructions of the cell cycle assay kit (Beyotime, Shanghai, China), cell cycle distribution was examined using NovoCyte flow cytometry (ACEA Biosciences, CA, USA). Forty-eight hours after transfection, the cells were washed with PBS and treated with Annexin V-FITC Apoptosis Detection Kit (KeyGEN, Nanjing, China). The cell apoptosis was detected by NovoCyte flow cytometry.
Terminal deoxynucleotidyl transferase dUTP nick end labeling (TUNEL) assay
Cells were infiltrated with 0.1% Triton X-100 (Beyotime, Shanghai, China) and incubated with TUNEL reaction liquid of In Situ Cell Death Detection Kit (Roche, Basel, Switzerland) at 37°C for 60 min. After washing with PBS 3 times, cells were stained with DAPI for 5 min. BX53 fluorescence microscope (Olympus, Tokyo, Japan) was used to take pictures.
To estimate the effects of UCK2 on the migration ability of trophoblast cells, a wound-healing assay was performed. When the cell culture reached the fusion state, the medium was replaced with a serum-free medium, and cells were treated with 1 μg/mL mitomycin C (Sigma, MO, USA) for 1 h. Cells were scratched with a 200 μL pipette tip and photographed by a microscope (100×). The cells were cultured in an incubator with 5% CO2 at 37°C for 24 h and then photographed to calculate the migration rate of the cells. The migration rate is calculated using the formula [Migration rate = (0 h average width of wound - 24 h width of the wound)/0 h average width of wound × 100%].
The cells were seeded in the 6-well plates until their density reached 90%. 200 μL of cell suspension (1 × 105 cells/mL) was added to the Transwell chamber (Corning, NY, USA) containing Matrigel glue (BD Biosciences, CA, USA), and 800 μL of medium containing 10% fetal bovine serum (FBS) was added to the lower chamber. After 48 h, cells were fixed with 4% paraformaldehyde (Aladdin, Shanghai, China) for 20 min and stained with 0.5% crystal violet (Amresco, OH, USA) for 5 min. The cells were counted under an IX53 inverted microscope (Olympus, Tokyo, Japan).
Statistical analysis and graphical representation were performed using GraphPad Prism 8 software. Differences between two groups were analyzed by Student’s t-test, and differences among three or more groups were determined using the one-way analysis of variance. The data were presented as the mean ± standard deviation. The p < 0.05 was considered that the results have statistical significance.
UCK2 shows low expression in the PE placental tissues
Differently expressed genes in the GSE147776 dataset are presented in Fig. 1A. The significant-downregulated genes were enriched in GO terms (Fig. 1B). The 15 GO terms with the highest count of enriched genes in the biological process category were visualized. The genes enriched in the GO terms associated with typical cell biological behavior in PE are displayed in Fig. 1C. UCK2 was enriched in “in utero embryonic development” and “regulation of trophoblast cell migration”; however, its role in PE is still not clear. We further estimated the expression of UCK2 in the dataset, and the results suggested that UCK2 had significantly lower expression in the placenta tissues of patients with PE than that of the healthy controls (Fig. 1D).
Figure 1: Differently expressed genes in the placenta of preeclampsia (PE) patients and healthy pregnancies. (A) Volcano plot of the expression of genes in the GSE147776 dataset. Each dot represents a gene. Genes were marked in different colors. (B) Gene Ontology (GO) enrichment analysis of the significant-downregulated genes. (C) Genes enriched in the GO terms that are associated with typical cell biological behavior in PE. (D) UCK2 expression in the placenta of control or PE patients was analyzed in the GSE147776 dataset. The data shown are mean ± standard deviation. **p < 0.01.
Downregulation of UCK2 in the placental tissues of N(ω)-nitro-L-arginine methyl ester-induced preeclampsia mice
Hypertension and proteinuria are important features of PE (Hauth et al., 2000; Sibai, 2003; Stefańska et al., 2020). During the establishment of the PE mouse model, we monitored their blood pressure and urinary protein levels. After L-NAME injection, the MAP was significantly upregulated compared with the control mice from day 12 to day 18 of pregnancy (Fig. 2A). The 24-h urine protein content of L-NAME-treated mice was significantly higher than that of the control group (Fig. 2B). The two images illustrated that the classical PE-like models were successfully established by L-NAME treatment. Moreover, the mRNA and protein expression of UCK2 was decreased in the placenta tissue of the PE mouse model (Figs. 2C and 2D). The above results demonstrated lowered expression of UCK2 in the placental tissues of the PE mouse model.
Figure 2: The expression level of Uridine-cytidine kinase 2 (UCK2) in PE. (A) Changes in mean arterial pressure (MAP) of pregnant mice from day 2 to day 18 of pregnancy. (B) The 24 h-urine protein of the pregnant mice on day 17. (C and D) The UCK2 mRNA and protein expression in the placental tissues of PE mice induced by L-NAME and normal mice were detected by RT-qPCR and western blot. The data shown are mean ± SD. **p < 0.01.
Uridine-cytidine kinase 2 promotes the proliferation of trophoblast cells
As shown in Figs. 3A and 3B, the results of real-time PCR and western blot clarified that the relative mRNA and protein expression of UCK2 was remarkably upregulated in the HTR-8/SVneo cells when UCK2 was overexpressed and was downregulated by the silence of UCK2. MTT results showed that the proliferation of trophoblast cells was enhanced by the overexpression of UCK2 and was suppressed by the siUCK2-1 and siUCK2-2 (Fig. 3C). When UCK2 was upregulated, the expression of cyclinD1 and cyclinE proteins increased significantly. When UCK2 was knocked-down, the expression decreased in the trophoblast cells (Fig. 3D). Results of flow cytometry verified that the cells in S and G2 phases were significantly increased by UCK2 overexpression, while the cells in the G1 phase were significantly decreased. After UCK2 was knocked down, the cells in the G1 phase were dramatically increased, and those in the S and G2 phases were remarkably reduced (Fig. 3E). These results indicated that UCK2 could promote the proliferation of trophoblast cells.
Figure 3: Uridine-cytidine kinase 2 (UCK2) facilitates the proliferation of trophoblast cells. (A and B) The efficiency of UCK2 overexpression and inhibition in trophoblast HTR-8/SVneo cells was detected by real-time quantitative polymerase chain reaction and western blot. (C) MTT assay was performed to detect the effects of UCK2 on the proliferation of HTR-8/SVneo cells. @@ p < 0.01 UCK2 vs. vector, ## p < 0.01 siUCK2-1, siUCK2-2 vs. siNC. (D) The protein expression of cyclinD1 and cyclinE was evaluated by western blot. (E) Cell cycle was detected using flow cytometry. The data shown are mean ± standard deviation. ns means not significant. **p < 0.01.
UCK2 gene knockdown contributes to the apoptosis of trophoblast cells
The apoptosis proportion of HTR-8/SVneo was detected by flow cytometry. When UCK2 was knockdown by siUCK2-1 and siUCK2-2, the apoptosis rate was significantly increased (Fig. 4A). UCK2-downregulation-induced apoptosis was also confirmed by the TUNEL assay (Fig. 4B). Moreover, the protein expression of cleaved-caspase-3 and cleaved-PARP was significantly increased after siUCK2-1 and siUCK2-2 treatment (Fig. 4C). Taken together, the knockdown of UCK2 enhanced the apoptosis of trophoblast cells.
Figure 4: Uridine-cytidine kinase 2 (UCK2) suppresses the apoptosis of trophoblast cells. (A) Cell apoptosis was examined using flow cytometry. (B) TdT-mediated dUTP Nick-End Labeling staining to evaluate the apoptosis of HTR-8/SVneo. Scale bar = 50 μm. (C) The protein changes of cleaved-caspase-3 and cleaved-PARP were measured by western blotting. The data shown are mean ± standard deviation. ns means not significant. **p < 0.01.
Uridine-cytidine kinase 2 enhances the migration and invasion of trophoblast cells
As depicted in Fig. 5A, the wound healing assay demonstrated that the migration rate was significantly facilitated by UCK2 overexpression and was suppressed by siUCK2-1 and siUCK2-2 in HTR-8/SVneo cells. Furthermore, the results of the transwell assay suggested that the upregulation of UCK2 significantly facilitated the invasion ability of trophoblast cells. The silence of UCK2 remarkably reduced the number of invaded HTR-8/SVneo cells (Fig. 5B). Matrix metalloproteinases (MMPs) are reported to participate in the process of cell adhesion and invasion, and their tissue inhibitors (TIMPs) are involved in the regulation of cell migration and invasion through interaction with MMPs (Chen, 1992; Polette et al., 2004; Bhuvarahamurthy et al., 2006). Results shown in Fig. 5C suggest that the protein expression of MMP2 and MMP9 was significantly increased, and the TIMP1 and TIMP2 expressions were obviously declined in HTR-8/SVneo cells by the upregulation of UCK2. When UCK2 was knocked-down, the protein expression of MMP2, MMP9, TIMP1, and TIMP2 was opposite to that of the above situation. Based on these results, UCK2 was demonstrated to promote the migration and invasion of trophoblast cells.
Figure 5: Uridine-cytidine kinase 2 (UCK2) facilitates cell migration and invasion of trophoblast cells. (A) Wound healing assay was performed to measure cell migration. Scale bar = 200 μm. (B) The invasive ability of HTR-8/SVneo cells was examined by transwell assay. Scale bar = 100 μm. (C) The protein expression of matrix metalloproteinase (MMP)2, MMP9, TIMP1, and TIMP2 was tested by western blot. The data shown are mean ± standard deviation. ns means not significant. **p < 0.01.
Uridine-cytidine kinase 2 (UCK2) facilitates the proliferation, migration, and invasion of trophoblast cells via activating the signal transducer and activator of transcription 3 pathway
STAT3 is a crucial mediator participating in the normal biological function of trophoblast cells (Ko et al., 2013). As shown in Fig. 6A, when UCK2 was overexpressed, the phosphorylated STAT3 (p-STAT3) expression was obviously upregulated, while there was no significant change in STAT3 expression. When UCK2 was knocked-down, the p-STAT3 expression was significantly decreased. To further investigate whether UCK2 regulates the biological behavior of trophoblast cells via the STAT3 signaling pathway, the STAT3 antagonist stattic was used to treat the UCK2-overexpressed trophoblast cells. Results of Fig. 6B reveal that the upregulation of p-STAT3 expression induced by UCK2 was suppressed by stattic. The UCK2-induced cell proliferation was also inhibited by stattic (Fig. 6C). The promotion of UCK2 on cell migration was dramatically inhibited after the addition of stattic (Fig. 6D). Besides, UCK2-induced invasion of HTR-8/SVneo cells was clearly rescued by static (Fig. 6E). Therefore, UCK2 facilitated cell proliferation, migration, and invasion of trophoblast cells partly through the activation of the STAT3 pathway.
Figure 6: UCK2 regulates the biological function of the trophoblast cells through the signal transducer and activator of transcription 3 (STAT3 pathway). (A, B) The protein expression of STAT3 and p-STAT3 affected by UCK2 or stattic was examined by western blot. (C) MTT assay estimated cell proliferation. (D, E) The migration and invasion activities of trophoblast cells rescued by stattic were detected by wound healing (Scale bar = 200 μm) and transwell assays (Scale bar = 100 μm). The data shown are mean ± SD. ns means not significant. *p < 0.05, **p < 0.01.
The current study indicated low expression of UCK2 in PE placental tissues compared with the normal tissues. In L-NAME induced the PE mouse model, UCK2 expression was significantly reduced at the transcriptional and translational levels. Through the up- or down-regulation of UCK2 in trophoblast cells, we confirmed that UCK2 could promote cell proliferation, migration, and invasion and inhibit apoptosis. Further exploration demonstrated that the STAT3 signaling pathway mediated the regulation of UCK2 on the biological function of trophoblast cells.
The bioinformatics analysis of GEO datasets presented that UCK2 had low expression in the placental of PE patients. To verify the result in vivo, the PE mouse model with hypertension and proteinuria was established using L-NAME treatment. The low expression of UCK2 in vivo supported that UCK2 might be a potential target for the prevention and diagnosis of PE. During a normal pregnancy, trophoblast cells invade the spiral artery and alter it, establishing blood circulation between the mother and the placenta. Thus, the ability of trophoblast cells to invade the placenta is closely related to the successful flow of maternal blood to the placenta (Weiss et al., 2016). Insufficient blood supply to the placenta is an important cause of PE. Increasing number of studies indicate that the inadequate migration and invasion of trophoblast cells may lead to PE (Pijnenborg et al., 2008). Promoting the migration and invasion ability of trophoblast cells can be one of the methods to improve or treat PE. A previous report has indicated that miR-135 expression is decreased in PE patients, and its upregulation enhanced the migration and invasion ability of trophoblast cells through attenuating inflammation of PE (Zhao et al., 2021). We explored that this molecule plays a similar role to UCK2. In addition, the molecule LINC00922 showed higher expression in PE tissues. Knockdown of LINC00922 suppressed the migration and invasion of trophoblast cells (Gao et al., 2021). The present study demonstrated that UCK2 is upregulated in PE tissues. It promoted proliferation, migration, and invasion, and blocked the cell apoptosis of trophoblast cells. This finding might provide an approach to prevent the occurrence of PE effectively.
Till now, there is no report on the effects of UCK2 on trophoblast cells. The migration and invasion of trophoblast cells often share many of the same molecular mechanisms as tumor metastasis (Kohan-Ghadr et al., 2016). UCK2 has been studied as an important regulatory factor in tumor occurrence and development. In 2021, UCK2 was found to contribute to melanoma cancer cell migration (Wu et al., 2021), which was similar to our findings that UCK2 enhanced HTR-8/SVneo migration. A study on lung cancer suggested that cell proliferation and migration were suppressed by the downregulation of UCK2 (Wu et al., 2019). In our investigation, the knockdown of UCK2 blocked the proliferation and migration of trophoblast cells. Moreover, in compliance with our findings, previous literature confirmed that the migration and invasion of hepatocellular carcinoma cells were enhanced by UCK2 via activating the STAT3 pathway (Zhou et al., 2018). Collectively, similar to the role of UCK2 on human cancers, the current study verified that UCK2 could promote the proliferation, migration, and invasion of trophoblast cells in PE. In other words, UCK2 can serve as a potential target for the diagnosis and treatment of PE.
STAT3 has been identified as an oncogene when it was first discovered (Bromberg et al., 1999) and is involved in regulating various physiological pathways such as cell growth, differentiation, and apoptosis. It has an SH2 domain that specifically recognizes phosphorylated tyrosine residues in intercellular signal transduction and could be phosphorylated for its activation (Jin et al., 2015). Phosphorylated STAT3 quickly enters the nucleus and binds to the promoter of the target gene to activate transcription. As a key factor in maintaining the balance of the normal biological behavior of the cell, STAT3 can promote the proliferation of tumor cells when it is continuously activated (Wang and Sun, 2014; Furtek et al., 2016). In our research, STAT3 was found to mediate the regulation of UCK2 on the normal biological function of trophoblast cells. When UCK2 was upregulated, STAT3 was activated in HTR-8/SVneo cells. When UCK2 was downregulated, the expression of phosphorylated STAT3 was obviously reduced. Stattic, the inhibitor of STAT3, specifically blocked the SH2 domain of STAT3 (Schust et al., 2006). The cell proliferation, migration, and invasion promoted by the overexpression of UCK2 were rescued by the inhibition of STAT3. The STAT3 signaling pathway was demonstrated to mediate the effect of UCK2 on the biological function of trophoblast cells.
In conclusion, UCK2 is under-expressed in the placental tissues of the PE patients and the PE mouse model. The loss of UCK2 impaired the proliferation, migration, and invasion and induced cell apoptosis of the trophoblast cells. The effect of UCK2 on the normal biological function of trophoblast cells was at least partly mediated by the STAT3 signaling pathway. These findings indicated that UCK2 might serve as a promoter for the proliferation, migration, and invasion of trophoblast cells via activating the STAT3 signaling pathway. It might be a potential diagnostic target for PE in the future.
Funding Statement: This work was supported by “Heart Health Research Fund,” Special Fund for Cardiovascular Disease Development of China, China International Medical Foundation (Grant No. Z-2019-42-1908-3), Tianjin Key Medical Discipline (Specialty) Construction Project, and the Young Talents Training Program of Tianjin First Central Hospital.
Author Contributions: The authors confirm their contribution to the paper as follows: study conception, design, and draft manuscript preparation: Chengzhi Lu and Wei Xia; data collection and analysis: Ning Yang, Xiaoyan Feng, Ting Xin, Yongle Jing, and Yuming Li. All authors reviewed the results and approved the final version of the manuscript.
Availability of Data and Materials: The datasets generated during and/or analyzed during the current study are available from the corresponding author on reasonable request.
Ethics Approval: The mice used in this research and all experimental procedures were approved by the Institutional of Animal Care and Use Committee of Nankai University (No. 2022-SYDWLL-000273).
Conflicts of Interest: The authors declare that they have no conflicts of interest to report regarding the present study.
References
Bhuvarahamurthy V, Kristiansen GO, Johannsen M, Loening SA, Schnorr D, Jung K, Staack A (2006). In situ gene expression and localization of metalloproteinases MMP1, MMP2, MMP3, MMP9, and their inhibitors TIMP1 and TIMP2 in human renal cell carcinoma. Oncology Reports 15: 1379–1384. https://doi.org/10.3892/or.15.5.1379 [Google Scholar] [CrossRef]
Bromberg JF, Wrzeszczynska MH, Devgan G, Zhao Y, Pestell RG, Albanese C, Darnell JrJE (1999). Stat3 as an oncogene. Cell 98: 295–303. https://doi.org/10.1016/S0092-8674(00)81959-5 [Google Scholar] [PubMed] [CrossRef]
Cai J, Sun X, Guo H, Qu X, Huang H, Yu C, Wu H, Gao Y, Kong X, Xia Q (2020). Non-metabolic role of UCK2 links EGFR-AKT pathway activation to metastasis enhancement in hepatocellular carcinoma. Oncogenesis 9: 103. https://doi.org/10.1038/s41389-020-00287-7 [Google Scholar] [PubMed] [CrossRef]
Chen CW, Jaffe IZ, Karumanchi SA (2014). Pre-eclampsia and cardiovascular disease. Cardiovascular Research 101: 579–586. https://doi.org/10.1093/cvr/cvu018 [Google Scholar] [PubMed] [CrossRef]
Chen WT (1992). Membrane proteases: Roles in tissue remodeling and tumour invasion. Current Opinion in Cell Biology 4: 802–809. https://doi.org/10.1016/0955-0674(92)90103-J [Google Scholar] [PubMed] [CrossRef]
Darnell JEJr, Kerr IM, Stark GR (1994). Jak-STAT pathways and transcriptional activation in response to IFNs and other extracellular signaling proteins. Science 264: 1415–1421. https://doi.org/10.1126/science.8197455 [Google Scholar] [PubMed] [CrossRef]
Duley L (2009). The global impact of pre-eclampsia and eclampsia. Seminars in Perinatology 33: 130–137. https://doi.org/10.1053/j.semperi.2009.02.010 [Google Scholar] [PubMed] [CrossRef]
Furtek SL, Backos DS, Matheson CJ, Reigan P (2016). Strategies and approaches of targeting STAT3 for cancer treatment. ACS Chemical Biology 11: 308–318. https://doi.org/10.1021/acschembio.5b00945 [Google Scholar] [PubMed] [CrossRef]
Gao C, Yang H, Xia F (2021). Increased LINC00922 in preeclampsia regulates the proliferation, invasion, and migration of placental trophoblast cells. Annals of Translational Medicine 9: 1553. https://doi.org/10.21037/atm-21-4923 [Google Scholar] [PubMed] [CrossRef]
Hanlon MM, Rakovich T, Cunningham CC, Ansboro S, Veale DJ, Fearon U, McGarry T (2019). STAT3 mediates the differential effects of oncostatin M and TNFα on RA synovial fibroblast and endothelial cell function. Frontiers in Immunology 10: 2328. https://doi.org/10.3389/fimmu.2019.02056 [Google Scholar] [PubMed] [CrossRef]
Hauth JC, Ewell MG, Levine RJ, Esterlitz JR, Sibai B, Curet LB, Catalano PM, Morris CD (2000). Pregnancy outcomes in healthy nulliparas who developed hypertension. Obstetrics and Gynecology 95: 24–28. https://doi.org/10.1016/s0029-7844(99)00462-7 [Google Scholar] [PubMed] [CrossRef]
Ishibashi K, Koguchi T, Matsuoka K, Onagi A, Tanji R et al. (2018). Interleukin-6 induces drug resistance in renal cell carcinoma. Fukushima Journal of Medical Science 64: 103–110. https://doi.org/10.5387/fms.2018-15 [Google Scholar] [PubMed] [CrossRef]
Jin LL, Wybenga-Groot LE, Tong J, Taylor P, Minden MD, Trudel S, McGlade CJ, Moran MF (2015). Tyrosine phosphorylation of the Lyn Src homology 2 (SH2) domain modulates its binding affinity and specificity. Molecular & Cellular Proteomics 14: 695–706. https://doi.org/10.1074/mcp.M114.044404 [Google Scholar] [PubMed] [CrossRef]
Johnson DE, O’Keefe RA, Grandis JR (2018). Targeting the IL-6/JAK/STAT3 signalling axis in cancer. Nature Reviews Clinical Oncology 15: 234–248. https://doi.org/10.1038/nrclinonc.2018.8 [Google Scholar] [PubMed] [CrossRef]
Ko HS, Choi SK, Kang HK, Kim HS, Jeon JH, Park IY, Shin JC (2013). Oncostatin M stimulates cell migration and proliferation by down-regulating E-cadherin in HTR8/SVneo cell line through STAT3 activation. Reproductive Biology and Endocrinology 11: 93. https://doi.org/10.1186/1477-7827-11-93 [Google Scholar] [PubMed] [CrossRef]
Kohan-Ghadr HR, Kadam L, Jain C, Armant DR, Drewlo S (2016). Potential role of epigenetic mechanisms in regulation of trophoblast differentiation, migration, and invasion in the human placenta. Cell Adhesion & Migration 10: 126–135. https://doi.org/10.1080/19336918.2015.1098800 [Google Scholar] [PubMed] [CrossRef]
Lin S, Shimizu I, Suehara N, Nakayama M, Aono T (1995). Uterine artery Doppler velocimetry in relation to trophoblast migration into the myometrium of the placental bed. Obstetrics and Gynecology 85: 760–765. https://doi.org/10.1016/0029-7844(95)00020-R [Google Scholar] [PubMed] [CrossRef]
Medina-Bastidas D, Guzmán-Huerta M, Borboa-Olivares H, Ruiz-Cruz C, Parra-Hernández S, Flores-Pliego A, Salido-Guadarrama I, Camargo-Marín L, Arambula-Meraz E, Estrada-Gutierrez G (2020). Placental microarray profiling reveals common mRNA and lncRNA expression patterns in preeclampsia and intrauterine growth restriction. International Journal of Molecular Sciences 21: 3597. https://doi.org/10.3390/ijms21103597 [Google Scholar] [PubMed] [CrossRef]
Mol BWJ, Roberts CT, Thangaratinam S, Magee LA, de Groot CJM, Hofmeyr GJ (2016). Pre-eclampsia. Lancet 387: 999–1011. https://doi.org/10.1016/S0140-6736(15)00070-7 [Google Scholar] [PubMed] [CrossRef]
Murray EJ, Gumusoglu SB, Santillan DA, Santillan MK (2021). Manipulating CD4+ T cell pathways to prevent preeclampsia. Frontiers in Bioengineering and Biotechnology 9: 811417. https://doi.org/10.3389/fbioe.2021.811417 [Google Scholar] [PubMed] [CrossRef]
Neerukonda S, Shariati F, Hart T, Stewart M, Elkayam U, Qamruddin S (2020). Cardiovascular effects of preeclampsia. Current Opinion in Cardiology 35: 357–359. https://doi.org/10.1097/HCO.0000000000000756 [Google Scholar] [PubMed] [CrossRef]
North RA, Ferrier C, Long D, Townend K, Kincaid-Smith P (1994). Uterine artery doppler flow velocity waveforms in the second trimester for the prediction of preeclampsia and fetal growth retardation. Obstetrics and Gynecology 83: 378–386. [Google Scholar] [PubMed]
Paauw ND, Lely AT (2018). Cardiovascular sequels during and after preeclampsia. Advances in Experimental Medicine and Biology 1065: 455–470. https://doi.org/10.1007/978-3-319-77932-4 [Google Scholar] [CrossRef]
Pijnenborg R, Vercruysse L, Hanssens M (2008). Fetal-maternal conflict, trophoblast invasion, preeclampsia, and the red queen. Hypertension in Pregnancy 27: 183–196. https://doi.org/10.1080/10641950701826711 [Google Scholar] [PubMed] [CrossRef]
Polette M, Nawrocki-Raby B, Gilles C, Clavel C, Birembaut P (2004). Tumour invasion and matrix metalloproteinases. Critical Reviews in Oncology/Hematology 49: 179–186. https://doi.org/10.1016/j.critrevonc.2003.10.008 [Google Scholar] [PubMed] [CrossRef]
Priego N, Zhu L, Monteiro C, Mulders M, Wasilewski D et al. (2018). STAT3 labels a subpopulation of reactive astrocytes required for brain metastasis. Nature Medicine 24: 1024–1035. https://doi.org/10.1038/s41591-018-0044-4 [Google Scholar] [PubMed] [CrossRef]
Rawlings JS, Rosler KM, Harrison DA (2004). The JAK/STAT signaling pathway. Journal of Cell Science 117: 1281–1283. https://doi.org/10.1242/jcs.00963 [Google Scholar] [PubMed] [CrossRef]
Schumacher FR, Wang Z, Skotheim RI, Koster R, Chung CC et al. (2013). Testicular germ cell tumor susceptibility associated with the UCK2 locus on chromosome 1q23. Human Molecular Genetics 22: 2748–2753. https://doi.org/10.1093/hmg/ddt109 [Google Scholar] [PubMed] [CrossRef]
Schust J, Sperl B, Hollis A, Mayer TU, Berg T (2006). Stattic: A small-molecule inhibitor of STAT3 activation and dimerization. Chemistry & Biology 13: 1235–1242. https://doi.org/10.1016/j.chembiol.2006.09.018 [Google Scholar] [PubMed] [CrossRef]
Sherman BT, Hao M, Qiu J, Jiao X, Baseler MW, Lane HC, Imamichi T, Chang W (2022). DAVID: A web server for functional enrichment analysis and functional annotation of gene lists (2021 update). Nucleic Acids Research 50: W216–W221. https://doi.org/10.1093/nar/gkac194 [Google Scholar] [PubMed] [CrossRef]
Sibai BM (2003). Diagnosis and management of gestational hypertension and preeclampsia. Obstetrics and Gynecology 102: 181–192. https://doi.org/10.1016/s0029-7844(03)00475-7 [Google Scholar] [PubMed] [CrossRef]
Smyth A, Ronco C, Garovic VD (2017). Preeclampsia: A cardiorenal syndrome in pregnancy. Current Hypertension Reports 19: 15. https://doi.org/10.1007/s11906-017-0714-5 [Google Scholar] [PubMed] [CrossRef]
Staff AC, Fjeldstad HE, Fosheim IK, Moe K, Turowski G, Johnsen GM, Alnaes-Katjavivi P, Sugulle M (2020). Failure of physiological transformation and spiral artery atherosis: Their roles in preeclampsia. American Journal of Obstetrics and Gynecology 226: S895–S906 https://doi.org/10.1016/j.ajog.2020.09.026 [Google Scholar] [PubMed] [CrossRef]
Stefańska K, Zieliński M, Zamkowska D, Adamski P, Jassem-Bobowicz J et al. (2020). Comparisons of dipstick test, urine protein-to-creatine ratio, and total protein measurement for the diagnosis of preeclampsia. International Journal of Environmental Research and Public Health 17: 4195. https://doi.org/10.3390/ijerph17124195 [Google Scholar] [PubMed] [CrossRef]
Turco MY, Moffett A (2019). Development of the human placenta. Development 146: 20170131. https://doi.org/10.1242/dev.163428 [Google Scholar] [PubMed] [CrossRef]
Van Rompay AR, Norda A, Lindén K, Johansson M, Karlsson A (2001). Phosphorylation of uridine and cytidine nucleoside analogs by two human uridine-cytidine kinases. Molecular Pharmacology 59: 1181–1186. https://doi.org/10.1124/mol.59.5.1181 [Google Scholar] [PubMed] [CrossRef]
Wang SW, Sun YM (2014). The IL-6/JAK/STAT3 pathway: Potential therapeutic strategies in treating colorectal cancer (Review). International Journal of Oncology 44: 1032–1040. https://doi.org/10.3892/ijo.2014.2259 [Google Scholar] [PubMed] [CrossRef]
Weiss G, Huppertz B, Siwetz M, Lang I, Moser G (2016). Arterial endothelial cytokines guide extravillous trophoblast invasion towards spiral arteries; an in-vitro study with the trophoblast cell line ACH-3P and female non-uterine endothelial cells. Placenta 38: 49–56. https://doi.org/10.1016/j.placenta.2015.12.010 [Google Scholar] [PubMed] [CrossRef]
Wu H, Xu H, Jia D, Li T, Xia L (2021). METTL3-induced UCK2 m6A hypermethylation promotes melanoma cancer cell metastasis via the WNT/β-catenin pathway. Annals of Translational Medicine 9: 1155. https://doi.org/10.21037/atm-21-2906 [Google Scholar] [PubMed] [CrossRef]
Wu Y, Jamal M, Xie T, Sun J, Song T et al. (2019). Uridine-cytidine kinase 2 (UCK2A potential diagnostic and prognostic biomarker for lung cancer. Cancer Science 110: 2734–2747. https://doi.org/10.1111/cas.14125 [Google Scholar] [PubMed] [CrossRef]
Yu S, Li X, Guo X, Zhang H, Qin R, Wang M (2019). UCK2 upregulation might serve as an indicator of unfavorable prognosis of hepatocellular carcinoma. IUBMB Life 71: 105–112. https://doi.org/10.1002/iub.1941 [Google Scholar] [PubMed] [CrossRef]
Yue P, Turkson J (2009). Targeting STAT3 in cancer: How successful are we? Expert Opinion on Investigational Drugs 18: 45–56. https://doi.org/10.1517/13543780802565791 [Google Scholar] [PubMed] [CrossRef]
Zhao X, Zhang X, Wu Z, Mei J, Li L, Wang Y (2021). Up-regulation of microRNA-135 or silencing of PCSK6 attenuates inflammatory response in preeclampsia by restricting NLRP3 inflammasome. Molecular Medicine 27: 82. https://doi.org/10.1186/s10020-021-00335-x [Google Scholar] [PubMed] [CrossRef]
Zhou Q, Jiang H, Zhang J, Yu W, Zhou Z, Huang P, Wang J, Xiao Z (2018). Uridine-cytidine kinase 2 promotes metastasis of hepatocellular carcinoma cells via the Stat3 pathway. Cancer Management and Research 10: 6339–6355. https://doi.org/10.2147/CMAR [Google Scholar] [CrossRef]
Cite This Article
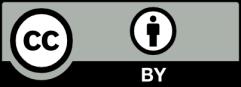
This work is licensed under a Creative Commons Attribution 4.0 International License , which permits unrestricted use, distribution, and reproduction in any medium, provided the original work is properly cited.