Open Access
REVIEW
How is the AKT/mTOR pathway involved in cell migration and invasion?
1 The Sperm Laboratory, College of Life Sciences, Zhejiang University, Hangzhou, 310058, China
2 Department of Pathology, School of Medicine, Zhejiang University, Hangzhou, 310058, China
* Corresponding Authors: WANXI YANG. Email: ; HONG DENG. Email:
# Authors contributed equally to this work
BIOCELL 2023, 47(4), 773-788. https://doi.org/10.32604/biocell.2023.026618
Received 16 September 2022; Accepted 28 November 2022; Issue published 08 March 2023
Abstract
As a pathway that plays a role in nutrient absorption, anabolic response, cell growth and survival, the important role of AKT/mTOR in tumorigenesis has also come to light. For cancer patients, most deaths are caused by the growth of metastatic tumors outside the primary focus. Therefore, migration and invasion in the late stage of tumor progression are the main unresolved issues in the study of tumor pathogenesis, and AKT/mTOR has been found to participate in the migration and invasion of cancer cells, which means that the study of this pathway may contribute to a solution for the problem. Because of its extensive and complex functions in the organism, this pathway can be regulated by a variety of different signals in the body, and then realize its function through different downstream signal molecules. This article reviews the proteins that can indirectly affect this pathway by regulating the common upstream signaling molecules of this pathway, and the proteins that can directly affect the level of phosphorylation of AKT/mTOR in cancer cells. We also review the proteins that can co-regulate this pathway and its downstream pathways. Through this study, we hope to gain a deeper understanding of the regulatory mechanism of the AKT/mTOR pathway in cancer cells, in hopes of finding effective and harmless cancer treatment targets in the future.Keywords
The AKT/mTOR pathway has been discovered to be activated in various cancers and is also considered a very good therapeutic target (Shorning et al., 2020; Fattahi et al., 2020; Ediriweera et al., 2019).
Protein kinase B (PKB), also known as AKT, is a serine/threonine-specific protein kinase, which in mammals, is mainly comprised of three isoforms, including AKT1, AKT2, and AKT3, that are all encoded by different genes (Toker and Marmiroli, 2014). AKT1 is widely expressed in various tissues, AKT2 is mainly expressed within the liver, skeletal muscle, and adipose tissue, whereas the expression of AKT3 is limited to the heart, kidney, brain, testis, lung, and skeletal muscle (Aoki and Fujishita, 2017). There are three main domains in AKT: the pleckstrin homology (PH) domain is rich in affinity for phosphatidylinositol bisphosphate (PIP2) and phosphatidylinositol 3,4,5-trisphosphate (PIP3), which is essential for their association with cellular membranes, and the Thr308 and Ter473 are two common phospho-activating sites of AKT, which are respectively located in the catalytic domain and regulatory domain (Datta et al., 1999). Moreover, the maintenance of the inactive state of AKT cannot do without the interaction between the catalytic and PH domains (Toker and Marmiroli, 2014).
As one of the classical signaling pathways, the role of the AKT/mTOR pathway is self-evident. It plays a significant physiological role in the process of cell survival, proliferation, growth, apoptosis and so on. The regulation of AKT is also extremely extensive, as it will be regulated by many upstream factors including phosphoinositide 3-kinase (PI3K) and further regulate its downstream targets.
The mammalian target of rapamycin (mTOR) is an atypical Ser/Thr protein kinase that can be activated by AKT as a downstream target. When bound to other proteins, mTOR can form two different protein complexes: mTOR complex 1 (mTORC1) and mTORC2. The MTORC1 consists of mTOR, regulatory-associated protein of mTOR (RAPTOR), mammalian lethal with SEC13 protein 8 (mLST8), the non-core component 40-kDa proline-rich AKT substrate (PRAS40) and DEP domain-containing mTOR-interacting protein (DEPTOR) (Murugan, 2019). Because AKT can phosphorylate PRAS40 at the Ser246 site, which in turn releases RAPTOR that is inhibited by PRAS40 (Sancak et al., 2007), the mTORC1 is activated and then leads to the phosphorylation of S6 Kinase 1 (S6K1) and 4E (eIF4E)- binding protein 1 (4E-BP1), which are essential for cell proliferation, survival, autophagy inhibition, invasion, and metastasis. The MTORC2 is composed of mTOR, rapamycin-insensitive companion of mTOR (RICTOR), DEPTOR, Protor-1, mLST8, and mammalian stress-activated protein kinase-interacting protein (mSIN1) (Murugan, 2019). Unlike mTORC1, the downstream effectors of mTORC2 are a series of cytoskeletal regulators that function primarily in the actin cytoskeleton, cell survival, and metabolism.
An increasing number of experiments have indicated that the activation of the AKT/mTOR pathway is highly related to the invasive and migratory abilities of cancer cells (Han et al., 2019; Shu et al., 2021; Xu et al., 2020b). In parallel, an increasing number of proteins have been found to influence cancer cell invasion and migration by regulating the AKT/mTOR pathway. Starting from the AKT/mTOR pathway, this paper will review the proteins that can promote or inhibit this pathway from upstream and then affect cancer cell migration, and explore the impact of the interaction between this pathway and other pathways on cancer cell migration, to answer the pertinent questions: which proteins can regulate the AKT/mTOR signaling pathway? How is their regulation realized? What is the impact of the modulation of this pathway on cancer cell invasion and migration? In the meantime, this paper also explores potential cancer treatment targets through the review of upstream regulatory proteins of the AKT/mTOR signaling pathway, to provide some ideas for cancer control and treatment in the future.
Proteins that Can Activate the AKT/mTOR
Fig. 1 shows the proteins that can activate the AKT/mTOR pathway in the upstream and their regulatory modes. The proteins mainly regulate the common upstream signaling molecules PI3K, FAK, EGFR and PTEN of AKT/mTOR to activate this pathway. The AFP and PTEN molecules co-localize in the cytoplasm, which can inhibit PTEN activity and affect the level of phosphorylation of PI3K. Mettl3 can control m6A modification to affect mRNA biosynthesis. Meanwhile, mir-125-6p, as a substance that can directly bind to PTEN and inhibit its function, cannot mature without m6A modification. Sall4 can bind to the promoter site of the PTEN gene and deacetylate histones in the binding region, thereby inhibiting PTEN expression. Fut7 can catalyze the formation of sLex, a substance that can modify EGFR glycoprotein and affect its phosphorylation. Golm1 can promote downstream signal transduction by interacting with EGFR/RTK. For intracellular EGFR and p-EGFR, the presence of EGF can induce their degradation, and TPO can act as an EGFR stabilizer to slow down the degradation process. ET-1 can increase the phosphorylation of Tyr397 site of FAK after binding to its high-affinity receptor ETAR.
Figure 1: Positive regulation of AKT/mTOR.
Proteins that inhibit the upstream regulatory factor PTEN to activate AKT/mTOR
The Phosphatase and tensin homolog (PTEN) gene was found to be a tumor suppressor closely related to tumorigenesis, and can encode the PTEN protein, which is composed of 403 amino acids. The PTEN protein, which has both lipid and protein phosphatase activity, has also been found to suppress tumorigenesis by directly dephosphorylating AKT in addition to inhibiting the activity of phosphorylated enzymes, such as receptor tyrosine kinases (Bu et al., 2021). As mentioned earlier, PIP3 binds with high affinity to the PH domain of AKT and can mediate its activation. At the same time, PIP3 is also an important substrate of PTEN, and under the action of PTEN, PIP3 will dephosphorylate, so that the concentration of PIP3 is maintained at a low level, hindering the activation of AKT (Maehama and Dixon, 1998). It has also been documented that PTEN can directly interact with AKT to dephosphorylate AKT1 at the Ser473 and Thr308 sites, which in turn acts to suppress tumor development (Bu et al., 2021). Therefore, when the expression or activity of PTEN in cells is inhibited, its inhibitory effect on the AKT/mTOR pathway will also be attenuated, leading to the activation of AKT/mTOR and its downstream signals.
Activated Cdc42-associated kinase 1 (ACK1) is mainly located in the cytoplasm and can catalyze the phosphorylation of tyrosine residues of substrate proteins. As Tyr284 is an autophosphorylation site of ACK1, activated ACK1 can downregulate the expression of PTEN and phosphorylate AKT at the Tyr176 site so that it is recruited to the cell membrane, and then AKT will be fully activated with the phosphorylation of the Ser473 and Thr308 sites, to promote the migration of cancer cells and other behaviors (Wang et al., 2020d).
Tripartite motif-containing 29 (TRIM29), as a part of the TRIM family, does not have a RING domain, suggesting that it lacks E3 ubiquitin ligase activity, but overexpression of this protein has been found in a variety of cancer patients. Thus, it has been found that TRIM29 can indeed promote cancer cell invasion and migration, and its overexpression will significantly downregulate the expression of PTEN protein, thereby increasing the level of phosphorylation AKT to activate the AKT pathway, while phosphoprotein 70 ribosomal protein S6 kinase (P70S6K) and 4EBP1, which are downstream of the pathway, are observed to increase in the level of phosphorylation as well (Zhou et al., 2016).
The DJ-1 protein is one of the members of the protein family belonging to peptidase C56 encoded by the Parkinson disease protein 7 (PARK7) gene, and previous studies have mostly associated it with Parkinson’s disease, but in fact, the protein is similarly closely related to tumors. An overexpression of DJ-1 in SNU-46 cells revealed an increase in the phosphorylation of both AKT and mTOR to some extent, along with an observed decrease in PTEN protein expression, leading to the speculation that the influence of DJ-1 on the invasive and migratory abilities of cancer cells might be mediated by affecting the AKT/mTOR pathway through the negative regulation of PTEN (Wang et al., 2014b).
A-kinase anchor protein 3 (AKAP3), a CTA family member essential for tumorigenesis, is specifically expressed in the testes of humans as well as other mammals. Upregulation of AKAP3 was found in breast cancer tissue along with its increased malignant phenotypes such as growth, proliferation, migration, and invasion. Follow-up experiments revealed that it was able to downregulate PTEN expression and activate the AKT/mTOR signaling pathway. In addition, a rise in epidermal growth factor receptor (EGFR) expression with a fall in p53 expression was also found in AKAP3 overexpressing cells (Zhan et al., 2022).
Alpha fetoprotein (AFP) is well recognized as a key regulator of cell proliferation in hepatocellular carcinoma, but apart from that, it plays a large role in inhibiting autophagy, apoptosis and enhancing cell migration, invasion, etc. Colocalization of AFP with PTEN in the cytoplasm was visualized by laser scanning confocal microscopy, which illustrated their interaction. Moreover, the levels of phosphorylation of intracellular AKT, PI3K and mTOR were markedly decreased when AFP was knocked down. From this, it can be speculated that the interaction between AFP and PTEN can inhibit the activity of PTEN, thus activating the pathway that is inhibited by PTEN, which in turn affects the behavior of cancer cells (Wang et al., 2018).
Methyltransferase like 3 (METTL3) is an RNA methyltransferase, and it can regulate the modification of N6-methyladenosine (m6A), which will affect the biosynthesis of mRNA. Meanwhile, microRNAs (miRNAs) have also been found to play an enormous part in several diseases, and Mir-126-5p was found to directly bind to PTEN and inhibit its function to activate downstream pathways. For Mir-126-5p, its maturation requires m6A modification of its precursor, which means that the maturation of Mir-126-5p cannot occur without METTL3. Therefore, METTL3 could inhibit the expression of PTEN and increase the levels of PI3K, p-AKT, and p-4EBP1 through the modified regulation of Mir-126-5p, thus promoting the development of tumors, which has also been demonstrated in ovarian cancer-related experiments (Bi et al., 2021).
Spalt like transcription factor 4 (SALL4) is responsible for regulating the self-renewal of embryonic stem cells and has a critical effect on many tumors. In experiments, it is found that its expression will be controlled by Mir-188-5p. Similar to microRNAs, but different from Mir-126-5p, Mir-188-5p achieves its effect on cancer cell migration by regulating SALL4. When Mir-188-5p is overexpressed, the expression of SALL4 protein increases, and then SALL4 will translocate into the nucleus (Wang et al., 2019a). It has been previously documented that SALL4 can bind to the promoter site of the PTEN gene and deacetylate histones within the binding region to inhibit PTEN expression (Lu et al., 2009). This demonstrates that Mir-188-5p can play a negative regulatory role on PTEN and then activate the AKT/mTOR pathway to promote cancer cell migration by upregulating SALL4 expression.
Proteins that upregulate the EGFR to activate AKT/mTOR
The epidermal growth factor receptor (EGFR) is a glycoprotein that belongs to the tyrosine kinase type receptors, and it is often located on cell membrane. When binding to its ligands, EGFR monomers will bond into dimers, and autophosphorylation of tyrosine residues at different sites occurs, which can lead to different downstream phosphorylation and subsequent activation of the corresponding kinase pathways in the cell (Normanno et al., 2006). In the AKT/mTOR pathway, when EGFR is activated by ligands, the adaptor protein growth factor receptor-bound protein 2 (GRB2)-associated binder-1 (Gab1) is phosphorylated, and the specific tyrosine at the C-terminal of Gab1 can bind to p85 of the PI3K regulatory subunit, which enables substantial recruitment of PI3K around Gab1, while its N-terminal PH domain can bind PIP3 at the cell membrane, and then enlarge the signal transmission (Rodrigues et al., 2000). EGFR can also activate PI3K after forming a dimer with Human epidermal growth factor receptor 3 (HER3) in the absence of Gab1, and the activated PI3K further catalyzes the generation of PIP3 at the membrane. Downstream AKT is thereafter activated upon binding of PIP3, which in turn activates the downstream target factors through phosphorylation, promoting multiple malignant behaviors of tumors.
Claudin-6 (CLDN6), a member of the CLDN family, acts as an intercellular adhesion molecule, which forms tight junctions around the cell and regulates the permeability of the epidermal layer, thereby preventing free passage of solutes and water through the cell. It is well established that loss of intercellular adhesion is critical for the migration of cancer cells, and this phenotype is often associated with altered tight junction protein expression. It has been studied and found that the protein is not detectably expressed in normal human tissues, but its abnormally high expression has been observed in various malignancies. When CLDN6 was silenced intracellularly, a significant reduction in the expression of EGFR, p-EGFR, p-AKT and p-mTOR was observed, as well as a significant reduction in the ratios of p-EGFR to EGFR, p-AKT to AKT, and p-mTOR to mTOR, indicating that the activation of the AKT/mTOR pathway was inhibited, while the reduction in cell proliferation, migration and invasion was also observed (Huang et al., 2020).
Annexin A8 (ANXA8) is a member of the annexin family, which has extensive physiological functions, including diverse cancer cell behaviors. After ANXA8 was silenced for a period, the expression of EGFR in cells decreased, so as the degree of phosphorylation of its downstream targets, including PI3K, AKT, mTOR, 4EBP1 and P70S6K. Also, a decrease in cell viability and migration ability can be observed (Zhou et al., 2021).
Fucosyltransferase VII (Fut7) belongs to a family of a1, 3-fucosyltransferases, it can catalyze the final step of Lewis antigen synthesis by fucosylation to yield the special glycosylation product, which is called the sialyl Lewis X (sLex). By acting as a ligand of cell surface E- or P-selectin, sLex mediates the adhesion of malignant cells, as well as contributes to cancer metastasis vs. angiogenesis. Experiments have indicated that the modification of EGFR glycoprotein by sLex may be associated with the EGFR phosphorylation and can effectively promote the activation of the AKT/mTOR pathway, although the detailed regulatory mechanism of sLex for EGFR is still not clear. It is also known that FUT7 can promote the activation of EGFR by mediating the synthesis of sLex and then activating the AKT/mTOR pathway, causing effects on the ability of cancer cells to invade and migrate (Liang et al., 2017).
Thrombopoietin (TPO), a hematopoietic cytokine mainly produced by the liver and kidney, can stimulate the production and maturation of megakaryocytes. Exogenous TPO fails to cause effects on tumor progression, and its expression is not observed or expressed in extremely low amounts in most tumor tissues and cell lines. However, there is high expression of TPO in most non-small cell lung cancer (NSCLC) cell lines, existing as an endogenous substance, without being secreted outward. When TPO was inhibited, there was a concomitant significant decrease in p-EGFR levels, which in turn affected changes in p-AKT (ser473) and p-mTOR (ser2448) levels. Indeed, intracellular EGF can induce the degradation of EGFR and p-EGFR, but the presence of TPO retards this process, thereby enhancing the stability of EGFR and increasing the duration and intensity of its signaling, which means that TPO is a new regulator of EGFR endocytic trafficking and degradation. Notably, the effects of TPO on cell proliferation and migration are cancer-specific and do not contribute to normal cells (Zou et al., 2020).
N-Acetylgalactosaminyltransferase 2 (GALNT2) can regulate the first step of mucin O-glycosylation, and aberrant glycosylation can affect a variety of cellular properties, including cell migration and invasion. It was experimentally found that GALNT2 can promote the binding of Vicia Villosa Lectin to EGFR, which can affect the O-glycosylation of EGFR and achieve the purpose of increasing the level of phosphorylation of EGFR, while the synchronous changes of p-AKT and p-mTOR were also observed (Sun et al., 2019).
Ubiquitin-conjugating enzyme E2 Q1 (UBE2Q1) belongs to the E2 ubiquitin-conjugating enzyme family, and can interact with ubiquitin-activating enzyme E1 and ubiquitin-protein ligase E3. It is at the heart of the ubiquitin cascade that can coordinately recognize and ubiquitinate specific targets, thereby facilitating the degradation of targets by the proteasome. β-catenin similarly plays a major role in cancer cells. Through experiments, the silence of UBE2Q1 was found to decrease the level of active β-Catenin. As β-Catenin’s direct target, the activity of EGFR tends to decrease with β-catenin activity and affects the phosphorylation levels of downstream PI3K, AKT, mTOR, 4EBP1, and p70S6K (Zhang et al., 2018a).
Golgi membrane protein 1 (GOLM1) is a 73 kDa CIS Golgi localized transmembrane glycoprotein, and although it is normally confined within the Golgi apparatus, it can likewise be secreted out of the cell via exosomes or microvesicles, and its high expression has been repeatedly detected in many cancer cases. The most recent studies have also shown that GOLM1 can promote the transduction of its downstream signals by interacting with EGFR/RTK, including PI3K (P110 α), p-AKT (Ser473), and p-mTOR (Ser2448), which were all observed to be upregulated in cells with GOLM1 overexpression (Yan et al., 2018).
Proteins that upregulate the FAK to activate AKT/mTOR
Focal adhesion kinase (FAK) is a non-receptor tyrosine kinase, and it belongs to the protein tyrosine kinase superfamily. FAK consists of a central kinase domain, an N-terminal protein 4.1-ezrin-radixin-moesin (FERM) domain and a C-terminal domain containing focal adhesion targeting (FAT) sequences (Zhao and Guan, 2011). Upon receiving a signal from the integrin or fibronectin, FAK undergoes autophosphorylation at Tyr397, and this will create a site with high affinity facilitating binding by the Src homology 2 (SH2) domain of the Src family kinases and further promoting Src recruitment and activation by forming a bipartite kinase complex (Parsons, 2003). Not only the Src, but also its autophosphorylation at Tyr397 can similarly promote the recruitment of proteins that contain the SH2 domain, with PI3K being one of them. FAK can directly bind to the SH2 domain of PI3K and activate PI3K, which in turn activates AKT, and regulates cell proliferation, migration, and invasion through mTOR and its downstream pathways (Zhao and Guan, 2011; Parsons, 2003).
Galectin-1 (GAL1) is a β Galactoside binding protein, which can participate in multiple aspects of tumorigenesis, its elevated expression in tumor tissue has also been repeatedly reported. GAL1 was found to regulate the phosphorylation of downstream FAK, PI3K, AKT and mTOR, also, its overexpression or silence all affected the invasion and migration of cells, presumably by the FAK/PI3K/AKT/mTOR pathway (Su et al., 2020).
Endothelin-1 (ET-1), one of the vasoactive peptides, is currently the most potent vasoconstrictor. The effects of ET are exerted by binding to two different receptors, endothelin-1 type A receptor (ETAR) and ETBR, both distributed on the cell surface. For ET-1, ETAR binds with higher affinity and their binding increases the phosphorylation of the Tyr397 site of FAK, which promotes matrix metalloproteinase-13 (MMP-13) expression and mediates the enhancement of invasion and migration through the PI3K/AKT/mTOR pathway (Wu et al., 2012).
The proteins that can influence the downstream epithelial–mesenchymal transition (EMT) process as well as the degradation of extracellular matrix (ECM) through the regulation of AKT/mTOR and how the regulation is achieved are shown in Fig. 2. E-cadherin, N-cadherin as well as vimentin are located on the cell surface, and when the AKT/mTOR pathway is activated, the downstream S6K can be phosphorylated to activate EMT transcription factors and thus allow the cell to acquire the ability of invasion, in which process, the elevated expression of N-cadherin and vimentin is obviously seen along with the decreased expression of E-cadherin. The MMP-2 and MMP-9 proteins are the only proteins of the MMP family capable of degrading ECM. Their secretion and activation are critical for ECM destruction and remodeling, again concerning the invasive capacity of tumor cells. When the AKT/mTOR pathway is activated, the expression of MMP-2 and MMP-9, which are in its downstream, will also be elevated, and thereafter, the MMPs that are secreted into the extracellular would be activated and degrade the ECM, promoting cell migration and invasion. Of the proteins shown in the figure, the proteins that are labeled in pink can regulate the downstream EMT process, and the proteins labeled in green can regulate the downstream MMP-2 or MMP-9.
Figure 2: Regulation of the AKT/mTOR downstream: EMT process and ECM degradation.
Proteins that activate the AKT/mTOR directly to promote the process of EMT
During the process of cancer cell migration, there is an essential biological process called epithelial mesenchymal transition (EMT), a process that converts epithelial cells into cells with a mesenchymal phenotype. Under the action of specific programs, the cell polarity of epithelial cells will be progressively lost, meanwhile, other epithelial phenotypes, including junctions with the basement membrane, will also be lost. For cancer cells, turning on the EMT process and acquiring a mesenchymal cell phenotype are key to enhancing their motility and migratory ability. In this process, with the transmission of upstream signals, EMT transcription factors (including Snail1, Snail2, Twist1, Twist2, ZEB1 and ZEB2) will be activated and function to regulate EMT. Among these, Snail and ZEB can repress E-cadherin transcription through their binding to E-box sequences located in the promoter. In addition, an increase or decrease in the expression of several proteins, such as N-cadherin and vimentin, which play roles in cell migration, will occur during this process, and these changes are now frequently used as markers indicating the occurrence of EMT (Yeung and Yang, 2017; Mittal, 2018).
Although the EMT process exists during normal biological development as well as tissue injury repair, epithelial cell-derived tumor cells acquire the ability to metastasize distally after undergoing EMT, which can promote tumor migration and cause distress for the treatment of cancer patients. Studies have shown that a variety of signals can trigger the EMT process, including growth factors, tumor cell stromal cell-cell interactions, microRNAs, tumor microenvironment, and others.
Although in different cancer cells AKT can modulate the expression of a variety of different transcription factors to regulate EMT, the activation of AKT has been proven to phosphorylate and activate the transcription factors of EMT. As one of the downstream activities of AKT, activation of mTOR has similarly been observed to induce EMT, notably, mTORC2 can directly phosphorylate the Ser473 site of AKT to induce EMT, and now mTORC2 is considered as a potential target to inhibit tumor migration (Karimi Roshan et al., 2019).
Many proteins have been found to activate the AKT/mTOR pathway directly, also, the expression of epithelial markers and mesenchymal markers will change during EMT, in parallel with the observed changes in cell invasion and migration. Given that the regulation by these proteins and the subsequent changes are all the same in essentials while differing in minor points, the individual proteins will not be described in detail here, and the specific proteins and how they are regulated are presented in Table 1.
Proteins that promote the MMPs through AKT/mTOR to promote cell migration and invasion
Matrix metalloproteinases (MMPs) are a group of zinc ion-dependent endopeptidases that are capable of degrading the extracellular matrix. Within malignant tumors, it is mainly secreted by stromal cells in the form of inactive zymogens, and when activated, it can mediate the cell degradation of ECM, control tumor neovascularization, and regulate cell adhesion and motility by affecting intracellular signaling. Adhesion between cancer cells and the extracellular matrix is indispensable for the invasive process, if the adhesion is too tight the cells cannot separate and move, and if the adhesion is too weak there is a consequent decrease in mobility, thus suitable adhesion and de-adhesion ability is important for tumor cells. MMP-2 and MMP-9 belong to the type IV collagenases and are the only two matrix proteinases in the MMP family capable of degrading type IV collagen, as the ECM is composed of the basic skeleton type IV collagen as well as other protein and polysaccharide components and is in a continuous dynamic balance of disruption and remodeling, so tumor cells have to tightly regulate MMP-2 and MMP-9 to degrade the ECM several times for the purpose of invasion and metastasis (Orlichenko and Radisky, 2008; Sternlicht and Werb, 2001; Ray and Stetler-Stevenson, 1994).
Different studies indicate that many proteins can affect the secretion as well as activation of MMP-2 and MMP-9 in cancer cells through regulation of the AKT/mTOR pathway to achieve an effect on the invasive and migratory ability of cancer cells. The specific mechanism of how these proteins regulate MMP-2 and MMP-9 through AKT/mTOR is not clear, but it is certain that they can influence cancer cell migration and invasion, and this effect cannot be achieved without the interaction between the AKT/mTOR and MMP proteins.
Proteins that Would Inhibit the AKT/mTOR
The foregoing demonstrates some of the upstream regulators associated with AKT/mTOR signaling as well as the downstream responders. It has been found that different proteins in cells can regulate the activation of pathways by regulating the expression of common regulators of AKT/mTOR, and also many proteins can directly regulate the levels of phosphorylation of AKT, mTOR and other substances. Changes in the downstream factors related to invasion and migration, like EMT transcription factors and MMPs, can be observed during this process, as well as the behaviors of invasion and migration of different cancer cells after treatment.
Unlike proteins with activating effects on the AKT/mTOR pathway, there are significantly relatively few proteins that can inhibit the AKT/mTOR pathway through upstream regulation, which may relate to the function of the AKT/mTOR pathway itself. For normal cells, the AKT/mTOR pathway mediates many functions in cells, including nutrient absorption, anabolic response, cell growth and survival, and so on (Yu and Cui, 2016), while most studies often overlook its importance in developmental processes due to its over-activation in cancer. To the embryo, inactivation of any one of the core components of the pathway results in embryonic death (Murakami et al., 2004, Peng et al., 2003) indicating its necessity for ontogeny and perhaps also explaining the apparently smaller number of pathway-associated repressor proteins than facilitator proteins.
Regulator of G protein signaling 12 (RGS12) is a structural protein with a Post-synaptic density-95, disks-large and zonula occludens-1 (PDZ) domain at its N-terminus. Meanwhile, PTEN has a C-terminal PDZ domain-binding motif (PBM), and some certain regulatory proteins that contain a PDZ domain can specifically recognize the PBM. So, with the help of PBM, RGS12 can recognize and then bond to PTEN, resulting in phosphorylation and sumoylation of PTEN, which in turn acts as an inhibitor (Fu et al., 2021).
PARK2 encodes an inter ring E3 ubiquitin ligase that mediates protein degradation via the ubiquitin-proteasome system. It has been found that the content of EGFR in cells would decrease with the overexpression of PARK2, which was also accompanied by a significant inhibition of p-EGFR, p-AKT, and p-mTOR, which shows that the overexpression of PARK2 can notably lower the expression of p-EGFR, p-AKT, and p-mTOR induced by EGF, and elevate the content of E-cadherin in cells, and play an inhibitory role on the migration and invasion of NSCLC (Duan et al., 2019).
In addition to RGS12 and PARK2, some proteins can act to inhibit the AKT/mTOR pathway by acting on PTEN, EGFR and others or AKT/mTOR itself, which will not be covered in detail here and specifically can be seen in Fig. 3 and Table 2.
Figure 3: Negative regulation of AKT/mTOR.
Fig. 3 shows the proteins that can inhibit the AKT/mTOR pathway in the upstream and their regulatory modes. Similar to activation, signaling molecules such as PI3K, FAK, EGFR, and PTEN remain the main mediators through which these proteins exert their regulatory effects. The PDZ domain at the N-terminus of RGS12 can specifically recognize and bind to the ITKV region on PTEN, promoting the phosphorylation and sumoylation of PTEN. Experimental findings show that α1-ACT co-immunoprecipitates with PTEN and inhibits AKT/mTOR activity. NC1 is the anti-tumor domain of collagen XIX, and the interaction between it and integrins αvβ3 decreases FAK phosphorylation. OGN would inhibit the pathway by affecting EGFR dimerization, internalization, and recruitment of Eps15 and epsin1 to EGFR. SLURP-1 can form a complex with EGFR and thereby affect the function of EGFR, which in turn plays an inhibitory role.
Proteins that May Link Other Pathways to AKT/mTOR
The nuclear factor kappa-B (NF-κB) family contains five members, namely RelA(p65), RelB, c-Rel, NF-κB1(p50) and NF-κB2(p52), all of which have a Rel homologous domain (RHD) of about 300 amino acids. NF-κB located in the cytoplasm is normally inactive as a result of binding to IκB and forming a trimeric complex. As a kinase, when NF-κB receives appropriate signals, IκB kinase (IKK) will phosphorylate the Ser32 and Ser36 of the IκBα and the Ser19 and Ser23 of the IκBβ, to dissociate IκB from the heterotrimer and degrade it by the proteasome after ubiquitination modification. At this time, the nuclear localization sequence of NF-κB is exposed, allowing rapid entry into the nucleus, and when it binds to a specific sequence of DNA, the transcription of related genes can be initiated or enhanced. In addition, cell adhesion molecules, MMPs and vascular endothelial growth factor (VEGF) are all able to be induced by NF-κB, thereby promoting cancer cell invasion and migration (Dolcet et al., 2005).
Zinc finger and scan domain containing 16 (ZSCAN16), a member of the DNA binding transcription factor family, is a protein-coding gene, and many studies have discovered that the abnormal expression of ZSCAN transcription factors occurs in diverse cancers. In bladder cancer, ZSCAN16 was found to have an abnormally high expression, which was associated with a stronger ability of invasion and migration. It is known from the literature that AKT, mTOR and NF-κB-p65 can form the AKT/mTOR/NF-κB pathway as well as the positive feedback loop, and the activity of downstream NF-κB is regulated by mTORC1 rather than mTORC2 (Li et al., 2016), while ZSCAN16 was silenced, and the expression of intracellular NF-κB-p65, p-AKT, p-mTOR were downregulated, which illustrates that ZSCAN16 would participate in regulating this pathway and contribute to cell metastasis (Li et al., 2020a).
Orai-1 together with orai-2 can work with stromal interaction molecules to regulate store-operated calcium (Ca2+) entry channels (SOCC) and then control cellular calcium intake. For cancer cells, changes in intracellular calcium levels can affect phosphorylation and activity of the calcium-dependent protein CaMKII and contribute to the malignant behavior of cancer cells. In oral cancer, when orai-1 was silenced simultaneously with orai-2, the levels of both p-AKT (Thr308 and Ser473) and p-NF-κB decreased. However, mTOR but not p-mTOR expression was observed by silencing orai-2 alone, and unlike orai-2, the silencing of orai-1 did not affect mTOR expression and phosphorylation, but the expression of NF-κB (Singh et al., 2020).
Tumor necrosis factor-α-induced protein 8-like 2 (TIPE2) is located in the cytoplasm and has a profound role in promoting proliferation, survival, and migration of lung cancer. The knockdown of TIPE2 has been shown to result in a collective downregulation of AKT1, p-AKT (Ser473), mTOR, p-mTOR (Ser2448), NF-κB and p-NF-κB (Ser535), and upregulation of PTEN expression was observed during this process, suggesting that TIPE2 may function through PTEN to control the AKT/mTOR/NF-κB pathway (Bordoloi et al., 2019).
TIPE3 plays a similar part to TIPE2 in cells, regulating the AKT/mTOR/NF-κB pathway and affecting cell migration. Differently, TIPE3 could also downregulate the expression of STAT-3 and p-STAT-3 (Bordoloi et al., 2022).
Wnt1 inducible signaling pathway protein-3 (CCN6) belongs to the CCN protein family, and its expression plays different and sometimes completely opposite roles in different tumor cells. In chondrosarcoma, a high expression of CCN6 promotes its migration and invasion, and this process is achieved through the response of MMP-9. Subsequent specific experiments found that the upregulation of MMP-9 expression by CCN6 was dependent on the promoter activity of NF-κB and this increase in activity cannot leave the function of AKT/mTOR pathway, whereby CCN6 can activate the PI3K/AKT/mTOR/NF-κB pathways to act on migration (Tzeng et al., 2018).
Signal transducer and activator of Transcription3 (STAT3) is a well conserved protein of approximately seven hundred and seventy amino acids with an SH2 domain in its structure, which plays an enormous role in intercellular signal transduction. The JAK/STAT pathway is a classic pathway in which STAT3 participates, and Janus kinases (JAK) in the cytoplasm can noncovalently bind to specific receptors on the cell surface and undergo conformational changes, allowing their tyrosine residues to couple and phosphorylate each other, releasing their intrinsic catalytic activity, and JAKs become activated. Activated JAKs can phosphorylate tyrosine sites on the cytoplasmic side of the receptor to create regions that can bind to STAT3, at which point the SH2 domain on STAT3 binds to tyrosine residues phosphorylated on the receptor and is phosphorylated by JAKs to form homo- or heterodimers that translocate to the nucleus where they interact with several other transducers to regulate gene transcription (Levy and Darnell, 2002). In addition to JAKs, STAT3 can also be phosphorylated by Src. When some growth factors such as EGFR bind to cell surface receptors, the Src is phosphorylated, which in turn can phosphorylate Stat3 (Park et al., 1996).
The Tumor necrosis factor-α induced protein 8 (TIPE/TNFAIP8) family consists of TIPE, TIPE1, TIPE2 and TIPE3, which have been implicated in tumorigenesis. In oral cancer cells, the expression of TIPE, TIPE2, and TIPE3 were all increased, whereas TIPE1 was decreased. The experiments showed that silencing of TIPE, TIPE1, and TIPE3 all affected the AKT/mTOR pathway. Silencing of TIPE causes a decrease in the levels of AKT, p-AKT, mTOR, p-mTOR, S6 and p-S6. Not only that, TIPE silencing is also related to the downregulation of EGFR, so TIPE can inhibit AKT/mTOR activation through the downregulation of EGFR. The downregulation of PTEN was associated with the silencing of TIPE1, and this allowed AKT and mTOR to be activated by phosphorylation, which subsequently led to S6 phosphorylation and affected STAT3 activation. The knockdown of TIPE2 suppressed AKT and mTOR expression and activation, and because AKT and mTOR have activating effects on NF-κB and STAT3, the levels of p-STAT3 and p-NF-κB would be consequently reduced, finally leading to a decrease in proliferation and migration. The knockdown of TIPE3 significantly decreases the levels of mTOR and p-mTOR as well, but different from other family members, the knockdown of TIPE3 failed to change the level of p-AKT, suggesting that its regulation may be through activation of the mTOR/STAT3 pathway (Padmavathi et al., 2021; Bordoloi et al., 2020).
Hypoxia-inducible factor (HIF)-1α can induce cytokine expression and thereby enhance adverse behaviors such as tumor migration. CXCL8 (i.e., interleukin-8) is a chemokine that has the characteristics of tumorigenesis and angiogenesis. HIF-1α has been observed in hepatocellular carcinoma, upregulated in synchrony with CXCL8 expression, and the expression of CXCL8 would be decreased through the silencing of HIF-1α. Meanwhile, the decrease of CXCL8 expression will lead to a significant decrease in p-AKT and p-mTOR signals, accompanied by a significant decrease in p-STAT3. This illustrates that when the expression of HIF-1α is increased by hypoxia induction, CXCL8 expression can be regulated to activate AKT/mTOR/STAT3, thereby promoting cancer cell invasion and migration (Li et al., 2015).
Discussion on AKT/mTOR Signal Pathway: Present Advances and Future Therapeutic Applications
Although the primary tumor is very dangerous, it will only cause the death of about 10% of tumor patients, and about 90% of tumor patients will eventually die of the growth of metastatic tumors outside the primary focus. Inhibiting cancer cell metastasis and reducing the occurrence of metastasis are important for cancer treatment. The AKT/mTOR pathway can be used to guide glucose biosynthesis and further promote the absorption of those glucose storage enzymes. In addition to glucose metabolism, mTOR can also be activated by growth factors and amino acids when there is surplus material and energy in cells. This process also reflects the pivotal role of this pathway in regulating growth and metabolism.
It is known to all that a tumor is a large energy consumer. Since the AKT/mTOR pathway can regulate cell growth and energy metabolism, it must be related to tumorigenesis. However, in fact, the influence of this pathway on the carcinogenesis process is not simply regulated through metabolism. Activated AKT/mTOR can directly or indirectly affect the behavior of cancer cells through different downstream signals, including proliferation, migration, invasion and so on. In view of the adverse effects of metastasis in the process of carcinogenesis, this paper mainly explores the effects of a variety of proteins on tumor migration and invasion through the AKT/mTOR pathway.
As a pathway that can play a role in many biological processes, AKT/mTOR has been widely studied. PTEN, EGFR and FAK are common in the upstream of this pathway, and their regulatory effects on this pathway have also been verified. In the process of searching the literature, we found that many proteins do not directly regulate the AKT/mTOR pathway, but rather activate or inhibit the pathway by regulating its upstream targets, and then play a corresponding role. There are not a few proteins that can affect the expression or phosphorylation level of AKT or mTOR. Although the specific mechanism of some proteins is not clear, these proteins still hold promise as potential targets of cancer treatment to affect tumor migration. As an indispensable step in the process of tumor cell migration, EMT is the downstream target of many proteins regulating AKT/mTOR pathway. As the only matrix proteases that can degrade type IV collagen, MMP-2 and MMP-9 also play a great part in the process of invasion and migration, which also enables many proteins to affect tumor invasion through their regulation.
As mentioned earlier, the AKT/mTOR pathway is the hub for regulating growth and metabolism, so its connection with other pathways can also affect various behaviors of tumors. Many proteins can often influence the activation or inhibition of other signal pathways while regulating the AKT/mTOR pathway, indicating that the influence of signal pathways is not single, but interrelated, and this connection sometimes needs to be realized through other factors.
All in all, the impact of the AKT/mTOR pathway on invasion and migration of cancer cells is obvious, and the discovery of proteins that regulate this pathway and, in turn, influence invasion and migration is crucial for the treatment of metastatic cancer. Nowadays, there are many tumor suppressor drugs developed based on the regulatory proteins of this pathway, many of which have pronounced side effects and are not clinically applicable. Through the screening of multiple proteins, perhaps, in the not-too-distant future, researchers will identify suitable AKT/mTOR pathway inhibitors that can be adapted for clinical tumor migration inhibition, yielding benefits for cancer metastasis therapy.
For cancer cells, the AKT/mTOR pathway is important in invasion and migration, and numerous proteins can exert their effects by regulating the AKT/mTOR upstream molecules or the pathway itself, indicating that this pathway is an effective target for cancer therapy. However, how to effectively inhibit cell invasion and migration through the action of different regulatory proteins and circumvent the adverse effects it brings is still a challenge. A review of the proteins that can directly or indirectly regulate the AKT/mTOR pathway may help us understand the regulatory mechanisms of different proteins on the AKT/mTOR pathway and the interaction relationship between them. In addition, as the AKT/mTOR pathway is associated with transplanted tumors, we also hope that the review about regulatory proteins of this pathway can help to find suitable cancer treatment targets in the future.
Acknowledgement: The authors’ appreciation goes to all members of the Sperm Laboratory of Zhejiang University for their help.
Funding Statement: This work was supported by the National Natural Science Foundation of China (Nos. 32102786 and 32270555).
Author Contributions: J. -Y. Xu conceived, wrote, revised the manuscript, and prepared all the figures; K. -Y. Han participated in the discussion; S. -L Hao and W. -X. Yang were involved in constructing the frame of the manuscript, participated in the revision and supplied the funds for this research.
Ethics Approval: Not applicable.
Conflicts of Interest: The authors declare that they have no conflicts of interest to report regarding the present study.
References
Aoki M, Fujishita T (2017). Oncogenic roles of the PI3K/AKT/mTOR axis. Current Topics in Microbiology and Immunology 407: 153–189. https://doi.org/10.1007/978-3-319-61804-3 [Google Scholar] [CrossRef]
Aruna, Li LM (2018). Overexpression of golgi membrane protein 1 promotes non-small-cell carcinoma aggressiveness by regulating the matrix metallopeptidase 13. American Journal of Cancer Research 8: 551–565. [Google Scholar] [PubMed]
Bao XY, Sun M, Peng TT, Han DM (2021). TRIB3 promotes proliferation, migration, and invasion of retinoblastoma cells by activating the AKT/mTOR signaling pathway. Cancer Biomarkers 31: 307–315. https://doi.org/10.3233/CBM-200050 [Google Scholar] [PubMed] [CrossRef]
Bi X, Lv X, Liu D, Guo H, Yao G, Wang L, Liang X, Yang Y (2021). METTL3-mediated maturation of miR-126-5p promotes ovarian cancer progression via PTEN-mediated PI3K/Akt/mTOR pathway. Cancer Gene Therapy 28: 335–349. https://doi.org/10.1038/s41417-020-00222-3 [Google Scholar] [PubMed] [CrossRef]
Bordoloi D, Banik K, Padmavathi G, Vikkurthi R, Harsha C et al. (2019). TIPE2 induced the proliferation, survival, and migration of lung cancer cells through modulation of Akt/mTOR/NF-κB signaling cascade. Biomolecules 9: 836. https://doi.org/10.3390/biom9120836 [Google Scholar] [PubMed] [CrossRef]
Bordoloi D, Banik K, Vikkurthi R, Thakur KK, Padmavathi G et al. (2020). Inflection of Akt/mTOR/STAT-3 cascade in TNF-α induced protein 8 mediated human lung carcinogenesis. Life Sciences 262: 118475. https://doi.org/10.1016/j.lfs.2020.118475 [Google Scholar] [PubMed] [CrossRef]
Bordoloi D, Harsha C, Padmavathi G, Banik K, Sailo BL et al. (2022). Loss of TIPE3 reduced the proliferation, survival and migration of lung cancer cells through inactivation of Akt/mTOR, NF-κB, and STAT-3 signaling cascades. Life Sciences 293: 120332. https://doi.org/10.1016/j.lfs.2022.120332 [Google Scholar] [PubMed] [CrossRef]
Bu L, Wang H, Pan JA, Chen L, Xing F, Wu J, Li S, Guo D (2021). PTEN suppresses tumorigenesis by directly dephosphorylating Akt. Signal Transduction and Targeted Therapy 6: 262. https://doi.org/10.1038/s41392-021-00571-x [Google Scholar] [PubMed] [CrossRef]
Bychkov ML, Shulepko MA, Shlepova OV, Kulbatskii DS, Chulina IA et al. (2021). SLURP-1 controls growth and migration of lung adenocarcinoma cells, forming a complex with α7-nAChR and PDGFR/EGFR heterodimer. Frontiers in Cell and Developmental Biology 9: 739391. https://doi.org/10.3389/fcell.2021.739391 [Google Scholar] [PubMed] [CrossRef]
Cai C, Dang W, Liu S, Huang L, Li Y et al. (2020). Anthrax toxin receptor 1/tumor endothelial marker 8 promotes gastric cancer progression through activation of the PI3K/AKT/mTOR signaling pathway. Cancer Science 111: 1132–1145. https://doi.org/10.1111/cas.14326 [Google Scholar] [PubMed] [CrossRef]
Cai M, Li H, Chen R, Zhou X (2021). MRPL13 promotes tumor cell proliferation, migration and EMT process in breast cancer through the PI3K-AKT-mTOR pathway. Cancer Management and Research 13: 2009–2024. https://doi.org/10.2147/CMAR.S296038 [Google Scholar] [PubMed] [CrossRef]
Cao Z, Chen H, Mei X, Li X (2021). Silencing of NACC1 inhibits the proliferation, migration and invasion of nasopharyngeal carcinoma cells via regulating the AKT/mTOR signaling pathway. Oncology Letters 22: 828. https://doi.org/10.3892/ol.2021.13088 [Google Scholar] [PubMed] [CrossRef]
Chen G, Chen SM, Wang X, Ding XF, Ding J, Meng LH (2012). Inhibition of chemokine (CXC motif) ligand 12/chemokine (CXC motif) receptor 4 axis (CXCL12/CXCR4)-mediated cell migration by targeting mammalian target of rapamycin (mTOR) pathway in human gastric carcinoma cells. The Journal of Biological Chemistry 287: 12132–12141. https://doi.org/10.1074/jbc.M111.302299 [Google Scholar] [PubMed] [CrossRef]
Datta SR, Brunet A, Greenberg ME (1999). Cellular survival: A play in three Akts. Genes & Development 13: 2905–2927. https://doi.org/10.1101/gad.13.22.2905 [Google Scholar] [PubMed] [CrossRef]
Dolcet X, Llobet D, Pallares J, Matias-Guiu X (2005). NF-kB in development and progression of human cancer. Virchows Archiv 446: 475–482. https://doi.org/10.1007/s00428-005-1264-9 [Google Scholar] [PubMed] [CrossRef]
Duan H, Lei Z, Xu F, Pan T, Lu D, Ding P, Zhu C, Pan C, Zhang S (2019). PARK2 suppresses proliferation and tumorigenicity in non-small cell lung cancer. Frontiers in Oncology 9: 790. https://doi.org/10.3389/fonc.2019.00790 [Google Scholar] [PubMed] [CrossRef]
Ediriweera MK, Tennekoon KH, Samarakoon SR (2019). Role of the PI3K/AKT/mTOR signaling pathway in ovarian cancer: Biological and therapeutic significance. Seminars in Cancer Biology 59: 147–160. https://doi.org/10.1016/j.semcancer.2019.05.012 [Google Scholar] [PubMed] [CrossRef]
Fattahi S, Amjadi-Moheb F, Tabaripour R, Ashrafi GH, Akhavan-Niaki H (2020). PI3K/AKT/mTOR signaling in gastric cancer: Epigenetics and beyond. Life Sciences 262: 118513. https://doi.org/10.1016/j.lfs.2020.118513 [Google Scholar] [PubMed] [CrossRef]
Fu C, Yuan G, Yang ST, Zhang D, Yang S (2021). RGS12 represses oral cancer via the phosphorylation and SUMOylation of PTEN. Journal of Dental Research 100: 522–531. https://doi.org/10.1177/0022034520972095 [Google Scholar] [PubMed] [CrossRef]
Geng Y, Zuo P, Li XO, Zhang L (2020). PODNL1 promotes cell proliferation and migration in glioma via regulating Akt/mTOR pathway. Journal of Cancer 11: 6234–6242. https://doi.org/10.7150/jca.46901 [Google Scholar] [PubMed] [CrossRef]
Guan Y, Luan Y, Xie Y, Zhou H, Li W et al. (2019). Chloride channel-3 is required for efficient tumour cell migration and invasion in human cervical squamous cell carcinoma. Gynecologic Oncology 153: 661–669. https://doi.org/10.1016/j.ygyno.2019.03.006 [Google Scholar] [PubMed] [CrossRef]
Guo C, Chu H, Gong Z, Zhang B, Li C, Chen J, Huang L (2021). HOXB13 promotes gastric cancer cell migration and invasion via IGF-1R upregulation and subsequent activation of PI3K/AKT/mTOR signaling pathway. Life Sciences 278: 119522. https://doi.org/10.1016/j.lfs.2021.119522 [Google Scholar] [PubMed] [CrossRef]
Han Y, Liu C, Zhang D, Men H, Huo L et al. (2019). Mechanosensitive ion channel Piezo1 promotes prostate cancer development through the activation of the Akt/mTOR pathway and acceleration of cell cycle. International Journal of Oncology 55: 629–644. https://doi.org/10.3892/ijo.2019.4839 [Google Scholar] [PubMed] [CrossRef]
Hu X, Li YQ, Li QG, Ma YL, Peng JJ, Cai SJ (2018). Osteoglycin (OGN) reverses epithelial to mesenchymal transition and invasiveness in colorectal cancer via EGFR/Akt pathway. Journal of Experimental & Clinical Cancer Research: CR 37: 41. https://doi.org/10.1186/s13046-018-0718-2 [Google Scholar] [PubMed] [CrossRef]
Huang YK, Kang WM, Ma ZQ, Liu YQ, Zhou L, Yu JC (2019). NUCKS1 promotes gastric cancer cell aggressiveness by upregulating IGF-1R and subsequently activating the PI3K/Akt/mTOR signaling pathway. Carcinogenesis 40: 370–379. https://doi.org/10.1093/carcin/bgy142 [Google Scholar] [PubMed] [CrossRef]
Huang L, Zhao C, Sun K, Yang D, Yan L et al. (2020). Downregulation of CLDN6 inhibits cell proliferation, migration, and invasion via regulating EGFR/AKT/mTOR signalling pathway in hepatocellular carcinoma. Cell Biochemistry and Function 38: 541–548. https://doi.org/10.1002/cbf.3489 [Google Scholar] [PubMed] [CrossRef]
Jia YY, Yu Y, Li HJ (2021). POSTN promotes proliferation and epithelial-mesenchymal transition in renal cell carcinoma through ILK/AKT/mTOR pathway. Journal of Cancer 12: 4183–4195. https://doi.org/10.7150/jca.51253 [Google Scholar] [PubMed] [CrossRef]
Jiang Z, Liu Y, Wang C (2016). Oncogenic NanogP8 expression regulates cell proliferation and migration through the Akt/mTOR signaling pathway in human gastric cancer—SGC-7901cell line. OncoTargets and Therapy 9: 4859–4866. https://doi.org/10.2147/OTT [Google Scholar] [CrossRef]
Jiang C, Ma Z, Zhang G, Yang X, Du Q, Wang W (2019a). CSNK2A1 promotes gastric cancer invasion through the PI3K-Akt-mTOR signaling pathway. Cancer Management and Research 11: 10135–10143. https://doi.org/10.2147/CMAR [Google Scholar] [CrossRef]
Jiang H, Shi QQ, Ge LY, Zhuang QF, Xue D, Xu HY, He XZ (2019b). Selenoprotein M stimulates the proliferative and metastatic capacities of renal cell carcinoma through activating the PI3K/AKT/mTOR pathway. Cancer Medicine 8: 4836–4844. https://doi.org/10.1002/cam4.2403 [Google Scholar] [PubMed] [CrossRef]
Jiang F, Zhang D, Li G, Wang X (2017). Knockdown of DDX46 inhibits the invasion and tumorigenesis in osteosarcoma cells. Oncology Research 25: 417–425. https://doi.org/10.3727/096504016X14747253292210 [Google Scholar] [PubMed] [CrossRef]
Karimi Roshan M, Soltani A, Soleimani A, Rezaie Kahkhaie K, Afshari AR, Soukhtanloo M (2019). Role of AKT and mTOR signaling pathways in the induction of epithelial-mesenchymal transition (EMT) process. Biochimie 165: 229–234. https://doi.org/10.1016/j.biochi.2019.08.003 [Google Scholar] [PubMed] [CrossRef]
Kim H, Ju JH, Son S, Shin I (2020). Silencing of CD133 inhibits GLUT1-mediated glucose transport through downregulation of the HER3/Akt/mTOR pathway in colon cancer. FEBS Letters 594: 1021–1035. https://doi.org/10.1002/1873-3468.13686 [Google Scholar] [PubMed] [CrossRef]
Levy DE, Darnell JEJr (2002). Stats: Transcriptional control and biological impact. Nature Reviews Molecular Cell Biology 3: 651–662. https://doi.org/10.1038/nrm909 [Google Scholar] [PubMed] [CrossRef]
Li L, Liu M, Zhang Z, Zhang W, Liu N, Sheng X, Wei P (2019). Derlin1 functions as an oncogene in cervical cancer via AKT/mTOR signaling pathway. Biological Research 52: 8. https://doi.org/10.1186/s40659-019-0215-x [Google Scholar] [PubMed] [CrossRef]
Li X, Shang D, Shen H, Song J, Hao G, Tian Y (2020a). ZSCAN16 promotes proliferation, migration and invasion of bladder cancer via regulating NF-kB, AKT, mTOR, P38 and other genes. Biomedicine & Pharmacotherapy 126: 110066. https://doi.org/10.1016/j.biopha.2020.110066 [Google Scholar] [PubMed] [CrossRef]
Li XP, Yang XY, Biskup E, Zhou J, Li HL, Wu YF, Chen ML, Xu F (2015). Co-expression of CXCL8 and HIF-1α is associated with metastasis and poor prognosis in hepatocellular carcinoma. Oncotarget 6: 22880–22889. https://doi.org/10.18632/oncotarget.4412 [Google Scholar] [PubMed] [CrossRef]
Li Z, Yang Z, Passaniti A, Lapidus RG, Liu X, Cullen KJ, Dan HC (2016). A positive feedback loop involving EGFR/Akt/mTORC1 and IKK/NF-kB regulates head and neck squamous cell carcinoma proliferation. Oncotarget 7: 31892–31906. https://doi.org/10.18632/oncotarget.7441 [Google Scholar] [PubMed] [CrossRef]
Li J, Yang R, Yang H, Chen S, Wang L, Li M, Yang S, Feng Z, Bi J (2020b). NCAM regulates the proliferation, apoptosis, autophagy, EMT, and migration of human melanoma cells via the Src/Akt/mTOR/cofilin signaling pathway. Journal of Cellular Biochemistry 121: 1192–1204. https://doi.org/10.1002/jcb.29353 [Google Scholar] [PubMed] [CrossRef]
Liang JX, Gao W, Cai L (2017). Fucosyltransferase VII promotes proliferation via the EGFR/AKT/mTOR pathway in A549 cells. OncoTargets and Therapy 10: 3971–3978. https://doi.org/10.2147/OTT [Google Scholar] [CrossRef]
Liu Y, Xu S, Huang Y, Liu S, Xu Z, Wei M, Liu J (2021). MARCH6 promotes papillary thyroid cancer development by destabilizing DHX9. International Journal of Biological Sciences 17: 3401–3412. https://doi.org/10.7150/ijbs.60628 [Google Scholar] [PubMed] [CrossRef]
Lu J, Jeong HW, Kong N, Yang Y, Carroll J, Luo HR, Silberstein LE, Yupoma, Chai L (2009). Stem cell factor SALL4 represses the transcriptions of PTEN and SALL1 through an epigenetic repressor complex. PLoS One 4: e5577. https://doi.org/10.1371/journal.pone.0005577 [Google Scholar] [PubMed] [CrossRef]
Maehama T, Dixon JE (1998). The tumor suppressor, PTEN/MMAC1, dephosphorylates the lipid second messenger, phosphatidylinositol 3,4,5-trisphosphate. Journal of Biological Chemistry 273: 13375–13378. https://doi.org/10.1074/jbc.273.22.13375 [Google Scholar] [PubMed] [CrossRef]
Mittal V (2018). Epithelial mesenchymal transition in tumor metastasis. Annual Review of Pathology 13: 395–412. https://doi.org/10.1146/annurev-pathol-020117-043854 [Google Scholar] [PubMed] [CrossRef]
Murakami M, Ichisaka T, Maeda M, Oshiro N, Hara K, Edenhofer F, Kiyama H, Yonezawa K, Yamanaka S (2004). mTOR is essential for growth and proliferation in early mouse embryos and embryonic stem cells. Molecular and Cellular Biology 24: 6710–6718. https://doi.org/10.1128/MCB.24.15.6710-6718.2004 [Google Scholar] [PubMed] [CrossRef]
Murugan AK (2019). mTOR: Role in cancer, metastasis and drug resistance. Seminars in Cancer Biology 59: 92–111. https://doi.org/10.1016/j.semcancer.2019.07.003 [Google Scholar] [PubMed] [CrossRef]
Nguyen K, Yan Y, Yuan B, Dasgupta A, Sun J, Mu H, Do KA, Ueno NT, Andreeff M, Battula VL (2018). ST8SIA1 regulates tumor growth and metastasis in TNBC by activating the FAK-AKT-mTOR signaling pathway. Molecular Cancer Therapeutics 17: 2689–2701. https://doi.org/10.1158/1535-7163.MCT-18-0399 [Google Scholar] [PubMed] [CrossRef]
Normanno N, de Luca A, Bianco C, Strizzi L, Mancino M, Maiello MR, Carotenuto A, de Feo G, Caponigro F, Salomon DS (2006). Epidermal growth factor receptor (EGFR) signaling in cancer. Gene 366: 2–16. https://doi.org/10.1016/j.gene.2005.10.018 [Google Scholar] [PubMed] [CrossRef]
Orlichenko LS, Radisky DC (2008). Matrix metalloproteinases stimulate epithelial-mesenchymal transition during tumor development. Clinical & Experimental Metastasis 25: 593–600. https://doi.org/10.1007/s10585-008-9143-9 [Google Scholar] [PubMed] [CrossRef]
Oudart JB, Doué M, Vautrin A, Brassart B, Sellier C, Dupont-Deshorgue A, Monboisse JC, Maquart FX, Brassart-Pasco S, Ramont L (2016). The anti-tumor NC1 domain of collagen XIX inhibits the FAK/PI3K/Akt/mTOR signaling pathway through αvβ3 integrin interaction. Oncotarget 7: 1516–1528. https://doi.org/10.18632/oncotarget.6399 [Google Scholar] [PubMed] [CrossRef]
Padmavathi G, Monisha J, Bordoloi D, Banik K, Roy NK, Girisa S, Singh AK, Longkumer I, Baruah MN, Kunnumakkara AB (2021). Tumor necrosis factor-α induced protein 8 (TNFAIP8/TIPE) family is differentially expressed in oral cancer and regulates tumorigenesis through Akt/mTOR/STAT3 signaling cascade. Life Sciences 287: 120118. https://doi.org/10.1016/j.lfs.2021.120118 [Google Scholar] [PubMed] [CrossRef]
Park OK, Schaefer TS, Nathans D (1996). In vitro activation of Stat3 by epidermal growth factor receptor kinase. PNAS 93: 13704–13708. https://doi.org/10.1073/pnas.93.24.13704 [Google Scholar] [PubMed] [CrossRef]
Parsons JT (2003). Focal adhesion kinase: The first ten years. Journal of Cell Science 116: 1409–1416. https://doi.org/10.1242/jcs.00373 [Google Scholar] [PubMed] [CrossRef]
Peng XD, Xu PZ, Chen ML, Hahn-Windgassen A, Skeen J et al. (2003). Dwarfism, impaired skin development, skeletal muscle atrophy, delayed bone development, and impeded adipogenesis in mice lacking Akt1 and Akt2. Genes & Development 17: 1352–1365. https://doi.org/10.1101/gad.1089403 [Google Scholar] [PubMed] [CrossRef]
Quan C, Sun J, Lin Z, Jin T, Dong B, Meng Z, Piao J (2019). Ezrin promotes pancreatic cancer cell proliferation and invasion through activating the Akt/mTOR pathway and inducing YAP translocation. Cancer Management and Research 11: 6553–6566. https://doi.org/10.2147/CMAR [Google Scholar] [CrossRef]
Ray JM, Stetler-Stevenson WG (1994). The role of matrix metalloproteases and their inhibitors in tumour invasion, metastasis and angiogenesis. The European Respiratory Journal 7: 2062–2072. https://doi.org/10.1183/09031936.94.07112062 [Google Scholar] [CrossRef]
Rodrigues GA, Falasca M, Zhang Z, Ong SH, Schlessinger J (2000). A novel positive feedback loop mediated by the docking protein Gab1 and phosphatidylinositol 3-kinase in epidermal growth factor receptor signaling. Molecular and Cellular Biology 20: 1448–1459. https://doi.org/10.1128/MCB.20.4.1448-1459.2000 [Google Scholar] [PubMed] [CrossRef]
Sancak Y, Thoreen CC, Peterson TR, Lindquist RA, Kang SA, Spooner E, Carr SA, Sabatini DM (2007). PRAS40 is an insulin-regulated inhibitor of the mTORC1 protein kinase. Molecular Cell 25: 903–915. https://doi.org/10.1016/j.molcel.2007.03.003 [Google Scholar] [PubMed] [CrossRef]
Shao X, Kuai X, Pang Z, Zhang L, Wu L, Xu L, Zhou C (2018). Correlation of Gli1 and HER2 expression in gastric cancer: Identification of novel target. Scientific Reports 8: 397. https://doi.org/10.1038/s41598-017-17435-6 [Google Scholar] [PubMed] [CrossRef]
Shao Q, Zhang Z, Cao R, Zang H, Pei W, Sun T (2020). CPA4 promotes EMT in pancreatic cancer via stimulating PI3K-AKT-mTOR signaling. OncoTargets and Therapy 13: 8567–8580. https://doi.org/10.2147/OTT.S257057 [Google Scholar] [PubMed] [CrossRef]
Shorning BY, Dass MS, Smalley MJ, Pearson HB (2020). The PI3K-AKT-mTOR pathway and prostate cancer: At the crossroads of AR, MAPK, and WNT signaling. International Journal of Molecular Sciences 21: 4507. https://doi.org/10.3390/ijms21124507 [Google Scholar] [PubMed] [CrossRef]
Shu X, Zhan PP, Sun LX, Yu L, Liu J, Sun LC, Yang ZH, Ran YL, Sun YM (2021). BCAT1 activates PI3K/AKT/mTOR pathway and contributes to the angiogenesis and tumorigenicity of gastric cancer. Frontiers in Cell and Developmental Biology 9: 659260. https://doi.org/10.3389/fcell.2021.659260 [Google Scholar] [PubMed] [CrossRef]
Siech C, Rutz J, Maxeiner S, Grein T, Sonnenburg M, Tsaur I, Chun FK, Blaheta RA (2022). Insulin-like growth factor-1 influences prostate cancer cell growth and invasion through an integrin α3, α5, αV, and β1 dependent mechanism. Cancers 14: 363. https://doi.org/10.3390/cancers14020363 [Google Scholar] [PubMed] [CrossRef]
Singh AK, Roy NK, Bordoloi D, Padmavathi G, Banik K, Khwairakpam AD, Kunnumakkara AB, Sukumar P (2020). Orai-1 and Orai-2 regulate oral cancer cell migration and colonisation by suppressing Akt/mTOR/NF-κB signalling. Life Sciences 261: 118372. https://doi.org/10.1016/j.lfs.2020.118372 [Google Scholar] [PubMed] [CrossRef]
Sternlicht MD, Werb Z (2001). How matrix metalloproteinases regulate cell behavior. Annual Review of Cell and Developmental Biology 17: 463–516. https://doi.org/10.1146/annurev.cellbio.17.1.463 [Google Scholar] [PubMed] [CrossRef]
Su YL, Luo HL, Huang CC, Liu TT, Huang EY et al. (2020). Galectin-1 overexpression activates the FAK/PI3K/AKT/mTOR pathway and is correlated with upper urinary urothelial carcinoma progression and survival. Cells 9: 806. https://doi.org/10.3390/cells9040806 [Google Scholar] [PubMed] [CrossRef]
Sun Z, Xue H, Wei Y, Wang C, Yu R et al. (2019). Mucin O-glycosylating enzyme GALNT2 facilitates the malignant character of glioma by activating the EGFR/PI3K/Akt/mTOR axis. Clinical Science 133: 1167–1184. https://doi.org/10.1042/CS20190145 [Google Scholar] [PubMed] [CrossRef]
Toker A, Marmiroli S (2014). Signaling specificity in the Akt pathway in biology and disease. Advances in Biological Regulation 55: 28–38. https://doi.org/10.1016/j.jbior.2014.04.001 [Google Scholar] [PubMed] [CrossRef]
Tzeng HE, Tang CH, Wu SH, Chen HT, Fong YC, Lu YC, Chen WC, Huang HD, Lin CY, Wang SW (2018). CCN6-mediated MMP-9 activation enhances metastatic potential of human chondrosarcoma. Cell Death & Disease 9: 955. https://doi.org/10.1038/s41419-018-1008-9 [Google Scholar] [PubMed] [CrossRef]
Vo BT, Morton Jr D, Komaragiri S, Millena AC, Leath C, Khan SA (2013). TGF-β effects on prostate cancer cell migration and invasion are mediated by PGE2 through activation of PI3K/AKT/mTOR pathway. Endocrinology 154: 1768–1779. https://doi.org/10.1210/en.2012-2074 [Google Scholar] [PubMed] [CrossRef]
Wang B, Cao C, Liu X, He X, Zhuang H, Wang D, Chen B (2020a). BRCA1-associated protein inhibits glioma cell proliferation and migration and glioma stem cell self-renewal via the TGF-β/PI3K/AKT/mTOR signalling pathway. Cellular Oncology 43: 223–235. https://doi.org/10.1007/s13402-019-00482-8 [Google Scholar] [PubMed] [CrossRef]
Wang RY, Chen XW, Zhang WW, Jiang F, Liu MQ, Shen XB (2020b). CYP2E1 changes the biological function of gastric cancer cells via the PI3K/Akt/mTOR signaling pathway. Molecular Medicine Reports 21: 842–850. https://doi.org/10.3892/mmr.2019.10890 [Google Scholar] [PubMed] [CrossRef]
Wang H, Duan L, Zou Z, Li H, Yuan S et al. (2014a). Activation of the PI3K/Akt/mTOR/p70S6K pathway is involved in S100A4-induced viability and migration in colorectal cancer cells. International Journal of Medical Sciences 11: 841–849. https://doi.org/10.7150/ijms.8128 [Google Scholar] [PubMed] [CrossRef]
Wang W, Guo X, Dan H (2020c). α2A-adrenergic receptor inhibits the progression of cervical cancer through blocking PI3K/AKT/mTOR pathway. OncoTargets and Therapy 13: 10535–10546. https://doi.org/10.2147/OTT.S264409 [Google Scholar] [PubMed] [CrossRef]
Wang C, Li L, Duan Q, Wang Q, Chen J (2017). Krüppel-like factor 2 suppresses human gastric tumorigenesis through inhibiting PTEN/AKT signaling. Oncotarget 8: 100358–100370. https://doi.org/10.18632/oncotarget.22229 [Google Scholar] [PubMed] [CrossRef]
Wang B, Qin H, Wang Y, Chen W, Luo J, Zhu X, Wen W, Lei W (2014b). Effect of DJ-1 overexpression on the proliferation, apoptosis, invasion and migration of laryngeal squamous cell carcinoma SNU-46 cells through PI3K/AKT/mTOR. Oncology Reports 32: 1108–1116. https://doi.org/10.3892/or.2014.3286 [Google Scholar] [PubMed] [CrossRef]
Wang M, Qiu R, Gong Z, Zhao X, Wang T, Zhou L, Lu W, Shen B, Zhu W, Xu W (2019a). miR-188-5p emerges as an oncomiRNA to promote gastric cancer cell proliferation and migration via upregulation of SALL4. Journal of Cellular Biochemistry 120: 15027–15037. https://doi.org/10.1002/jcb.28764 [Google Scholar] [PubMed] [CrossRef]
Wang B, Song K, Chen L, Su H, Gao L, Liu J, Huang A (2020d). Targeted inhibition of ACK1 can inhibit the proliferation of hepatocellular carcinoma cells through the PTEN/AKT/mTOR pathway. Cell Biochemistry and Function 38: 642–650. https://doi.org/10.1002/cbf.3522 [Google Scholar] [PubMed] [CrossRef]
Wang J, Zhang Y, Dou Z, Jiang H, Wang Y, Gao X, Xin X (2019b). Knockdown of STIL suppresses the progression of gastric cancer by down-regulating the IGF-1/PI3K/AKT pathway. Journal of Cellular and Molecular Medicine 23: 5566–5575. https://doi.org/10.1111/jcmm.14440 [Google Scholar] [PubMed] [CrossRef]
Wang S, Zhu M, Wang Q, Hou Y, Li L et al. (2018). Alpha-fetoprotein inhibits autophagy to promote malignant behaviour in hepatocellular carcinoma cells by activating PI3K/AKT/mTOR signalling. Cell Death & Disease 9: 1027. https://doi.org/10.1038/s41419-018-1036-5 [Google Scholar] [PubMed] [CrossRef]
Wu MH, Lo JF, Kuo CH, Lin JA, Lin YM, Chen LM, Tsai FJ, Tsai CH, Huang CY, Tang CH (2012). Endothelin-1 promotes MMP-13 production and migration in human chondrosarcoma cells through FAK/PI3K/Akt/mTOR pathways. Journal of Cellular Physiology 227: 3016–3026. https://doi.org/10.1002/jcp.23043 [Google Scholar] [PubMed] [CrossRef]
Wu D, Yu HQ, Xiong HJ, Zhang YJ, Lin XT, Zhang J, Wu W, Wang T, Liu XY, Xie CM (2021). Elevated sodium pump α3 Subunit Expression promotes colorectal liver metastasis via the p53-PTEN/IGFBP3-AKT-mTOR axis. Frontiers in Oncology 11: 743824. https://doi.org/10.3389/fonc.2021.743824 [Google Scholar] [PubMed] [CrossRef]
Xie J, Sun Y, Xu Q (2021). Inhibition of SRSF3 alleviates proliferation and migration of gastric cancer cells by regulating the PI3K/AKT/mTOR signalling pathway. Folia Biologica 67: 102–107. [Google Scholar] [PubMed]
Xing Y, Meng Q, Chen X, Zhao Y, Liu W, Hu J, Xue F, Wang X, Cai L (2016). TRIM44 promotes proliferation and metastasis in non‐small cell lung cancer via mTOR signaling pathway. Oncotarget 7: 30479–30491. https://doi.org/10.18632/oncotarget.8586 [Google Scholar] [PubMed] [CrossRef]
Xiong D, Jin C, Ye X, Qiu B, Jianjun X, Zhu S, Xiang L, Wu H, Yongbing W (2018). TRIM44 promotes human esophageal cancer progression via the AKT/mTOR pathway. Cancer Science 109: 3080–3092. https://doi.org/10.1111/cas.13762 [Google Scholar] [PubMed] [CrossRef]
Xu R, Ji J, Zhang X, Han M, Zhang C et al. (2017). PDGFA/PDGFRα-regulated GOLM1 promotes human glioma progression through activation of AKT. Journal of Experimental & Clinical Cancer Research: CR 36: 193. https://doi.org/10.1186/s13046-017-0665-3 [Google Scholar] [PubMed] [CrossRef]
Xu T, Rao T, Yu WM, Ning JZ, Yu X, Zhu SM, Yang K, Bai T, Cheng F (2021). Upregulation of NFKBIZ affects bladder cancer progression via the PTEN/PI3K/Akt signaling pathway. International Journal of Molecular Medicine 47: 109. https://doi.org/10.3892/ijmm.2021.4942 [Google Scholar] [PubMed] [CrossRef]
Xu M, Wang J, Li H, Zhang Z, Cheng Z (2020a). AIM2 inhibits colorectal cancer cell proliferation and migration through suppression of Gli1. Sedentary Life and Nutrition 13: 1017–1031. https://doi.org/10.18632/aging.202226 [Google Scholar] [PubMed] [CrossRef]
Xu M, Zhang X, Zhang S, Piao J, Yang Y, Wang X, Lin Z (2020b). SPOCK1/SIX1axis promotes breast cancer progression by activating AKT/mTOR signaling. Aging 13: 1032–1050. https://doi.org/10.18632/aging.202231 [Google Scholar] [PubMed] [CrossRef]
Yan G, Ru Y, Wu K, Yan F, Wang Q et al. (2018). GOLM1 promotes prostate cancer progression through activating PI3K-AKT-mTOR signaling. The Prostate 78: 166–177. https://doi.org/10.1002/pros.23461 [Google Scholar] [PubMed] [CrossRef]
Yan G, Ru Y, Yan F, Xiong X, Hu W, Pan T, Sun J, Zhang C, Wang Q, Li X (2019). MIIP inhibits the growth of prostate cancer via interaction with PP1α and negative modulation of AKT signaling. Cell Communication and Signaling 17: 44. https://doi.org/10.1186/s12964-019-0355-1 [Google Scholar] [PubMed] [CrossRef]
Yang HY, Fang DZ, Ding LS, Hui XB, Liu D (2017). Overexpression of protease serine 8 inhibits glioma cell proliferation, migration, and invasion via suppressing the Akt/mTOR signaling pathway. Oncology Research 25: 923–930. https://doi.org/10.3727/096504016X14798241682647 [Google Scholar] [PubMed] [CrossRef]
Yang N, Hui L, Wang Y, Yang H, Jiang X (2014). SOX2 promotes the migration and invasion of laryngeal cancer cells by induction of MMP-2 via the PI3K/Akt/mTOR pathway. Oncology Reports 31: 2651–2659. https://doi.org/10.3892/or.2014.3120 [Google Scholar] [PubMed] [CrossRef]
Yeung KT, Yang J (2017). Epithelial-mesenchymal transition in tumor metastasis. Molecular Oncology 11: 28–39. https://doi.org/10.1002/1878-0261.12017 [Google Scholar] [PubMed] [CrossRef]
Yin F, Huang X, Xuan Y (2021). Pyrroline-5-carboxylate reductase-2 promotes colorectal cancer progression via activating PI3K/AKT/mTOR pathway. Disease Markers 2021: 9950663. https://doi.org/10.1155/2021/9950663 [Google Scholar] [PubMed] [CrossRef]
Yu JS, Cui W (2016). Proliferation, survival and metabolism: The role of PI3K/AKT/mTOR signalling in pluripotency and cell fate determination. Development143: 3050–3060. https://doi.org/10.1242/dev.137075 [Google Scholar] [PubMed] [CrossRef]
Yu G, Huang B, Chen G, Mi Y (2015). Phosphatidylethanolamine-binding protein 4 promotes lung cancer cells proliferation and invasion via PI3K/Akt/mTOR axis. Journal of Thoracic Disease 7: 1806–1816. https://doi.org/10.3978/j.issn.2072-1439.2015.10.17 [Google Scholar] [PubMed] [CrossRef]
Zhan CH, Ding DS, Zhang W, Wang HL, Mao ZY, Liu GJ (2022). The cancer-testis antigen a-kinase anchor protein 3 facilitates breast cancer progression via activation of the PTEN/PI3K/AKT/mTOR signaling. Bioengineered 13: 8478–8489. https://doi.org/10.1080/21655979.2022.2051687 [Google Scholar] [PubMed] [CrossRef]
Zhang B, Deng C, Wang L, Zhou F, Zhang S et al. (2018a). Upregulation of UBE2Q1 via gene copy number gain in hepatocellular carcinoma promotes cancer progression through β-catenin-EGFR-PI3K-Akt-mTOR signaling pathway. Molecular Carcinogenesis 57: 201–215. https://doi.org/10.1002/mc.22747 [Google Scholar] [PubMed] [CrossRef]
Zhang T, Ji D, Wang P, Liang D, Jin L, Shi H, Liu X, Meng Q, Yu R, Gao S (2018b). The atypical protein kinase RIOK3 contributes to glioma cell proliferation/survival, migration/invasion and the AKT/mTOR signaling pathway. Cancer Letters 415: 151–163. https://doi.org/10.1016/j.canlet.2017.12.010 [Google Scholar] [PubMed] [CrossRef]
Zhang J, Lv W, Liu Y, Fu W, Chen B, Ma Q, Gao X, Cui X (2021a). Nucleoporin 37 promotes the cell proliferation, migration, and invasion of gastric cancer through activating the PI3K/AKT/mTOR signaling pathway. In Vitro Cellular & Developmental Biology Animal 57: 987–997. https://doi.org/10.1007/s11626-021-00627-w [Google Scholar] [PubMed] [CrossRef]
Zhang F, Meng J, Jiang H, Feng X, Wei D, Meng W (2021b). GTSE1 facilitates the malignant phenotype of lung cancer cells via activating AKT/mTOR signaling. Analytical Cellular Pathology 2021: 5589532. https://doi.org/10.1155/2021/5589532 [Google Scholar] [PubMed] [CrossRef]
Zhang X, Zhang X, Mao T, Xu H, Cui J, Lin H, Wang L (2021c). CSE1L, as a novel prognostic marker, promotes pancreatic cancer proliferation by regulating the AKT/mTOR signaling pathway. Journal of Cancer 12: 2797–2806. https://doi.org/10.7150/jca.54482 [Google Scholar] [PubMed] [CrossRef]
Zhao H, Chen G, Ye L, Yu H, Li S, Jiang WG (2019). DOK7V1 influences the malignant phenotype of lung cancer cells through PI3K/AKT/mTOR and FAK/paxillin signaling pathways. International Journal of Oncology 54: 381–389. https://doi.org/10.3892/ijo.2018.4624 [Google Scholar] [PubMed] [CrossRef]
Zhao X, Guan JL (2011). Focal adhesion kinase and its signaling pathways in cell migration and angiogenesis. Advanced Drug Delivery Reviews 63: 610–615. https://doi.org/10.1016/j.addr.2010.11.001 [Google Scholar] [PubMed] [CrossRef]
Zhao Z, Ma J, Mao Y, Dong L, Li S, Zhang Y (2018). Silence of α1-antitrypsin inhibits migration and proliferation of triple negative breast cancer cells. Medical Science Monitor 24: 6851–6860. https://doi.org/10.12659/MSM.910665 [Google Scholar] [PubMed] [CrossRef]
Zheng J, Liu C, Shi J, Wen K, Wang X (2022). AIM2 inhibits the proliferation, invasion and migration, and promotes the apoptosis of osteosarcoma cells by inactivating the PI3K/AKT/mTOR signaling pathway. Molecular Medicine Reports 25: 53. https://doi.org/10.3892/mmr.2021.12569 [Google Scholar] [PubMed] [CrossRef]
Zhou XM, Sun R, Luo DH, Sun J, Zhang MY, Wang MH, Yang Y, Wang HY, Mai SJ (2016). Upregulated TRIM29 promotes proliferation and metastasis of nasopharyngeal carcinoma via PTEN/AKT/mTOR signal pathway. Oncotarget 7: 13634–13650. https://doi.org/10.18632/oncotarget.7215 [Google Scholar] [PubMed] [CrossRef]
Zhou GZ, Sun YH, Shi YY, Zhang Q, Zhang L, Cui LQ, Sun GC (2021). ANXA8 regulates proliferation of human non-small lung cancer cells A549 via EGFR-AKT-mTOR signaling pathway. Molekuliarnaia Biologiia 55: 870–880. https://doi.org/10.31857/S0026898421050116 [Google Scholar] [PubMed] [CrossRef]
Zhu H, Liu Q, Tang J, Xie Y, Xu X, Huang R, Zhang Y, Jin K, Sun B (2017). Alpha1-ACT functions as a tumour suppressor in hepatocellular carcinoma by inhibiting the PI3K/AKT/mTOR signalling pathway via activation of PTEN. Cellular Physiology and Biochemistry 41: 2289–2306. https://doi.org/10.1159/000475648 [Google Scholar] [PubMed] [CrossRef]
Zou Z, Fan X, Liu Y, Sun Y, Zhang X, Sun G, Li X, Xu S (2020). Endogenous thrombopoietin promotes non-small-cell lung carcinoma cell proliferation and migration by regulating EGFR signalling. Journal of Cellular and Molecular Medicine 24: 6644–6657. https://doi.org/10.1111/jcmm.15314 [Google Scholar] [PubMed] [CrossRef]
Cite This Article
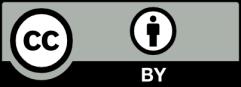