Open Access
ARTICLE
Vitamin B3 inhibits apoptosis and promotes autophagy of islet β cells under high glucose stress
1 Key Laboratory for Food Science and Biotechnology of Hunan Province, College of Food Science and Technology, Hunan Agricultural University, Changsha, 410128, China
2 Horticulture and Landscape College, Hunan Agricultural University, Changsha, 410128, China
3 Hunan Co-Innovation Center for Utilization of Botanical Functional Ingredients, Changsha, 410128, China
4 State Key Laboratory of Subhealth Intervention Technology, Changsha, 410128, China
5 Hunan Agricultural Products Processing Institute, Hunan Food Test and Analysis Center, Changsha, 410004, China
* Corresponding Author: YANYANG WU. Email:
BIOCELL 2023, 47(4), 859-868. https://doi.org/10.32604/biocell.2023.026429
Received 07 September 2022; Accepted 11 November 2022; Issue published 08 March 2023
Abstract
Background: Hyperglycemia is a typical symptom of diabetes. High glucose induces apoptosis of islet β cells. While autophagy functions in cytoprotection and autophagic cell death. The interaction between autophagy and apoptosis is important in the modulation of the function of islet β cells. Vitamin B3 can induce autophagy and inhibit islet β apoptosis.Method: The mechanism of vitamin B3-mediated protective effect on the function of islet β cells was explored by the method of western blot, immunofluorescence and flow cytometry.Results: In the present study, high glucose stress increased the apoptosis rate, while vitamin B3 reduced the apoptosis rate. The effect of vitamin B3 on autophagy flux under normal and high glucose stress was also investigated. Vitamin B3 increased the number of autophagosomes and increased the light chain (LC)3-II/LC3-I ratio. In contrast, vitamin B3 decreased sequestosome 1 (SQSTM1)/p62 protein expression and inhibited the phosphorylation of mammalian ribosomal protein S6 kinase β-1 (p70S6K/S6K1), which was a substrate of mammalian target of rapamycin (mTOR) under normal and high glucose stress. To further verify the protective effect of vitamin B3 on apoptosis, we treated islet β cell RIN-m5F with autophagy inhibitor 3-methyladenine (3-MA). Vitamin B3 decreased the apoptosis rate under high glucose stress, while the inhibition of apoptosis by vitamin B3 was blocked after adding 3-MA.Conclusion: Our data suggested that vitamin B3 reduced the apoptosis rate of β cells, possibly through inducing autophagy under high glucose stress.Keywords
Abbreviations
T2DM | type II diabetes mellitus |
T1DM | type I diabetes mellitus |
AMPK | AMP-activated protein kinase |
mTORC1 | mTOR complex 1 |
mTORC2 | mTOR complex 2 |
IGF1 | insulin-like growth factor-1 |
PI3K | phosphoinositide 3-kinase |
AKT | protein kinase B |
KD | Ketogenic diet |
Vitamin B3, referred to as niacin (NA) or niacinamide (NAM) (Chaykin, 1967), has a broad role in human health, such as attenuating skin aging and hyperpigmentation, as a potential drug to treat heart failure, promoting differentiation of the retinal pigment epithelium, protect neuronal cells, atherosclerosis, hypercholesterolemia, and diabetes (Abdellatif et al., 2021; Boo, 2021; Denu, 2005; Hazim et al., 2022; Mastropasqua et al., 2022). Diabetes is a syndrome characterized by hyperglycemia. A recent study showed that vitamin B3 promotes the release of C peptide and insulin, protects pancreatic β cell function, and reduces the risk of diabetes (Yilmaz et al., 2017); however, the associated molecular mechanism remains to be explored.
Diabetes is categorized as type I, type II, and gestational (Kitabchi et al., 2009). Type II diabetes mellitus (T2DM) is characterized by insulin resistance, which leads to a disorder of blood glucose metabolism. Due to insulin resistance, islet β cells have to secrete more insulin, eventually resulting in islet β cell failure (Gasmi et al., 2021; Marasco and Linnemann, 2018). Type I diabetes mellitus (T1DM) occurs from the damage of the β-cells mediated by the autoimmune process. Numerous experiments report that autophagy regulates glucose homeostasis and insulin resistance, averts β cell dysfunction, improves insulin sensitivity, and protects β cells from apoptosis during diabetes (Barlow and Thomas, 2015; Ebato et al., 2008; Li et al., 2018; Lim et al., 2018; Muralidharan and Linnemann, 2021; Zhang et al., 2015; Zhou et al., 2022). Autophagy is a conserved intracellular self-protection mechanism that functions directly in cell death and survival (Booth et al., 2014; Ploumi et al., 2022). Autophagy can be activated under various physiological conditions and is involved in the regulation of energy metabolism, stress response, pathogen clearance, and tumor occurrence and development (Shen et al., 2015). For example, it helps host cells degrade accumulated proteins, damaged mitochondria, and other aging or disordered organelles (Klionsky et al., 2021; Tu et al., 2013). Then small molecules such as amino acids can be produced, which is conducive to cell growth and ensures cell survival (Barlow and Thomas, 2015; Ebato et al., 2008; Ploumi et al., 2022; Zhang et al., 2015). Autophagy is essential, and up-regulation of autophagy activity may help to prevent the progression of diabetes. In diabetic human islets, progressive deterioration in β-cell function, reduction of glucose-stimulated insulin secretion, decreased β-cell mass, and increased β-cell apoptosis have been found (Del Prato et al., 2007; Butler et al., 2003; Sakuraba et al., 2002; Noa et al., 2007; Sidarala et al., 2020; UKPDS-Group, 1998).
Islet β cells are important cells in the human body that regulate blood glucose levels (Guo et al., 2019). Cell homeostasis is inseparable from autophagy. When autophagy is defective, the loss of the function of islet β cells can eventually lead to diabetes (Lytrivi et al., 2020; Yang et al., 2019). During hyperglycemia in patients with diabetes, normal autophagy of islet β cells can eliminate the large aggregation of ubiquitinated proteins (Jung, 2016). Insulin is produced mainly intracellularly in islet β cells. Under normal conditions, islet β cells are damaged or undergo apoptosis, further reducing insulin secretion (Chung et al., 2021). Studies have shown many reasons for islet β cell apoptosis, such as damage to the autoimmune system and imbalance of the autophagy regulation mechanism (Masini et al., 2009).
The current focus on pharmacological and nutritional research in the treatment of diabetes has shifted to the use of bioactive substances from natural sources (Wu et al., 2021). Diet intervention and exercise are the best programs for diabetes management. Vitamin B3, a natural product found in food, is an indispensable nutrient for humans and animals (Denu, 2005; Hrubsa et al., 2022). In this study, we constructed a hyperglycemic cell model and stressed islet β cells with vitamin B3 to explore the mechanism of vitamin B3 in the role of promoting the release of insulin. Our study also suggests that protecting Islet β cells by regulating autophagy through dietary vitamin B3 is an effective way of preventing the onset of diabetes.
Vitamin B3 (8060) was purchased from Beijing Solarbio Biological Technology Co., Ltd. (Shanghai, China). Anti-light chain 3 (LC3) polyclonal antibody (PM036), anti-LC3 monoclonal antibody (M186-3), and anti-sequestosome 1 (SQSTM1)/p62 (PM045) antibody were obtained from Medical Biological Laboratory (MBL, Japan). Anti-p70S6K (2708) and anti-phosphorylated p70S6K (9206) were obtained from Cell Signaling Technology (Beverly, MA). Anti-3-phosphoglyceraldehyde dehydrogenase (GAPDH) antibody (ZB002) was purchased from YTHX Biotechnology Co., Ltd. (Beijing, China). Propidium iodide (PI)-annexin V/fluorescein isothiocyanate (FITC) for flow cytometry was purchased from BD Biotechnology Research Co., Ltd. Goat anti-mouse IgG (1070-05) and goat anti-rabbit (4050-05) antibodies were purchased from Southern Biotechnology Company (Birmingham, UK). Alexa Fluor 488 Goat anti-Rabbit (A11034) antibody was obtained from Invitrogen Life Technology (Shanghai, China).
RIN-m5F cells were cultured in Roswell Park Memorial Institute-1640 medium with 10% fetal bovine serum (FBS) (BI, Israel) at 37°C, 5% CO2.
The RIN-m5F cells were incubated in 96-well plates for 24 h (37°C, 5% CO2). Then, cells were added with 10 μL Cell Counting Kit-8 (CCK-8) solution to each well after the cells were treated in different ways. Finally, the absorbance at 450 nm of each well was measured by a microplate reader. Each experiment was repeated at least three times.
RIN-m5F cells were cultured in 24-well plates with round cover glasses. The cells were washed with phosphate-buffered saline (PBS) three times and then soaked with 4% paraformaldehyde for 10 min. The cells were sealed with PBS containing 10% FBS for 30 min after washing three times with PBS. The cells were washed with PBS three times after incubation with an anti-LC3 antibody at 37°C for 1 h. Then, the cells were incubated with Alexa Fluor 488 goat anti-rabbit antibody at 37°C for 1 h and finally sealed with a fluorescence quencher. Images were observed with a confocal microscope (Zeiss LSM 710, Germany). Each experiment was repeated at least three times.
RIN-m5F cells were cultured in 6-well plates. Then, the medium was removed, and the cells were washed with PBS three times. The cells were treated with 2% sodium dodecyl sulfate (SDS; 200 μL per well). Then, the extract was heated at 100°C for 10 min and mixed with 6× protein loading buffer (Transgen; J21020). The proteins in this extract were separated with sodium dodecyl sulfate-polyacrylamide gel (4% stacking gel, 15% separating gel, 50 V for 30 min, 90 V for 120 min) electrophoresis after the extract was heated for 10 min at 100°C and then transferred to a nitrocellulose membrane (250 mA, 30–60 min). The membranes were sealed with 5% skimmed milk powder for 1 h and incubated overnight with primary antibody at 4°C. The membranes were incubated with a secondary antibody for 1 h after washing with PBST (PBS plus 0.2% Tween-20) three times. Then the membranes were visualized, and images were acquired with a luminescent image analyzer (Model: Image Quant LAS4000 Mini, Serial No. 3614294; GE Healthcare Bio-Sciences AB, Uppsala, Sweden) after incubation for a few minutes with WesternBrightTM ECL chemiluminescent HRP substrate (SuperSignal West Dura, 32106, Thermo Pierce). Each experiment was repeated at least three times.
Annexin V-FITC-PI Apoptosis Detection Kit was used for the analysis of apoptotic cells by flow cytometry. Rin-m5F cells were cultured in 12-well plates for 24–36 h, then washed with PBS and digested with trypsin. The cells were obtained by centrifugation and suspended in 100 μL binding buffer (10e6 cells/mL). Each tube was stained with 5 μL annexin V-FITC and 5 μL PI for 15 min, and then 400 μL binding buffer was added. The intensity of these cells was analyzed with flow cytometry (Beckman MoFlo XDP, USA). Each experiment was repeated at least three times.
Protective effect of vitamin B3 on apoptosis of RIN-m5F cells under high glucose stress
We observed that 20 mM glucose increased the apoptosis rate from 8.46% to 18.71%, while 20 μM vitamin B3 reduced the apoptosis rate of RIN-m5F cells from 18.71% to 11.03% (Figs. 1a–1e).
Figure 1: Vitamin B3 protects cells from apoptosis induced by high glucose. (a) Molecular structure of vitamin B3. (b) Apoptosis rates of RIN-m5F cells cultured with or without vitamin B3 at 20 μmol/L for 36 h under high glucose stress; the rate of apoptosis was measured by flow cytometry. (c–e) Total apoptosis, late apoptosis, and early apoptosis in (a) were statistically analyzed. The experiment was repeated at least three times (**p < 0.01 compared to the control group by one-way ANOVA, ##p < 0.01 compared to the high glucose-treated group by one-way ANOVA).
Our data showed that 20 μM vitamin B3 had no effect on the cell viability of RIN-m5F cells, while 40 and 80 μM vitamin B3 decreased the cell viability of RIN-m5F cells from 89.68% to 78.91% and 53.06%, respectively (Fig. 2a). So, in the following experiments, the cells were treated with 20 μM vitamin B3 to test the effect of vitamin B3-induced autophagy on RIN-m5F cell function. The number of autophagosomes is usually an indicator of autophagy. Autophagosomes accumulated even under normal conditions once lysosomal enzyme activity was reduced. To estimate autophagic activity, we used an immunofluorescence assay to analyze changes in the number of autophagosomes in RIN-m5F cells treated with 20 μM vitamin B3. The number of RIN-m5F cell autophagosomes increased from 5 to 10 after treatment with vitamin B3 for 24 h (Figs. 2b and 2c). Our data also showed that vitamin B3 increased the LC3-II/LC3-I ratio and degradation rates of SQSTM1/p62 (Figs. 2d–2g). The phosphorylation activity of phospho-p70 S6K was also decreased (Figs. 2h and 2i).
Figure 2: Vitamin B3 induced autophagy in RIN-m5F cells. (a) RIN-m5F cells were treated with 20, 40, and 80 μmol/L vitamin B3 and the cell viability was tested by the method of CCK-8. (b) RIN-m5F cells were treated with 20 μmol/L vitamin B3 for 24 h and stained with an anti-LC3 antibody (scale bar = 5 μm). (c) The cells were treated as in (b) to calculate the number of autophagosomes per cell. At least 30 cells were counted through t-test analysis, and the experiment was repeated at least three times (**p < 0.01 compared to the control group by one-way ANOVA *p < 0.05 compared to the control group by one-way ANOVA). (d), (f), and (h) The cells were treated as in (b) and a western blot was performed to test the expression of SQSTM1/P62, S6K, P-P70S6K, LC3, and GAPDH with specific antibodies of anti-SQSTM1/P62, anti-S6K, anti-P-P70S6K, anti-LC3, and anti-GAPDH. (e), (g), and (i) The ratios of LC3-II/LC3-I, P62/GAPDH, or pS6K/S6K for each sample in (d), (f), or (h) were tested by integrated optical density (IOD) using Image-pro Plus 6.0 software. The experiment was repeated at least three times. GAPDH, glyceraldehyde 3-phosphate dehydrogenase; P-S6K, phosphorylation of 70 KDa mammalian ribosomal protein S6 kinase β-1; LC3, light chain 3; SQSTM1/p62, Sequestosome 1. Data are presented as the mean ± S.D., *p < 0.05, **p < 0.01.
Vitamin B3-induced autophagy under high glucose stress
Glucose at 10 and 20 mM had no effect on cell viability; however, after treatment with 30 mM glucose, cell viability decreased (Fig. 3a). Then, the RIN-m5F cells were treated with 10 and 20 mM glucose and immunofluorescence assay showed that the number of autophagosomes and the ratio of LC3-II/LC3-I did not change at the mentioned glucose concentrations (Figs. 3b–3e). Our data also showed that the degradation rates of SQSTM1/p62 and the phosphorylation of p70 S6K did not decrease in the 10 or 20 mM glucose-treated groups (Figs. 3f–3i). The cells were thus treated with 20 mM glucose in the following experiments to simulate high glucose stress.
Figure 3: The effect of high glucose on autophagy in RIN-m5F cells. (a) Cells were treated with indicated concentrations of glucose, and the cytotoxicity was tested using a CCK-8 kit. (b) Cells were cultured with 10 or 20 mmol/L glucose for 36 h, and the effect of glucose on autophagy was verified by immunofluorescence. The anti-LC3 polyclonal antibody was used in this experiment. (c) Cells were treated as in (b) and the number of autophagosomes per cell was statistically estimated; at least 30 cells were counted through t-test analysis. The experiment was repeated at least three times (**p < 0.01, *p < 0.05). (d), (f), and (h) The cells were treated with 10 or 20 mmol/L glucose for 36 h, and a western blot was performed to test the expression of SQSTM1/P62, S6K, P-P70S6K, LC3, and GAPDH with specific antibodies of anti-SQSTM1/P62, anti-S6K, anti-P-P70S6K, anti-LC3, and anti-GAPDH. (e), (g), and (i) The ratios of LC3-II/LC3-I, P62/GAPDH or pS6K/S6K for each sample in (d), (f), or (h) were tested by (integrated optical density) IOD using Image-pro Plus 6.0 software. The experiment was repeated at least three times. GAPDH, glyceraldehyde 3-phosphate dehydrogenase; P-S6K, phosphorylation of 70 KDa mammalian ribosomal protein S6 kinase β-1; LC3, light chain 3; SQSTM1/p62, Sequestosome 1.
To test the effect of vitamin B3 on autophagy under high glucose stress, the cells were treated with 20 μM vitamin B3 under high glucose stress for 36 h. The number of autophagosomes increased with the increased dose of vitamin B3 under high glucose stress (Figs. 4a and 4b), the ratio of LC3-II/LC3-I, degradation rates of SQSTM1/p62 increased, and phospho-p70 S6K decreased with increased vitamin B3 concentration (Figs. 4c–4h). The ratio of LC3-II and the number of autophagosomes decreased in the 3-MA plus vitamin B3-treated group compared with the vitamin B3-treated group (Figs. 5a–5d).
Figure 4: Vitamin B3 induced autophagy under high glucose stress. (a) The cells were treated with 20 μmol/L vitamin B3 for 36 h under 20 mM high glucose stress, and the number of autophagosomes was determined by immunofluorescence. (b) Cells were treated as in (a) and statistics were performed to calculate the number of autophagosomes per cell, and at least 30 cells were counted through t-test analysis. The experiment was repeated at least three times (**p < 0.01, *p < 0.05 compared to the control group by one-way ANOVA). (c), (e), and (g) The cells were treated with 20 mmol/L glucose for 36 h, and the expression of SQSTM1/P62, S6K, P-P70S6K, LC3, and GAPDH was detected by western blotting using specific antibodies of anti-SQSTM1/P62, anti-S6K, anti-P-P70S6K, anti-LC3, and anti-GAPDH. (d), (f), and (h) The ratios of LC3-II/LC3-I, P62/GAPDH or pS6K/S6K for each sample in (c), (e), or (g) were tested by (integrated optical density) IOD using Image-pro Plus 6.0 software. The experiment was repeated at least three times. GAPDH, glyceraldehyde 3-phosphate dehydrogenase; P-S6K, phosphorylation of 70 KDa mammalian ribosomal protein S6 kinase β-1; LC3, light chain 3; SQSTM1/p62, Sequestosome 1.
Figure 5: 3-Methyladenine (3-MA) inhibited vitamin B3-induced autophagy under high glucose stress. (a) RIN-m5F cells were cultured with or without 20 mmol/L glucose, 10 mmol/L 3-MA, 20 mmol/L glucose plus 10 mmol/L 3-MA, 20 μmol/L vitamin B3, 20 mmol/L glucose plus 20 μmol/L vitamin B3, 20 mmol/L glucose plus 20 μmol/L vitamin B3 plus 10 mmol/L 3-MA for 36 h and stained with an anti-LC3 antibody (scale bar = 5 μm). (b) Cells were treated as in (a) and the number of autophagosomes per cell was counted. At least 30 cells were analyzed. The experiment was repeated at least three times (**p < 0.01, *p < 0.05 compared to the control group by one-way ANOVA). (c) The cells were treated with 20 mmol/L glucose for 36 h, and the expression of LC3 and GAPDH was determined by western blotting using specific antibodies of anti-LC3 and anti-GAPDH. (d) The ratio of LC3-II/LC3-I for each sample in (c) was tested by (integrated optical density) IOD using Image-pro Plus 6.0 software. The experiment was repeated at least three times.
Protective effect of vitamin B3 on apoptosis of RIN-m5F cells under high glucose stress
Our data showed that vitamin B3 reduced the total apoptosis rate of β cells from 18.05% to 10.25% under high glucose stress (Figs. 6a and 6b). The total apoptosis rate of β cells increased from 10.25% to 23.17% after the cells were treated with 3-MA (Figs. 6a and 6b). Similar phenomena were observed in early and late apoptosis rates (Figs. 6c and 6d).
Figure 6: Vitamin B3 protects RIN-m5F cells from apoptosis via inducing autophagy under high glucose stress. (a) RIN-m5F cells were cultured with 20 mmol/L glucose plus 10 mmol/L 3-MA, 20 mmol/L glucose plus 20 μmol/L vitamin B3, or 20 mmol/L glucose plus 10 mmol/L 3-MA plus 20 μmol/L vitamin B3 and the apoptosis rate of the cells was measured by flow cytometry. (b–d) Cells were treated as in (a) and total apoptosis, late apoptosis, and early apoptosis in (a) were statistically analyzed. The experiment was repeated at least three times (**p < 0.01 compared to the control group by one-way ANOVA, ##p < 0.01 compared to the high glucose-treated group by one-way ANOVA).
Diabetes is one of the most prevalent diseases in the world and is a serious public health threat. Drug therapy and dietary interventions are effective ways to treat diabetes. Metformin, sulfonylureas, insulin, and other drugs can significantly relieve T2DM with a hypoglycemic effect, but they cannot prevent islet cell failure (Kitazawa et al., 2021; Taylor et al., 2021). Research on the dietary intervention of diabetes has been increasing rapidly, with multiple natural and nutritional products thought to benefit the treatment of diabetes. For example, mangiferin significantly reduces glucose levels and prevents tissue damage in animal models of diabetes (Wu et al., 2021). Intervention with low-calorie Mediterranean-style and low-carbohydrate dietary regimens can effectively reduce insulin resistance, alleviate fasting hyperinsulinemia, and recover the function of β cells in patients with T2DM (Bolla et al., 2019; Karatzi and Manios, 2021). Vitamin B3, a natural nutrient in foods, can also recover the function of β cells by promoting the release of C-peptide and insulin, reducing the risk of type I diabetes mellitus (T1DM) (Yilmaz et al., 2017). Vitamin B3 is also involved in regulating several redox and non-redox reactions of cell energy metabolism (Dudev and Lim, 2010), which can prevent oxidative stress and improve cell survival rate, and may function in ameliorating T2DM (Yilmaz et al., 2017). Vitamin B3 has been reported to induce autophagy via an mTOR-dependent pathway, regulating the expression of autophagy-related ATG5 protein, blocking oxidative stress, and inhibiting the activity of silent mating type information regulation 2 homolog 1 (SIRT1) (Li et al., 2019; Maiese, 2021; Oblong et al., 2020; Shen et al., 2017b). Here, we found that vitamin B3 had a limited protective effect on β islet cells. However, the molecular mechanism was unknown.
Hyperglycemia is a typical symptom of diabetes. High glucose can cause complications of diabetes, such as diabetic nephropathy and cardiovascular disease (Hou et al., 2022; Kang et al., 2020a). It also induces ferroptosis by facilitating glutathione peroxidase 4 ubiquitination, promotes necroptosis via inhibition activity of aldehyde dehydrogenases 2, increases NETosis, apoptosis, and pyroptosis (Han et al., 2022; Kang et al., 2020b; Menegazzo et al., 2015; Zhang et al., 2021). Thus, high glucose-induced apoptosis of β cells and the mechanism needed to be explored.
Autophagy is regulated by different signaling pathways, including the mTOR/AMP-activated protein kinase (AMPK)-dependent and -independent signaling pathways. Hyperglycemia can activate the mTOR signaling pathway by inhibiting autophagy (Ding and Choi, 2015). The targets of mTOR are classical nutritional pathways that regulate autophagy by forming two complexes, mTOR complex 1 (mTORC1) and mTORC2. MTORC1 inhibits the activity of Unc-51-like autophagy activating kinase (ULK1) through direct phosphorylation of ULK1, thus inhibiting the expression of autophagy-related proteins LC3-II and Beclin-1 (Dehdashtian et al., 2018). When glucose is insufficient, AMPK promotes autophagy by directly activating ULKl through the phosphorylation of Ser 317 and Ser 777 (Gödel et al., 2011). In contrast, increased mTOR or protein kinase B (AKT) activity under high glucose results in ULKl phosphorylation and inhibits the activity of ULK1, thereby disrupting the interaction between ULK1 and AMPK and then blocking autophagy (Kubli and Gustafsson, 2014; Yao et al., 2016). Natural products and diet interventions have been reported to effectively alleviate diabetes through the mTOR signaling pathway. For example, isorhamnetin and metformin ameliorate insulin resistance associated with T2DM through the mTOR signaling pathway (Taylor et al., 2021). A ketogenic diet (KD) lowers diabetic hyperketonemia by regulating glucose metabolism and suppressing insulin-like growth factor-1 and phosphoinositide 3-kinase/AKT/mTOR pathways (Kumar et al., 2021). Nicotinamide, as an inhibitor of sirtuin pathways, has been shown to protect hepatocytes against palmitate-induced lipotoxicity via SIRT1-dependent autophagy induction. Nicotinamide can also inhibit the phosphorylated activity of mTOR (Maiese, 2020; Shen et al., 2017a). In the present study, we found that vitamin B3-induced autophagy might occur via an mTOR-dependent pathway, and the protection of islet cells is limited. However, partial recovery of β-cell function is also of great significance in alleviating diabetes. Therefore, its mechanism and in vivo study need to be further explored.
Funding Statement: This paper was supported by the National-Natural Science Foundation of China (32072334), the General Project of the Education Department of Hunan Province (20C0959), and the Changsha Natural Science Foundation (kq2007020).
Author Contributions: Yanyang Wu and Yu Zhang conceived and designed the experiments; Yu Zhang performed the experiments; Yu Zhang and Xi’an Zhou analyzed the data; Yanyang Wu and Dongbo Liu contributed reagents/materials/analysis tools; Yu Zhang wrote the paper.
Availability of Data and Materials: All data generated or analyzed during this study are included in this published article (and its supplementary information files).
Ethics Approval: Not applicable.
Conflicts of Interest: The authors declare that they have no conflicts of interest to report regarding the present study.
References
Abdellatif M, Trummer-Herbst V, Koser F, Durand S, Adao R, Vasques-Novoa F, Freundt JK, Voglhuber J, Pricolo MR, Kasa M (2021). Nicotinamide for the treatment of heart failure with preserved ejection fraction. Science Translational Medicine 13: eabd7064. https://doi.org/10.1126/scitranslmed.abd7064 [Google Scholar] [PubMed] [CrossRef]
Barlow AD, Thomas DC (2015). Autophagy in diabetes: β-cell dysfunction, insulin resistance, and complications. DNA Cell Biology 34: 252–260. https://doi.org/10.1089/dna.2014.2755 [Google Scholar] [PubMed] [CrossRef]
Bolla AM, Caretto A, Laurenzi A, Scavini M, Piemonti L (2019). Low-carb and ketogenic diets in type 1 and type 2 diabetes. Nutrients 11: 962. https://doi.org/10.3390/nu11050962 [Google Scholar] [PubMed] [CrossRef]
Boo YC (2021). Mechanistic Basis and clinical evidence for the applications of nicotinamide (niacinamide) to control skin aging and pigmentation. Antioxidants 10: 1315. https://doi.org/10.3390/antiox10081315 [Google Scholar] [PubMed] [CrossRef]
Booth LA, Tavallai S, Hamed HA, Cruickshanks N, Dent P (2014). The role of cell signalling in the crosstalk between autophagy and apoptosis. Cell Signal 26: 549–555. https://doi.org/10.1016/j.cellsig.2013.11.028 [Google Scholar] [PubMed] [CrossRef]
Butler AE, Janson J, Bonner-Weir S, Ritzel R, Rizza RA, Butler PC (2003). β-cell deficit and increased β-cell apoptosis in humans with type 2 diabetes. Diabetes 52: 102–110. https://doi.org/10.2337/diabetes.52.1.102 [Google Scholar] [PubMed] [CrossRef]
Chaykin S (1967). Nicotinamide coenzymes. Annual Review of Biochemistry 36: 149–170. https://doi.org/10.1146/annurev.bi.36.070167.001053 [Google Scholar] [PubMed] [CrossRef]
Chung H, Nam H, Nguyen-Phuong T, Jang J, Hong SJ, Choi SW, Park SB, Park CG (2021). The blockade of cytoplasmic HMGB1 modulates the autophagy/apoptosis checkpoint in stressed islet beta cells. Biochemical and Biophysical Research Communications 534: 1053–1058. https://doi.org/10.1016/j.bbrc.2020.10.038 [Google Scholar] [PubMed] [CrossRef]
Dehdashtian E, Mehrzadi S, Yousefi B, Hosseinzadeh A, Reiter RJ, Safa M, Ghaznavi H, Naseripour M (2018). Diabetic retinopathy pathogenesis and the ameliorating effects of melatonin; involvement of autophagy, inflammation and oxidative stress. Life Science 193: 20–33. https://doi.org/10.1016/j.lfs.2017.12.001 [Google Scholar] [PubMed] [CrossRef]
Del Prato S, Bianchi C, Marchetti P (2007). β-cell function and anti-diabetic pharmacotherapy. Diabetes Metabolism Research and Reviews 23: 518–527. https://doi.org/10.1002/(ISSN)1520-7560 [Google Scholar] [CrossRef]
Denu JM (2005). Vitamin B3 and sirtuin function. Trends in Biochemical Sciences 30: 479–483. https://doi.org/10.1016/j.tibs.2005.07.004 [Google Scholar] [PubMed] [CrossRef]
Ding Y, Choi ME (2015). Autophagy in diabetic nephropathy. The Journal of Endocrinology 224: R15–R30. https://doi.org/10.1530/JOE-14-0437 [Google Scholar] [PubMed] [CrossRef]
Dudev T, Lim C (2010). Factors controlling the mechanism of NAD+ non-redox reactions. Journal of the American Chemical Society 132: 16533–16543. https://doi.org/10.1021/ja106600k [Google Scholar] [PubMed] [CrossRef]
Ebato C, Uchida T, Arakawa M, Komatsu M, Ueno T, Komiya K, Azuma K, Hirose T, Tanaka K, Kominami E (2008). Autophagy is important in islet homeostasis and compensatory increase of beta cell mass in response to high-fat diet. Cell Metabolism 8: 325–332. https://doi.org/10.1016/j.cmet.2008.08.009 [Google Scholar] [PubMed] [CrossRef]
Gasmi A, Noor S, Menzel A, Dosa A, Pivina L, Bjorklund G (2021). Obesity and insulin resistance: Associations with chronic inflammation, genetic and epigenetic factors. Current Medicinal Chemistry 28: 800–826. https://doi.org/10.2174/0929867327666200824112056 [Google Scholar] [PubMed] [CrossRef]
Guo J, Liu Z, Gong R (2019). Long noncoding RNA: An emerging player in diabetes and diabetic kidney disease. Clinical Science 133: 1321–1339. https://doi.org/10.1042/CS20190372 [Google Scholar] [PubMed] [CrossRef]
Gödel M, Hartleben B, Herbach N, Liu S, Zschiedrich S, Lu S, Debreczeni-Mór A, Lindenmeyer MT, Rastaldi MP, Hartleben G (2011). Role of mTOR in podocyte function and diabetic nephropathy in humans and mice. The Journal of Clinical Investigation 121: 2197–2209. https://doi.org/10.1172/JCI44774 [Google Scholar] [PubMed] [CrossRef]
Han N, Wang Z, Luo H, Chi Y, Zhang T, Wang B, Li Y (2022). Effect and mechanism of TFEB on pyroptosis in HK-2 cells induced by high glucose. Biochemical and Biophysical Research Communications 610: 162–169. https://doi.org/10.1016/j.bbrc.2022.04.062 [Google Scholar] [PubMed] [CrossRef]
Hazim RA, Paniagua AE, Tang L, Yang K, Kim KKO, Stiles L, Divakaruni AS, Williams DS (2022). Vitamin B3, nicotinamide, enhances mitochondrial metabolism to promote differentiation of the retinal pigment epithelium. The Journal of Biological Chemistry 298: 102286. https://doi.org/10.1016/j.jbc.2022.102286 [Google Scholar] [PubMed]
Hou W, Lu L, Li X, Sun M, Zhu M, Miao C (2022). c-Myc participates in high glucose-mediated endothelial inflammation via upregulation of IRAK1 expression in diabetic nephropathy. Cellular Signalling 92: 110263. https://doi.org/10.1016/j.cellsig.2022.110263 [Google Scholar] [PubMed] [CrossRef]
Hrubsa M, Siatka T, Nejmanova I, Voprsalova M, Kujovska Krcmova L, Matousova K, Javorska L, Macakova K, Mercolini L, Remiao F (2022). Biological properties of vitamins of the B-complex, part 1: Vitamins B1, B2, B3, and B5. Nutrients 14: 484. https://doi.org/10.3390/nu14030484 [Google Scholar] [PubMed] [CrossRef]
Jung HS (2016). Autophagy: Starved β-cells seem different from starved body. Journal of Diabetes Investigation 7: 169–170. https://doi.org/10.1111/jdi.12396 [Google Scholar] [PubMed] [CrossRef]
Kang P, Wang J, Fang D, Fang T, Yu Y, Zhang W, Shen L, Li Z, Wang H, Ye H (2020a). Activation of ALDH2 attenuates high glucose induced rat cardiomyocyte fibrosis and necroptosis. Free Radical Biology & Medicine 146: 198–210. https://doi.org/10.1016/j.freeradbiomed.2019.10.416 [Google Scholar] [PubMed] [CrossRef]
Kang PF, Wang JH, Fang DA, Fang TT, Yu Y, Zhang WP, Shen L, Li ZH, Wang HJ, Ye HW (2020b). Activation of ALDH2 attenuates high glucose induced rat cardiomyocyte fibrosis and necroptosis. Free Radical Biology & Medicine 146: 198–210. https://doi.org/10.1016/j.freeradbiomed.2019.10.416 [Google Scholar] [PubMed] [CrossRef]
Karatzi K, Manios Y (2021). The role of lifestyle, eating habits and social environment in the prevention and treatment of type 2 diabetes and hypertension. Nutrients 13: 1460. https://doi.org/10.3390/nu13051460 [Google Scholar] [PubMed] [CrossRef]
Kitabchi AE, Umpierrez GE, Miles JM, Fisher JN (2009). Hyperglycemic crises in adult patients with diabetes. Diabetes Care 32: 1335–1343. https://doi.org/10.2337/dc09-9032 [Google Scholar] [PubMed] [CrossRef]
Kitazawa M, Katagiri T, Suzuki H, Matsunaga S, Yamada MH, Ikarashi T, Yamamoto M, Furukawa K, Iwanaga M, Hatta M (2021). A 52-week randomized controlled trial of ipragliflozin or sitagliptin in type 2 diabetes combined with metformin: The N-ISM study. Diabetes, Obesity & Metabolism 23: 811–821. https://doi.org/10.1111/dom.14288 [Google Scholar] [PubMed] [CrossRef]
Klionsky DJ, Petroni G, Amaravadi RK, Baehrecke EH, Ballabio A, Boya P, Bravo-San Pedro JM, Cadwell K, Cecconi F, Choi AMK (2021). Autophagy in major human diseases. The EMBO Journal 40: e108863. https://doi.org/10.15252/embj.2021108863 [Google Scholar] [PubMed] [CrossRef]
Kubli DA, Gustafsson AB (2014). Cardiomyocyte health: Adapting to metabolic changes through autophagy. Trends in Endocrinology and Metabolism 25: 156–164. https://doi.org/10.1016/j.tem.2013.11.004 [Google Scholar] [PubMed] [CrossRef]
Kumar S, Behl T, Sachdeva M, Sehgal A, Kumari S, Kumar A, Kaur G, Yadav HN, Bungau S (2021). Implicating the effect of ketogenic diet as a preventive measure to obesity and diabetes mellitus. Life Science 264: 118661. https://doi.org/10.1016/j.lfs.2020.118661 [Google Scholar] [PubMed] [CrossRef]
Li B, Wu X, Chen H, Zhuang C, Zhang Z, Yao S, Cai D, Ning G, Su Q (2018). miR199a-5p inhibits hepatic insulin sensitivity via suppression of ATG14-mediated autophagy. Cell Death & Disease 9: 405. https://doi.org/10.1038/s41419-018-0439-7 [Google Scholar] [PubMed] [CrossRef]
Li W, Zhu L, Ruan ZB, Wang MX, Ren Y, Lu W (2019). Nicotinamide protects chronic hypoxic myocardial cells through regulating mTOR pathway and inducing autophagy. European Review for Medical and Pharmacological Sciences 23: 5503–5511. https://doi.org/10.26355/eurrev_201906_18220 [Google Scholar] [PubMed] [CrossRef]
Lim H, Lim YM, Kim KH, Jeon YE, Park K, Kim J, Hwang HY, Lee DJ, Pagire H, Kwon HJ (2018). A novel autophagy enhancer as a therapeutic agent against metabolic syndrome and diabetes. Nature Communications 9: 1438. https://doi.org/10.1038/s41467-018-03939-w [Google Scholar] [PubMed] [CrossRef]
Lytrivi M, Castell AL, Poitout V, Cnop M (2020). Recent insights into mechanisms of β-cell lipo- and glucolipotoxicity in type 2 diabetes. Journal of Molecular Biology 432: 1514–1534. https://doi.org/10.1016/j.jmb.2019.09.016 [Google Scholar] [PubMed] [CrossRef]
Maiese K (2020). New Insights for nicotinamide metabolic disease autophagy and mTOR. Frontiers in Bioscience 25: 1925–1973. https://doi.org/10.2741/4886 [Google Scholar] [PubMed] [CrossRef]
Maiese K (2021). Nicotinamide: Oversight of metabolic dysfunction through SIRT1, mTOR, and clock genes. Current Neurovascular Research 17: 765–783. https://doi.org/10.2174/18755739MTEx2NDIjx [Google Scholar] [CrossRef]
Marasco MR, Linnemann AK (2018). β-cell autophagy in diabetes pathogenesis. Endocrinology 159: 2127–2141. https://doi.org/10.1210/en.2017-03273 [Google Scholar] [PubMed] [CrossRef]
Masini M, Bugliani M, Lupi R, Guerra SD, Boggi U, Filipponi F, Marselli L, Marchetti PM (2009). Autophagy in human type 2 diabetes pancreatic beta cells. Diabetologia 52: 1083–1086. https://doi.org/10.1007/s00125-009-1347-2 [Google Scholar] [PubMed] [CrossRef]
Mastropasqua L, Agnifili L, Ferrante C, Sacchi M, Figus M, Rossi GCM, Brescia L, Aloia R, Orlando G (2022). Citicoline/Coenzyme Q10/Vitamin B3 fixed combination exerts synergistic protective effects on neuronal cells exposed to oxidative stress. Nutrients 14. https://doi.org/10.3390/nu14142963 [Google Scholar] [PubMed] [CrossRef]
Menegazzo L, Ciciliot S, Poncina N, Mazzucato M, Persano M, Bonora B, Albiero M, de Kreutzenberg SV, Avogaro A, Fadini GP (2015). NETosis is induced by high glucose and associated with type 2 diabetes. Acta Diabetologica 52: 497–503. https://doi.org/10.1007/s00592-014-0676-x [Google Scholar] [PubMed] [CrossRef]
Muralidharan C, Linnemann AK (2021). β-cell autophagy in the pathogenesis of type 1 diabetes. American Journal of Physiology. Endocrinology and Metabolism 321: E410–E416. https://doi.org/10.1152/ajpendo.00151.2021 [Google Scholar] [PubMed] [CrossRef]
Noa W, Limor O-Y, Sarah K, Shimon E, Yuval D (2007). Lineage tracing evidence for in vitro dedifferentiation but rare proliferation of mouse pancreatic β-cells. Diabetes 56: 1299–1304. https://doi.org/10.2337/db06-1654 [Google Scholar] [PubMed] [CrossRef]
Oblong JE, DeAngelis YM, Jarrold BB, Bierman JC, Rovito HA, Vires L, Fang B, Laughlin T, Zhao W, Hartman SM (2020). Optimized low pH formulation of niacinamide enhances induction of autophagy marker ATG5 gene expression and protein levels in human epidermal keratinocytes. Journal of the European Academy of Dermatology and Venereology: JEADV 34: 3–11. https://doi.org/10.1111/jdv.16582 [Google Scholar] [PubMed] [CrossRef]
Ploumi C, Papandreou ME, Tavernarakis N (2022). The complex interplay between autophagy and cell death pathways. The Biochemical Journal 479: 75–90. https://doi.org/10.1042/BCJ20210450 [Google Scholar] [PubMed] [CrossRef]
Sakuraba H, Mizukami H, Yagihashi N, Wada R, Hanyu C, Yagihashi S (2002). Reduced beta-cell mass and expression of oxidative stress-related DNA damage in the islet of Japanese type II diabetic patients. Diabetologia 45: 85–96. [Google Scholar] [PubMed]
Shen C, Dou X, Ma Y, Ma W, Li S, Song Z (2017a). Nicotinamide protects hepatocytes against palmitate-induced lipotoxicity via SIRT1-dependent autophagy induction. Nutrition Research 40: 40–47. https://doi.org/10.1016/j.nutres.2017.03.005 [Google Scholar] [PubMed] [CrossRef]
Shen C, Dou XB, Ma Y, Ma W, Li ST, Song ZY (2017b). Nicotinamide protects hepatocytes against palmitate-induced lipotoxicity via SIRT1-dependent autophagy induction. Nutrition Research 40: 40–47. https://doi.org/10.1016/j.nutres.2017.03.005 [Google Scholar] [PubMed] [CrossRef]
Shen H, Zhao S, Xu Z, Zhu L, Han Y, Ye J (2015). Evodiamine inhibits proliferation and induces apoptosis in gastric cancer cells. Oncology Letters 10: 367–371. https://doi.org/10.3892/ol.2015.3153 [Google Scholar] [PubMed] [CrossRef]
Sidarala V, Pearson GL, Parekh VS, Thompson B, Christen L, Gingerich MA, Zhu J, Stromer T, Ren J, Reck EC (2020). Mitophagy protects beta cells from inflammatory damage in diabetes. Journal of Clinical Investigation Insight 5: e141138. https://doi.org/10.1101/2020.06.07.138917 [Google Scholar] [CrossRef]
Taylor SI, Yazdi ZS, Beitelshees AL (2021). Pharmacological treatment of hyperglycemia in type 2 diabetes. The Journal of Clinical Investigation 131: e142243. https://doi.org/10.1172/JCI142243 [Google Scholar] [PubMed] [CrossRef]
Tu YJ, Fan X, Yang X, Zhang C, Liang HP (2013). Evodiamine activates autophagy as a cytoprotective response in murine Lewis lung carcinoma cells. Oncology Reports 29: 481–490. https://doi.org/10.3892/or.2012.2125 [Google Scholar] [PubMed] [CrossRef]
UKPDS-Group (1998). Intensive blood-glucose control with sulphonylureas or insulin compared with conventional treatment and risk of complications in patients with type 2 diabetes prospective diabetes study (UKPDS) Group. Lancet 352: 837–853. https://doi.org/10.1016/S0140-6736(98)07019-6 [Google Scholar] [CrossRef]
Wu Y, Liu W, Yang T, Li M, Qin L, Wu L, Liu T (2021). Oral administration of mangiferin ameliorates diabetes in animal models: A meta-analysis and systematic review. Nutrition Research 87: 57–69. https://doi.org/10.1016/j.nutres.2020.12.017 [Google Scholar] [PubMed] [CrossRef]
Yang Y, Chen Q, Zhao Q, Luo Y, Xu Y, Du W, Wang H, Li H, Yang L, Hu C (2019). Inhibition of COX2/PGD2-related autophagy is involved in the mechanism of brain injury in T2DM rat. Frontiers in Cellular Neuroscience 13: 68. https://doi.org/10.3389/fncel.2019.00068 [Google Scholar] [PubMed] [CrossRef]
Yao F, Zhang M, Chen L (2016). 5′-Monophosphate-activated protein kinase (AMPK) improves autophagic activity in diabetes and diabetic complications. Acta pharmaceutica Sinica B 6: 20–25. https://doi.org/10.1016/j.apsb.2015.07.009 [Google Scholar] [PubMed] [CrossRef]
Yilmaz Z, Piracha F, Anderson L, Mazzola N (2017). Supplements for diabetes mellitus: A review of the literature. Journal of Pharmacy Practice 30: 631–638. https://doi.org/10.1177/0897190016663070 [Google Scholar] [PubMed] [CrossRef]
Zhang N, Cao MM, Liu H, Xie GY, Li YB (2015). Autophagy regulates insulin resistance following endoplasmic reticulum stress in diabetes. Journal of Physiology and Biochemistry 71: 319–327. https://doi.org/10.1007/s13105-015-0384-1 [Google Scholar] [PubMed] [CrossRef]
Zhang JF, Qiu QH, Wang HY, Chen C, Luo DW (2021). TRIM46 contributes to high glucose-induced ferroptosis and cell growth inhibition in human retinal capillary endothelial cells by facilitating GPX4 ubiquitination. Experimental Cell Research 407: 112800. https://doi.org/10.1016/j.yexcr.2021.112800 [Google Scholar] [PubMed] [CrossRef]
Zhou F, Yang LL, Yang LQ, Wang X, Guo N, Sun WW, Ma HJ (2022). Trpc5-regulated AMPKα/mTOR autophagy pathway is associated with glucose metabolism disorders in low birth weight mice under overnutrition. Biochemical and Biophysical Research Communications 630: 1–7. https://doi.org/10.1016/j.bbrc.2022.09.045 [Google Scholar] [PubMed] [CrossRef]
Cite This Article
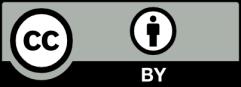
This work is licensed under a Creative Commons Attribution 4.0 International License , which permits unrestricted use, distribution, and reproduction in any medium, provided the original work is properly cited.