Open Access
REVIEW
Research progress of protein phosphatase 2A in cellular autophagy
Department of Health Toxicology, School of Public Health, Guilin Medical University, Guilin, 541199, China
* Corresponding Author: XIAONIAN ZHU. Email:
(This article belongs to the Special Issue: )
BIOCELL 2023, 47(3), 485-491. https://doi.org/10.32604/biocell.2023.026049
Received 12 August 2022; Accepted 06 October 2022; Issue published 03 January 2023
Abstract
Autophagy is an important metabolic process. It facilitates the recycling of intracellular substances by removing, degrading, and recycling damaged organelles, proteins, and lipids in lysosomal vacuoles and plays an important role in maintaining cellular homeostasis. Protein phosphatase 2A (PP2A) is a key serine/threonine phosphatase and one of the main cell cycle regulatory enzymes. As PP2A activity is essential for the cell, dysfunction or dysregulation of PP2A can affect various physiological processes, including autophagy. Here, we review the autophagy-related factors that target PP2A in different diseases, such as breast cancer, colorectal cancer, liver cancer, and Alzheimer’s disease, to maintain cell homeostasis by modulating the level of autophagy through mTORC1/ULK1 pathway, MAPK pathway, or AMPK pathway.Keywords
In eukaryotic cells, cellular growth processes, such as differentiation, proliferation, survival, and apoptosis, are controlled by the regulation of protein phosphorylation. According to the physiological state of the cell, proteins can interconvert between phosphorylated and dephosphorylated status, and the process is regulated by specific protein kinases and protein phosphatases (Mumby and Walter, 1993). Biological systems have many protein kinases, mainly two families—protein tyrosine kinase and protein serine/threonine kinase (Johnson and Hunter, 2005). Phosphatases can be categorized into three superfamilies: serine/threonine phosphatase (PSP), tyrosine phosphatase (PTP), and bispecific phosphatase (DSP) (Hunter, 1995; Shi, 2009; Virshup and Shenolikar, 2009). PP2A is a member of the PSP family. As a current research hotspot, many studies have proved that PP2A has an important regulatory effect on the cell cycle, apoptosis, and autophagy through the dephosphorylation of substrates. Here we comprehensively review the participation of PP2A in the autophagy process in recent years to guide the further role exploring of PP2A in cellular autophagy.
The biological role of protein phosphatase 2A in cells
PP2A is an important serine/threonine phosphatase that plays a multifaceted key role in cell cycle regulation. PP2A regulates cell cycle initiation pathways and cell cycle checkpoints and dephosphorylates more than 300 substrates involved in the cell cycle (Wlodarchak and Xing, 2016). PP2A is complex in structure and usually exists in two different forms: dimer or trimer (Mayer-Jaekel and Hemmings, 1994; Cohen, 1997). Each PP2A holoenzyme consists of a catalytic subunit C, a regulatory subunit B, and a scaffold subunit A, whose assembly, intracellular localization, enzyme activity, and substrate specificity are dynamically regulated (Xu et al., 2006). PP2A catalytic subunit (PP2Ac) has a spherical structure encoded by two different genes, α and β, which have 97% sequence similarity. PP2Ac is commonly expressed in almost all tissues, with the greatest content in the heart and brain. The level of PP2Ac protein expression in cells is translationally regulated to maintain constant levels (Baharians and Schönthal, 1998). Similar to PP2Ac, the PP2A scaffold subunit can also generate two isoforms, Aα and Aβ encoded by PPP2R1A and PPP2R1B, which have 86% sequence homology (Hemmings et al., 1990). The PP2A regulatory subunits encoded by 15 different genes in the human genome are structurally diverse, with at least 26 different transcripts and splice variants. It is considered the primary regulator of the PP2A whole enzyme and may act as a targeted regulator to provide temporal and spatial specificity (Zolnierowicz et al., 1994). PP2A participates in cellular processes by forming structurally distinct whole enzyme families that are spatially and temporally regulated by specific regulators. Activated PP2A can regulate all cellular signaling pathways, including the mammalian target of rapamycin/Unc51-like kinase (mTORC1/ULK1) pathway and mitogen-activated protein kinase (MAPK) pathway (Wlodarchak and Xing, 2016).
The biological function of autophagy in cells
Autophagy is a process in which components within eukaryotic cells self-degrade; in this process, the substance to be degraded is transported to the lysosome or vacuole to be degraded by hydrolases. Autophagy is a relatively conserved process in biological evolution; it is an important way to maintain homeostasis within cells and plays an integral role in cell proliferation, differentiation, and aging (Kroemer, 2015). The three main subtypes of autophagy are microautophagy (obtaining intracytoplasmic matter directly through the inversion of the endosomal chamber restriction membrane), chaperone-mediated autophagy (chaperone-mediated recruitment of unfolded proteins to lysosomes through KFERQ-like polypeptide sequences), and macroautophagy (characterized by autophagosomes of bimeal vesicles that completely engulf specific substances before fusing with lysosomes to enter the lysis chamber). Macroautophagy is the best-characterized form of mammalian autophagy and is often referred to as autophagy (Kocak et al., 2022). Autophagy includes the initial formation of a bi-membrane structure called a pre-autophagosomal structure, engulfing the autophagic target and continuing to form closed autophagosomes. More than 30 autophagy-related gene products control the formation of autophagosomes, which are the core of autophagy (Dikic and Elazar, 2018). Autophagosomes are double-membrane vesicle structures that transport damaged organelles, long-lived proteins, and even invading pathogens to lysosomes through the fusion of the outer membrane with the lysosomal membrane (He and Klionsky, 2009). After lysosomal fusion, the contents of the lysosome are degraded, and nutrients are recovered by cells (Mizushima, 2018). The main function of autophagy is to recover and re-uptake nutrients from the cytoplasm and degrade specific components to prevent cell damage under metabolic stress conditions, promote cell survival against a state of energy and nutrient deficiency, and respond to various cytotoxic injuries. For example, damaged mitochondria are treated by mitophagy (Pickles et al., 2018)or xenophagy (Sharma et al., 2018). Autophagy was initially thought to involve non-selective chelation and degradation of cytoplasmic contents. Of late, many types of selective autophagy have been found in various physiological processes, such as mitochondrial autophagy, reticular phagocytosis (Reggiori and Molinari, 2022), and sugar autophagy (Zhao et al., 2018).
Participations of protein phosphatase 2A in cellular autophagy
Protein phosphatase 2A changes autophagy levels through the mammalian target of the rapamycin signaling pathway
The mTOR is the target of rapamycin, or sirolimus, produced by the bacterium Streptomyces hygroscopius. It belongs to the phosphoinositide 3-kinase (PI3K)-related kinase family and is an atypical serine/threonine protein kinase, which can form mTOR complexes 1 (mTORC1) and 2 (mTORC2) with several proteins (Laplante and Sabatini, 2012). Many different cellular processes are associated with the mTOR pathway. The regulation of this pathway is also extremely complex, especially in relation to PP2A. Here we simply explain how PP2A regulates autophagy levels through a pathway involving mTOR.
Oncoprotein phosphatase 2A inhibitor (CIP2A), an endogenous PP2A inhibitor, has been identified as an oncoprotein that promotes the initiation and progression of various cancers. Many studies have pointed out that CIP2A is involved in the regulation of mTORC1 and autophagy (Puustinen and Jäättelä, 2014; Puustinen et al., 2014; Liu et al., 2017). While CIP2A has been shown to inhibit autophagy, its degradation can be enhanced by autophagy activation (Chen et al., 2010; Liu et al., 2017). By studying the role of CIP2A in doxorubicin-resistant breast cancer, (Zhu and Wei, 2021) found that CIP2A knockdown led to an increase in the activity of PP2A, and the expression of autophagy markers MAP1LC3B (LC3B) and Beclin1 was up-regulated (Zhu and Wei, 2021). It suggests that PP2A has a positive regulatory effect on autophagy, and the specific mechanism remains to be explored.
Hypoxia-inducible factors 1 (HIF1) and HIF2, negatively regulated by HIF prolyl hydroxylase (PHD) family members PHD1, PHD2, and PHD3, are major executors of cellular responses to hypoxia. The study by di Conza et al. (2017) shows that the mTOR downstream kinase P70S6K phosphorylates PHD2 at serine 125 (S125), resulting in enhanced HIF1α degradation. PP2A directly dephosphorylates PHD2 at S125 through its regulatory subunit B55α, leading to a further decrease in PHD2 activity and, ultimately, increased HIF1α accumulation (di Conza et al., 2017). Under hypoxic conditions, HIF1α stabilizes to promote cancer cell survival by initiating autophagy, which requires transcriptional induction of BNIP3 and BNIP3L (Mazure and Pouysségur, 2010). Silencing B55α prevents the upregulation of hypoxia-induced autophagy markers BNIP3 and BNIP3L and inhibits the degradation and induction of autophagy substrates p62 and LC3B (di Conza et al., 2017). These results suggest that the stabilization of HIF1α by PP2A/B55α promotes colorectal cancer cell survival through autophagy in a PHD2-dependent manner.
The cluster of differentiation (CD)24 is a glycoprotein expressed on the surface of most B lymphocytes and in some tumors. Recent studies have shown that CD24 expression is associated with the occurrence and development of many tumors, including prostate cancer (Zhang et al., 2016), cervical cancer (Sung et al., 2010), non-small cell lung cancer (Majores et al., 2015), gastric cancer (Sastry et al., 2014), and breast cancer (Suyama et al., 2016). However, in different tumors or tumor environments, CD24 has different roles and different ways of activating its downstream signaling (Baumann et al., 2005; Tang et al., 2014). When studying CD24 in hepatocellular carcinoma (HCC) with sorafenib resistance, Lu et al. (2018) found that CD24 overexpression induces the mTOR/protein kinase B (Akt) pathway inactivation, increases PP2A protein production, and increases autophagy levels. Sorafenib treatment in sorafenib-resistant cells induces morphological and biochemical features of autophagy. Compared to CD24-knockdown sorafenib-resistant cells, non-knockdown cells show abundant bimodal vacuolar structures, which are characteristic morphology of autophagosome, with increased LC3-II protein expression and decreased p62 (Lu et al., 2018). These phenomena suggest that CD24 regulates sorafenib resistance by activating autophagy in HCC.
Manganese (Mn) exposure leads to autophagy disruption and contributes to neurodegenerative diseases like Parkinson’s syndrome (PD) and Alzheimer’s disease, but the specific toxicity mechanism is unclear. One study revealed that PP2Ac methylation is involved in autophagy modulation by Mn. Through the activation of the mTORC1 signaling pathway, the down-regulation of PP2Ac methylation can ameliorate the abnormal autophagy induced by Mn in mouse neuroblastoma cells (N2a). In this way, the cytotoxicity and oxidative stress caused by Mn exposure can be effectively alleviated, suggesting that autophagy regulation plays a protective role in Mn-induced neurotoxicity (Xu et al., 2021b).
PD is a neurodegenerative disease, more common in the elderly. Autophagy is considered a promising therapeutic approach due to its important regulatory role in PD. Piperine (PIP) is a kind of traditional Chinese medicine containing amide alkaloids, which has pharmacological effects such as protecting the cardiovascular system, anti-tumor, and anti-inflammatory. Liu et al. (2016) reported that PIP inhibits mTORC1 by activating PP2A, thereby inducing autophagy. However, these protective effects were attenuated after the inhibition of PP2A activity using okadaic acid, suggesting that PP2A is a target of PIP (Liu et al., 2016). These findings indicate that PIP exerts neuroprotective effects by inducing autophagy in PD models and may become a drug with therapeutic effects on PD.
The autophagy pathway for glycogen degradation is known as glycogen autophagy, and it includes the sequestration and degradation of glycogen within autophagy vesicles with the release of free glucose (Kalamidas and Kondomerkos, 2010). Activation of mTOR is found to reduce glycogen autophagy by inhibiting PP2A, which acts as a common target of glucagon, insulin, and cAMP downstream of mTOR, and promotes α-1,4-glucosidase synthesis when active (Kalamidas et al., 2004). A study of cardiotoxin (CTX) by Chiou et al. (2019) showed that the addition of CTX causes calcium-dependent degradation of PP2Ac and phosphorylation of AMP-activated protein kinase subunit α (AMPKα). In CTX3-treated cells, the phosphorylation of AMPKα increased, and the expression of autophagy-related protein LC3 increased, while the expression of p62 decreased. Overexpression of PP2Ac attenuates CTX-induced AMPKα phosphorylation. CTX-induced autophagy is achieved through AMPK-mediated inhibition of the Akt/mTOR pathway. CTX is suggested to cause autophagy and apoptosis through the Ca2+/PP2A/AMPK axis (Chiou et al., 2019).
Protein phosphatase 2A changes autophagy levels through the mitogen-activated protein kinase (MAPK) pathway
MAPKs are serine/threonine protein kinases activated by substances such as cytokines, growth factors, hormones, and neurotransmitters (Widmann et al., 1999). MAPK signaling pathway plays an important role in regulating many physiological processes in eukaryotic cells, such as gene expression, metabolism, apoptosis, and survival (Cargnello and Roux, 2011). All MAPK signaling cascades consist of a three-layered module of protein kinases, with the MAPK kinase at the top (also known as MKKK or MAP3K), the MAPK kinase in the middle (also known as MKK, MEK, or MAP2K), and the bottom MAPK (Martínez-Limón et al., 2020).
A morphological feature of autophagy is the formation of acidic vesicular organelles (AVOs) (Puglisi et al., 2019). Wu et al. (2019) showed that apoptosis induced by the drug penfluidol could be inhibited by activating PP2A, which can inhibit Akt and MAPK activities. Furthermore, penfluidol-treated cells induced ROS-mediated autophagy by triggering LC3 turnover (LC3B-I to LC3B-II transition), p62 degradation, and formation of AVOs. Inhibition of ROS-mediated autophagy significantly enhances penfluidol-induced apoptosis. At the same time, patients with acute myeloid leukemia (AML) with high expression of PP2A have also been observed clinically to have a good prognosis (Wu et al., 2019). Taken together, these findings suggest that penfluidol-mediated autophagy is a pro-survival mechanism, and inhibiting its protective effect may improve the efficacy of AML treatment.
Protein phosphatase 2A changes autophagy levels through other pathways
Microcystin-leucine-arginine (MC-LR) has been identified as a harmful substance that causes liver toxicity. A study on a mice model with MC-LR-induced breakdown of apical ectoplasmic specialization (ES) revealed that down-regulation of the actin cross-linking protein palladin might be associated with the apical ES disassembly in mouse testis after MC-LR exposure (Xu et al., 2021a). MC-LR interferes with the interconnection between palladin and other actin-related proteins, thereby hindering the organization of F-actin. After exposure to MC-LR, AMPK could be activated by reduced PP2A activity, to then up-regulate the expression of LKB1 and CAMKK2, increase LC3B-II expression, down-regulate the autophagy substrate SQSTM1/p62 expression, and increase the level of autophagy. MC-LR induces degradation of palladin through AMPK/ULK1-mediated autophagy, which may lead to apical ES disturbance and shedding of spermatocytes from the seminiferous epithelium. These experimental phenomena may provide new perspectives for understanding MC-LR-induced male infertility.
The effect of PP2A on substrate phosphorylation determines its important role in the regulation of the cell cycle. Zhong et al. (2020) found that fingolimod (FTY720), a novel immunosuppressant, could dephosphorylate AMPKα at Thr172 by activating PP2A to activate the PP2A/AMPKα pathway. Induction of autophagy resulted in increased expression of LC3B, decreased expression of p62, and decreased expression of phosphorylated eukaryotic elongation factor 2 (eEF2), ultimately leading to myeloma cell death (Zhong et al., 2020).
Renal tubular epithelial cells require a large amount of fatty acid oxidation for energy, and energy production requires the regulation of AMPK. The function of AMPK in acute kidney disease and tubular epithelial cells has not been elucidated. Ma et al. (2022) found that AMPKα prevents tubular epithelial cell damage in ischemia/reperfusion-induced acute kidney injury. Ischemia/reperfusion activates PP2A and dephosphorylates AMPKα at the Thr172 site. Decreased AMPK activity suppresses autophagy levels and reduces the ability of cells to clear dysfunctional mitochondria. The allosteric AMPK activator C24 restores fatty acid oxidation and reduces tubular apoptosis in renal tubules after ischemia/reperfusion-induced cell injury. It works by targeting the PP2A-AMPK axis to antagonize the dephosphorylation process of PP2A, significantly increase autolysosomes in renal tubules, and promote mitophagy (Ma et al., 2022).
Diabetes mellitus-related cardiomyopathy (DMCMP) is one of the important cardiovascular complications of diabetes mellitus. It is a type of primary and specific cardiomyopathy with changes in myocardial structure and function caused by hyperglycemia and insulin resistance. Researchers studied the role and mechanism of PP2A in DMCMP in vivo and in vitro and found that the enhanced activity or increased expression of PP2A in DMCMP can up-regulate the expression of nuclear factor NF-E2-related factor 2 (Nrf2) by increasing the LC3B2/LC3B1 ratio and decreasing P62 protein expression, to increase the level of autophagy, thereby initiating protective autophagy in cardiomyocytes (Guan et al., 2019). In the study of basal muscle-invasive bladder cancer, Xu et al. (2020) found that long non-coding RNA-small nuclear RNA host gene 1 (SNHG1) can competitively bind with PP2Ac to inhibit its interaction with c-Jun, thereby promoting the phosphorylation of c-Jun, and mediate the transcription of MMP2. SNHG1 is also found to significantly induce autophagy in cells by inducing an increase in the abundance of autophagy-related proteins. Overexpression of SNHG1 not only induces the formation of autophagosomes but also converts LC3-I into LC3-II; consequently, the expression of ATG3 and ATG7 is up-regulated, and the binding rate of ATG5 and ATG12 is also high, which indirectly suggests the relationship between PP2A and autophagy (Xu et al., 2020). In a comprehensive phosphoproteomic analysis, the researchers found that ULK1, a protein required for autophagy vacuolation, is not only a target of PP2A, but also directly phosphorylates the PP2A subunit striatin, activates PP2A, and exerts positive feedback to promote autophagy-dependent protein turnover (Hu et al., 2021).
Clinically, multiple myeloma (MM) is incurable due to drug resistance. The results of some studies on the pathogenesis of MM suggest that growth factor-independent-1 (GFI-1) can increase the level of sphingosine 1-phosphate (S1P) by regulating sphingolipid metabolism, independent of the p53 state (Petrusca et al., 2022). GFI1 inhibits the expression of S1P phosphatase (SGPP1), thereby maintaining high intracellular S1P levels and keeping PP2A inactive, resulting in high c-Myc protein levels. Reduced intracellular S1P levels induce cell death via autophagy and conversion of LC3-I to LC3-II and reveal that GFI1-mediated protection of MM cell viability through S1P and p53WT is an independent pathway.
Metformin is a commonly used drug in the clinical treatment of diabetes. Studies have demonstrated that in addition to increasing insulin sensitivity, it can also induce mitophagy (Zhao and Sun, 2020). To initiate mitophagy, PINK1 is phosphorylated to activate parkin, which then builds ubiquitin chains with proteins on the mitochondrial outer membrane to recruit autophagy receptors (Lazarou et al., 2015). However, the molecular mechanism of metformin-induced mitophagy has not been elucidated. (Zhao and Sun, 2020) found that the use of metformin can reduce the apoptosis of high glucose-induced human renal epithelial cells. Mechanistically, metformin restores parkin protein expression and mitophagy by activating PP2A and inhibiting NF-κB. The relative mRNA and protein expressions of the mitophagy genes MFN2, PARKIN, PINK1, LC3-II, and LAMP2 are up-regulated in metformin-treated cells, indicating that metformin significantly affects the mitophagy process (Zhao and Sun, 2020). Deep brain stimulation (DBS) of the subthalamic nucleus (STN) is an effective treatment to alleviate disability in patients with moderate to severe PD. Preclinical studies have shown that stimulation of the rat STN can reduce the loss of substantia nigra dopaminergic neurons (Musacchio et al., 2017), but the specific mechanism is unclear. Du et al. (2018) found that in a rat model of PD, STN-DBS could induce autophagy through PP2A inactivation and dissociation of the Bcl-2/Beclin1 complex, thereby inhibit 6-OHDA-induced PD cell damage, and exert a neuroprotective effect. In short, STN-DBS inactivates PP2A and initiates autophagy to protect neurons, thereby providing the molecular basis for the neuroprotective effect of STN-DBS on PD (Du et al., 2018).
As shown in Fig. 1 and Table 1, different molecules can target PP2A through the mTORC1/ULK1 pathway and MAPK pathway or directly target the PP2A-AMPK axis, to increase or decrease the level of autophagy, improve physiological conditions, and maintain cell homeostasis. In short, a complex regulatory network between PP2A and autophagy, and the two are interconnected and inseparable, but the specific molecular mechanism for the interconnection between the two is still unclear. This study briefly explains the regulatory relationship between PP2A and autophagy. Only by continuing to study the relationship between the two can we provide new clues for the further study of PP2A and the application of clinical PP2A inhibitors.
Figure 1: PP2A alters autophagy levels through different pathways. Note: PP2A, protein phosphatase 2A; mTOR, mammalian target of rapamycin; CTX, cardiotoxin; Mn, manganese; PIP, piperine; CIP2A, oncoprotein phosphatase 2A inhibitor; MC-LR, microcystin-leucine-arginine; FTY720, fingolimod; GFI1, growth factor independence-1. The black arrow indicates “promoting effect,” the red line indicates “inhibiting effect,” and P indicates “dephosphorylation.”
Author Contribution: The authors confirm their contribution to the paper as follows: study conception and design: HMW, DL, XNZ; data collection: DL, RYL; draft manuscript preparation: HMW, XNZ. All authors compared different studies and approved the final version of the manuscript.
Ethics Approval: Not applicable.
Funding Statement: This work was supported by the National Natural Science Foundation of China (82060607, 81860586) and the Natural Science Foundation of Guangxi Province (2020GXNSFDA297010).
Conflicts of Interest: The authors declare that they have no conflicts of interest to report regarding the present study.
References
Baharians Z, Schönthal AH (1998). Autoregulation of protein phosphatase type 2A expression. The Journal of Biological Chemistry 273: 19019–19024. DOI 10.1074/jbc.273.30.19019. [Google Scholar] [CrossRef]
Baumann P, Cremers N, Kroese F, Orend G, Chiquet-Ehrismann R, Uede T, Yagita H, Sleeman JP (2005). CD24 expression causes the acquisition of multiple cellular properties associated with tumor growth and metastasis. Cancer Research 65: 10783–10793. DOI 10.1158/0008-5472.CAN-05-0619. [Google Scholar] [CrossRef]
Cargnello M, Roux PP (2011). Activation and function of the MAPKs and their substrates, the MAPK-activated protein kinases. Microbiology and Molecular Biology Reviews 75: 50–83. DOI 10.1128/MMBR.00031-10. [Google Scholar] [CrossRef]
Chen KF, Liu CY, Lin YC, Yu HC, Liu TH, Hou DR, Chen PJ, Cheng AL (2010). CIP2A mediates effects of bortezomib on phospho-Akt and apoptosis in hepatocellular carcinoma cells. Oncogene 29: 6257–6266. DOI 10.1038/onc.2010.357. [Google Scholar] [CrossRef]
Chiou JT, Shi YJ, Wang LJ, Huang CH, Lee YC, Chang LS (2019). Naja atra cardiotoxin 3 elicits autophagy and apoptosis in U937 human leukemia cells through the Ca2+/PP2A/AMPK axis. Toxins 11: 527. DOI 10.3390/toxins11090527. [Google Scholar] [CrossRef]
Cohen PT (1997). Novel protein serine/threonine phosphatases: Variety is the spice of life. Trends in Biochemical Sciences 22: 245–251. DOI 10.1016/S0968-0004(97)01060-8. [Google Scholar] [CrossRef]
di Conza G, Trusso Cafarello S, Loroch S, Mennerich D, Deschoemaeker S et al. (2017). The mTOR and PP2A pathways regulate PHD2 phosphorylation to fine-tune HIF1α levels and colorectal cancer cell survival under hypoxia. Cell Reports 18: 1699–1712. DOI 10.1016/j.celrep.2017.01.051. [Google Scholar] [CrossRef]
Dikic I, Elazar Z (2018). Mechanism and medical implications of mammalian autophagy. Nature Reviews Molecular Cell Biology 19: 349–364. DOI 10.1038/s41580-018-0003-4. [Google Scholar] [CrossRef]
Du TT, Chen YC, Lu YQ, Meng FG, Yang H, Zhang JG (2018). Subthalamic nucleus deep brain stimulation protects neurons by activating autophagy via PP2A inactivation in a rat model of Parkinson’s disease. Experimental Neurology 306: 232–242. DOI 10.1016/j.expneurol.2018.05.017. [Google Scholar] [CrossRef]
Guan Y, Zhou L, Zhang Y, Tian H, Li A, Han X (2019). Effects of PP2A/Nrf2 on experimental diabetes mellitus-related cardiomyopathy by regulation of autophagy and apoptosis through ROS dependent pathway. Cellular Signalling 62: 109339. DOI 10.1016/j.cellsig.2019.06.004. [Google Scholar] [CrossRef]
He C, Klionsky DJ (2009). Regulation mechanisms and signaling pathways of autophagy. Annual Review of Genetics 43: 67–93. DOI 10.1146/annurev-genet-102808-114910. [Google Scholar] [CrossRef]
Hemmings BA, Adams-Pearson C, Maurer F, Müller P, Goris J, Merlevede W, Hofsteenge J, Stone SR (1990). α- and β-forms of the 65-kDa subunit of protein phosphatase 2A have a similar 39 amino acid repeating structure. Biochemistry 29: 3166–3173. DOI 10.1021/bi00465a002. [Google Scholar] [CrossRef]
Hu Z, Sankar DS, Vu B, Leytens A, Vionnet C, Wu W, Stumpe M, Martínez-Martínez E, Stork B, Dengjel J (2021). ULK1 phosphorylation of striatin activates protein phosphatase 2A and autophagy. Cell Reports 36: 109762. DOI 10.1016/j.celrep.2021.109762. [Google Scholar] [CrossRef]
Hunter T (1995). Protein kinases and phosphatases: The yin and yang of protein phosphorylation and signaling. Cell 80: 225–236. DOI 10.1016/0092-8674(95)90405-0. [Google Scholar] [CrossRef]
Johnson SA, Hunter T (2005). Kinomics: Methods for deciphering the kinome. Nature Methods 2: 17–25. DOI 10.1038/nmeth731. [Google Scholar] [CrossRef]
Kalamidas SA, Kondomerkos DJ (2010). Autophagosomal glycogen-degrading activity and its relationship to the general autophagic activity in newborn rat hepatocytes: The effects of parenteral glucose administration. Microscopy Research and Technique 73: 495–502. DOI 10.1002/jemt.20788. [Google Scholar] [CrossRef]
Kalamidas SA, Kondomerkos DJ, Kotoulas OB, Hann AC (2004). Electron microscopic and biochemical study of the effects of rapamycin on glycogen autophagy in the newborn rat liver. Microscopy Research and Technique 63: 215–219. DOI 10.1002/jemt.20032. [Google Scholar] [CrossRef]
Kocak M, Ezazi Erdi S, Jorba G, Maestro I, Farrés J, Kirkin V, Martinez A, Pless O (2022). Targeting autophagy in disease: Established and new strategies. Autophagy 18: 473–495. DOI 10.1080/15548627.2021.1936359. [Google Scholar] [CrossRef]
Kroemer G (2015). Autophagy: A druggable process that is deregulated in aging and human disease. The Journal of Clinical Investigation 125: 1–4. DOI 10.1172/JCI78652. [Google Scholar] [CrossRef]
Laplante M, Sabatini DM (2012). mTOR signaling in growth control and disease. Cell 149: 274–293. DOI 10.1016/j.cell.2012.03.017. [Google Scholar] [CrossRef]
Lazarou M, Sliter DA, Kane LA, Sarraf SA, Wang C, Burman JL, Sideris DP, Fogel AI, Youle RJ (2015). The ubiquitin kinase PINK1 recruits autophagy receptors to induce mitophagy. Nature 524: 309–314. DOI 10.1038/nature14893. [Google Scholar] [CrossRef]
Liu J, Chen M, Wang X, Wang Y, Duan C, Gao G, Lu L, Wu X, Wang X, Yang H (2016). Piperine induces autophagy by enhancing protein phosphotase 2A activity in a rotenone-induced Parkinson’s disease model. Oncotarget 7: 60823–60843. DOI 10.18632/oncotarget.11661. [Google Scholar] [CrossRef]
Liu X, Cao W, Qin S, Zhang T, Zheng J et al. (2017). Overexpression of CIP2A is associated with poor prognosis in multiple myeloma. Signal Transduction and Targeted Therapy 2: 17013. DOI 10.1038/sigtrans.2017.13. [Google Scholar] [CrossRef]
Lu S, Yao Y, Xu G, Zhou C, Zhang Y, Sun J, Jiang R, Shao Q, Chen Y (2018). CD24 regulates sorafenib resistance via activating autophagy in hepatocellular carcinoma. Cell Death & Disease 9: 646. DOI 10.1038/s41419-018-0681-z. [Google Scholar] [CrossRef]
Ma H, Guo X, Cui S, Wu Y, Zhang Y, Shen X, Xie C, Li J (2022). Dephosphorylation of AMP-activated protein kinase exacerbates ischemia/reperfusion-induced acute kidney injury via mitochondrial dysfunction. Kidney International 101: 315–330. DOI 10.1016/j.kint.2021.10.028. [Google Scholar] [CrossRef]
Majores M, Schindler A, Fuchs A, Stein J, Heukamp L, Altevogt P, Kristiansen G (2015). Membranous CD24 expression as detected by the monoclonal antibody SWA11 is a prognostic marker in non-small cell lung cancer patients. BMC Clinical Pathology 15: 19. DOI 10.1186/s12907-015-0019-z. [Google Scholar] [CrossRef]
Martínez-Limón A, Joaquin M, Caballero M, Posas F, de Nadal E (2020). The p38 pathway: From biology to cancer therapy. International Journal of Molecular Sciences 21: 1913. DOI 10.3390/ijms21061913. [Google Scholar] [CrossRef]
Mayer-Jaekel RE, Hemmings BA (1994). Protein phosphatase 2A—A ‘ménage à trois’. Trends in Cell Biology 4: 287–291. DOI 10.1016/0962-8924(94)90219-4. [Google Scholar] [CrossRef]
Mazure NM, Pouysségur J (2010). Hypoxia-induced autophagy: Cell death or cell survival? Current Opinion Cell Biology 22: 177–180. DOI 10.1016/j.ceb.2009.11.015. [Google Scholar] [CrossRef]
Mizushima N (2018). A brief history of autophagy from cell biology to physiology and disease. Nature Cell Biology 20: 521–527. DOI 10.1038/s41556-018-0092-5. [Google Scholar] [CrossRef]
Mumby MC, Walter G (1993). Protein serine/threonine phosphatases: Structure, regulation, and functions in cell growth. Physiological Reviews 73: 673–699. DOI 10.1152/physrev.1993.73.4.673. [Google Scholar] [CrossRef]
Musacchio T, Rebenstorff M, Fluri F, Brotchie JM, Volkmann J, Koprich JB, Ip CW (2017). Subthalamic nucleus deep brain stimulation is neuroprotective in the A53T α-synuclein Parkinson’s disease rat model. Annals of Neurology 81: 825–836. DOI 10.1002/ana.24947. [Google Scholar] [CrossRef]
Petrusca DN, Mulcrone PL, Macar DA, Bishop RT, Berdyshev E et al. (2022). GFI1-dependent repression of SGPP1 increases multiple myeloma cell survival. Cancers 14: 772. DOI 10.3390/cancers14030772. [Google Scholar] [CrossRef]
Pickles S, Vigié P, Youle RJ (2018). Mitophagy and quality control mechanisms in mitochondrial maintenance. Current Biology 28: R170–R185. DOI 10.1016/j.cub.2018.01.004. [Google Scholar] [CrossRef]
Puglisi F, Parrinello NL, Giallongo C, Cambria D, Camiolo G, (2019). Plasticity of high-density neutrophils in multiple myeloma is associated with increased autophagy via STAT3. International Journal of Molecular Sciences 20: 3548. DOI 10.3390/ijms20143548. [Google Scholar] [CrossRef]
Puustinen P, Jäättelä M (2014). KIAA1524/CIP2A promotes cancer growth by coordinating the activities of MTORC1 and MYC. Autophagy 10: 1352–1354. DOI 10.4161/auto.29076. [Google Scholar] [CrossRef]
Puustinen P, Rytter A, Mortensen M, Kohonen P, Moreira JM, Jäättelä M (2014). CIP2A oncoprotein controls cell growth and autophagy through mTORC1 activation. The Journal of Cell Biology 204: 713–727. DOI 10.1083/jcb.201304012. [Google Scholar] [CrossRef]
Reggiori F, Molinari M (2022). ER-phagy: Mechanisms, regulation, and diseases connected to the lysosomal clearance of the endoplasmic reticulum. Physiological Reviews 102: 1393–1448. DOI 10.1152/physrev.00038.2021. [Google Scholar] [CrossRef]
Sastry KS, Al-Muftah MA, Li P, Al-Kowari MK, Wang E et al. (2014). Targeting proapoptotic protein BAD inhibits survival and self-renewal of cancer stem cells. Cell Death and Differentiation 21: 1936–1949. DOI 10.1038/cdd.2014.140. [Google Scholar] [CrossRef]
Sharma V, Verma S, Seranova E, Sarkar S, Kumar D (2018). Selective autophagy and xenophagy in infection and disease. Frontiers in Cell and Developmental Biology 6: 147. DOI 10.3389/fcell.2018.00147. [Google Scholar] [CrossRef]
Shi Y (2009). Serine/threonine phosphatases: Mechanism through structure. Cell 139: 468–484. DOI 10.1016/j.cell.2009.10.006. [Google Scholar] [CrossRef]
Sung CO, Park W, Choi YL, Ahn G, Song SY, Huh SJ, Bae DS, Kim BG, Lee JH (2010). Prognostic significance of CD24 protein expression in patients treated with adjuvant radiotherapy after radical hysterectomy for cervical squamous cell carcinoma. Radiotherapy and Oncology 95: 359–364. DOI 10.1016/j.radonc.2010.01.007. [Google Scholar] [CrossRef]
Suyama K, Onishi H, Imaizumi A, Shinkai K, Umebayashi M et al. (2016). CD24 suppresses malignant phenotype by downregulation of SHH transcription through STAT1 inhibition in breast cancer cells. Cancer Letters 374: 44–53. DOI 10.1016/j.canlet.2015.12.013. [Google Scholar] [CrossRef]
Tang MR, Wang YX, Guo S, Han SY, Li HH, Jin SF (2014). CD24 expression predicts poor prognosis for patients with cutaneous malignant melanoma. International Journal of Clinical and Experimental Medicine 7: 4337–4341. [Google Scholar]
Virshup DM, Shenolikar S (2009). From promiscuity to precision: Protein phosphatases get a makeover. Molecular Cell 33: 537–545. DOI 10.1016/j.molcel.2009.02.015. [Google Scholar] [CrossRef]
Widmann C, Gibson S, Jarpe MB, Johnson GL (1999). Mitogen-activated protein kinase: Conservation of a three-kinase module from yeast to human. Physiological Reviews 79: 143–180. DOI 10.1152/physrev.1999.79.1.143. [Google Scholar] [CrossRef]
Wlodarchak N, Xing Y (2016). PP2A as a master regulator of the cell cycle. Critical Reviews in Biochemistry and Molecular Biology 51: 162–184. DOI 10.3109/10409238.2016.1143913. [Google Scholar] [CrossRef]
Wu SY, Wen YC, Ku CC, Yang YC, Chow JM, Yang SF, Lee WJ, Chien MH (2019). Penfluridol triggers cytoprotective autophagy and cellular apoptosis through ROS induction and activation of the PP2A-modulated MAPK pathway in acute myeloid leukemia with different FLT3 statuses. Journal of Biomedical Science 26: 63. DOI 10.1186/s12929-019-0557-2. [Google Scholar] [CrossRef]
Xu D, Wang J, Ma Y, Ding J, Han X, Chen Y (2021a). Microcystin-leucine-arginine induces apical ectoplasmic specialization disassembly. Chemosphere 264: 128440. DOI 10.1016/j.chemosphere.2020.128440. [Google Scholar] [CrossRef]
Xu J, Yang R, Hua X, Huang M, Tian Z, Li J, Lam HY, Jiang G, Cohen M, Huang C (2020). lncRNA SNHG1 promotes basal bladder cancer invasion via interaction with PP2A catalytic subunit and induction of autophagy. Molecular Therapy Nucleic Acids 21: 354–366. DOI 10.1016/j.omtn.2020.06.010. [Google Scholar] [CrossRef]
Xu Y, Wei L, Tang S, Shi Q, Wu B et al. (2021b). Regulation PP2Ac methylation ameliorating autophagy dysfunction caused by Mn is associated with mTORC1/ULK1 pathway. Food and Chemical Toxicology 156: 112441. DOI 10.1016/j.fct.2021.112441. [Google Scholar] [CrossRef]
Xu Y, Xing Y, Chen Y, Chao Y, Lin Z, Fan E, Yu HW, Strack S, Jeffrey PD, Shi Y (2006). Structure of the protein phosphatase 2A holoenzyme. Cell 127: 1239–1251. DOI 10.1016/j.cell.2006.11.033. [Google Scholar] [CrossRef]
Zhang W, Yi B, Wang C, Chen D, Bae S et al. (2016). Silencing of CD24 enhances the PRIMA-1-induced restoration of mutant p53 in prostate cancer cells. Clinical Cancer Research 22: 2545–2554. DOI 10.1158/1078-0432.CCR-15-1927. [Google Scholar] [CrossRef]
Zhao H, Tang M, Liu M, Chen L (2018). Glycophagy: An emerging target in pathology. Clinica Chimica Acta 484: 298–303. DOI 10.1016/j.cca.2018.06.014. [Google Scholar] [CrossRef]
Zhao Y, Sun M (2020). Metformin rescues Parkin protein expression and mitophagy in high glucose-challenged human renal epithelial cells by inhibiting NF-κB via PP2A activation. Life Sciences 246: 117382. DOI 10.1016/j.lfs.2020.117382. [Google Scholar] [CrossRef]
Zhong Y, Tian F, Ma H, Wang H, Yang W, Liu Z, Liao A (2020). FTY720 induces ferroptosis and autophagy via PP2A/AMPK pathway in multiple myeloma cells. Life Sciences 260: 118077. DOI 10.1016/j.lfs.2020.118077. [Google Scholar] [CrossRef]
Zhu Z, Wei Z (2021). CIP2A silencing alleviates doxorubicin resistance in MCF7/ADR cells through activating PP2A and autophagy. Clinical &Translational Oncology 23: 1542–1548. DOI 10.1007/s12094-021-02616-7. [Google Scholar] [CrossRef]
Zolnierowicz S, Csortos C, Bondor J, Verin A, Mumby MC, DePaoli-Roach AA (1994). Diversity in the regulatory B-subunits of protein phosphatase 2A: Identification of a novel isoform highly expressed in brain. Biochemistry 33: 11858–11867. DOI 10.1021/bi00205a023. [Google Scholar] [CrossRef]
Cite This Article
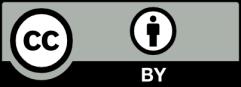