Open Access
ARTICLE
Role of foliar spray of plant growth regulators in improving photosynthetic pigments and metabolites in Plantago ovata (Psyllium) under salt stress–A field appraisal
1 Department of Botany, University of Balochistan, Quetta, 08770, Pakistan
2 Department of Bioinformatics and Biotechnology, Government College University, Faisalabad, 38000, Pakistan
3 Department of Botany, University of Agriculture, Faisalabad, 38040, Pakistan
4 Department of Food Science and Technology, Muhammad Nawaz Shareef University of Agriculture, Multan, 66000, Pakistan
5 Department of Agronomy, Muhammad Nawaz Shareef, University of Agriculture, Multan, 66000, Pakistan
6 College of Agriculture, Fujian Agriculture and Forestry University, Fuzhou, 350002, China
7 Department of Genetics and Plant Breeding, Faculty of Agriculture, University of Belgrade, Belgrade, 11000, Serbia
8 Institute of Field and Vegetable Crops, National Institute of the Republic of Serbia, Maxim Gorki 30, Novi Sad, 21000, Serbia
* Corresponding Author: KANVAL SHAUKAT. Email:
(This article belongs to the Special Issue: Physiology and Molecular Biology of Plant Stress Tolerance)
BIOCELL 2023, 47(3), 523-532. https://doi.org/10.32604/biocell.2023.023704
Received 10 May 2022; Accepted 13 September 2022; Issue published 03 January 2023
Abstract
Salinity is one of the major abiotic factors that limit the growth and productivity of plants. Foliar application of plant growth regulators (PGRs) may help plants ameliorate the negative impacts of salinity. Thus, a field experiment was conducted at the Botanical Garden University of Balochistan, Quetta, to explore the potential role of PGRs, i.e., moringa leaf extract (MLE; 10%), proline (PRO; 1 µM), salicylic acid (SA; 250 µM), and thiourea (TU; 10 mM) in ameliorating the impacts of salinity (120 mM) on Plantago ovata, an important medicinal plant. Salinity hampered plant photosynthetic pigments and metabolites but elevated oxidative parameters. However, foliar application of PGRs enhanced photosynthetic pigments, including Chl b (21.11%), carotenoids (57.87%) except Chl a, activated the defense mechanisms by restoring and enhancing the metabolites, i.e., soluble sugars (49.68%), soluble phenolics (33.34%), and proline (31.47%), significantly under salinity stress. Furthermore, foliar supplementation of PGRs under salt stress led to a decrease of about 43.02% and 43.27% in hydrogen peroxide and malondialdehyde content, respectively. Thus, PGRs can be recommended for improved photosynthetic efficiency and metabolite content that can help to get better yield under salt stress, with the best and most effective treatments being those of PRO and MLE to predominately ameliorate the harsh impacts of salinity.Keywords
Salinity is one of the major abiotic stresses affecting agriculture worldwide (Hafeez et al., 2021; Raza et al., 2022a). Over 45 million hectares of irrigated area, which accounts for 20% of the total area, have been vandalized. Each year, 1.5 million hectares of cultivable lose productivity due to saline conditions (Munns and Tester, 2008). Soil salinity adversely affects plant growth, development, and eventually successful crop stand establishment (Saddiq et al., 2019; Granaz et al., 2022). It affects various morpho-physiological attributes, such as the rate of photosynthesis, by affecting photosynthetic pigments, stomatal conductance, and chlorophyll (Chl) fluorescence (Saddiq et al., 2021a; Hafeez et al., 2021; Raza et al., 2022a). Photosynthesis is a key metabolic pathway; a higher rate of photosynthesis leads to normal plant growth under abiotic stress. The photosynthetic activity declines under salinity due to both stomatal and non-stomatal limitations (Zahra et al., 2022; Zahra et al., 2021a). The deficiency of nutrients under salinity is due to the Na and Cl ion that affects the uptake, transport, and distribution of nutrients within plants (Iqbal et al., 2019). The micronutrient availability in saline soil depends upon their solubility and soil pH. NaCl can also affect the micro-nutrient concentration depending on the crop species and salinity level (Rashad and Hussien, 2014; Shrivastava et al., 2019).
Plant growth regulators (PGRs) show diverse functions in plants under various stress conditions (Sabagh et al., 2021; Mir et al., 2022; Dai et al., 2022; Corpas et al., 2022). These are chemical messengers are produced in plants in small concentrations and control several morpho-physiological and biochemical attributes of plants (Ahmad et al., 2022; Mubarik et al., 2021; Sabagh et al., 2021; Mir et al., 2022; Raza et al., 2022b). The amino acid proline (PRO) is an osmolyte that is produced and accumulated under different environmental stresses, such as salinity stress (Mundada et al., 2021). The exogenous application of PRO to plants under salinity stress enhances their growth and physiological and biochemical attributes by alleviating and hampering the negative impacts of salinity stress (Tabssum et al., 2019). Thiourea (TU) is a synthetic compound containing sulfur and nitrogen and is increasingly being explored as a PGR under abiotic stresses (Patade et al., 2020). In pearl millet, TU treatment improved PRO content, relative water content, and total Chl and, consequently, improved grain yield while reducing lipid peroxidation under salt stress (Yadav et al., 2020). Salicylic acid (SA) is a common plant-produced phenolic compound that can function as a PGR, although there are various reports of positive physiological and biochemical functions of SA in plants under stress conditions (Sabagh et al., 2021; Shaukat et al., 2022). Exogenous application of SA increases the Chl contents and enhances the accumulation of glycine betaine, PRO, and total phenolic contents, which boosts the activities of antioxidant enzymes and the growth of Gladiolus grandiflorus (Ferdosi et al., 2021). Moringa leaf extract (MLE) is a vital PGR, acknowledged among the scientific community because it is a rich source of vitamins, growth hormones, mineral nutrients, and antioxidants (Aslam et al., 2020, Khan et al., 2021). A recent study showed that supplementation with MLE improved growth and yield attributes in milk thistle under saline conditions (Zahra et al., 2021b).
Plantago ovata Forssk. is a member of the family Plantaginaceae. It is an herb that is known by different names, such as psyllium, jiru, and aspaghol (Shah et al., 2020). It is grown in many regions due to its medicinal properties (Sabzehzari et al., 2019). The seed of plantago is used because it contains pharmacologically beneficial compounds (plantiose sugar, aucubin, and glycoside) and many secondary metabolites (Franco et al., 2020). Several studies have documented the medicinal potential of Plantago; however, there is no report on this medicinally important plant with respect to its salinity tolerance potential after exogenous supplementation of various PGRs and the impact on physiological and metabolic attributes of its growth in the environmental regimes of Quetta, Balochistan, Pakistan. Hypothesizing that “exogenous supplementation of PGRs may mitigate salinity stress by improving the physio-biochemical attributes of Plantago ovata”, an attempt was made to evaluate the comparative efficacy of PGRs and to delineate physiological mechanisms mediating salinity tolerance in P. ovata.
A field experiment was conducted at the Botanical Garden University of Balochistan Quetta (Pakistan). The design of the experiment was randomized complete block design (RCBD) under factorial. The plots were divided into two major plots, control and salinity. Furthermore, each plot has treatments of PGRs with three replications (3 rows). Seeds of P. ovata were sown at a one-inch depth, with the row-to-row and plant-to-plant spacing of 1 ft. After 10 days of germination, salt solution was applied to the field (120 mM of salt solution was prepared and applied after calculating the field capacity of the experimental field soil); to avoid leaching, a salt black sheet was placed at a depth of 5 ft (this black sheet was used to prevent leaching of salt to nearby field plants). After one week of salt stress, optimized levels (i.e., levels selected based on preliminary trials; data not shown) of TU (10 mM), MLE (10%), SA (250 µM), and PRO (1 µM) applied as foliar treatment in comparison to water spray (H2O Spray) and no foliar spray (NFS) to both fields, i.e., control (Con) and salt-stressed (SS). After a week of foliar treatment, plants were harvested, and data for various attributes were recorded.
Analysis of the photosynthetic pigments
Chlorophyll contents were determined by following the method of Arnon (1949). For extraction, 0.1 g of leaves were ground in 1-2 mL of 80% acetone, maintained the final volume to 10 mL using acetone 80%. The absorbance was measured at 663, 645, and 480 nm, keeping 80% acetone as blank.
Carotenoid content was estimated following the method of Kirk (1967).
The absorbance showed unit as Acar per gram fresh weight
V = volume of acetone extract
W = leaf weight (gram fresh weight).
The anthocyanin content of both the shoot and root was determined by using the method of (Stark and Wray, 1989). Briefly, 0.1 g of plant sample (both shoot and root) were used to extract 1 to 2 mL of acidified methanol. The sample was placed in a water bath for 1 h at 50°C. The absorbance was measured at 535 nm using a spectrophotometer using acidified methanol as blank.
Soluble sugars (in shoot and root) were determined by the method developed by Yoshida and Coronel (1976). The sample (0.1 g) was taken and boiled in 5 mL of distilled water in a water bath for 1 h; from this, 1 mL of extract was taken, and 9 mL of distilled water was added for dilution. From this extract, 0.5 mL of extract was taken, and 5 mL of anthrone reagent was added and placed in a water bath for 20 min at 90°C. Later, absorbance was read at 620 nm using a spectrophotometer. Distilled water was used as blank.
Soluble phenolics of both shoot and root were determined by using Julkunen-Tiitto (1985) method. In short, 0.1 g of the sample was ground in 1–2 mL of 80% acetone. In the test tube, we added 1 mL of distilled water and 100 µL of the sample extract, followed by the addition of 0.5 mL of Folin phenol reagent and 2.5 mL of the 20% sodium carbonate. The samples were vortexed, and absorbance was noted at 745 nm using an 80% acetone blank.
For the estimation of free PRO, the method of Bates et al. (1973) was used. Briefly, 0.1 g fresh sample (shoot and root) was ground in 1–2 mL of 3% sulfosalicylic acid, and then the extract was filtered. Then, 1 mL of extract, 1 mL each of ninhydrin, and glacial acetic acid were added in each test tube. Then test tubes were shifted to a water bath for 1 h at 100°C; after that, the samples were shifted to an ice bath, and 2 mL of toluene was added, vortexed, and the absorbance was recorded at 520 nm. As a blank, 3% sulfosalicylic acid was used.
Hydrogen peroxide (H2O2) contents
Hydrogen peroxide was determined by the method developed by Velikova et al. (2000). Briefly, 0.1 g fresh plant sample was ground in 2 mL of 0.1% (w/v) trichloroacetic acid (TCA) under pre-chilled conditions (placed on ice bath). The extract was centrifuged at 12,000 rpm for 15 min. The supernatant (0.5 mL) was mixed with potassium phosphate buffer (0.5 mL, pH 7.0) and 1 mL of potassium iodide solution, and then thoroughly mixed. Absorbance was measured at 390 nm, and distilled water was used as blank.
MDA was determined following the method developed by Heath and Packer (1968). Briefly, 0.1 g of fresh plant sample was ground in 1 mL of (1% w/v) TCA and centrifuged at 12,000 rpm for 15 min. Then, 1 mL of suspension was taken and mixed with 1 mL of 0.5% thiobarbituric acid in 20% TCA [0.5% in 20% (w/v) TCA] and kept in a water bath preheated at 90°C for 50 min. Extracted sample was cooled in an ice bath. The absorbance was measured at 532 and 600 nm. TCA (1%) was used as a blank.
The data was statistically analyzed using “STATISTICX 8.1” (Analytical Software, Tallahassee, Florida), and the mean, SD, and graphs were generated using MS EXCEL. Furthermore, a post hoc test, i.e., the least significant difference (LSD), was used, and the labels/alphabets were added to graph bars of statistically significant attributes (P < 0.05).
Data obtained for Chl a of plantago grown under salt stress (SS) showed statistically significant results (P < 0.05). Under control conditions, TU led to the maximum Chl a (3.89 mg/g FWT) while MLE treatment led to the minimum (1.27 mg/g FWT) content; however, under saline conditions, Chl a content was the highest and the lowest when SA was applied (Fig. 1A). TU and SA foliar supplementation predominately increased Chl a content by about 80.40% and 26.42%, respectively, under control conditions; however, Chl a content decreased several folds under salt stress. Thus, Chl a of P. ovata was affected under salinity stress (Fig. 1A).
Figure 1: Changes in photosynthetic pigment contents. A = chlorophyll a, B = chlorophyll b, C = carotenoid content of Plantago ovata as affected under salinity stress. Plants were foliar-treated with different PGRs.
Data obtained for Chl b showed statistically significant results (P < 0.05). TU (Con) had maximum Chl b content (3.53 mg/g FWT), while minimum content (0.62 mg/g FWT) was observed in NFS (Con) (Fig. 1B). Considering the overall improvements in plantago Chl b content, it was observed that salinity affected the Chl b content, while a foliar spray of PGRs, especially PRO, predominately alleviated the negative impact of salinity stress (Fig. 1B).
Data obtained for the carotenoids (Caro) showed no statistically significant differences (P > 0.05). TU had the maximum (0.92 mg/g FWT), while MLE had the least carotenoid content (0.49 mg/g FWT) under control conditions (Fig. 1C). Exogenous supplementation of PGRs increased carotenoid contents by 24.42%–77.33% and 11.15% to 57.87% under control and salinity, respectively. TU predominantly increased carotenoid content by 77.33% and 57.87% under control and salinity correspondingly, thus evidently confirming the potential role of TU to alleviate salinity stress (Fig. 1C).
*NFS = no foliar spray, H2O = water spray, SA = salicylic acid (Foliar Spray), TU = thiourea (foliar spray), PRO = proline (foliar spray), MLE = moringa leaf extract (foliar spray). Different alphabets/letters on bars represent statistically significant interactions (P < 0.05) according to the least significant difference test.
Bars lacking the labels show non-significant interactions at P < 0.05.
Determination of Metabolites and Osmoprotectant
Data obtained for the shoot anthocyanin content (SAC; Fig. 2A) showed statistically significant results (P < 0.05) under saline and control conditions. The data revealed that TU (SS) had a maximum (2.32) anthocyanin content and a minimum (0.14) H2O under normal conditions (Fig. 2A). Exogenous supplementation of PGRs increased shoot anthocyanin content by 126.03%–461.22% and 8.89%–130.13% under control and salinity stress, respectively. MLE is the most effective treatment under control and TU under salinity stress (Fig. 2A).
Figure 2: Changes in plant metabolites. A = shoot anthocyanin, B = root anthocyanin, C = shoot soluble sugar, D = root-soluble sugar, E = shoot soluble phenolics, F = root Soluble phenolics of Plantago ovata as affected under salinity stress; plants were foliar treated with different PGRs.
Furthermore, results obtained for root anthocyanin content (RAC; Fig. 2B) showed statistically significant results (P < 0.05) under both conditions. Data illustrated that anthocyanin contents were higher under TU (SS), and a minimum was observed in TU (Con) (Fig. 2B). TU foliar treatment increased root anthocyanin content by 101.63% under salinity stress; however, PRO treatment increased root anthocyanin by 177.78% and 111.87% under control and salinity stress, respectively (Fig. 2B).
Data obtained for shoot soluble sugars (SSS; Fig. 2C) content showed statistically significant results (P < 0.05). Plantago plants under H2O spray had maximum (9.77 µmol/g FWT) soluble sugar content and minimum observed (6.53 µmol/g FWT) in SA under control conditions. Thus, foliar application of MLE alleviated salinity stress predominately after water foliar spray. Exogenous supplementation of PGRs increased SSS content under salinity stress by 32.74%–45.74%, with SA being the most effective treatment (45.74%) (Fig. 2C).
Furthermore, results recorded for the root-soluble sugar (RSS; Fig. 2D) content showed statistically significant results (P < 0.05). TU had maximum soluble sugar content (10.45 µmol/g FWT) and minimum content (9.58 µmol/g FWT) observed in PRO treatment under control conditions (Fig. 2D). Results revealed that the root-soluble sugar content of P. ovata was affected under salinity stress. However, foliar application of SA ameliorated the negative impacts of SS and increased root-soluble sugar content by 41.94% (Fig. 2D).
Data obtained for the shoot soluble phenolics (SSP; Fig. 2E) of plantago grown under salt stress (SS) showed statistically non-significant results (P < 0.05). Data further suggested that PRO-treated plants treated had maximum SSP content (9.22 µmol/g FWT) and minimum observed (7.48 µmol/g FWT) in SA plants under control conditions (Fig. 2E). Foliar supplementation of PGRs successfully ameliorated the negative impacts of salinity by increasing SSP in plantago by about 7.01%–33.34%, with SA the most effective treatment increasing SSP by 33.34% under SS (Fig. 2E).
Considering data obtained for root soluble phenolics (RSP; Fig. 2F) showed significant results (P < 0.05). Data recorded reveal that H2O spray had maximum RSP content (34.53 µmol/g FWT) and least content (27.29 µmol/g FWT) observed in H2O spray plants under saline conditions (Fig. 2F).
An increase in both shoot and root soluble phenolics content under salinity stress validates that the concentration of secondary metabolites increases as a part of its defense mechanism; thus, exogenous supplementation of PGRs enhances the tolerance potential of plants to withstand stress effectively (Fig. 2F).
*NFS = no foliar spray, H2O = water spray, SA = salicylic acid (foliar spray), TU = thiourea (foliar spray), PRO = proline (foliar spray), MLE = moringa leaf extract (foliar spray).
Different alphabets/letters on bars represent statistically significant interactions (P < 0.05) according to the least significant difference test. Bars lacking the labels show non-significant interactions at P < 0.05.
Data obtained for shoot-free PRO (SFP) content showed statistically non-significant results (P > 0.05). Under saline conditions, SFP content was maximum when MLE (13.53 mg/g) was applied, and minimum content when H2O2 (9.17 mg/g) was applied while the maximum content was noted (13.23 mg/g) when MLE was applied, and the minimum content (8.25 mg/g) was observed in NFS under control conditions. MLE increased SFP content by 60.37% under control conditions, while the content increased by 31.47% under salinity stress (SS; Fig. 3A).
Figure 3: Changes in Osmoprotectant. A = shoot proline B = root proline content of Plantago ovata as affected under salinity stress. Plants were foliar treated with different PGRs.
Data recorded for root-free PRO (RFP) showed statistically significant results (P < 0.05); maximum PRO content observed (16.43 mg/g) in MLE (SS) while minimum content (9.44 mg/g) observed under MLE (Con) (Fig. 3B). MLE was predominately the best treatment under SS improving RFP content by about 48.33%. Furthermore, TU and PRO increased root-free PRO by 40.61% and 39.52%, respectively, under control conditions (Fig. 3B).
*NFS = no foliar spray, H2O = water spray, SA = salicylic acid (foliar spray), TU = thiourea (foliar spray), PRO = proline (foliar spray), MLE = moringa leaf extract (foliar spray).
Different alphabets/letters on bars represent statistically significant interactions (P < 0.05) according to the least significant difference test. Bars lacking the labels show non-significant interactions at P < 0.05.
Oxidative Stress Determination
Data pertaining to shoot H2O2 showed statistically significant results (P < 0.05). The highest content of H2O2 in the shoot was observed (91.19 µmol/g) under NFS (SS), while the lowest was observed (50.43 µmol/g) under H2O (Con). H2O2 concentration in the shoot decreased under foliar supplementation of PGRs by about 14.50% to 17.28% under control and 4.09%–43.02% under salinity stress, thereby protecting membrane integrity by reducing the generation of reactive oxygen species (Fig. 4A).
Figure 4: Changes in oxidative-stress parameters. A = shoot H2O2, B = root H2O2, C = shoot MDA, D = root MDA content of Plantago ovata as affected by salinity stress. Plants were foliar-treated with different PGRs.
Data obtained for root H2O2 concentration of P. ovata under salinity stress reported statistically significant results (P < 0.05) (Figs. 4A and 4B). Root H2O2 content was the highest (90.78 µmol/g) for NFS (SS), and the lowest (47.37 µmol/g) in PRO (Con) treated plants. Under the foliar supplementation of PGRs, root H2O2 decreased by 12.04%–27.05% under control and about 1.87%–23.47% under salinity stress (Fig. 4B).
The variations observed in both root and shoot H2O2 content in plantago revealed no such high degree of differentiation among root and shoot H2O2 contents, although, under salinity stress, H2O2 has been reported to account for a high content in both roots and shoot as compared to the control plants. In comparison to NFS salinity-treated plants, the concentration of H2O2 decreased under the foliar application of PGRs (notably PRO and SA), indicating that PGRs have a very beneficial role in alleviating or decreasing the negative effects of salinity by fulfilling the criteria for better plant growth and development in term of its morpho-physiological attributes (Figs. 4A and 4B).
Data recorded for both shoot and root MDA content of plantago under salinity stress showed significant results statistically (P < 0.05). The highest MDA content (642.83 mmol/g) was observed in NFS (SS), and the least amount of MDA (234.83 mmol/g) was observed in SA (Con). A decrease of about 18.43%–37.41% was observed under the control condition and about 2.89%–36.56% under salinity stress with PGRs supplementation (Fig. 4D).
Data obtained for shoot MDA content also showed statistically significant (P < 0.05) results; the maximum content of MDA was recorded (614.19 mmol/g) in NFS (SS), and the minimum MDA content in shoots (214.70 mmol/g) was observed in TU-treated (Con) plants (Fig. 4C). Shoot MDA content astonishingly decreased under salinity stress under the application of different PGRs, i.e., about 3.17%–40.33%.
The shoot MDA content of P. ovata plants was affected by salinity stress; however, the application of TU and PRO helped to reduce the effects of salinity stress. However, MDA content in the root was comparatively higher than in the shoot. The synergistic effects of PGRs were very helpful in alleviating the salinity stress in P. ovata by decreasing MDA content (Figs. 4C and 4D).
*NFS = no foliar spray, H2O = water spray, SA = salicylic acid (foliar spray), TU = thiourea (foliar spray), PRO = proline (foliar spray), MLE = moringa leaf extract (foliar spray), PGRs = plant growth regulators.
Different alphabets/letters on bars represent statistically significant interactions (P < 0.05) according to the least significant difference test.
The present study was conducted to explore the role of potentially important PGRs, i.e., SA, PRO, TU, and MLE, on the physiological performance of P. ovata under salinity stress. Salinity is deleterious as it hampers the growth and development of plants primarily by affecting photosynthetic activity (Zahra et al., 2021b; Saddiq et al., 2021a; Saddiq et al., 2021b.). Various researchers document that salinity significantly leads to a reduction in Chl contents in numerous plant species, including wheat (Saddiq et al., 2021b), camelina (Huang et al., 2021), and pepper (Sarwar et al., 2022). The rate of electron transport and activity of PSII was also inhibited under NaCl-treated cucumber (Zhang et al., 2022). Our results agree with the study of Yadav et al. (2020), who observed that salinity treatment particularly reduces the photosynthetic pigments; however, when PGRs, especially PRO and TU, were applied in this study, the contents of photosynthetic pigments (Chl a, b, and carotenoids) increased under salinity stress (Figs. 1A and 1C). Similar findings were documented (Zahra et al., 2021a), wherein the application of PGRs profoundly impacted the content of photosynthetic pigments in plants under saline stress. Thus, the present study further validates that Chl a, b, and carotenoids content increases by foliar supplementation of PGRs under salinity stress (Figs. 1A and 1C), thereby improving/protecting the photosynthetic efficiency of the plant (Koo et al., 2020).
Various studies have documented that plant species vary in their strategy to cope with stress, e.g., salinity stress (Flowers and Colmer, 2008; Raza et al., 2022a); in many plants, the compatible osmoprotectants, i.e., (PRO and soluble sugar) are produced to protect the cells against adverse impacts of salt stress, particularly, high accumulation of PRO is associated with stress tolerance (Rady et al., 2018; Wang et al., 2017). The fundamental mechanisms of SA-induced tolerance of abiotic stress involve SA-mediated PRO, GB, amines, and soluble sugars, which are highly helpful in maintaining the osmotic homeostasis and improving the production of secondary metabolites, such as terpenes, phenolics, nitrogen, and sulfur compounds (Koo et al., 2020; Sabagh et al., 2021). The present study showed that the concentration of soluble sugar in the shoot was the highest under salinity stress as compared to that in the roots. This increase in the amount of soluble sugar was due to the role of PGRs that effectively hampered salinity stress (Figs. 2C and 2D). Further, the osmoprotectants, free PRO, i.e., endogenous PRO both in shoot and root, increased with the foliar supplementation of MLE under salinity stress (Fig. 3B). Thus, this study proves the potential novelty of MLE, which is the best source to cope with salinity stress and facilitates better growth and development of plants in terms of physiological attributes (Zahra et al., 2021a).
Phenolic compounds are highly recognized as secondary metabolites, which play a crucial role in the protection of plants against oxidative stress. Enhanced phenolic compound synthesis is reported to be one of the most significant defensive strategies that help plants to tolerate stress (Kumar et al., 2020). Parida et al. (2004) documented a significant increase in phenolic compounds along with flavonoids content in Aegiceras corniculatum at moderate and at maximum salinity levels, indicating the significant role of such compounds in a relationship with carotenoids by showing enhanced tolerance to salinity stress. However, in the present study, the contents of anthocyanins in both root and shoot were enhanced by the application of TU under salinity stress, and the content was higher in the as compared to roots (Figs. 2A and 2B). Similarly, the phenolic content was also higher in shoots as compared to the roots. The highest content of soluble phenolics was observed under SA and PRO foliar spray in shoots and roots, respectively, of salt-stressed plants proving the protective role of these PGRs in saline-stressed plants (Figs. 2E and 2F).
The toxicity of Na+ leads to ROS production, which also increases the damage to the membrane due to lipid peroxidation (Ali et al., 2021) and the MDA formation is the final product of lipid peroxidation during oxidative stress (Yadav et al., 2020). A minimum concentration of MDA implies protection against oxidative stress, which is also correlated with a maximum increase in the antioxidant activity in plants (Koca et al., 2007). Under stressful conditions, antioxidant enzymes, i.e., ascorbate peroxidase and catalase are reported to protect the cellular structures from oxidative stress via ROS scavenging, thereby enhancing stress tolerance (Hasanuzzaman et al., 2020). Abdelaziz et al. (2018) reported the difference in concentration of MDA in rice cultivars under salinity stress, while our results suggest that under the essential role of different PGRs, such as TU and PRO, the concentration of MDA decreases. The present study further documents significant results by demonstrating that MDA content in P. ovata increased in the roots and was higher as compared to that in the shoot, thereby suggesting that the roots are exposed to a severe influx of salt (Figs. 4C and 4D). Correspondingly, H2O2 accumulation was also higher in roots as compared to the shoot, but PGRs played a potential role in minimizing the impacts of salinity in both (root and shoot) (Figs. 4A and 4B). For these oxidative parameters, PRO was the most effective treatment for hampering the concentration/synthesis of ROS under salt stress. Thus, foliar supplementation of PGRs can be recommended to enhance the tolerance potential of plants under salinity stress.
To conclude, salinity adversely affects physio-biochemical attributes; however, when plants are foliar supplemented with PGRs (such as SA, MLE, PRO, and TU), the impacts of salinity stress is alleviated by improving the content of photosynthetic pigments and enhancing the accumulation of various metabolites thereby improving the stress-tolerance potential of plants. Thus, exogenous supplementation, particularly foliar supplementation, can be recommended as an effective strategy to help plants to cope with the adverse impacts of salinity stress. Furthermore, omics approaches are needed to explore the tolerance genes in various medicinal plants growing on saline soils.
Acknowledgement: The authors are thankful to all members of the Department of Botany, University of Balochistan, Quetta, Pakistan, for providing a research environment and support to conduct this study.
Availability of Data and Materials: The datasets generated during and/or analyzed during the current study are available from the corresponding author on reasonable request.
Author Contribution: The authors confirm their contribution to the paper as follows: study conception and design: K.S.; data collection: A.S., K.S., and M.N.; analysis and interpretation of results: K.S., A.S., A.R.A., A.R., and N.Z. draft manuscript preparation, K.S., A.S., M.N., M.R.A., N.Z., A.R., A.N., H.M.W.I., V.P., and I.D. All authors reviewed the results and approved the final version of the manuscript.
Ethics Approval: Not applicable.
Funding Statement: This research was supported by the Ministry of Education, Science and Technological Development of the Republic of Serbia, Grant No. 451–03–68/2022–124/200032.
Conflicts of Interest: The authors declare that they have no conflicts of interest to report regarding the present study.
References
Abdelaziz MN, Xuan TD, Mekawy AM, Wang H, Khanh TD (2018). Relationship of salinity tolerance to Na+ exclusion, proline accumulation, and antioxidant enzyme activity in rice seedlings. Agriculture 8: 166. DOI 10.3390/agriculture8110166. [Google Scholar] [CrossRef]
Ahmad N, Virk AL, Hussain S, Hafeez MB, Haider FU, Rehmani MIA, Yasir TA, Asif A (2022). Integrated application of plant bioregulator and micronutrients improves crop physiology, productivity and grain biofortification of delayed sown wheat. Environmental Science and Pollution Research 29: 52534–52543. DOI 10.1007/s11356-022-19476-5. [Google Scholar] [CrossRef]
Ali M, Afzal S, Parveen A, Kamran M, Javed MR, Abbasi GH, Malik Z, Riaz M, Ahmad S, Chattha MS (2021). Silicon mediated improvement in the growth and ion homeostasis by decreasing Na+ uptake in maize (Zea mays L.) cultivars exposed to salinity stress. Plant Physiology and Biochemistry 158: 208–218. DOI 10.1016/j.plaphy.2020.10.040. [Google Scholar] [CrossRef]
Arnon DI (1949). Copper enzymes in isolated chloroplasts. Polyphenoloxidase in Beta vulgaris. Plant Physiology 24: 1–15. DOI 10.1104/pp.24.1.1. [Google Scholar] [CrossRef]
Aslam MF, Basra S, Hafeez MB, Khan S, Irshad S, Iqbal S, Saqqid MS, Akram MZ (2020). Inorganic fertilization improves quality and biomass of Moringa oleifera L. Agroforestry Systems 94: 975–983. DOI 10.1007/s10457-019-00464-7. [Google Scholar] [CrossRef]
Bates LS, Waldren RP, Teare I (1973). Rapid determination of free proline for water-stress studies. Plant and Soil 39: 205–207. DOI 10.1007/BF00018060. [Google Scholar] [CrossRef]
Corpas FJ, González-Gordo S, Rodríguez-Ruiz M, Munoz-Vargas MA, Palma Martínez JM (2022). Nitric oxide and hydrogen sulfide share regulatory functions in higher plant events. BIOCELL 46: 1–5. DOI 10.32604/biocell.2022.017300. [Google Scholar] [CrossRef]
Dai F, Rong Z, Wu Q, Abduallah EF, Liu C, Shengrui L (2022). Mycorrhiza improves plant growth and photosynthetic characteristics of tea plants in response to drought stress. BIOCELL 46: 1339–1346. DOI 10.32604/biocell.2022.018909. [Google Scholar] [CrossRef]
Ferdosi MFH, Shoaib A, Habib S, Khan KA (2021). Modulation of salt-induced stress impact in Gladiolus grandiflorus L. by exogenous application of salicylic acid. Scientific Reports 11: 1–13. DOI 10.1038/s41598-021-95243-9. [Google Scholar] [CrossRef]
Flowers TJ, Colmer TD (2008). Salinity tolerance in halophytes. New Phytologist, 945–963. DOI 10.1111/j.1469-8137.2008.02531.x. [Google Scholar] [CrossRef]
Franco EAN, Sanches-Silva A, Ribeiro-Santos R, de Melo NR (2020). Psyllium (Plantago ovata ForsskFrom evidence of health benefits to its food application. Trends in Food Science and Technology 96: 166–175. DOI 10.1016/j.tifs.2019.12.006. [Google Scholar] [CrossRef]
Granaz, Shaukat K, Baksh G, Zahra N, Hafeez MB, Raza A, Samad A, Nizar M, Wahid A (2022). Foliar application of thiourea, salicylic acid, and kinetin alleviate salinity stress in maize grown under etiolated and de-etiolated conditions. Discover Food 2: 1–14. DOI 10.1007/s44187-022-00027-3. [Google Scholar] [CrossRef]
Hafeez MB, Raza A, Zahra N, Shaukat K, Akram MZ, Iqbal S, Basra SMA (2021). Gene regulation in halophytes in conferring salt tolerance. In: Handbook of Bioremediation. pp. 341–370. DOI 10.1016/B978-0-12-819382-2.00022-3. [Google Scholar] [CrossRef]
Hasanuzzaman M, Bhuyan M, Zulfiqar F, Raza A, Mohsin SM, Mahmud JA, Fujita M, Fotopoulos V (2020). Reactive oxygen species and antioxidant defense in plants under abiotic stress: Revisiting the crucial role of a universal defense regulator. Antioxidants 9: 681. DOI 10.3390/antiox9080681. [Google Scholar] [CrossRef]
Heath RL, Packer L (1968). Photoperoxidation in isolated chloroplasts: I. Kinetics and stoichiometry of fatty acid peroxidation. Archives of Biochemistry and Biophysics 125: 189–198. DOI 10.1016/0003-9861(68)90654-1. [Google Scholar] [CrossRef]
Huang P, He L, Abbas A, Hussain S, Hussain S, Du D, Hafeez MB, Balooch S, Zahra N, Ren X (2021). Seed priming with sorghum water extract improves the performance of camelina (Camelina sativa (L.) crantz.) under salt stress. Plants 10: 749. DOI 10.3390/plants10040749. [Google Scholar] [CrossRef]
Iqbal S, Basra SM, Afzal I, Wahid A, Saddiq MS, Hafeez MB, Jacobsen SE (2019). Yield potential and salt tolerance of quinoa on salt-degraded soils of Pakistan. Journal of Agronomy and Crop Science 205: 13–21. DOI 10.1111/jac.12290. [Google Scholar] [CrossRef]
Julkunen-Tiitto R (1985). Phenolic constituents in the leaves of northern willows: Methods for the analysis of certain phenolics. Journal of Agricultural and Food Chemistry 33: 213–217. DOI 10.1021/jf00062a013. [Google Scholar] [CrossRef]
Khan S, Basit A, Hafeez MB, Irshad S, Bashir S, Bashir S, Maqbool MM, Saddiq MS, Hasnain Z, Aljuaid BS (2021). Moringa leaf extract improves biochemical attributes, yield and grain quality of rice (Oryza sativa L.) under drought stress. PLoS One 16: e0254452. DOI 10.1371/journal.pone.0254452. [Google Scholar] [CrossRef]
Kirk J (1967). Studies on the dependence of chlorophyll synthesis on protein synthesis in Euglena gracilis, together with a nomogram for determination of chlorophyll concentration. Planta 78: 200–207. DOI 10.1007/BF00406651. [Google Scholar] [CrossRef]
Koca H, Bor M, Özdemir F, Türkan İ (2007). The effect of salt stress on lipid peroxidation, antioxidative enzymes and proline content of sesame cultivars. Environmental and Experimental Botany 60: 344–351. DOI 10.1016/j.envexpbot.2006.12.005. [Google Scholar] [CrossRef]
Koo YM, Heo AY, Choi HW (2020). Salicylic acid as a safe plant protector and growth regulator. The Plant Pathology Journal 36: 1–10. DOI 10.5423/PPJ.RW.12.2019.0295. [Google Scholar] [CrossRef]
Kumar S, Abedin M, Singh AK, Das S (2020). Role of phenolic compounds in plant-defensive mechanisms. Plant Phenolics in Sustainable Agriculture 1: 517–532. DOI 10.1007/978-981-15-4890-1. [Google Scholar] [CrossRef]
Mir RA, Bhat BA, Yousuf H, Islam ST, Raza A, Rizvi MA, Charagh S, Albaqami M, Sofi PA, Zargar SM (2022). Multidimensional role of silicon to activate resilient plant growth and to mitigate abiotic stress. Frontiers in Plant Science 13: 819658. DOI 10.3389/fpls.2022.819658. [Google Scholar] [CrossRef]
Mubarik MS, Khan SH, Sajjad M, Raza A, Hafeez MB, Yasmeen T, Rizwan M, Ali S, Arif MS (2021). A manipulative interplay between positive and negative regulators of phytohormones: A way forward for improving drought tolerance in plants. Physiologia Plantarum 172: 1269–1290. DOI 10.1111/ppl.13325. [Google Scholar] [CrossRef]
Mundada PS, Jadhav SV, Salunkhe SS, Gurme ST, Umdale SD, Nikam TD, Ahire ML (2021). Plant performance and defensive role of proline under environmental stress. In: Plant performance under Environmental Stress, pp. 201–223. Springer, Cham. [Google Scholar]
Munns R, Tester M (2008). Mechanisms of salinity tolerance. Annual Review of Plant Biology 59: 651–681. DOI 10.1146/annurev.arplant.59.032607.092911. [Google Scholar] [CrossRef]
Parida AK, Das AB, Sanada Y, Mohanty P (2004). Effects of salinity on biochemical components of the mangrove, Aegiceras corniculatum. Aquatic Botany 80: 77–87. DOI 10.1016/j.aquabot.2004.07.005. [Google Scholar] [CrossRef]
Patade VY, Nikalje GC, Srivastava S (2020). Role of thiourea in mitigating different environmental stresses in plants. In: Protective Chemical agents in the Amelioration of Plant Abiotic Stress: Biochemical and Molecular Perspectives, pp. 467–482. Wiley Blackwell, UK. [Google Scholar]
Rady MO, Semida WM, Abd El-Mageed TA, Hemida KA, Rady MM (2018). Up-regulation of antioxidative defense systems by glycine betaine foliar application in onion plants confer tolerance to salinity stress. Scientia Horticulturae 240: 614–622. DOI 10.1016/j.scienta.2018.06.069. [Google Scholar] [CrossRef]
Rashad RT, Hussien RA (2014). A comparison study on the effect of some growth regulators on the nutrients content of maize plant under salinity conditions. Annals of Agricultural Sciences 59: 89–94. DOI 10.1016/j.aoas.2014.06.013. [Google Scholar] [CrossRef]
Raza A, Tabassum J, Fakhar AZ, Sharif R, Chen H et al. (2022a). Smart reprograming of plants against salinity stress using modern biotechnological tools. Critical Reviews in Biotechnology 12: 1–28. DOI 10.1080/07388551.2022.2093695. [Google Scholar] [CrossRef]
Raza A, Salehi H, Rahman MA, Zahid Z, Madadkar Haghjou M et al. (2022b). Plant hormones and neurotransmitter interactions mediate antioxidant defenses under induced oxidative stress in plants. Frontiers in Plant Science 13: 961872. DOI 10.3389/fpls.2022.961872. [Google Scholar] [CrossRef]
Sabzehzari M, Hoveidamanesh S, Modarresi M, Mohammadi V (2019). Morphological, anatomical, physiological, and cytological studies in diploid and tetraploid plants of Plantago psyllium. Plant Cell, Tissue and Organ Culture 139: 131–137. DOI 10.1007/s11240-019-01670-y. [Google Scholar] [CrossRef]
Sabagh AE, Mbarki S, Hossain A, Iqbal MA, Islam MS et al. (2021). Potential role of plant growth regulators in administering crucial processes against abiotic stresses. Frontiers in Agronomy 3: 648694. DOI 10.3389/fagro.2021.648694. [Google Scholar] [CrossRef]
Saddiq MS, Iqbal S, Afzal I, Ibrahim AM, Bakhtavar MA, Hafeez MB, Jahanzaib, Maqbool MM (2019). Mitigation of salinity stress in wheat (Triticum aestivum L.) seedlings through physiological seed enhancements. Journal of Plant Nutrition 42: 1192–1204. DOI 10.1080/01904167.2019.1609509. [Google Scholar] [CrossRef]
Saddiq MS, Iqbal S, Hafeez MB, Ibrahim AM, Raza A, Fatima EM, Baloch H, Woodrow P, Ciarmiello LF (2021a). Effect of salinity stress on physiological changes in winter and spring wheat. Agronomy 11: 1193. DOI 10.3390/agronomy11061193. [Google Scholar] [CrossRef]
Saddiq MS, Afzal I, Iqbal S, Hafeez MB, Raza A (2021b). Low leaf sodium content improves the grain yield and physiological performance of wheat genotypes in saline-sodic soil. Pesquisa Agropecuária Tropical 51. DOI 10.1590/1983-40632021v5167663. [Google Scholar] [CrossRef]
Sarwar M, Anjum S, Alam MW, Ali Q, Ayyub C, Haider MS, Ashraf MI, Mahboob W (2022). Triacontanol regulates morphological traits and enzymatic activities of salinity affected hot pepper plants. Scientific Reports 12: 1–8. DOI 10.1038/s41598-022-06516-w. [Google Scholar] [CrossRef]
Shah AR, Sharma P, Longvah T, Gour VS, Kothari S, Shah YR, Ganie SA (2020). Nutritional composition and health benefits of psyllium (Plantago ovata) husk and seed. Nutrition Today 55: 313–321. DOI 10.1097/NT.0000000000000450. [Google Scholar] [CrossRef]
Shaukat K, Zahra N, Hafeez MB, Naseer R, Batool A, Batool H, Raza A, Wahid A (2022). Role of salicylic acid–induced abiotic stress tolerance and underlying mechanisms in plants. In: Emerging Plant Growth Regulators in Agriculture, pp. 73–98. DOI 10.1016/B978-0-323-91005-7.00008-4. [Google Scholar] [CrossRef]
Shrivastava M, Kumar S, Yadav S, Manasvini KL (2019). Deficiency and toxicity of trace elements in salt-affected soils. Indian Society of Soil Salinity and Water Quality (Registered under Societies Registration Act XXI of 1860) Editorial Board 11: 31–44. [Google Scholar]
Stark D, Wray V (1989). Anthocyanins. In: Methods in Plant Biology; Plant Phenolics. London: Academic Press. [Google Scholar]
Tabssum F, uz Zaman Q, Chen Y, Riaz U, Ashraf W, Aslam A, Ehsan N, Nawaz R, Aziz H (2019). Exogenous application of proline improved salt tolerance in rice through modulation of antioxidant activities. Pakistan Journal of Agricultural Research 32: 140–151. DOI 10.17582/journal.pjar/2019/32.1.140.151. [Google Scholar] [CrossRef]
Velikova V, Yordanov I, Edreva A (2000). Oxidative stress and some antioxidant systems in acid rain-treated bean plants: Protective role of exogenous polyamines. Plant Science 151: 59–66. DOI 10.1016/S0168-9452(99)00197-1. [Google Scholar] [CrossRef]
Wang Y, Gu W, Meng Y, Xie T, Li L, Li J, Wei S (2017). γ-Aminobutyric acid imparts partial protection from salt stress injury to maize seedlings by improving photosynthesis and upregulating osmoprotectants and antioxidants. Scientific Reports 7: 1–13. DOI 10.1038/srep43609. [Google Scholar] [CrossRef]
Yadav T, Kumar A, Yadav R, Yadav G, Kumar R, Kushwaha M (2020). Salicylic acid and thiourea mitigate the salinity and drought stress on physiological traits governing yield in pearl millet-wheat. Saudi Journal of Biological Sciences 27: 2010–2017. DOI 10.1016/j.sjbs.2020.06.030. [Google Scholar] [CrossRef]
Yoshida S, Coronel V (1976). Nitrogen nutrition, leaf resistance, and leaf photosynthetic rate of the rice plant. Soil Science and Plant Nutrition 22: 207–211. DOI 10.1080/00380768.1976.10432983. [Google Scholar] [CrossRef]
Zahra N, Wahid A, Hafeez MB, Shaukat K, Shahzad S, Shah T, Alyemeni MN (2021a). Plant growth promoters alleviate oxidative damages and improve the growth of milk thistle (Silybum marianum L.) under salinity stress. Journal of Plant Growth Regulation 41: 3091–3116. DOI 10.1007/s00344-021-10498-w. [Google Scholar] [CrossRef]
Zahra N, Wahid A, Hafeez MB, Alyemeni MN, Shah T, Ahmad P (2021b). Plant growth promoters mediated quality and yield attributes of milk thistle (Silybum marianum L.) ecotypes under salinity stress. Scientific Reports 11: 1–17. DOI 10.1038/s41598-021-02435-4. [Google Scholar] [CrossRef]
Zahra N, Al Hinai MS, Hafeez MB, Rehman A, Wahid A, Siddique KH, Farooq M (2022). Regulation of photosynthesis under salt stress and associated tolerance mechanisms. Plant Physiology and Biochemistry 178: 55–69. DOI 10.1016/j.plaphy.2022.03.003. [Google Scholar] [CrossRef]
Zhang X, Zhang L, Ma C, Su M, Wang J, Zheng S, Zhang T (2022). Exogenous strigolactones alleviate the photosynthetic inhibition and oxidative damage of cucumber seedlings under salt stress. Scientia Horticulturae 297: 110962. DOI 10.1016/j.scienta.2022.110962. [Google Scholar] [CrossRef]
Cite This Article
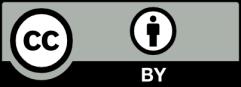
This work is licensed under a Creative Commons Attribution 4.0 International License , which permits unrestricted use, distribution, and reproduction in any medium, provided the original work is properly cited.